DOI:
10.1039/D3RA01633A
(Review Article)
RSC Adv., 2023,
13, 17297-17314
A systematic assessment of matrix-assisted laser desorption/ionization time of flight mass spectrometry (MALDI-TOF MS) application for rapid identification of pathogenic microbes that affect food crops: delivered and future deliverables
Received
13th March 2023
, Accepted 20th May 2023
First published on 9th June 2023
Abstract
MALDI-TOF MS has decades of experience in the detection and identification of microbial pathogens. This has now become a valuable analytical tool when it comes to the identification and detection of clinical microbial pathogens. This review gives a brief synopsis of what has been achieved using MALDI-TOF MS in clinical microbiology. The major focus, however, is on summarizing and highlighting the effectiveness of MALDI-TOF MS as a novel tool for rapid identification of food crop microbial pathogens. The methods used and the sample preparation methodologies reported thus far have been highlighted and the challenges and gaps and recommendations for fine tuning the technique have been put forth. In an era where anything close to the health and welfare of humanity has been considered as the top priority, this review pitches on one such relevant research topics.
1. Introduction
Matrix-assisted laser desorption/ionization Time of Flight Mass spectrometry (MALDI TOF MS), is one of the soft ionization methods that detect molecular ions from the analyte, with the aid of laser energy generally from UV or IR range lasers and matrices.1,2 Ever since the discovery of MALDI TOF MS, it has been employed for the analysis and identification of a vast variety of molecules. MALDI MS technology have become popular among analytical methods because of its rapidity, accuracy, simple sample preparation methods, minimal fragmentation and ability to yield precise information of the analyte molecules. These features have enabled its consideration and applications as one of the most important analytical tools in physical, chemical, biological, engineering and medical research.3 The role of MALDI MS is humongous when it comes to the analysis of biopolymers such as proteins,4,5 peptides,6,7 saccharides,8–10 and nucleic acids.11,12 Further research has also been extended to small molecule analysis.13,14 Apart from these, in medical sciences, MALDI MS has been employed to unravel the molecular dysfunctions in human diseases i.e., cancer,15 cardiovascular,16 and liver-associated diseases17 utilizing different sample processing protocols.
Though MALDI MS-based microbial identification method is a burgeoning platform, it has extensively been reported for the detection and identification of bacteria in clinical samples,18–21 mycobacteria,22–24 fungi,21,25,26 viruses27,28 protozoans and arthropods.29,30 With respect to microbial identification, the clear edge lies in its low cost and short analysis time, compared to the traditional microbial identification methods. Generally, for microbial identification via biochemical and 16S/18S rRNA gene sequencing, approximately 48 h and an operational cost of USD 100 will be required.31 In MALDI-TOF MS based bacterial identification system, the organisms can be directly identified by subjecting either intact cells or cell extracts along with matrix solution for direct MALDI-TOF MS analysis. This has enabled the technique to be become an attractive option for microbial identification, strain typing, epidemiological studies and detection of biowarfare agents, water and food-borne pathogens, antibiotic resistance microbes, and pathogens from the blood and urinary tract.20,32 Unlike organism-specific procedures of the traditional microbial identification methods, the generalized sample preparation procedure devised for MALDI TOF MS-based microbial identification for a plethora of organisms including bacteria, fungi, and yeast, makes it a unique standalone method in microbial identification.
Plant resources, in particular food crops, are prime sources that the human society rely on for basic survival. MALDI MS platform has been effectively applied for the identification of food-associated pathogenic bacteria like Campylobacter sp. Cronobacter sp., Listeria sp., Salmonella sp. and Vibrio sp. and their clinical isolates after isolating them from their respective food sources and subjecting them to MALDI MS analysis.33–40 Food crops are often affected by a wide variety of plant pathogens, that inflict severe damage to food crops. This triggers annual agricultural trade losses amounting to $220 billion worldwide (FAO, 2019). It is important to identify plant bacterial pathogens to control their impact on plant growth and health. Generally, plant diseases had been studied using MALDI TOF MS with traditional sample preparation methods via extracting samples (protein/metabolites), separating them on either columns/gels (1DE or 2DE), excision of differentially expressed protein spots, in-gel digestion and subsequent analysis of digested protein/peptide using MALDI TOF MS.41 This has been the overall protocol, that has been prevalently reported by previous workers in this area. However, there is an impeding limitation in this conventional protocol, with working on MALDI MS-based identification of plant pathogens either from cultures or from pre-processed samples of the cultures/infected plant tissues. In this case, the MALDI MS-based plant pathogenic microbial identification is only possible if MS reference spectra of the type strains of specific genera/species/subspecies/strains are available on the standard database. This becomes a constraint limiting the identification of unknowns. Also, compared to the other specialized areas of microbial identification using MALDI-MS, plant disease-causing pathogen identification is still in its primitive phase.
