DOI:
10.1039/C7QM00453B
(Research Article)
Mater. Chem. Front., 2018,
2, 296-299
Aggregation-responsive ON–OFF–ON fluorescence-switching behaviour of twisted tetrakis(benzo[b]furyl)ethene made by hafnium-mediated McMurry coupling†
Received
3rd October 2017
, Accepted 17th November 2017
First published on 27th November 2017
Abstract
Fluorescence-switching dyes serve as important tools for bioimaging and environment sensing. Herein, we report a new type of molecule, tetrakis(benzo[b]furyl)ethene (TBFE), that undergoes two-wavelength ON–OFF–ON three-stage fluorescence switching triggered by aggregation. This compound features a sterically hindered tetrasubstituted ethene, made available by a hafnium-mediated McMurry coupling reaction. A fluorescent band of TBFE at 360 nm in THF disappears upon dilution with water towards 40% water in THF, giving way to a new peak at 510 nm. This switching behaviour is attributed to the combination of THF/H2O ratio-dependent aggregation-caused quenching and aggregation-induced emission, as supported by the change of size distribution measured by dynamic light scattering and scanning electron microscopy.
Introduction
Environment-responsive fluorescent materials have attracted attention due to their chemical properties by themselves and for applications to bioimaging1,2 and environmental sensing.3,4 Aggregation-responsive dyes have been most widely studied for their fluorescence-switching property, and previous studies have focused on two-stage on–off switching, caused by either aggregation-caused quenching (ACQ) or aggregation-induced emission (AIE).5 The former mechanism is generally found in planar π molecules,6 while the latter in molecules with rotatable or vibratory units, such as tetraarylethenes (TAEs).4,5 Three-stage switching7 involving a continuous change of fluorescence has also been achieved by a combination of intramolecular charge transfer (ICT) and AIE.
Herein, we report a two-wavelength ON–OFF–ON fluorescence-switching behaviour of a new type of molecule, tetrakis(benzo[b]furyl)ethene (TBFE), triggered by aggregation (Fig. 1a). This compound features a sterically hindered tetrasubstituted ethene (Fig. 1b), made available by a hafnium-mediated McMurry coupling reaction. A fluorescence band of TBFE at 360 nm in THF disappears upon dilution with water towards 40% water in THF, giving way to a new peak at 510 nm (Fig. 1c). The intensity of the 360 nm fluorescence at 0% water weakens by 3.4 times at 40% water in THF, where the 510 nm fluorescence appears and its intensity increases by 9.8 times at 90% water. This switching behaviour is attributed to the combination of the ACQ effect of the 360 nm fluorescence and the AIE effect of the 510 nm fluorescence, as supported by the change of size distribution measured by dynamic light scattering (DLS) and scanning electron microscopy (SEM). This compound features a TAE as an AIE-phore framework (Fig. 1a, blue) and a benzofuran (BF) as a fluorescent unit (red), a class of fused furan compounds with high fluorescence quantum yield in solution and even in the solid state in some π-expanded molecules.8 A key synthetic enabler of this research is the use of hafnium (Hf) for the McMurry coupling, which we found useful for the synthesis of the sterically congested TAE core.
