DOI:
10.1039/D4RA03157A
(Paper)
RSC Adv., 2024,
14, 20120-20129
Synthesis and biological research of new imidazolone-sulphonamide-pyrimidine hybrids as potential EGFR-TK inhibitors and apoptosis-inducing agents†
Received
29th April 2024
, Accepted 9th June 2024
First published on 24th June 2024
Abstract
Development of new effective EGFR-targeted antitumor agents is needed because of their clinical significance. A new series of imidazolone-sulphonamide-pyrimidine hybrids was designed and synthesized as modified analogs of some reported EGFR inhibitors. The cytotoxic activity of all the synthesized hybrids was investigated against the breast MCF-7 cancerous cell line using doxorubicin (Dox) as a positive control. 4-(Furan-2-ylmethylene)imidazolone-sulphonamide-pyrimidine 6b had the best potent activity against MCF-7 cells with IC50 result of 1.05 μM, which was better than Dox (IC50 = 1.91 μM). In addition, mechanistic studies revealed the ability of compounds 5g, 5h and 6b to inhibit EGFR kinase. Cell cycle analysis revealed that compound 6b can halt MCF-7 cells at the G1 phase with a concomitant decrease in cellular percentage at the S and G2/M phases. This compound produced a noticeable rise in the proportion of apoptotic cells with regard to the untreated control. Furthermore, the effects of hybrid 6b on the expression levels of pro-apoptotic Bax and pro-survival Bcl2 were assessed. The results showed that this compound upregulated the level of Bax expression as well as declined the expression value of Bcl-2 with regard to the untreated control.
1. Introduction
Cancer, medically defined as a malignant neoplasm, is a class of disorders characterized by the cells dividing and growing uncontrollably forming malignant tumors, and invading neighboring parts of the body.1,2 Cancer can also spread via the lymphatic system or bloodstream to more distant areas of the body.3 If the spread is not controlled, it can lead to death.4 The development of cancer is a long-term process in which carcinogenesis events go forward step-by-step and ultimately result in the spread of cancer cells from one area of the body to other parts of the body during the metastasis stage.5,6 Although great improvements have been made in the survival rate from many cancers using contemporary therapeutic strategies, several side effects are unavoidable.7 Therefore, the search for new molecules with good antitumor effectiveness and low adverse effects is encouraging.8 Molecular breakthroughs have led to the discovery of cellular parts involved in the initiation, propagation and proliferation of lesions that lead to primary cancer and metastases.9–11 These may be an attractive proposition for cancer treatment.12 Thus, focusing on these biological targets that may inhibit or reverse the interrelated processes is crucial for cancer remedy.13,14 Kinases have emerged as one of the most powerful intensive classes of therapeutic targets, with several kinase targets being explored to the stage of clinical trials.15,16 Epidermal growth factor receptor (EGFR) represents one of the most significant validated cancer therapeutics.17 In recent years, a substantial number of small molecule EGFR-kinase inhibitors with high antiproliferative activity have been described.18,19 The EGFR inhibitors have some essential pharmacophoric features that can occupy a specific pocket in the ATP binding site of EGFR.20 The pharmacophoric features include a heterocyclic system that is typically supplemented by two hydrophobic groups, a small spacer group, hydrogen bond donor–acceptor group, and finally flat heterocyclic ring connected to the allosteric site outside the ATP binding site.21 An alternative chemotherapeutic strategy involves the use of agents that can induce cellular apoptosis to rapidly eradicate premalignant instead of just inhibiting their growth and/or encouraging some degree of differentiation.22,23 Indeed, activation of apoptosis is becoming more valued as a biologically meaningful anticancer method in the realm of cancer therapy.24
The imidazolone framework is a remarkable heterocyclic motif that has a unique function in the development of anti-cancer drugs.25,26 So far, a variety of imidazolones with different substitution patterns have been developed and validated against numerous molecular targets for the treatment of cancer molecules with outstanding outcomes.27 The imidazolone pharmacophore can acquire synergistic activity with other agents and also, re-sensitize that have acquired resistance.28 Alternatively, sulphonamide and its derivatives are bestowed with diverse biological properties and have received significant attention in the drug development process.29,30 Moreover, from a chemical structure point of view, the common skeleton of several kinase inhibitors is the pyrimidine ring31 (Fig. 1).
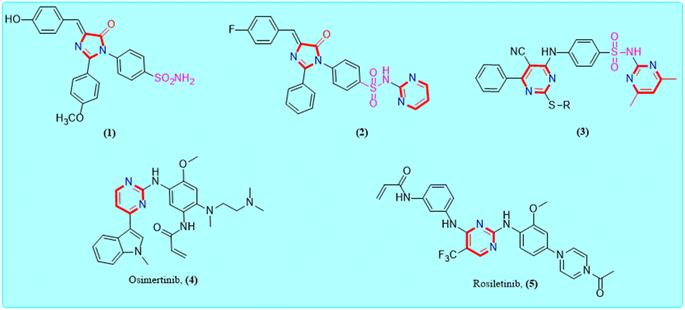 |
| Fig. 1 Examples of some reported imidazolone, sulphonamide and clinically approved pyrimidine scaffold as anti-EGFR candidates. | |
In this investigation, a new set of imidazolone-sulphonamide-pyrimidine hybrids 5a–l and 6a,b were designed and synthesized. The cytotoxic activity of the new imidazolone-sulphonamide-pyrimidine hybrids was assessed on the breast MCF-7 cancer cell line. In addition, the most potent hybrid molecules were evaluated in vitro to assess their EGFR inhibitory activities. Furthermore, a flow cytometric examination of the most active member was also carried out in the study in order to ascertain if the cytotoxic effect is accompanied by a shift in the cell cycle. Moreover, the ability to downregulate Bcl-2 and activate Bax was examined in order to evaluate apoptotic induction and reveal the mechanism of how the active hybrid killed MCF-7 cells.
2. Results and discussion
2.1. Chemistry
The synthetic procedure adopted to prepare the newly synthesized imidazolone-sulphonamide-pyrimidine hybrids 5a–l and 6a,b is illustrated in Scheme 1. In the initial step appropriate hippuric acid 2a,b was obtained by stirring appropriate aroyl chloride 1a,b and glycine in an aqueous sodium hydroxide solution, which was then heated in acetic anhydride at 80 °C to obtain active oxazolone compound 3a,b.32 This was followed by condensation with suitable aryl aldehyde in the presence of anhydrous pyridine furnished the key intermediate 4-arylidene oxazolone compound 4a,b.33 Refluxing acetic acid suspension of appropriate 4-arylidene oxazolone compound 4a,b with sulfadiazine in the existence of freshly melted sodium acetate afforded the titled compound 5a–l and 6a,b. The yields of the title molecules ranged from 53–78% after recrystallization with aqueous ethanol (70%). The purity of the title molecules was reviewed by TLC utilizing n-hexane/ethyl acetate (1
:
3) as the eluent and elemental analyses. Both the analytical and spectral results (1H-NMR and 13C-NMR) of all the synthesized hybrids were completely consistent with the speculated structures.