The objective of this review is to project an overview of the status and recent applications of MALDI-TOF-MS for microbial identification. The usefulness of this exciting new technology for the diagnosis of plant diseases (resulting from bacteria, viruses, and fungi), has been summarized based on compiling the published reports in this field. The existing methodologies have been listed and the state-of-the-art updated methodologies have been reported and the future recommendations for fine-tuning this technique, to break the current limitations have been discussed and presented.
2. MALDI-TOF MS operating principle and workflow in the context of microbiology
MALDI-MS operates through desorption and ionization of the analyte molecules with the aid of matrix and laser energy. The time-of-flight of the accelerated charged analyte molecules (m/z) is then detected by the detectors (Fig. 1). The mass-to-charge ratio of the analyte molecules is determined based on the following equation:
where e is the charge of an electron; V is the voltage; t is the time-of-flight of the analyte and L is the length of the tube.
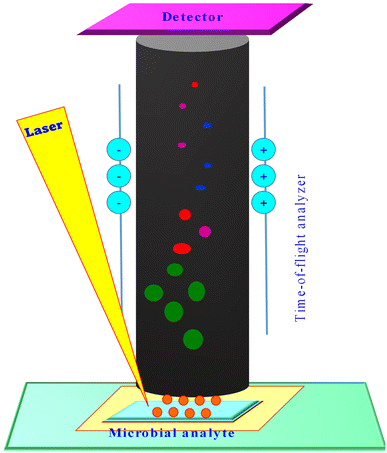 |
| Fig. 1 Schematic showing components of MALDI-TOF MS. | |
MALDI MS sample preparation involves the addition of an analyte and matrix compound (which is an organic compound that absorbs laser energy), the matrix ionizes the adherent analyte molecules via proton transfer. The matrix can be added by thorough mixing or simple overlay onto the samples spotted on MALDI-MS target plates. During MALDI-MS analysis, samples that are co-crystalized with matrix will be ionized on irradiation by the laser beam. The singly protonated ions desorb from the sample spot and are accelerated at a fixed potential to reach the detector positioned at the TOF tube according to their mass-to-charge ratio. MALDI TOF instruments that integrate an ion mirror to increase the analyte ion flight distance, provide increased accuracy of analysed molecules.42,43 The characteristic mass spectrum of the analytes is in accordance with the TOF information from the molecular ions resulting from the samples (Fig. 1).
Fig. 2 presents the steps involved with respect to biological sample preparation for MALDI MS analysis. Generally, when it comes to bioanalysis, MALDI MS has predominantly been employed for the analysis of proteins rather than other biomolecules. In case of protein analysis, proteins from biological samples i.e., cells and tissues from animal and plant origin are extracted using the appropriate solvent or buffer system and analyzed in MALDI MS either as crude samples or purified products using column chromatographs, 1DE and 2DE gel separations. In addition to protein identification, MALDI MS has also been used for the analysis of other biomolecules such as nucleic acids, polysaccharides, lipids, and small organic compounds from all forms of life.4–7,9–14 As in the case of plant and animal protein analysis, protein preparation for the identification of microbes, especially for the detection of cellular proteins, require breaking of cell wall via sonication/extraction using solvent or buffer system prior to MALDI MS analysis. However, MALDI MS of microbial extracellular protein analysis involves using salt/solvents and purification using chromatographic/gel-based methods. MALDI MS has thus emerged as a powerful tool for characterizing innumerable proteins and other biomolecules and metabolites of microbial origin.