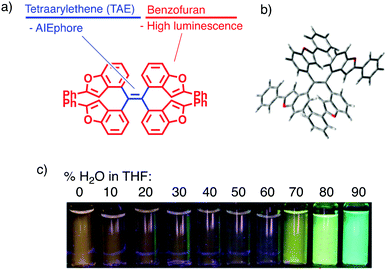 |
| Fig. 1 Tetrakis(benzo[b]furyl)ethene (TBFE). (a) Molecular design. (b) Crystal structure showing twisting around the TAE centre (see ESI†). (c) Fluorescence intensity dependent on THF/H2O ratio (excitation at 365 nm). | |
Results and discussion
Synthesis of TBFE
A retrosynthesis of TBFE calls for the deoxygenative McMurry-type coupling of two aryl ketone molecules (7 in Scheme 1). To this end, we first prepared benzofuran 5 from a commercially available 3-bromophenol (1) in four steps via a procedure reported by Sanz et al.9 Lithiation of 5 and nucleophilic addition of the resulting aryllithium to methyl formate gave alcohol 6. Oxidation by MnO2 afforded ketone 7. The subsequent McMurry-type reductive coupling reaction of 7 was effectively promoted using ZrCl4 or HfCl4 with metallic Zn to obtain TBFE in 42% and 50% yields, respectively, whereas no reaction occurred with the use of the corresponding Ti reagent (Table 1). The use of zinc as a reducing reagent was inspired by Mukaiyama's protocol, where a combination of TiCl4 and Zn was used.10
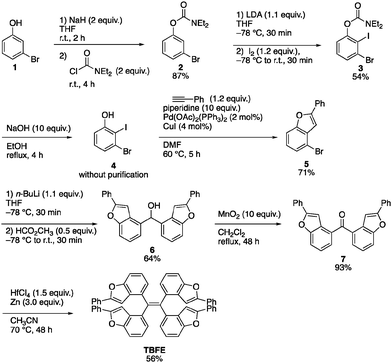 |
| Scheme 1 Synthesis of TBFE. | |
Table 1 Group 4 metal-mediated McMurry-type coupling reactions of compound 7
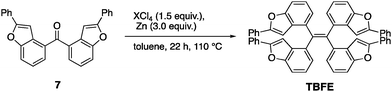
|
XCl4 |
r
(pm) |

(V) |
Yield (%) |
Atomic radius of metal.
Standard electrode potential of group 4 metals.13
|
TiCl4 |
148 |
−1.37 |
0 |
ZrCl4 |
164 |
−1.45 |
42 |
HfCl4 |
164 |
−1.55 |
50 |
The high efficacy of the hafnium-mediated McMurry coupling was rather unexpected because of publications reporting the contrary.11,12 We attribute the difference in efficacy among Ti, Zr, and Hf to the difference of atomic radius and/or the reducing ability of low-valent metal states. Zr and Hf with a larger atomic radius than Ti may be able to circumvent a steric problem encountered in the conventional Ti-mediated coupling known for its sluggishness upon the application to bulky ketones.10,11 An alternative possibility includes the larger standard electrode potentials (M4+/M) of Hf and Zn than Ti,13 which provides a larger driving force for this deoxygenative reaction.
Optimized structure and UV absorption
Geometry optimization of TBFE at the B3LYP level of theory using the 6-31G* basis set showed that there are four stable conformational isomers within 4.28 kJ mol−1 (Fig. 1b and ESI,† Fig. S1–S5). In the most stable and hence most populated conformer, the 2-phenylbenzo[b]furan (BF) unit and the alkenyl C
C double bond of the alkene are twisted by 87.8° to each other. This optimized structure indicates that the π-conjugations of the BF unit have little electronic interaction with each other in the ground state, which accounts for the similarity of the absorption spectrum of TBFE in THF (Fig. 2a) to that of BF.
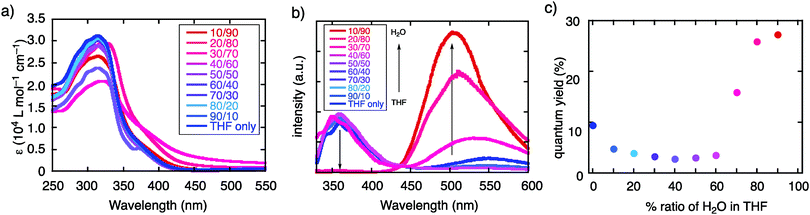 |
| Fig. 2 Photophysical properties of TBFE in a THF/H2O mixed solvent system. (a) Absorption spectra (1.0 × 10−5 M), (b) fluorescence spectra (1.0 × 10−6 M, λex = 317 nm), and (c) transition of photoluminescence quantum yield measured by the absolute method. | |
Fluorescence switching in THF/H2O
TBFE shows two-wavelength ON–OFF–ON switching behaviour of its fluorescence in a THF/H2O mixed-solvent system (Fig. 1c and 2b, c), while the maximum absorption wavelength around 317 nm remains unchanged for THF/H2O volume ratios from 0% to 90% (Fig. 2a). In pure THF (Fig. 2b, blue line and Fig. 2c, blue dot), we see a fluorescence peak at 360 nm due to emission from the BF moieties (ESI,† Fig. S7) with a fluorescence quantum yield (ΦFL) of 0.094. Upon gradual dilution with H2O, the intensity of the emission band decreases to ΦFL = 0.028 at 40% water. At 70% water (purple dot in Fig. 2c), however, a new peak appears around 530 nm with ΦFL of 0.159, which undergoes a gradual blueshift and intensifies as the H2O ratio is increased. At a 90% H2O ratio, a well-defined signal at 503 nm appears with ΦFL = 0.263, accompanied by a slight blueshift to 503 nm. At this ratio, the peak at 360 nm was not seen at all; TBFE precipitates in pure water.