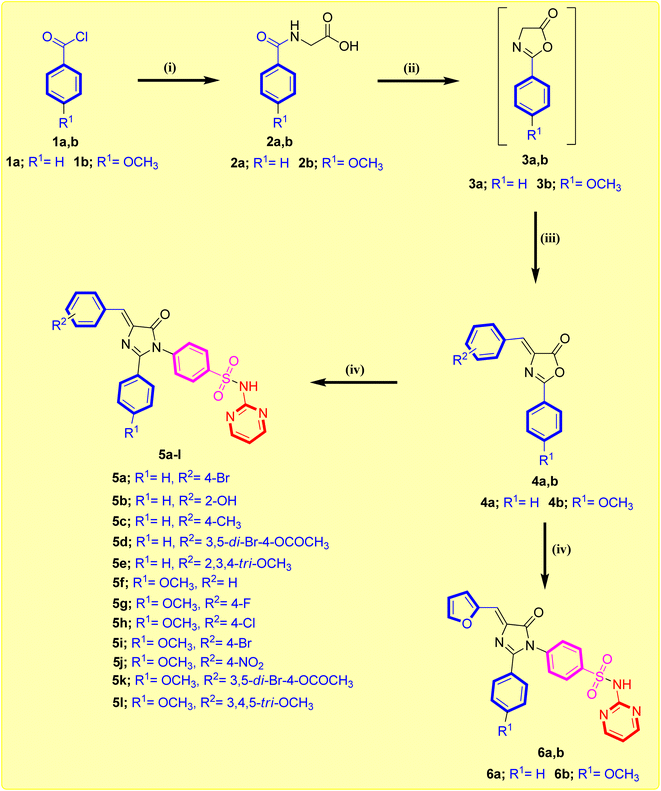 |
| Scheme 1 Synthesis of imidazolone-sulphonamide-pyrimidine compounds 5a–l and 6a,b. Reagents: (i) glycine, aqueous sodium hydroxide, rt, 2 h; (ii) Ac2O, 80 °C, 2 h; (iii) respective aryl aldehyde, NaOAc, 80 °C, 2 h; (iv) sulfadiazine, NaOAc, glacial acetic acid, reflux 16–18 h. | |
In the nuclear magnetic resonance spectra (1H-NMR) the signals of the particular protons of the prepared title were attained by employing their chemical shifts, multiplicities and coupling constants. Compounds 5f-5e and 6b showed a singlet peak in the range of δ 3.78–3.85 ppm related to the methoxy (OCH3) group in the benzene ring. 1H-NMR spectra of imidazolone compounds 5a–l and 6a,b showed a common signal that appeared as a broad singlet between δ 11.20–12.07 ppm attributed to sulphamoyl –SO2NH proton. In addition, common signals appeared as a doublet in the range of δ 8.42–8.54 ppm corresponding to C3,5-Hs of the 1,3-diazine ring; triplet at δ 6.93–7.10 ppm related C4–H of the 1,3-diazine ring; singlet at δ 7.00–7.32 ppm corresponding to olefinic (CH
) hydrogen. The elemental analysis findings were around ±0.4% of the recommended value. On the other hand, 13C-NMR spectra of the compounds 5f-5e and 6b showed a common carbon signal appearing at δ 55.88–56.08 ppm related to the methoxy (OCH3) carbon of the benzene ring. In 13C-NMR spectra of compounds 5a–6b, peaks of olefinic and aromatic carbons were found at δ 109.28–162.48 ppm. Moreover, the 13C-NMR spectra of imidazolone derivatives 5a–6b showed a common characteristic signal at δ 157.20–158.65 ppm attributed to C2 of the imidazolone ring and another peak related C
O of the imidazolone ring in the range at δ 169.22–169.82 ppm. In addition, two new signals at δ 158.31–161.84 and 159.53–164.80 ppm were attributed to C3,5 and C1 of the 1,3-diazine moiety. Besides, 1H-NMR and 13C-NMR spectra of all imidazolone-sulphonamide-pyrimidine hybrids 5a–l and 6a,b are presented in the ESI.†
2.2. Biology
2.2.1. Assessment of cytotoxic effectiveness. The MTT colorimetric method, a frequently utilized assay for detecting the in vitro cytotoxic activities of drugs, was chosen to evaluate the cytotoxic action of the imidazolone-sulphonamide-pyrimidine hybrids 5a–l and 6a,b in hands against breast MCF-7 adenocarcinoma cell line, and they were compared to Dox as the positive control. The obtained data are presented in Table 1. The results demonstrated that the tested imidazolone-sulphonamide-pyrimidine molecules showed significant cytotoxic activity against the tested MCF-7 cells and their potency was affected by both the nature and the type of substituents on the C2-aryl and C4-arylidene moieties of the imidazolone function. The imidazolone compound 6b bearing heterocyclic ring-like 4-(furan-2-yl)methylene group was the most potent hybrid molecule in this study (IC50 = 1.05 μM) compared with Dox (IC50 = 1.91 μM). In addition, the potency was also favored by grafting an electron-withdrawing substituent to benzylidene function on the imidazolone ring-like chlorine; 5h (IC50 = 3.71 μM) or nitro; 5j (IC50 = 3.14 μM). On the other hand, introducing electron-donating substituent on the benzylidene ring as methyl or methoxy group resulted in compounds having moderate cytotoxic activity. Moreover, changing the C2-phenyl imidazolone to C2-(4-methoxyphenyl) imidazolone resulted in compounds having good cytotoxic activity with IC50 values between 1.05 to 25.79 μM.
Table 1 IC50 values (μM) of tested 4-(arylidene)imidazolone-sulphonamide-pyrimidine hybrids 5a–l and 6a,b against breast MCF-7 cancerous cell line
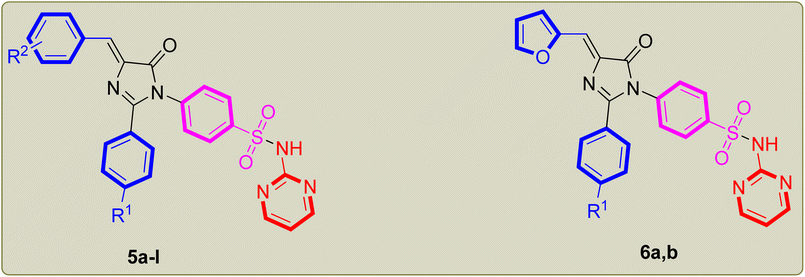
|
Comp. No. |
R1 |
R2 |
IC50 (μM) |
MCF-7 |
5a |
H |
4-Br |
14.05 ± 0.68 |
5b |
H |
2-OH |
31.44 ± 1.64 |
5c |
H |
4-CH3 |
43.94 ± 2.24 |
5d |
H |
3,5-di-Br-4-OCOCH3 |
29.35 ± 1.64 |
5e |
H |
2,3,4-tri-OCH3 |
37.23 ± 1.98 |
5f |
OCH3 |
H |
85.23 ± 4.72 |
5g |
OCH3 |
4-F |
4.57 ± 0.09 |
5h |
OCH3 |
4-Cl |
3.71 ± 0.20 |
5i |
OCH3 |
4-Br |
9.98 ± 0.61 |
5j |
OCH3 |
4-NO2 |
3.14 ± 0.16 |
5k |
OCH3 |
3,5-di-Br-4-OCOCH3 |
14.46 ± 0.65 |
5l |
OCH3 |
3,4,5-tri-OCH3 |
25.79 ± 1.24 |
6a |
H |
— |
5.09 ± 0.31 |
6b |
OCH3 |
— |
1.05 ± 0.04 |
Dox |
— |
— |
1.91 ± 0.07 |
2.2.2. In vitro EGFR kinase inhibitory activity. EGFR-TK is a well-known and validated anticancer target with several clinically used drugs.34 To examine the potential mechanism falling behind the high anticancer effects of imidazolone-sulphonamide-pyrimidine hybrids, the inhibitory potential was evaluated against EGFR-TK. The most effective cytotoxic hybrid molecules 5h, 5j and 6b were assessed for their suppressive efficacy against EGFR-TK. The results were given as an IC50 value calculated from the concentration inhibition responsiveness graph, and they are presented in Fig. 2. Lapatinib as a reference EGFR inhibitor was used and also tested as the positive control during this experiment. The tested imidazolone hybrids demonstrated high to adequate inhibitory efficacy with an IC50 value fluctuating between 0.09–0.21 μM. Among them, 4-(furan-2-ylmethylene)imidazolone-sulphonamide-pyrimidine 6b came out to be the most effective derivative inhibiting EGFR at an IC50 value of 0.09 μM, this is nearly the same as that of standard drug Lapatinib with an IC50 result of 0.06 μM. Furthermore, both 4-(4-chlorobenzylidene)imidazolone-sulphonamide-pyrimidine 5h and 4-(4-nitrobenzylidene)imidazolone-sulphonamide-pyrimidine 5j hybrids possessed a very good EGFR inhibition with an IC50 results of 0.16 and 0.21 μM, respectively.