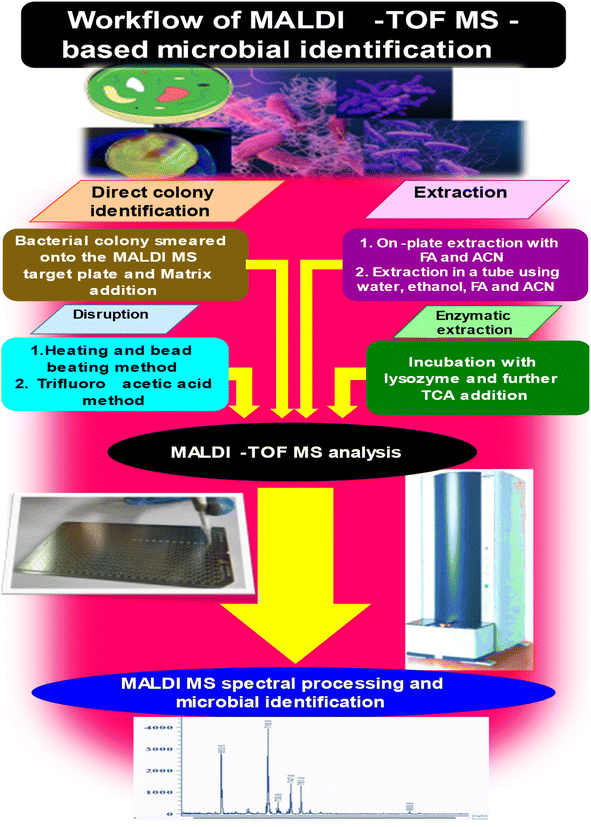 |
| Fig. 2 Overall work flow of steps involved in microbial identification using MALDI-TOF MS. TCA-trichloroacetic acid; FA-formic acid; ACN-acetonitrile. | |
Of late, the technique has been extended to identify microbes, especially bacteria and fungi based on the molecular signature peaks obtained from MALDI MS analysis. The signature peaks obtained from MALDI MS analysis of intact bacterial samples (intact cell MALDI-MS) or protein preparations of whole bacterial cells are predominantly the ribosomal proteins of the bacteria which are generally unique for each bacterial species. These provide critical information that helps distinguish bacterial types within a short period of time.19,44 MALDI-TOF MS-based microbial identification does not rely on marker ion signals but rather it considers the whole MALDI MS profile spectra that predominantly consisted of highly conserved ribosomal proteins of bacteria that are specific upto genus, species, and even subspecies level.35,45,46
3. MALDI MS spectrum-based identification of microbial pathogens
The basic principle behind the identification of microorganisms using MALDI MS, particularly bacteria, relies on the mass spectral profile of that specific organisms in comparison with the reference strains available either in a constructed library or with public proteomics/genomics databases.46,47 The accuracy of bacterial identification, represented by the identification scores, absolutely depends on the sample pre-processing methods involved, including the uniformity in protocols followed and the handling skills of the technician for the reference and test bacterial strains.47,48
3.1. Methods of sample preparations
For MALDI MS based microbial identification, MALDI MS instruments from four different manufacturers namely Bruker Daltonics (Microflex), Shimatzu inc., (Axima Assurance system), VITEK MS (bioMérieux Inc.) and Autobio Inc., (Autof MS 2000) have been predominantly used. These instruments are endowed with MS reference spectral database consisting of around 5000 species of bacterial and fungal origin. Out of the MALDI MS instruments used, Microflex of Bruker is the most extensively used instrument for microbial identification.49–53
Fig. 2 represents the different sample preparation methods that have been in practice for the MALDI MS identification of bacterial isolates. Different sample preparation methods (pre-analytical processing) have been followed for generating spectra for the identification of the bacterial/fungal isolates in MALDI MS-based microbial identification. These methods include (i) direct colony identification,54–56 (ii) extraction,55–57 (iii) disruption58 and (iv) enzymatic extraction.54,59
In the direct method, a small portion of the bacterial colony was smeared onto the MALDI MS target plate and co-crystallized by overlaying 1 μL of the desired matrix. After drying for 10 min, the samples were analyzed using MALDI MS.54–56 In the on-plate extraction method, the bacterial smear on MALDI MS target plate was extracted by adding 0.5 μL of 70% formic acid and 0.5 μL of acetonitrile. The sample was then analyzed using MALDI MS after adding 1.5 μL matrix solution and allowing it to dry for 10 min.56 Yet another method is evolved with ethanol and formic acid mixture for the extraction of molecules, predominantly proteins, for MALDI MS-based identification of bacteria. In this method, a loop full of the bacterial colony was suspended and vortexed in 300 μL of molecular-grade water and further 900 μL of 100% ethanol was added to the mixture, followed by centrifugation (12000 rpm for 2 min) and reconstitution of the pellet in 70% formic acid, lastly acetonitrile (equal volume) was added to prepare the sample for MALDI MS analysis. From the sample 1 μL of the sample and the matrix were spotted and analysed in MALDI MS.55,57 Additionally, the cells in water–alcohol mixture can be subjected to heating (95 °C for 1 h) or 3 cycles bead beating (60 s) for disruption-based protein/molecule extraction.58 Similarly, trifluoro acetic acid (TFA) is also used for the extraction of protein/molecules from the bacteria. In this method, a loop full of culture from the agar plates is suspended in 50 μL of 80% TFA, vortexed after addition of 150 μL distilled water and 200 μL of pure acetonitrile, and centrifuged (12
