DOI:
10.1039/C7QM00528H
(Research Article)
Mater. Chem. Front., 2018,
2, 291-295
Imidazole and triazole head group-containing polydiacetylenes for colorimetric monitoring of pH and detecting HCl gas†
Received
15th November 2017
, Accepted 6th December 2017
First published on 6th December 2017
Abstract
The family of conjugated polymers known as polydiacetylenes (PDAs) has been extensively utilized in designing stimuli-responsive colorimetric and fluorometric sensors. In the current study, we have developed highly sensitive and rapidly responsive colorimetric chemosensors composed of PDAs bearing imidazole (PDA-Im) and triazole (PDA-Ta) head groups for the determination of pH and the detection of HCl gas. In solution, PDA-Im and PDA-Ta display distinct colorimetric changes from blue to red accordingly in response to pH changes. Also, a PDA-Im film was fabricated to detect HCl by a visibly observed blue-to-red color change.
Introduction
Owing to the significant role it plays in the environment and biology, acidity is an important value to measure both readily and accurately.1 In addition, HCl is a strong irritant to the eyes, nose and upper respiratory tract of humans because its dissolution in water yields a highly corrosive acidic solution. Also, exposure to >5 ppm of HCl gas is considered to be a workplace hazard.2 Among the chemosensors for gases that have been widely developed in recent years,3 colorimetric or fluorometric probes for hydrogen chloride gas are of particular interest because of the hazardous nature of this substance.4 Even though they have been actively explored, the development of colorimetric chemosensors for HCl gas faces considerable challenges. Specifically, sensor systems for the detection of this toxic and corrosive gas must be procedurally simple, economical and portable, and it is preferable that the readout can be visualized using the naked eye.5
One member of the class of conjugated polymers known as polydiacetylenes (PDAs) has been extensively explored as stimuli-responsive colorimetric and fluorometric sensors.6 PDAs, which are typically generated by UV irradiation of self-assembled diacetylene monomers (DAs), have a blue color (λmax ≈ 640 nm). A variety of stimuli induce a blue-to-red (λmax ≈ 550 nm) color transition in the PDAs. Depending on the nature of the head groups present in the PDAs, the stimuli promoting this change include temperature,7 metal ions,8 surfactants,9 anions,10 moisture,11 gas,12 bacteria,13 and others.14
From another perspective, imidazole and triazole derivatives, which have strong hydrogen bonding characters and respective pKa values of ca. ∼6.25 and ∼10.3 in water at 25 °C,15 undergo interesting spectroscopic changes upon protonation/deprotonation.16 Even though these compounds have been actively studied over the last decade,17 few efforts have been conducted on PDAs that possess imidazole and triazole head groups.8b,13a
In the study described below, we developed the PDA-based colorimetric chemosensors PDA-Im and PDA-Ta, which contain imidazole and triazole head groups, for monitoring pH changes and detecting HCl gas. In this effort, we showed that PDA-Im and PDA-Ta can be employed to monitor the pH changes in aqueous solutions by undergoing a distinct, visually observable blue-to-red color change (pKa = 4.06 and then 12.30) at pH values that correspond to the pKa values of the imidazole and triazole head groups. The results of UV-Vis absorption spectroscopic analysis show that the wavelength maximum in the spectrum of PDA-Im disappears in concert with a corresponding gradual increase in the appearance of an absorption peak at 530 nm as the pH of the solution decreases. The same principles were utilized for the detection of HCl gas with PDA-Im coated glass prepared by spin-coating and UV irradiation of the corresponding monomer. Exposure to HCl gas causes this film to undergo a blue-to-red color change within 3 s, and HCl gas can be detected at a volume of as low as 0.5 μL in a 100 mL chamber.