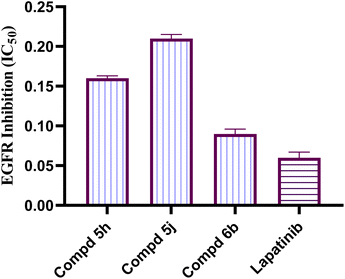 |
| Fig. 2 Graphical illustration of IC50 (μM) for imidazolone-sulphonamide-pyrimidine hybrids 5h, 5j, 6b and Lapatinib on EGFR enzyme. Values indicate the mean ± SE for three replicates. | |
2.2.3. Cell cycle analysis. The impact of the best potent hybrid molecule on the cellular cycle distribution was further evaluated on the MCF-7 cell line. The tested cell line received treatment with 4-(furan-2-ylmethylene)imidazolone-sulphonamide-pyrimidine 6b with a dose of 1.05 μM (IC50 result) over 48 h. The results revealed that 6b caused a significant accumulation of MCF-7 cells in the G1 phase (64.9%) at the IC50 concentration as compared with the control (51.2%). While, a reduced accumulation was observed in the S phase (24.3%) and G2/M phase (10.8%) as compared to the control, which showed 35.1% accumulation in the S phase and 13.7%, in the S phase. These overall results suggested that 4-(furan-2-ylmethylene)imidazolone-sulphonamide-pyrimidine hybrid 6b halts the proliferation of MCF-7 cells by arresting them at the G1 phase, which ultimately leads to cellular death (Fig. 3).
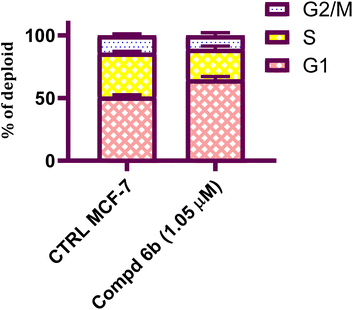 |
| Fig. 3 Cell cycle investigation of phosphatidylserine externalized Annexin-V interaction and cell membrane integrity (PI labelling). MCF-7 cells underwent treatment with 4-(furan-2-ylmethylene)imidazolone-sulphonamide-pyrimidine 6b at (A) 0, (B) 1.05 μM, respectively. | |
2.2.4. Apoptosis staining assay. Apoptosis, a form of programmed cellular death, is a widespread phenomenon, occurring in processes such as morphogenesis, growth and development as well as normal turnover in adult tissue.35 Most cells are programmed to die if survival signals are not received regularly from their environment.36 It can be initiated by endogenous tissue-specific agents such as cytokines or exogenous cell-damaging agents such as chemicals.37 It is evidenced from the literature that exposure to EGFR-TK inhibitors leads to cellular apoptosis.38 Thus, the effect of examined 4-(furan-2-ylmethylene)imidazolone-sulphonamide-pyrimidine hybrid 6b on the apoptosis of the MCF-7 cells was assessed utilizing Annexin V-FITC/PI double labeling followed by flow cytometry (Fig. 4). Annexin V-FITC/PI flow cytometry analysis revealed that MCF-7 cells undergo apoptosis after treatment with IC50 concentration of the compound 6b as shown in Fig. 4. 4-(furan-2-ylmethylene)imidazolone-sulphonamide-pyrimidine hybrid 6b induced 46.1% apoptosis in the MCF-7 cancer cells. Thus, it is evident that this compound had substantial apoptosis-inducing action against the MCF-7 breast cancerous cell line.
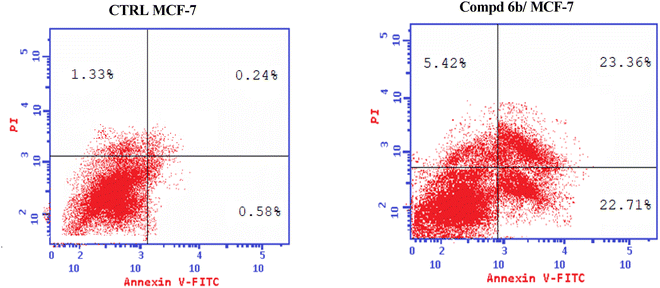 |
| Fig. 4 Flow cytometric method by Annexin V/PI method of the tested MCF-7 cells treated with 4-(furan-2-ylmethylene)imidazolone-sulphonamide-pyrimidine hybrid 6b at the IC50 compared with having no treatment control for 48 h. | |
2.2.5. Compound 6b regulates the expression of the Bcl-2 family of proteins. It is also evidenced from the literature that a link between apoptosis and mitochondrial physiology is suggested by the presence of the Bcl-2 family of proteins in the mitochondrial membrane.39 The Bcl-2 family members are essential in determining whether a cell will survive or perish since they reside upstream of the irreversible cellular damage.40 Two groups of the Bcl-2 family proteins are distinguished by pro-survival (e.g. Bcl-2) or pro-apoptotic (Bax) activity.41 The pro-apoptotic factors promote cellular death. When Bcl-2-associated x-protein (Bax) homodimerizes it enhances apoptosis. The anti-apoptotic effect of Bcl-2 may be due to its ability to inhibit Bax homodimerization.42,43 In this study, the findings of qRT-PCR revealed that 4-(furan-2-ylmethylene)imidazolone-sulphonamide-pyrimidine hybrid 6b inhibited the expression of pro-survival Bcl-2, and upregulated the expression of pro-apoptotic Bax. It is noteworthy, that the level of pro-survival Bcl-2 protein declined by 0.28-fold compared to the control untreated cells. On the other hand, the level of the pro-apoptotic Bax was increased by 4.54-fold as compared with the untreated control (Fig. 5). These findings support that 4-(furan-2-ylmethylene)imidazolone-sulphonamide-pyrimidine hybrid 6b induces apoptosis by regulating the expression of Bcl-2 family proteins.