We surmise that this ON–OFF–ON switching behaviour originates from the aggregation of TBFE, which causes ACQ of the 360 nm fluorescence, and AIE of the 510 nm emission. There was no redshift of the 360 nm fluorescence band at 0% to 40% water, suggesting that an ICT process, which generally causes a redshift,7 is not responsible for the observed switching phenomenon. The lack of CT participation in the present switching phenomenon was suggested by a solvatochromic study. Thus, the fluorescence spectra of TBFE in polar and nonpolar solvents for a range of relative permittivities from 2.02 to 26 showed only a 10 nm variation of the fluorescence maxima (ESI,† Fig. S9), indicating also that the photoexcited states of TBFE are rather nonpolar.14
Fluorescence lifetime measurements in THF and THF/H2O mixed solvents (50, 70, 90% water) afforded further insights on the photophysical properties of TBFE. First, significantly slower nonradiative decay in the THF/H2O mixed solvents than in THF was observed, which is a signature of AIE processes.4,5,15 The nonradiative rate constant (knr) did not decrease by water addition in 0–50% water ratio. At 70% water, where the AIE effect was seen in the fluorescence spectra, a decrease in knr was observed as well. Further water addition dropped the knr at 90% water to about one-third of that in THF (ESI,† Table S10), calculated from their ΦFL and average lifetimes (〈τ〉), while the radiative rate constant (kr) did not change significantly. Second, the rather low fluorescence quantum yield of TBFE in THF compared with the parent BF in the same solvent is attributable to both larger knr and smaller kr of TBFE than those of BF. Interestingly, the knr value at 90% water where TBFE is aggregated was the same as that of BF in THF. The large knr value at 70% indicates that molecules are still not as densely aggregated as at 90% water. Time-dependent density functional theory calculations for each conformer of TBFE showed much smaller oscillator strengths for S0–S1 excitation than for BF (ESI,† Table S7). Third, the decay curves at 70–90% water were fitted with a biexponential function while the curves in pure THF and at 50% water were fitted with a monoexponential function. This difference in the fitting indicates that TBFE forms two major conformers in the aggregated state through suppression of intramolecular bond rotation to afford conformers that are energetically close to each other (Table 2).
Table 2 Fluorescence quantum yield ΦFL, average fluorescence lifetime 〈τ〉, radiative rate constant kr, and nonradiative rate constant knr of TBFE and BF
|
TBFE
|
BF |
In THF |
In THF/H2O (50/50) |
(30/70) |
(10/90) |
In THF |
Calculated from ΦFL and τ.
|
Φ
FL
|
0.094 |
0.050 |
0.159 |
0.273 |
0.656 |
〈τ〉 (ns) |
1.2 |
1.3 |
1.6 |
2.9 |
1.2 |
k
r (107 s−1)a |
7.8 |
3.8 |
9.7 |
9.5 |
55 |
k
nr (107 s−1)a |
75 |
72 |
51 |
25 |
29 |
Dynamic light scattering and scanning electron microscopy observation
The above spectral evidence of aggregation-induced changes was supported by direct observation of the aggregation behaviour by DLS and SEM. Thus, the observed size distribution of aggregates of TBFE in THF/H2O matched the switching behaviour of the fluorescence spectra, supporting the aggregation-induced mechanism that we are proposing here. In the low H2O ratio (0–50%), molecules are either dissolved unimolecularly or form aggregates made of several molecules, as indicated by the DLS size distribution observed in the region below 10 nm (Fig. 3a, bottom). When the H2O ratio reached 60%, the size distribution suddenly became larger than 103 nm. In the high H2O ratio region (>60%), the size decreased gradually from a maximum16 of 4.8 × 103 nm at 60% to 8.3 × 102 nm at 90% (Fig. 3a, top). This is probably because the hydrophobic TBFE aggregates more compactly in the aqueous solution due to surface tension.17 Hence, the side units of TBFE would not be sufficiently fixed, and its fluorescence spectrum was similar to that of the disaggregated state (in Fig. 2b and 3a, H2O ratio = 60%). SEM of the THF/H2O solution of TBFE spin coated on ITO supported the DLS observations. The sample in pure THF showed only amorphous domains on ITO (Fig. 3b), the sample in THF/H2O (10/90) showed spherical aggregates of 50–300 nm (Fig. 3c and ESI,† Fig. S12). This diameter matches the data from DLS. No sharp diffraction peak in the powder X-ray diffraction pattern of the TBFE aggregates in THF/H2O (10/90) indicates that the molecules are amorphous in the aggregates (ESI,† Fig. S14).