000 rpm for 2 min). One μL sample was analyzed as described above.55
Generally, all MALDI MS-based microbial identification systems worked on a similar principle, however, the workflow followed for the preanalytical process of samples, such as extraction procedure, the matrix used and the database constructed by the different manufacturers are different.60,61 The accuracy of identification depends on the sample preparation procedure followed. The reference spectral data from the database of each manufacturer differs; it reflects on the accuracy of identification while using the spectral data obtained from other instruments. The mode of processing of the acquired spectra from the test organisms differs. In the Biotyper (Bruker Daltronics) platform, multiple spectra of a test strain can be processed, a representative spectral profile is generated, which is used to search the database for the most similar matches, leading to identification.62 On the other hand, in Vitek MS (BioMerieux), a single spectrum is generated out of ten spectra of the test strains after growing them on different media and growth conditions.63
3.2. Processing of MALDI MS spectral information for pathogenic microbe identification
The MALDI MS spectra (2000–20000 Da) of an unknown microbe obtained using any of the methods described in Section 3.1 is compared with a similarly constructed reference spectral library for hitting on the identity of an unknown test microbe, using statistical analysis packages such as, principal component analysis (PCA), dendrograms and composite correlation matrix. This leads to identifying clinically irrelevant microbes from other environmental niches, including soil, water, air, plants, and others. According to Ashfaq et al.,64 of the total MALDI-MS-based microbial identification from environmental samples, plants sample analysis contributed nearly 20.45%. Thus, the ability of MALDI MS for the rapid identification of clinically important microbes has been proven to be equal to or even better than the conventional identification methods.20,64–66 Although the MALDI MS is employed broadly for the identification of clinical pathogens of various diseases, we have given a brief overview of the selective studies where MALDI-MS has been successfully used to identify pathogens from diseases such as urinary tract infection, a bacterial infection in blood cultures, cerebrospinal fluids and respiratory tract infections.
3.3. MALDI MS-based identification of clinical pathogens
3.3.1. Urinary tract pathogens. MALDI-MS surpassed the conventional pathogenic identification methods with respect to urinary tract infection because of its rapidity and accuracy. In this method, first, the leukocytes are removed by differential centrifugation and the pathogens collected are subjected to MALDI MS identification.67–70 Further, Demarco and Burnham,71 used diafiltration-assisted MALDI MS analysis to identify uropathogens namely Escherichia coli, Klebsiella pneumoniae, and Proteus mirabilis, after it was desalted, fractionated, and concentrated, the urine samples were used to MALDI MS. Recently, Doncevic et al.,72 had developed a tandem mass based MALDI MS method, and the custom program package for the identification of pathogens using peptide tandem mass library contained with 20
917 bacterial species which helped to identify the organisms without the need for bacterial culturing, from urine samples.