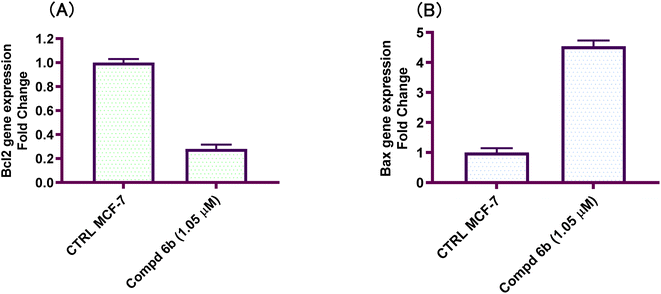 |
| Fig. 5 Roles of Bcl-2 family protein in apoptosis induced by 4-(furan-2-ylmethylene)imidazolone-sulphonamide-pyrimidine hybrid 6b. (A) Pro-survival Bcl-2 protein; (B) pro-apoptotic Bax protein. Cells underwent treatment with an IC50 concentration of imidazolone hybrids 6b for 48 h and the expression of apoptosis-related proteins was examined by qRT-PCR assay. | |
3. Conclusions
In this study, a congeneric series of imidazolone-sulphonamide-pyrimidine hybrids 5a–l and 6a,b were designed and synthesized as potential anticancer agents. The results indicated that 4-(4-chlorobenzylidene)imidazolone-sulphonamide-pyrimidine hybrid 5h (IC50 = 3.71 μM), 4-(4-nitrobenzylidene)imidazolone-sulphonamide-pyrimidine hybrid 5j (IC50 = 3.14 μM) and 4-(furan-2-ylmethylene)imidazolone-sulphonamide-pyrimidine hybrid 6b (IC50 = 1.05 μM) were the most effective hybrids against the MCF-7 cell line compared to the Dox as positive control (IC50 = 1.91 μM). In addition, 4-(furan-2-ylmethylene)imidazolone-sulphonamide-pyrimidine hybrid 6b showed promising EGFR inhibitory activity (IC50 = 0.09 μM) compared to Lapatinib (IC50 = 0.06 μM). Additionally, 4-(4-chlorobenzylidene)imidazolone-sulphonamide-pyrimidine 5h (IC50 = 0.16 μM) and 4-(4-nitrobenzylidene)imidazolone-sulphonamide-pyrimidine 5j (IC50 = 0.21 μM) showed good EGFR inhibitory activity. Furthermore, 4-(furan-2-ylmethylene)imidazolone-sulphonamide-pyrimidine hybrid 6b exerted a notable rise in the population of MCF-7 cells at the G1 phase from 51.2 to 64.9%. Moreover, 4-(furan-2-ylmethylene)imidazolone-sulphonamide-pyrimidine hybrid 6b exerted a significant increase in the apoptotic cells in the early stage from 0.6 to 22.7%, and in the late stage from 0.2 to 23.4%. Finally, 4-(furan-2-ylmethylene)imidazolone hybrid 6b produced a significant increase in the expression value of Bcl-2 associated x-protein (Bax) by 4.54-fold. On the other hand, this compound caused a significant decrease in the expression value of the pro-survival marker Bcl-2 by 0.28-fold with regard to the no-treatment control.
4. Experimental
4.1. Chemistry
4.1.1 The general method for the preparation of (Z)-4-(4-arylidene)-5-oxo-2-aryl-4,5-dihydro-1H-imidazole-1-yl-N-(pyrimidin-2-yl)benzenesulfonamides 5a–l and 6a,b. To a suspension of the respective oxazolone (0.12 mmol) in glacial acetic acid (20 mL), 4-amino-N-(pyrimidin-2-yl)benezenesulphonamide (3.50 g, 0.14 mmol) was placed. The reaction mixture was refluxed for 16–18 h. Once the reaction was completed (consumption of oxazolone molecule), the resulting mixture was kept refrigerated for one night. The precipitated residue was gathered by using filtration and rinsed with absolute ethanol (10 mL). The product solid was purified from aqueous ethanol by crystallization to attain imidazolone-sulphonamide-pyrimidine hybrid 5a–l and 6a,b.
4.1.1.1 (Z)-4-(4-(4-bromobenzylidene)-5-oxo-2-phenyl-4,5-dihydro-1H-imidazole-1-yl)-N-(pyrimidin-2-yl)benzenesulfonamide (5a). Yield: 74%, m.p. 290–292 °C. 1H-NMR (DMSo-d6, 400 MHz) δ (ppm): 11.91 (s, 1H, NH), 8.51 (d, J = 4.9 Hz, 2H, C3,5-H pyrimidine), 8.31 (d, J = 8.6 Hz, 2H, Ar–H), 8.03 (d, J = 8.6 Hz, 2H, Ar–H), 7.73 (d, J = 8.6 Hz, 2H, Ar–H), 7.52 (t, J = 7.3 Hz, 1H, Ar–H), 7.49–7.46 (m, 2H, Ar–H), 7.46–7.44 (m, 2H, Ar–H), 7.37 (t, J = 7.7 Hz, 2H, Ar–H), 7.31 (s, 1H, CH
N), 7.07 (t, J = 4.9 Hz, 1H, C4–H pyrimidine). 13C-NMR (DMSo, 101 MHz) δ: 169.55, 161.19, 158.74, 157.56, 140.92, 139.17, 138.24, 134.58, 134.49, 133.68, 132.43, 132.20, 129.45, 128.99, 128.68, 128.44, 127.14, 127.05, 124.84. Analysis: calc. for C26H18BrN5O3S (560.42): C 55.72, H 3.24, N 12.50%, found: C 55.81, H 3.29, N 12.39%.
4.1.1.2 (Z)-4-(4-(2-hydroxybenzylidene)-5-oxo-2-phenyl-4,5-dihydro-1H-imidazole-1-yl)-N-(pyrimidin-2-yl)benzenesulfonamide (5b). Yield: 53%, m.p. 188–190 °C. 1H-NMR (DMSo-d6, 400 MHz) δ (ppm): 11.20 (s, 1H, NH), 9.67 (s, 1H, OH), 8.64–8.48 (m, 1H, C3,5-H pyrimidine), 8.53–8.45 (m, 1H, Ar–H), 8.02 (d, J = 7.0 Hz, 1H, Ar–H), 7.98 (d, J = 7.5 Hz, 2H, Ar–H), 7.79 (d, J = 7.5 Hz, 1H, Ar–H), 7.65 (t, J = 7.3 Hz, 1H, Ar–H), 7.60 (d, J = 8.4 Hz, 1H, Ar–H), 7.58–7.49 (m, 3H, Ar–H), 7.46 (d, J = 8.2 Hz, 2H, Ar–H), 7.42 (d, J = 5.9 Hz, 1H, Ar–H), 7.40–7.27 (m, 2H, Ar–H and CH
N), 7.06–6.93 (m, 1H, C4–H pyrimidine). 13C-NMR (DMSo, 101 MHz) δ: 166.37, 158.58, 158.31, 150.67, 133.92, 132.77, 130.74, 129.49, 129.15, 128.96, 128.92, 128.62, 128.37, 128.11, 128.04, 127.45, 127.34, 125.53, 124.62, 119.79, 116.46. Analysis: calc. for C26H19N5O4S (497.53): C 62.77, H 3.85, N 14.08%, found: C 62.94, H 3.76, N 13.96%.
4.1.1.3 (Z)-4-(4-(4-methylbenzylidene)-5-oxo-2-phenyl-4,5-dihydro-1H-imidazole-1-yl)-N-(pyrimidin-2-yl)benzenesulfonamide (5c). Yield: 68%, m.p. 277–279 °C. 1H-NMR (DMSo-d6, 400 MHz) δ (ppm): 11.98 (s, 1H, NH), 8.52 (d, J = 4.9 Hz, 2H, C3,5-H pyrimidine), 8.26 (d, J = 8.2 Hz, 2H, Ar–H), 8.03 (d, J = 8.5 Hz, 2H, Ar–H), 7.51 (t, J = 7.4 Hz, 1H, Ar–H), 7.48 (s, 1H, Ar–H), 7.46 (s, 1H, Ar–H), 7.46–7.43 (m, 2H, Ar–H), 7.38 (d, J = 7.6 Hz, 2H, Ar–H), 7.34 (d, J = 7.7 Hz, 2H, Ar–H), 7.29 (s, 1H, CH
N), 7.09 (t, J = 4.9 Hz, 1H, C4–H pyrimidine), 2.39 (s, 3H, CH3). 13C-NMR (DMSo, 101 MHz) δ: 169.60, 160.11, 158.73, 157.30, 141.55, 138.50, 137.91, 132.99, 132.88, 131.97, 131.77, 130.11, 130.04, 129.36, 128.96, 128.94, 128.90, 128.85, 128.44, 21.71. Analysis: calc. for C27H21N5O3S (495.55): C 65.44, H 4.27, N 14.13%, found: C 65.35, H 4.38, N 14.23%.