Results and discussion
Synthesis and polymerization of vesicles
The diacetylene monomers PCDA-Im and PCDA-Ta were synthesized by using a modification of the reported procedure.9b Specifically, 10,12-pentacosadiynoic acid (PCDA) reacted with N,N-dimethylethylenediamine in the presence of N,N′-dicyclohexylcarbodiimide to yield a succinimidyl ester derivative, which was then treated with 1-(3-aminopropyl)-imidazole to produce N-(3-(1H-imidazol-1-yl)propyl)pentacosa-10,12-diynamide (PCDA-Im) (58%). Alternatively, PCDA was treated with oxalyl chloride to generate the corresponding acid chloride, which was reacted with 3-amino-1,2,4-triazole to form N-(2H-1,2,3-triazol-4-yl)pentacosa-10,12-diynamide (PCDA-Ta) (97%) (Scheme S1, ESI†). PCDA-Im and PCDA-Ta were characterized by using 1H and 13C NMR spectroscopy and FAB high-resolution mass spectrometry (ESI†). The solutions of PDA-Ta and PCDA-Ta have a clear blue color. Inspection of scanning electron microscopy (SEM) and transmission electron microscopy (TEM) images (Fig. S5, ESI†) shows that self-assembled PDA-Im has a thin-film-like ribbon structure. The self-assemblies of PCDA-Im and PCDA-Ta were converted to the corresponding PDAs upon exposure to UV irradiation for 30 s and 120 s, respectively (Fig. 1).
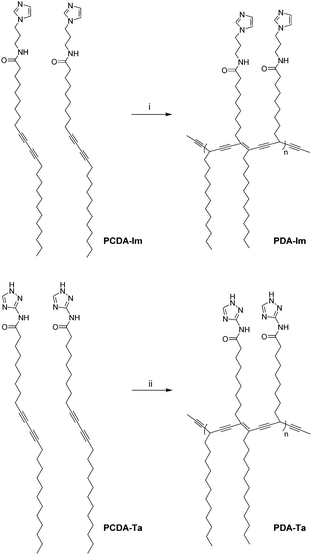 |
| Fig. 1 Self-assembly and polymerization of PCDA-Im, and PCDA-Ta. (i) Irradiation using a 4 W 254 nm UV lamp for 30 s. (ii) Irradiation using a 9 W 254 nm UV lamp for 120 s. | |
Colorimetric and fluorometric changes of PDA-Im and PDA-Ta in response to pH
As shown in Fig. 2, PDA-Im in water has a clear blue color and undergoes a blue-to-red color transition when the solution pH is lowered to below 5.0. Moreover, PDA-Ta undergoes a blue-to-red color transition when the pH is lower than 11.5. These color changes are a consequence of protonation of the imidazole and triazole head groups of the PDAs, which results in the production of charge–charge repulsive interactions that induce stress on the conjugated polymer backbone. Analysis of UV/visible absorption spectroscopic changes in an aqueous solution of PDA-Im (10 μM), promoted by decreasing the pH in the range of 7.8 to 2.2, shows that the original 650 nm absorption band of PDA-Im decreases simultaneously with an increase in a band at 530 nm. PDA-Ta displays similar absorption spectroscopic changes upon decreasing the pH from 13.0 to 6.0 (Fig. 3). Furthermore, an aqueous solution of PDA-Im is nonfluorescent at pH = 7.8 but becomes fluorescent with a maximum at 558 nm when the pH is decreased from 7.8 to 2.2 (Fig. S6, ESI†). Analysis of a titration curve obtained by monitoring fluorescence changes indicates that the pKa of PDA-Im is 4.06 (Fig. S8, ESI†). Likewise, the fluorescence intensity of PDA-Ta at 627 nm increases more than 90-fold upon changing the pH from 13 to 9. Using the Henderson–Hasselbalch equation, the pKa value of PDA-Ta was calculated to be 12.30 (Fig. S7 and S9, ESI†).