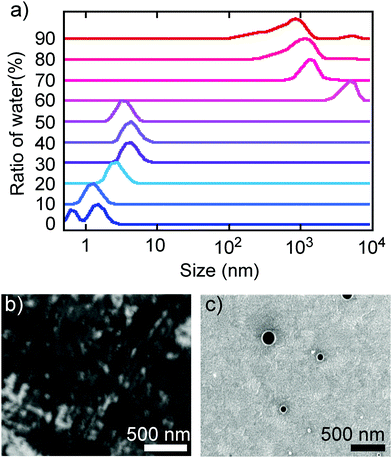 |
| Fig. 3 Aggregation behaviour of TBFE. (a) Size distribution in THF/H2O solvents obtained by DLS measurements. This figure was obtained as a correlation function (Fig. S11, ESI†) and outputted as a volume distribution. (b and c) SEM images of TBFE on ITO formed by spin-coating using THF and THF/H2O = 10/90, respectively. The scale bars are 500 nm. | |
Conclusion
In summary, we found an intriguing example of two-wavelength ON–OFF–ON three-stage fluorescence switching triggered by the aggregation for a new TAE derivative, TBFE, in a THF/H2O mixed-solvent system. The THF/H2O ratio-dependent change of size distribution found by DLS and SEM suggests that the fluorescence switching is caused by the combination of ACQ and AIE. The similarity of the photophysical properties of TBFE in THF to those of 2-phenylbenzo[b]furan and the absence of solvatochromic shift also support that ICT, which has been widely found in such switching phenomena, does not participate in the present system. The Hf-mediated McMurry-type coupling developed for the TBFE synthesis exploits the large atomic radius and high reduction capability of the Hf metal and will expand the synthetic scope of this classical method towards the synthesis of tetrasubstituted ethenes with bulky substituents.
Conflicts of interest
There are no conflicts to declare.
Acknowledgements
This work was supported by Grants-in-Aid for Scientific Research (no. 15H05754 for EN, and no. 15H00987 and 17H05163 to HT on Innovative Areas “π-System Figuration: Control of Electron and Structural Dynamism for Innovative Functions”).
Notes and references
-
(a) N. Fernándes-Suárez and A. Y. Ting, Nat. Rev. Mol. Cell Biol., 2008, 9, 929–943 CrossRef PubMed;
(b) G. D. Liang, J. W. Y. Lam, W. Qin, J. Li, N. Xie and B. Z. Tang, Chem. Commun., 2014, 50, 1725–1727 RSC.
- M. Irie, T. Fukaminato, K. Matsuda and S. Kobatake, Chem. Rev., 2014, 114, 12174–12277 CrossRef CAS PubMed.
-
(a) S. Sasaki, G. P. C. Drummen and G.-i. Konishi, J. Mater. Chem. C, 2016, 4, 2731–2743 RSC;
(b) S. Chen, Y. Hong, Y. Liu, J. Liu, C. W. T. Leung, M. Li, R. T. K. Kwok, E. Zhao, J. W. Y. Lam, Y. Yu and B. Z. Tang, J. Am. Chem. Soc., 2013, 135, 4926–4929 CrossRef CAS PubMed;
(c) Y. Hong, S. Chen, C. W. T. Leung, J. W. Y. Jam, J. Liu, N.-W. Tseng, R. T. K. Kwok, Y. Yu, Z. Wang and B. Z. Tang, ACS Appl. Mater. Interfaces, 2011, 3, 3411–3418 CrossRef CAS PubMed.