4.1.1.4 (Z)-2,6-dibromo-4-((5-oxo-2-phenyl-1-(4-(N-pyrimidin-2-ylsulfamoyl)phenyl)-1H-imidazole-4(5H)-ylidene)methyl)phenyl acetate (5d). Yield: 72%, m.p. 243–245 °C. 1H-NMR (DMSo-d6, 400 MHz) δ (ppm): 8.76 (s, 2H, Ar–H), 8.42 (d, J = 4.8 Hz, 2H, C3,5-H pyrimidine), 7.99 (d, J = 8.4 Hz, 2H, Ar–H), 7.54 (t, J = 7.3 Hz, 1H, Ar–H), 7.47 (s, 1H, Ar–H), 7.45 (d, J = 5.3 Hz, 2H, Ar–H), 7.41 (d, J = 8.4 Hz, 2H, Ar–H), 7.38–7.34 (m, 1H, Ar–H), 7.31 (s, 1H, CH
N), 6.93 (t, J = 4.7 Hz, 1H, C4–H pyrimidine), 2.44 (s, 3H, CH3). 13C-NMR (DMSO, 101 MHz) δ: 172.48, 169.41, 167.66, 162.48, 158.48, 146.80, 140.40, 135.88, 135.60, 132.47, 129.46, 129.02, 128.83, 128.72, 128.50, 128.23, 128.06, 123.90, 117.96, 20.69. Analysis: calc. for C28H19Br2N5O5S (697.35): C 48.23, H 2.75, N 10.04%, found: C 48.36, H 2.83, N 9.95%.
4.1.1.5 (Z)-4-(5-oxo-2-phenyl-4-(2,3,4-trimethoxybenzylidene)-4,5-dihydro-1H-imidazole-1-yl)-N-(pyrimidin-2-yl)benzenesulfonamide (5e). Yield: 57%, m.p. 210–212 °C. 1H-NMR (DMSo-d6, 400 MHz) δ (ppm): 11.98 (s, 1H, NH), 8.73 (d, J = 9.0 Hz, 1H, Ar–H), 8.53 (d, J = 4.9 Hz, 2H, C3,5-H pyrimidine), 8.03 (d, J = 8.5 Hz, 2H, Ar–H), 7.51 (d, J = 7.2 Hz, 1H, Ar–H), 7.47 (s, 1H, Ar–H), 7.45 (s, 1H, Ar–H), 7.44 (s, 2H, Ar–H), 7.43 (s, 1H, CH
N), 7.36 (t, J = 7.6 Hz, 2H, Ar–H), 7.10 (t, J = 4.9 Hz, 1H, C4-H pyrimidine), 7.06 (d, J = 9.1 Hz, 1H, Ar–H), 3.92 (s, 3H, OCH3), 3.90 (s, 3H, OCH3), 3.80 (s, 3H, OCH3). 13C-NMR (DMSo, 101 MHz) δ: 169.58, 159.53, 158.76, 158.69, 157.21, 156.68, 154.31, 152.98, 141.87, 140.44, 138.60, 137.15, 131.91, 129.30, 128.98, 128.90, 128.60, 128.45, 122.11, 120.76, 109.28, 62.41, 60.99, 56.60. Analysis: calc. for C29H25N5O6S (571.60): C 60.94, H 4.41, N 12.25%, found: C 61.08, H 4.53, N 12.13%.
4.1.1.6 (Z)-4-(4-benzylidene-2-(4-methoxyphenyl)-5-oxo-4,5-dihydro-1H-imidazole-1-yl)-N-(pyrimidin-2-yl)benzenesulfonamide (5f). Yield: 64%, m.p. 258–260 °C. 1H-NMR (DMSo-d6, 400 MHz) δ (ppm): 11.97 (s, 1H, NH), 8.53 (d, J = 4.9 Hz, 2H, C3,5-H pyrimidine), 8.36 (d, J = 8.8 Hz, 2H, Ar–H), 8.03 (d, J = 8.5 Hz, 2H, Ar–H), 7.51 (d, J = 7.3 Hz, 1H, Ar–H), 7.46 (d, J = 8.3 Hz, 3H, Ar–H), 7.44 (s, 1H, Ar–H), 7.36 (t, J = 7.7 Hz, 2H, Ar–H), 7.29 (s, 1H, CH
N), 7.11 (d, J = 4.2 Hz, 2H, Ar–H), 7.09 (s, 1H, C4-H pyrimidine), 3.85 (s, 3H, OCH3). 13C-NMR (DMSo, 101 MHz) δ: 169.53, 161.94, 159.21, 158.81, 157.20, 140.41, 138.65, 136.60, 135.02, 131.83, 129.29, 129.06, 128.97, 128.94, 128.89, 128.45, 127.23, 115.07, 55.92. Analysis: calc. for C27H21N5O4S (511.55): C 63.39, H 4.14, N 13.69%, found: C 63.29, H 4.02, N 13.76%.
4.1.1.7 (Z)-4-(4-(4-fluorobenzylidene)-2-(4-methoxyphenyl)-5-oxo-4,5- dihydro-1H-imidazole-1-yl)-N-(pyrimidin-2-yl)benzenesulfonamide (5g). Yield: 69%, m.p. 257–259 °C. 1H-NMR (DMSo-d6, 400 MHz) δ (ppm): 12.04 (s, 1H, NH), 8.54 (d, J = 4.8 Hz, 2H, C3,5-H pyrimidine), 8.49–8.40 (m, 2H, Ar–H), 8.06 (d, J = 8.3 Hz, 2H, Ar–H), 7.50 (d, J = 8.3 Hz, 2H, Ar–H), 7.42 (d, J = 8.7 Hz, 2H, Ar–H), 7.37 (t, J = 8.8 Hz, 2H, Ar–H), 7.26 (s, 1H, CH
N), 7.10 (t, J = 4.7 Hz, 1H, C4-H pyrimidine), 6.91 (d, J = 8.7 Hz, 2H, Ar–H), 3.79 (s, 3H, OCH3). 13C-NMR (DMSo, 101 MHz) δ: 172.48, 169.79, 164.80, 162.39, 160.28, 157.24, 140.62, 138.74, 138.36, 135.10, 131.34, 131.27, 129.02, 128.61, 126.15, 126.02, 120.65, 116.64, 116.36, 114.54, 114.39, 55.88. Analysis: calc. for C27H20FN5O4S (529.54): C 61.24, H 3.81, N 13.23%, found: C 61.11, H 3.89, N 13.32%.
4.1.1.8 (Z)-4-(4-(4-chlorobenzylidene)-2-(4-methoxyphenyl)-5-oxo-4,5-dihydro-1H-imidazole-1-yl)-N-(pyrimidin-2-yl)benzenesulfonamide (5h). Yield: 74%, m.p. 262–264 °C. 1H-NMR (DMSo-d6, 400 MHz) δ (ppm): 12.07 (s, 1H, NH), 8.54 (d, J = 4.9 Hz, 2H, C3,5-H pyrimidine), 8.38 (d, J = 8.6 Hz, 2H, Ar–H), 8.10–8.04 (m, 2H, Ar–H), 7.60 (t, J = 8.0 Hz, 2H, Ar–H), 7.50 (d, J = 8.5 Hz, 2H, Ar–H), 7.42 (d, J = 8.9 Hz, 2H, Ar–H), 7.24 (s, 1H, CH
N), 7.10 (t, J = 4.8 Hz, 1H, C4-H pyrimidine), 6.92 (d, J = 8.9 Hz, 2H, Ar–H), 3.79 (s, 3H, OCH3). 13C-NMR (DMSo, 101 MHz) δ: 169.74, 162.47, 160.64, 139.16, 135.45, 134.30, 134.15, 133.57, 132.96, 131.40, 131.34, 129.54, 129.45, 129.03, 128.64, 125.63, 120.57, 114.55, 114.41, 56.07. Analysis: calc. for C27H20ClN5O4S (546.00): C 59.39, H 3.69, N 12.83%, found: C 59.55, H 3.81, N 12.68%.