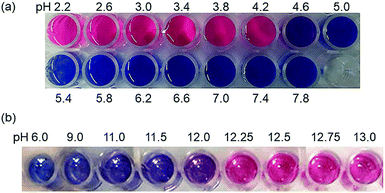 |
| Fig. 2 (a) Colorimetric changes of PDA-Im (10 μM) at different pHs (2.2–7.8). (b) Colorimetric changes of PDA-Ta (1 mM) at different pHs (6.0–13.0). | |
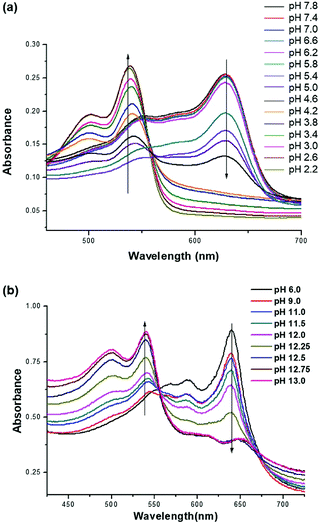 |
| Fig. 3 (a) UV/Vis spectra of PDA-Im (10 μM) at different pHs between 2.2 and 7.8. (b) UV/Vis spectra of PDA-Ta (1 mM) at different pHs between 6.0 and 13.0. | |
Detection of HCl gas with polymer films
The general procedure used to prepare the PDA-Im film is illustrated in Fig. 4. The procedure involves spin coating a solution containing 5 mg of PCDA-Im in 0.2 mL of CHCl3 onto a UV-ozone pre-treated glass at a speed of 1000 rpm for 20 s. The resulting film on the glass was irradiated using a 4 W 254 nm UV lamp for 30 s to yield a blue PDA-Im film.
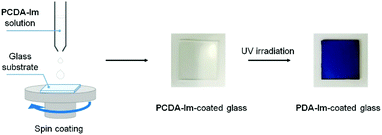 |
| Fig. 4 A schematic representation of the preparation of the PDA-Im-coated glass. | |
A distinct color transition from blue to red occurs when the PDA-Im-coated glass is exposed to as low as 0.5 μL of HCl gas in a 100 mL chamber (Fig. 5). As stated above, in the presence of HCl gas, the imidazole group in PDA-Im is protonated leading to charge repulsion-induced stress on the conjugated polymer backbone. The photographic images in Fig. 5 show the time- and concentration-dependent color changes that take place when the PDA-Im-coated glass is exposed to HCl gas. The color transition promoted by 0.8 μL of HCl in a 100 mL chamber begins within 3 s and is complete within 10 s. The blue-to-red color transition of the PDA film can also be monitored by using UV absorption spectroscopy. As shown in Fig. S10, ESI,† the maximum absorption wavelength at 650 nm is shifted in a time-dependent manner to 540 nm upon the addition of HCl gas. Furthermore, the Raman spectrum of the PDA-Im-coated glass contained peaks assigned to the carbon triple-bond stretch at 2076 cm−1 and the carbon double-bond stretch at 1456 cm−1. The peaks within these regions were correlated to the blue phase of the conventional PDA backbone.18 The Raman spectra of the PDA-Im-coated glass were recorded after exposure to HCl gas. When exposed to HCl gas, the carbon triple-bond peak shifted to 2121 cm−1, corresponding to the red portion of the PDA film. In contrast, vapors arising from organic solvents, such as acetone, acetonitrile, chloroform, hexane, methyl alcohol, methylene chloride and tetrahydrofuran, do not induce significant color changes of the PDA-Im-coated glass (Fig. S12, ESI†).