- J. Mei, Y. Hong, J. W. Y. Lam, A. Qin, Y. Tang and B. Z. Tang, Adv. Mater., 2014, 26, 5429–5479 CrossRef CAS PubMed.
- J. Mei, N. L. C. Leung, R. T. K. Kwok, J. W. Y. Lam and B. Z. Tang, Chem. Rev., 2015, 115, 11718–11940 CrossRef CAS PubMed.
- H. Tong, Y. Dong, Y. Hong, M. Häussler, J. W. Y. Lam, H. H.-Y. Sung, X. Yu, J. Sun, I. D. Williams, H. S. Kwok and B. Z. Tang, J. Phys. Chem. C, 2007, 111, 2287–2294 CAS.
-
(a) S. J. Ananhakrishnan, E. Varathan, E. Ravindran, N. Somanathan, V. Subramanian, A. B. Mandal, J. D. Sudha and R. Ramakrishnan, Chem. Commun., 2013, 49, 10742–10744 RSC;
(b) Y. Li, Y. Wu, J. Chang, J. Chen, L. Liu and F. Li, Chem. Commun., 2013, 49, 11335–11337 RSC;
(c) E. Wang, J. W. Y. Lam, R. Hu, C. Zhang, Y. S. Zhao and B. Z. Tang, J. Mater. Chem. C, 2014, 2, 1801–1807 RSC;
(d) R. Hu, E. Lager, A. Aguilar-Aguilar, J. Liu, J. W. Y. Lam, H. H. Y. Sung, I. D. Williams, Y. Zhong, K. S. Wong, E. Peña-Cabrera and B. Z. Tang, J. Phys. Chem. C, 2009, 113, 15845–15853 CrossRef CAS.
-
(a) N. Hayashi, Y. Saito, H. Higuchi and K. Suzuki, J. Phys. Chem. A, 2009, 113, 5342–5347 CrossRef CAS PubMed;
(b) N. Hayashi, Y. Saito and H. Higuchi, Heterocycles, 2009, 77, 1261–1268 CrossRef CAS;
(c) H. Tsuji and E. Nakamura, Acc. Chem. Res., 2017, 50, 396–406 CrossRef CAS PubMed.
- R. Sanz, M. P. Castroviejo, Y. Fernández and F. J. Fañanás, J. Org. Chem., 2005, 70, 6548–6551 CrossRef CAS PubMed.
- T. Mukaiyama, T. Sato and J. Hanna, Chem. Lett., 1973, 1041–1044 CrossRef CAS.
-
(a) J. E. McMurry, Chem. Rev., 1989, 89, 1513–1524 CrossRef CAS;
(b) M. Ephritikhine, Chem. Commun., 1998, 2549–2554 RSC.
- R. Dams, M. Malinowski and H. J. Geise, Bull. Soc. Chim. Belg., 1982, 91, 19–152 Search PubMed.
-
CRC Handbook of Chemistry and Physics, ed. W. M. Haynes, CRC Press, Boca Ration FL, 92nd edn, 2011, pp. 8-20–8-29 Search PubMed.
- S. Nigam and S. Rutan, Appl. Spectrosc., 2001, 55, 362–370 CrossRef.
- Y. Ren, J. W. Y. Lam, Y. Dong, B. Z. Tang and K. S. Wong, J. Phys. Chem. B, 2005, 109, 1135–1140 CrossRef CAS PubMed.
- K. Harano, T. Homma, Y. Niimi, M. Koshino, K. Suenaga, L. Leibler and E. Nakamura, Nat. Mater., 2012, 11, 877–881 CrossRef CAS PubMed.
- T. V. Chalikian, J. Phys. Chem. B, 2001, 105, 12566–12578 CrossRef CAS.
Footnote |
† Electronic supplementary information (ESI) available: Synthetic details, and geometry and measurement data. See DOI: 10.1039/c7qm00453b |
|
This journal is © the Partner Organisations 2018 |
Click here to see how this site uses Cookies. View our privacy policy here.