4.1.1.9 (Z)-4-(4-(4-bromobenzylidene)-2-(4-methoxyphenyl)-5-oxo-4,5-dihydro-1H-imidazole-1-yl)-N-(pyrimidin-2-yl)benzenesulfonamide (5i). Yield: 78%, m.p. 284–286 °C. 1H-NMR (DMSo-d6, 400 MHz) δ (ppm): 8.50 (d, J = 4.9 Hz, 2H, C3,5-H pyrimidine), 8.30 (d, J = 8.6 Hz, 2H, Ar–H), 8.05 (d, J = 8.5 Hz, 2H, Ar–H), 7.73 (d, J = 8.5 Hz, 2H, Ar–H), 7.48 (d, J = 8.5 Hz, 2H, Ar–H), 7.42 (d, J = 8.8 Hz, 2H, Ar–H), 7.22 (s, 1H, CH
N), 7.04 (t, J = 4.8 Hz, 1H, C4-H pyrimidine), 6.92 (d, J = 8.9 Hz, 2H, Ar–H), 3.79 (s, 3H, OCH3). 13C-NMR (DMSo, 101 MHz) δ: 169.78, 162.48, 160.70, 158.65, 139.27, 138.43, 134.43, 134.31, 133.88, 132.38, 131.41, 131.35, 129.07, 128.95, 128.54, 125.78, 125.67, 124.49, 120.58, 114.56, 114.40, 56.08. Analysis: calc. for C27H20BrN5O4S (590.45): C 54.92, H 3.41, N 11.86%, found: C 55.10, H 3.58, N 11.67%.
4.1.1.10 (Z)-4-(2-(4-methoxyphenyl)-4-(4-nitrobenzylidene)-5-oxo-4,5-dihydro-1H-imidazole-1-yl)-N-(pyrimidin-2-yl)benzenesulfonamide (5j). Yield: 75%, m.p. > 300 °C. 1H-NMR (DMSo-d6, 400 MHz) δ (ppm): 8.59 (d, J = 9.0 Hz, 2H, Ar–H), 8.53 (d, J = 4.9 Hz, 2H, C3,5-H pyrimidine), 8.34 (d, J = 9.0 Hz, 2H, Ar–H), 8.12–8.04 (m, 2H, Ar–H), 7.52 (d, J = 8.6 Hz, 2H, Ar–H), 7.46 (d, J = 8.9 Hz, 2H, Ar–H), 7.32 (s, 1H, CH
N), 7.07 (t, J = 4.9 Hz, 1H, C4-H pyrimidine), 6.93 (d, J = 9.0 Hz, 2H, Ar–H), 3.80 (s, 3H, OCH3). 13C-NMR (DMSo, 101 MHz) δ: 169.78, 162.82, 162.41, 158.83, 157.53, 147.78, 141.42, 141.10, 138.43, 133.33, 133.25, 131.64, 129.16, 128.65, 124.35, 124.23, 123.63, 123.53, 120.26, 114.62, 114.46, 55.94. Analysis: calc. for C27H20N6O6S (556.55): C 58.27, H 3.62, N 15.10%, found: C 58.43, H 3.74, N 14.89%.
4.1.1.11 (Z)-2,6-dibromo-4-((2-(4-methoxyphenyl)-5-oxo-1-(4-(N-pyrimidin-2-ylsulfamoyl)phenyl)-1H-imidazole-4(5H)-ylidene)methyl)phenyl acetate (5k). Yield: 69%, m.p. 239–241 °C. 1H-NMR (DMSo-d6, 400 MHz) δ (ppm): 11.98 (s, 1H, NH), 8.75 (s, 2H, Ar–H), 8.54 (d, J = 4.9 Hz, 2H, C3,5-H pyrimidine), 8.10–8.05 (m, 2H, Ar–H), 7.51 (d, J = 8.6 Hz, 2H, Ar–H), 7.40 (d, J = 8.9 Hz, 2H, Ar–H), 7.23 (s, 1H, CH
N), 7.10 (t, J = 4.9 Hz, 1H, C4-H pyrimidine), 6.94 (d, J = 8.9 Hz, 2H, Ar–H), 3.80 (s, 3H, OCH3), 2.44 (s, 3H, CH3). 13C-NMR (DMSo, 101 MHz) δ: 172.47, 169.51, 167.68, 162.74, 161.84, 157.17, 146.58, 140.46, 140.01, 138.55, 135.80, 135.71, 131.41, 129.11, 128.68, 122.60, 120.29, 117.91, 114.63, 56.02, 21.52. Analysis: calc. for C29H21Br2N5O6S (727.38): C 47.89, H 2.91, N 9.63%, found: C 48.03, H 3.04, N 9.51%.
4.1.1.12 (Z)-4-(2-(4-methoxyphenyl)-5-oxo-4-(3,4,5-trimethoxybenzylidene)-4,5-dihydro-1H-imidazole-1-yl)-N-(pyrimidin-2-yl)benzenesulfonamide (5l). Yield: 66%, m.p. 247–249 °C. 1H-NMR (DMSo-d6, 400 MHz) δ (ppm): 8.48 (d, J = 4.9 Hz, 2H, C3,5-H pyrimidine), 8.06–8.01 (m, 2H, Ar–H), 7.83 (s, 2H, Ar–H), 7.46 (d, J = 8.6 Hz, 2H, Ar–H), 7.42 (d, J = 8.9 Hz, 2H, Ar–H), 7.18 (s, 1H, CH
N), 7.00 (t, J = 4.8 Hz, 1H, C4-H pyrimidine), 6.91 (d, J = 9.0 Hz, 2H, Ar–H), 3.86 (s, 6H, 2OCH3), 3.79 (s, 3H, OCH3), 3.76 (s, 3H, OCH3). 13C-NMR (DMSo, 101 MHz) δ 169.82, 164.06, 162.34, 159.46, 158.61, 153.26, 140.25, 137.87, 131.11, 130.48, 130.10, 129.41, 128.97, 128.38, 127.50, 120.76, 117.62, 115.48, 114.54, 110.36, 110.15, 60.69, 56.35, 55.95. Analysis: calc. for C30H27N5O7S (601.63): C 59.89, H 4.52, N 11.64%, found: C 60.08, H 4.40, N 11.52%.
4.1.1.13 (Z)-4-(4-(furan-2-ylmethylene)-5-oxo-2-phenyl-4,5-dihydro-1H-imidazole-1-yl)-N-(pyrimidin-2-yl)benzenesulfonamide (6a). Yield: 54%, m.p. 291–293 °C. 1H-NMR (DMSo-d6, 400 MHz) δ (ppm): 11.98 (s, 1H, NH), 8.52 (d, J = 4.9 Hz, 2H, C3,5-H pyrimidine), 8.07–8.04 (m, 1H, C5-H furan), 8.03 (d, J = 8.7 Hz, 2H, Ar–H), 7.58 (d, J = 3.5 Hz, 1H, C3-H furan), 7.52–7.48 (m, 1H, Ar–H), 7.47 (d, J = 2.0 Hz, 1H, Ar–H), 7.45 (t, J = 2.6 Hz, 2H, Ar–H), 7.43 (d, J = 1.4 Hz, 1H, Ar–H), 7.35 (t, J = 7.7 Hz, 2H, Ar–H), 7.15 (s, 1H, Ar–H), 7.09 (t, J = 4.9 Hz, 1H, C4-H pyrimidine), 6.85–6.79 (m, 1H, C4-H furan). Analysis: calc. for C24H17N5O4S (471.49): C 61.14, H 3.63, N 14.85%, found: C 60.93, H 3.76, N 15.02%.