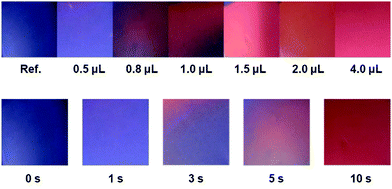 |
| Fig. 5 Top: Concentration-dependent color changes of the PDA-Im film in the presence of HCl gas for 3 s. Bottom: Time-dependent color changes in the presence of HCl gas (0.8 μL of HCl in a 100 mL chamber). | |
Experimental
Equipment and measurement
10,12-Pentacosadiynoic acid (PCDA), N,N-dimethylethylenediamine, N,N′-dicyclohexylcarbodiimide, 1-(3-aminopropyl)imidazole and 3-amino-1,2,4-triazole were purchased from Aldrich, South Korea. All organic solvents used in the reactions employed in the synthesis pathway were obtained from Aldrich and were used without further purification. Flash chromatography was carried out on a silica gel (230–400 mesh). 1H and 13C NMR spectra were recorded using a Bruker Avance 300 MHz NMR spectrometer. UV absorption spectroscopy measurements were carried out on a Scinco S-3100 at room temperature. Scanning Electron Microscopy (SEM) images were acquired on a JEOL JMS-6700F operating at an accelerating voltage of 10.0 kV. Transmission electron microscopy (TEM) images were acquired on a JEM-2100F TEM operating at an accelerating voltage of 200 kV. A spin coater ACE-200 Series of Dong Ah Tech & Trade Corp. was used for spin coating.
Synthesis of PCDA-Im and PCDA-Ta
For synthesis of PCDA-Im (N-(3-(1H-imidazol-1-yl)propyl)pentacosa-10,12-diynamide), N-hydroxysuccinimide (NHS, 300 mg, 2.61 mmol) and 600 mg (2.91 mmol) of N,N′-dicyclohexylcarbodiimide (DCC) were added to a solution containing 0.750 g (2.01 mmol) of 10,12-pentacosadiynoic acid in 20 mL of CH2Cl2. The resulting solution was stirred overnight at room temperature under a N2 atmosphere. The concentration of the mixture in vacuo gave a white precipitate that was filtered, and the filtrate was concentrated in vacuo giving a residue that was subjected to flash column chromatography (silica gel, dichloromethane) to yield the NHS-modified PCDA PDA-NHS. PDA-NHS was added to a solution of 1-(3-aminopropyl)-imidazole (500 mg, 4.0 mmol) in 10 mL of CH2Cl2. The resulting mixture was stirred for 24 h at room temperature. The concentration of the mixture in vacuo gave a residue that was subjected to a silica gel column (dichloromethane
:
methanol = 97
:
3) to yield N-(3-(1H-imidazol-1-yl)propyl)pentacosa-10,12-diynamide (560 mg, 58%) as a white solid. 1H NMR (CDCl3, 300 MHz) δ (ppm): 7.70 (s, 1H), 7.09 (s, 1H), 6.97 (s, 1H), 5.70 (br, 1H), 4.02 (t, 2H, J = 6.9 Hz), 3.26 (q, 2H, J = 6.9 Hz), 2.26–2.13 (m, 6H), 2.06–1.97 (m, 2H), 1.66–1.22 (m, 32 H), 0.84 (t, 3H, J = 6.3 Hz). 13C NMR (CDCl3, 62.5 MHz) δ (ppm): 173.6, 137.0, 129.6, 118.9, 65.3, 65.2, 44.7, 36.6, 31.9, 29.6, 29.5, 29.3, 29.2, 29.1, 29.0 28.9, 28.8, 28.7, 28.3, 28.2, 22.7, 19.2, 19.1. FAB MS m/z = 482.4112 [M + H]+, calc. for C31H52N3O = 482.4110.