4.1.1.14 (Z)-4-(4-(furan-2-ylmethylene)-2-(4-methoxyphenyl)-5-oxo-4,5-dihydro-1H-imidazole-1-yl)-N-(pyrimidin-2-yl)benzenesulfonamide (6b). Yield: 61%, m.p. 278–280 °C. 1H-NMR (DMSo-d6, 400 MHz) δ (ppm): 12.04 (s, 1H, NH), 8.53 (d, J = 4.9 Hz, 2H, C3,5-H pyrimidine), 8.06 (d, J = 8.5 Hz, 2H, Ar–H), 8.02 (d, J = 1.5 Hz, 1H, C5-H furan), 7.57 (d, J = 3.4 Hz, 1H, C3-H furan), 7.48 (d, J = 8.5 Hz, 2H, Ar–H), 7.40 (d, J = 8.8 Hz, 2H, Ar–H), 7.09 (t, J = 4.9 Hz, 1H, C4-H pyrimidine), 7.07 (s, 1H, CH
N), 6.90 (d, J = 8.9 Hz, 2H, Ar–H), 6.81 (dd, J = 3.4, 1.6 Hz, 1H, C4-H furan), 3.78 (s, 3H, OCH3). 13C-NMR (DMSo, 101 MHz) δ: 172.48, 169.22, 162.31, 159.28, 158.78, 157.26, 151.02, 147.49, 140.58, 138.79, 135.98, 131.25, 129.07, 128.57, 120.69, 119.63, 114.44, 114.41, 114.36, 55.95. Analysis: calc. for C25H19N5O5S (501.51): C 59.87, H 3.82, N 13.96%, found: C 60.03, H 4.96, N 13.84%.
Conflicts of interest
The authors report no conflicts of interest.
Acknowledgements
The authors extend their appreciation to Princess Nourah Bint Abdulrahman University Researchers Supporting Project number (PNURSP2024R155), Princess Nourah Bint Abdulrahman University, Riyadh, Saudi Arabia, and the authors extend their appreciation to the Deanship of Scientific Research and Graduate Studies at King Khalid University for funding this work through the Large Research Project under grant number RGP2/61/45.
References
- S. S. Hakkimane and S. L. Gaonkar, Chapter 1–Biology of cancer: current insights and perspectives, in Treatment Landscape of Targeted Therapies in Oncology, ed. P. K. Maurya and V. Saini, Academic Press, 2023, pp. 1–11 Search PubMed.
- S. Sriharikrishnaa, P. S. Suresh and K. S. Prasada, An Introduction to Fundamentals of Cancer Biology, in Optical Polarimetric Modalities for Biomedical Research, ed. N. Mazumder, et al., Springer International Publishing, Cham, 2023, pp. 307–330 Search PubMed.
- J. S. Brown, S. R. Amend, R. H. Austin, R. A. Gatenby, E. U. Hammarlund and K. J. Pienta, Updating the Definition of Cancer, Mol. Cancer Res., 2023, 21, 1142–1147 CrossRef CAS PubMed.
- K. M. Cuthrell and N. Tzenios, Breast Cancer: Updated and Deep Insights, Int. J. Oncol., 2023, 6, 104–118 Search PubMed.
- L. S. Carvalho, N. Gonçalves, N. A. Fonseca and J. N. Moreira, Cancer Stem Cells and Nucleolin as Drivers of Carcinogenesis, Pharmaceuticals, 2021, 14, 60–80 CrossRef CAS PubMed.
- A. Ring, B. D. Nguyen-Sträuli, A. Wicki and N. Aceto, Biology, vulnerabilities and clinical applications of circulating tumour cells, Nat. Rev. Cancer, 2023, 23, 95–111 CrossRef CAS PubMed.
- L. Vitiello, F. Lixi, G. Coco and G. Giannaccare, Ocular Surface Side Effects of Novel Anticancer Drugs, Cancers, 2024, 16, 344–355 CrossRef CAS PubMed.
- D. N. Binjawhar, F. A. Al-Salmi, O. A. A. Ali, M. A. Alghamdi, E. Fayad, R. M. Saleem, I. Zaki and N. Farouk, Design, synthesis and cytotoxic activity of molecular hybrids based on quinolin-8-yloxy and cinnamide hybrids and their apoptosis inducing property, RSC Adv., 2024, 14, 11443–11451 RSC.
- M. C. G. Winge, L. N. Kellman, K. Guo, J. Y. Tang, S. M. Swetter, S. Z. Aasi, K. Y. Sarin, A. L. S. Chang and P. A. Khavari, Advances in cutaneous squamous cell carcinoma, Nat. Rev. Cancer, 2023, 23, 430–449 CrossRef CAS PubMed.
- H. M. Abd El-Lateef, L. M. A. Ghany, R. M. Saleem, A. H. A. Maghrabi, M. A. Y. Alahdal, E. H. K. Ali, B. Y. Beshay, I. Zaki and R. E. Masoud, Design, synthesis and antiproliferative screening of newly synthesized coumarin-acrylamide hybrids as potential cytotoxic and apoptosis inducing agents, RSC Adv., 2023, 13, 32547–32557 RSC.
- S. Halder, S. Basu, S. P. Lall, A. K. Ganti, S. K. Batra and P. Seshacharyulu, Targeting the EGFR signaling pathway in cancer therapy: What's new in 2023?, Expert Opin. Ther. Targets, 2023, 27, 305–324 CrossRef CAS PubMed.
- S. Ramani, S. Samant and S. M. Manohar, The story of EGFR: from signaling pathways to a potent anticancer target, Future Med. Chem., 2022, 14, 1267–1288 CrossRef CAS PubMed.
- K. S. Alharbi, M. A. Javed Shaikh, O. Afzal, A. S. Alfawaz Altamimi, W. H. Almalki, S. I. Alzarea, I. Kazmi, F. A. Al-Abbasi, S. K. Singh, K. Dua and G. Gupta, An overview of epithelial growth factor receptor (EGFR) inhibitors in cancer therapy, Chem.-Biol. Interact., 2022, 366, 110108–110124 CrossRef CAS PubMed.
- E. Fayad, S. A. Altalhi, M. M. Abualnaja, A. H. Alrohaimi, F. G. Elsaid, A. H. Abu Almaaty, R. M. Saleem, M. A. Bazuhair, A. H. Ahmed Maghrabi, B. Y. Beshay and I. Zaki, Novel Acrylate-Based Derivatives: Design, Synthesis, Antiproliferative Screening, and Docking Study as Potential Combretastatin Analogues, ACS Omega, 2023, 8, 38394–38405 CrossRef CAS PubMed.
- F. Giraud, E. Pereira, F. Anizon and P. Moreau, Recent Advances in Pain Management: Relevant Protein Kinases and Their Inhibitors, Molecules, 2021, 26(9), 2696–2716 CrossRef CAS PubMed.
- R. Roskoski Jr, Properties of FDA-approved small molecule protein kinase inhibitors: A 2022 update, Pharmacol. Res., 2022, 175, 106037–106052 CrossRef CAS PubMed.
- V. Favorito, I. Ricciotti, A. De Giglio, L. Fabbri, R. Seminerio, A. Di Federico, E. Gariazzo, S. Costabile and G. Metro, Non-small cell lung cancer: an update on emerging EGFR-targeted therapies, Expert Opin. Emerging Drugs, 2024, 1–16 Search PubMed.
- T. Zubair and D. Bandyopadhyay, Small Molecule EGFR Inhibitors as Anti-Cancer Agents: Discovery, Mechanisms of Action, and Opportunities, Int. J. Mol. Sci., 2023, 24, 2651–2669 CrossRef CAS PubMed.
- D. Nasser Binjawhar, F. A. Al-Salmi, M. A. Alghamdi, A. s. Alqahtani, E. Fayad, R. M. Saleem, I. Zaki and A. M. Youssef Moustafa, Design, Synthesis, and Biological Evaluation of Newly Synthesized Cinnamide-Fluorinated Containing Compounds as Bioactive Anticancer Agents, ACS Omega, 2024, 9(16), 18505–18515 CrossRef CAS PubMed.
- M. A. Ashiru, S. O. Ogunyemi, O. R. Temionu, A. C. Ajibare, N. C. Cicero-Mfon, O. A. Ihekuna, M. O. Jagun, L. Abdulmumin, Q. K. Adisa, Y. E. Asibor, C. J. Okorie, M. O. Lawal, M. O. Babalola, I. T. Abdulrasaq, L. B. Salau, I. O. Olatunji, M. A. Bankole, A. B. Daud and A. O. Adeyemi, Identification of EGFR inhibitors as potential agents for cancer therapy: pharmacophore-based modeling, molecular docking, and molecular dynamics investigations, J. Mol. Model., 2023, 29, 128 CrossRef CAS PubMed.
- S. Mishra, A. Sahu, A. Kaur, M. Kaur and P. Wal, Recent Development in the Search for Epidermal Growth Factor Receptor (EGFR) Inhibitors based on the Indole Pharmacophore, Curr. Top. Med. Chem., 2024, 24, 581–613 CrossRef CAS PubMed.
- O. Morana, W. Wood and C. D. Gregory, The Apoptosis Paradox in Cancer, Int. J. Mol. Sci., 2022, 23, 1328–1341 CrossRef CAS PubMed.
- S. M. van Neerven and L. Vermeulen, Cell competition in development, homeostasis and cancer, Nat. Rev. Mol. Cell Biol., 2023, 24, 221–236 CrossRef CAS PubMed.
- A. Jain, J. Gosling, S. Liu, H. Wang, E. M. Stone, S. Chakraborty, P.-S. Jayaraman, S. Smith, D. B. Amabilino, M. Fromhold, Y.-T. Long, L. Pérez-García, L. Turyanska, R. Rahman and F. J. Rawson, Wireless electrical–molecular quantum signalling for cancer cell apoptosis, Nat. Nanotechnol., 2024, 19, 106–114 CrossRef CAS PubMed.
- M. K. Abdelhameid, I. Zaki, M. R. Mohammed and K. O. Mohamed, Design, synthesis, and cytotoxic screening of novel azole derivatives on hepatocellular carcinoma (HepG2 Cells), Bioorg. Chem., 2020, 101, 103995–104014 CrossRef CAS PubMed.
- A. Husain, A. F. AlAsmari, S. N. H. Azmi, N. Ali, M. M. R. Sarker, M. Alharbi, M. Ishtikhar and S. A. Khan, Rational drug design, synthesis, and in vivo biological activity of new indolyl-imidazolone hybrids as potential and safer non-steroidal anti-inflammatory agents, J. King Saud Univ., Sci., 2022, 34, 102023 CrossRef.
- B. Sivakumar and K. Ilango, 5-Imidazolinone Derivatives as a Potent Pharmacological Agents—A Review, Russ. J. Bioorg. Chem., 2023, 49, 167–179 CrossRef CAS.
- G. Teli and P. A. Chawla, Hybridization of Imidazole with Various Heterocycles in Targeting Cancer: A Decade's Work, ChemistrySelect, 2021, 6, 4803–4836 CrossRef CAS.
- L. F. Castaño, J. Quiroga, R. Abonia, D. Insuasty, O. M. Vidal, R. Seña, V. Rubio, G. Puerto, M. Nogueras, J. Cobo, J. Guzman, A. Insuasty and B. Insuasty, Synthesis, Anticancer and Antitubercular Properties of New Chalcones and Their Nitrogen-Containing Five-Membered
Heterocyclic Hybrids Bearing Sulfonamide Moiety, Int. J. Mol. Sci., 2022, 23, 12589–12601 CrossRef PubMed.
- R. Ghomashi, S. Ghomashi, H. Aghaei, S. Massah and A. R. Massah, Recent advances in biological active sulfonamide based hybrid compounds part c: multicomponent sulfonamide hybrids, Curr. Med. Chem., 2023, 30, 4181–4255 CrossRef CAS PubMed.
- K. R. A. Abdellatif and R. B. Bakr, Pyrimidine and fused pyrimidine derivatives as promising protein kinase inhibitors for cancer treatment, Med. Chem. Res., 2021, 30, 31–49 CrossRef CAS.
- D. S. Alshaya, R. M. Tawakul, I. Zaki, A. H. A. Almaaty, E. Fayad and Y. M. Abd El-Aziz, Design, synthesis and antiproliferative screening of newly synthesized acrylate derivatives as potential anticancer agents, RSC Adv., 2023, 13, 23538–23546 RSC.
- I. Zaki, M. K. Abdelhameid, I. M. El-Deen, A. H. A. Abdel Wahab, A. M. Ashmawy and K. O. Mohamed, Design, synthesis and screening of 1,2,4-triazinone derivatives as potential antitumor agents with apoptosis inducing activity on MCF-7 breast cancer cell line, Eur. J. Med. Chem., 2018, 156, 563–579 CrossRef CAS PubMed.
- R. Hajjo, D. A. Sabbah, S. K. Bardaweel and H. A. Zhong, Targeting the EGFR/RAS/RAF signaling pathway in anticancer research: a recent update on inhibitor design and clinical trials (2020–2023), Expert Opin. Ther. Pat., 2024, 34, 51–69 CrossRef CAS PubMed.
- E. H. Maleki, A. R. Bahrami and M. M. Matin, Cancer cell cycle heterogeneity as a critical determinant of therapeutic resistance, Genes Dis., 2024, 11, 189–204 CrossRef CAS PubMed.
- S. Shen, Y. Shao and C. Li, Different types of cell death and their shift in shaping disease, Cell Death Discovery, 2023, 9, 284 CrossRef PubMed.
- R. J. Hill, N. Bona, J. Smink, H. K. Webb, A. Crisp, J. I. Garaycoechea and G. P. Crossan, p53 regulates diverse tissue-specific outcomes to endogenous DNA damage in mice, Nat. Commun., 2024, 15, 2518–2533 CrossRef CAS PubMed.
- M. Alam, S. Alam, A. Shamsi, M. Adnan, A. M. Elasbali, W. A. Al-Soud, M. Alreshidi, Y. M. Hawsawi, A. Tippana, V. R. Pasupuleti and M. I. Hassan, Bax/Bcl-2 Cascade Is Regulated by the EGFR Pathway: Therapeutic Targeting of Non-Small Cell Lung Cancer, Front. Oncol., 2022, 12, 869672–869694 CrossRef CAS PubMed.
- C. F. A. Warren, M. W. Wong-Brown and N. A. Bowden, BCL-2 family isoforms in apoptosis and cancer, Cell Death Dis., 2019, 10, 177 CrossRef PubMed.
- S. Qian, Z. Wei, W. Yang, J. Huang, Y. Yang and J. Wang, The role of BCL-2 family proteins in regulating apoptosis and cancer therapy, Front. Oncol., 2022, 12, 985363–985378 CrossRef CAS PubMed.
- P. E. Czabotar and A. J. Garcia-Saez, Mechanisms of BCL-2 family proteins in mitochondrial apoptosis, Nat. Rev. Mol. Cell Biol., 2023, 24, 732–748 CrossRef CAS PubMed.
- Z. Zhang, L. Hou, D. Liu, S. Luan, M. Huang and L. Zhao, Directly targeting BAX for drug discovery: Therapeutic opportunities and challenges, Acta Pharm. Sin. B, 2024, 2, 120–132 Search PubMed.
- M. Guo, M. Lv, Y. Shao, W. Zhang, X. Zhao and C. Li, Bax functions as coelomocyte apoptosis regulator in the sea cucumber Apostichopus japonicus, Dev. Comp. Immunol., 2020, 102, 103490–103503 CrossRef CAS PubMed.
|
This journal is © The Royal Society of Chemistry 2024 |