For synthesis of PCDA-Ta (N-(2H-1,2,3-triazol-4-yl)pentacosa-10,12-diynamide), oxalyl chloride (0.686 mL, 8 mmol) was added dropwise to a CH2Cl2 solution containing 0.75 g (2 mmol) of 10,12-pentacosadiynoic acid. After stirring for 1 h, one drop of dimethylformamide (DMF) was added to the solution and the resulting mixture was stirred for another 4 h. The concentration of the mixture in vacuo gave crude PDCA-Cl to which a solution containing 0.168 g (2 mmol) of 3-amino-1,2,4-triazole and 1.11 mL (8 mmol) of triethylamine in 20 mL of CH2Cl2 was added. The resulting solution was stirred overnight at room temperature under a N2 atmosphere. The concentration of the mixture in vacuo gave a residue that was subjected to a silica gel column (hexane
:
ethyl acetate = 1
:
1) to give N-(2H-1,2,3-triazol-4-yl)pentacosa-10,12-diynamide (860 mg, 97%) as a white solid. 1H NMR (CDCl3, 300 MHz) δ (ppm): 7.53 (s, 1H), 6.83 (s, 1H), 3.01 (t, 2H, J = 2.5 Hz), 2.24 (t, 4H, J = 2.2 Hz), 1.72 (s, 11H), 1.50 (q, 4H, J = 2.4 Hz), 1.36–1.25 (m, 26 H), 0.88 (t, 3H, J = 2.1 Hz). 13C NMR (CDCl3, 125 MHz) δ (ppm): 175.1, 171.4, 150.5, 77.9, 77.6, 77.5, 77.0, 68.2, 65.6, 65.5, 60.6, 35.4, 32.2, 29.9, 29.8, 29.7, 29.6, 29.3, 29.2, 29.1, 29.0, 28.6, 28.5, 25.8, 24.1, 22.9, 21.2, 19.4, 14.4, 14.3. ESI MS m/z = 441.3585 [M + H]+, calc. for C27H44N4O = 441.358.
Preparation and PDA of vesicles
Independent solutions of PCDA-Im (9.65 mg, 0.02 mmol) and PCDA-Ta (8.83 mg, 0.02 mmol) in small amounts of THF (2 mL) and DMF (2 mL), respectively, were injected into 18 mL of deionized water while shaking. The solutions containing total monomer concentrations of 1 mM were sonicated at 80 °C for 30 min. The resulting solutions were filtered using a 0.8 μm filter, and the filtrates were incubated at 4 °C for 12 h. Polymerization of each solution was carried out at room temperature upon UV irradiation at 254 nm (1 mW cm−2) for 30 and 120 s, respectively.
Preparation of the PDA-Im-coated glass
A glass substrate was treated with UV-ozone for 20 min. The PCDA-Im solution (5 mg in 0.2 mL of chloroform) was then spin-coated onto the glass at a speed of 1000 rpm for 20 s. The spin-coated samples were irradiated with a 4 W 254 nm UV lamp at room temperature for 30 s to yield the PDA-Im-coated glass.
pH titrations of PDA-Im and PDA-Ta
Potentiometric titrations were performed on PDA-Im and PDA-Ta (10 μM) in 20 mL of aqueous solutions at room temperature using an AZ pH meter 86501, standardized with Sigma-certified buffers at pH 4.01 and 10.01 or 7.01 before each titration. To these solutions, 0.1 N HCl and NaOH were added to adjust the pH followed by incubation for at least 5 min before the measurements were carried out.
Conclusions
In the investigation described above, we have developed polydiacteylene-based colorimetric chemosensors for naked eye monitoring of pH changes and detecting HCl gas. PDA-Im and PDA-Ta, fabricated by UV irradiation of the corresponding monomeric diacetylene vesicles, have a blue color. The conjugated backbones of PDA-Im and PDA-Ta are efficiently disrupted by the protonation of the respective imidazole and triazole head groups. As a result, PDA-Im and PDA-Ta undergo distinct blue-to-red color changes at pHs that correspond to the respective pKa values of 4.06 and 12.30. Furthermore, a blue-to-red color change occurs within 3 s when the spin-coated PDA-Im film on glass is exposed to as low as 0.5 μL of HCl gas in a 100 mL chamber. The results show that the PDA-Im film has high potential for use as a colorimetric sensor for HCl gas that does not require analytical instrumentation.
Conflicts of interest
There are no conflicts to declare.
Acknowledgements
This research was supported by the National Research Foundation of Korea (NRF) grant funded by the Korean government (the National Creative Research Initiative: No. 2012R1A3A2048814) for J. Y. and the Ministry of Education (No. 2017R1A6A3A04004954) for S. L. Mass spectral data were obtained from the Korea Basic Science Institute (Daegu) using a JEOL JMS 700 high-resolution mass spectrometer.
Notes and references
-
(a) J. Han and K. Burgess, Chem. Rev., 2010, 110, 2709–2728 CrossRef CAS PubMed;
(b) Y. Yue, F. Huo, S. Lee, C. Yin and J. Yoon, Analyst, 2017, 142, 30–41 RSC.
-
(a) P. Kalimuthu, A. Sivanesan and S. A. John, J. Chem. Sci., 2012, 124, 1315–1325 CrossRef CAS;
(b) P. Muthukumar and S. A. John, Sens. Actuators, B, 2011, 159, 238–244 CrossRef CAS;
(c) Y. Itagaki, S. Yamanaka and Y. Sadaoka, Sens. Lett., 2011, 9, 114–117 CrossRef CAS.
- X. Zhou, S. Lee, Z. Xu and J. Yoon, Chem. Rev., 2015, 115, 7944–8000 CrossRef CAS PubMed.
-
(a) L. Zhang, Y. Chen and J. Jiang, Dyes Pigm., 2016, 124, 110–119 CrossRef CAS;
(b) H. Jeon, J. Lee, M. H. Kim and J. Yoon, Macromol. Rapid Commun., 2012, 33, 972–976 CrossRef CAS PubMed;
(c) S. K. Tripathy, J. Y. Woo and C.-S. Han, Anal. Chem., 2011, 83, 9206–9212 CrossRef CAS PubMed;
(d) S. Kang, S. Kim, Y.-K. Yang, S. Bae and J. Tae, Tetrahedron Lett., 2009, 50, 2010–2012 CrossRef CAS;
(e) K. Nakagawa, K. Tanaka, T. Kitagawa and Y. Sadaoka, J. Mater. Chem., 1998, 8, 1199–1204 RSC.
-
(a) H. S. Jung, P. Verwilst, W. Y. Kim and J. S. Kim, Chem. Soc. Rev., 2016, 45, 1242–1256 RSC;
(b) H. Choi, J. H. Lee and J. H. Jung, Analyst, 2014, 139, 3866–3870 RSC.
-
(a) S. Lee, J.-Y. Kim, X. Chen and J. Yoon, Chem. Commun., 2016, 52, 9178–9196 RSC;
(b) X. Sun, T. Chen, S. Huang, L. Li and H. Peng, Chem. Soc. Rev., 2010, 39, 4244–4257 RSC;
(c) R. Jelinek and M. Ritenberg, RSC Adv., 2013, 3, 21192–21201 RSC;
(d) K. Lee, L. K. Povlich and J. Kim, Analyst, 2010, 135, 2179–2189 RSC.
-
(a) S. Lee, J. Lee, M. Lee, Y. K. Cho, J. Baek, J. Kim, S. Park, M. H. Kim, R. Chang and J. Yoon, Adv. Funct. Mater., 2014, 24, 3699–3705 CrossRef CAS;
(b) S. Ryu, I. Yoo, S. Song, B. Yoon and J.-M. Kim, J. Am. Chem. Soc., 2009, 131, 3800–3801 CrossRef CAS PubMed;
(c) C. Phollookin, S. Wacharasindhu, A. Ajavakom, G. Tumcharern, S. Ampornpun and T. Eaidkong, Macromolecules, 2010, 43, 7540–7548 CrossRef CAS.
-
(a) A. D. Jose and B. Konig, Org. Biomol. Chem., 2010, 8, 655–662 RSC;
(b) J. Lee, H. Jun and J. Kim, Adv. Mater., 2009, 21, 3674–3677 CrossRef CAS.
-
(a) X. Chen, S. Kang, M. J. Kim, J. Kim, Y. S. Kim, H. Kim, B. Chi, S.-J. Kim, J. Y. Lee and J. Yoon, Angew. Chem., Int. Ed., 2010, 49, 1422–1425 CrossRef CAS PubMed;
(b) S. Kolusheva, R. Yossef, A. Kugel, M. Katz, R. Volinsky, M. Welt, U. Hadad, V. Drory, M. Kliger, E. Rubin, A. Porgador and R. Jelinek, Anal. Chem., 2012, 84, 5925–5931 CrossRef CAS PubMed.
- Y.-S. Cho, K. M. Kim, D. Lee, W. J. Kim and K. H. Ahn, Chem. – Asian J., 2013, 8, 755–759 CrossRef CAS PubMed.
-
(a) J. Lee, M. Pyo, S. Lee, J. Kim, M. Ra, W.-Y. Kim, B. J. Park, C. W. Lee and J.-M. Kim, Nat. Commun., 2014, 5, 3736–3739 CAS;
(b) D.-H. Park, B. J. Park and J.-M. Kim, Acc. Chem. Res., 2016, 49, 1211–1222 CrossRef CAS PubMed.
-
(a) Q. Xu, S. Lee, Y. Cho, M. H. Kim, J. Bouffard and J. Yoon, J. Am. Chem. Soc., 2013, 135, 17751–17754 CrossRef CAS PubMed;
(b) B. W. Davis, A. J. Burris, N. Niamnont, C. D. Hare, C.-Y. Chen, M. Sukwattanasinitt and Q. Cheng, Langmuir, 2014, 30, 9616–9622 CrossRef CAS PubMed;
(c) M.-C. Tu, J. A. Cheema, U. H. Yildiz, A. Palaniappan and B. Liedberg, J. Mater. Chem. C, 2017, 5, 1803–1809 RSC.
-
(a) S. Lee, H. Cheng, M. Chi, Q. Xu, X. Chen, C.-Y. Eom, T. D. James, S. Park and J. Yoon, Biosens. Bioelectron., 2016, 77, 1016–1019 CrossRef CAS PubMed;
(b) M.-K. Park, K.-W. Kim, D. J. Ahn and M.-K. Oh, Biosens. Bioelectron., 2012, 35, 44–49 CrossRef CAS PubMed;
(c) J. Wu, A. Zawistowski, M. Ehrmann, T. Yi and C. Schmuck, J. Am. Chem. Soc., 2011, 133, 9720–9723 CrossRef CAS PubMed.
-
(a) H. Jiang, G. Hershtig, S. Richter and R. Jelinek, J. Phys. Chem. Lett., 2016, 7, 1628–1631 CrossRef CAS PubMed;
(b) R. Pimsen, A. Khumsri, S. Wacharasindhu, G. Tumcharern and M. Sukwattanasinitt, Biosens. Bioelectron., 2014, 62, 8–12 CrossRef CAS PubMed.
-
(a) C. Lin and Z. M. C. Lok, Bioconjugate Chem., 2007, 18, 138–145 CrossRef CAS PubMed;
(b) S. Li, Z. Zhou, Y. Zhang and M. Liu, Chem. Mater., 2005, 17, 5884–5886 CrossRef CAS.
- J. Yoon, S. K. Kim, N. J. Singh and K. S. Kim, Chem. Soc. Rev., 2006, 35, 355–360 RSC.
- D. Mantu, V. Antoci, C. Moldoveanu, G. Zbancioc and I. I. Mangalagiu, J. Enzyme Inhib. Med. Chem., 2016, 31, 96–103 CrossRef CAS PubMed.
- J. S. Kauffman, B. M. Ellerbrock, K. A. Stevens, P. J. Brown, W. T. Pennington and T. W. Hanks, ACS Appl. Mater. Interfaces, 2009, 1, 1287–1291 CAS.
Footnotes |
† Electronic supplementary information (ESI) available. See DOI: 10.1039/c7qm00528h |
‡ These authors contributed equally to this work. |
|
This journal is © the Partner Organisations 2018 |
Click here to see how this site uses Cookies. View our privacy policy here.