3.3.2. Blood infections. Bloodstream infections are a serious concern in that they can cause mortality (3–8% through endocarditis) through infestation of bacterial or fungal microorganisms in the bloodstream73 and MALDI MS-based rapid pathogen identification would be useful for choosing appropriate antibiotic treatments without delay, through early detection. The application of MALDI MS for the identification of blood-stream infection was reported for the first time by La Scola and Raoult.74 Further, Foster et al.,75 developed a rapid lysis centrifugation method for identifying microorganisms in positive blood cultures in the Vitek MS system (bioMérieux) by which 253 positive monomicrobial blood cultures were identified to the genus level (92.1%) and species level (88.1%). In addition to that, Haigh et al.,76 and Tadros and Petrich,77 also identified the blood stream infection by MALDI MS directly from blood culture bottles. Short-term incubation of blood cultures on a solid medium was found to enhance the diagnostic/identification efficiency of MALDI MS in the identification of microbes in blood stream infection.78–81
3.3.3. Respiratory tract infections. Pneumonia affects the respiratory tract in children and the elderly population and it accounts for 14% death in children under 5 years. Although Pneumonia is caused by a variety of pathogens, Streptococcus pneumonia, Legionella pneumophila, are the only two organisms identified by MALDI MS in which Streptococcus pneumonia from nonpneumococcal S. mitis group species was successfully distinguished with <1% (1/116 isolates) misidentification in Vitek MS v2.0 MALDI-TOF-MS System.82 On the other hand, out of 75 isolates studied, 64 were precisely identified to species level using MALDI Biotyper (version 2.0; Bruker Daltonics).83 Recently, MALDI MS based Optochin susceptibility test which is the standard test for differentiating Streptococcus pneumoniae from other Streptococcus mitis group Streptococci was done.84 More recently, a systematic review had been published on the identification of pathogens that cause blood stream infection using MALDI MS tool.85Apart from that, MALDI MS based Klebsiella pneumonia identification from Cerebrospinal fluid of a patient with bacterial meningitis is reported.86,87 The effectiveness of MALDI MS in anaerobic pathogenic bacterial identification was assessed using meta-analysis of 28 studies covering 6685 anaerobic bacterial strains. The outcome of the study showed that the identification accuracy of anaerobic bacteria for species level was 84% (I2 = 98.0%, P < 0.1), and 92% (I2 = 96.6%, P < 0.1) at species and genus levels respectively.88
4. MALDI MS-based identification of plant-associated microbes
4.1. Endophytes
The microbes generally associated with plants pose both beneficial and harmful effects. Plant growth promotion is one of the beneficial effects, caused by microbes. On the other hand, plant diseases caused by microbes are the predominant harmful effect. In most cases, those microbes are predominately identified by 16S rRNA gene sequencing. However, after the development of MALDI MS-based microbial identification, it was largely extended to identify plant-associated bacteria and fungi. To check the feasibility of MALDI MS in assessing bacterial diversity in wheat roots, in comparison with 16S rRNA sequencing, Stets et al.,89 isolated the bacterial isolates from the root extracts of wheat plants and subjected them to Autoflex II MALDI MS (Bruker daltonics inc.) and analyzed the spectra on Speclust algorithm,90 a clustering platform. It was this preliminary study that proved that the mass spectra could be clustered.
Mathuri et al., (2012) also used MALDI MS for clustering studies of spectral clustering along with 16S rRNA gene sequence-based identification of the endophytic bacterial isolates from various tissues of banana plants. Their study revealed that 43 bacterial isolates that cluster with Pseudomonas, Enterobacter, Ewingella, Bacillus, Rahnella, Serratia, Yokenella, Enterobacter, Yersinia, Raoultella, and Klebsiella species, and most of them possessed plant growth promoting (PGP) activities through nitrogen fixation, phosphate solubilization, and siderophores production. In another study, Tani et al.,91 studied methylotrophs diversity by isolating them from 115 plant species on methanol agar plates and identifying them using whole-cell MALDI MS otherwise called WS MS. Apart from that, Sura-de Jong et al.,92 studied the cultivable endophytic bacterial diversity of hyper selenium accumulators namely, Stanleya pinnata and Astragalus bisulcatus roots, stems, leaves through MALDI MS/Biotyper platform and 16S rRNA gene sequencing that revealed the presence of bacterial endophytes such as Variovorax, Bacillus, Staphylococcus, Pseudomonas, Paenibacillus, Advenella, Pantoea, and Arthrobacter.
Further the endophytic bacterial diversity was assessed from different plant tissues of Curcuma zedoaria, white zinger, by Sulistiyani et al., (2018) after isolating them, and subjecting the isolates to MALDI MS (Axima system). The authors claimed that the technology identified 16.67% and 34.85% respectively to genus and species level, accurately (Sulistiyani et al., 2018). Using ultraflex Xtreme mass spectrometer (Microflex-Bruker Daltonics/BioTyper™), Junior et al.93 elucidated the cultivable diversity of endophytic bacteria that showed a very promising effect on the growth of garlic plants (Allium sativum L.).93 The organisms subjected to the analysis (N = 48) were distinctively grouped into 11 groups based on the mass signatures and 54.2% (26 isolates) and 16.7% (8 isolates) were reliably identified at the species and genus level respectively based on their score values demarked by Bruker, (2019).
Toubal et al.,94 characterized the endophytic bacterial diversity of Algerian Great Nettle (Urtica dioica L.), a medicinal plant used to treat painful muscles and joints, eczema, arthritis, gout, and anemia in Algeria using MALDI-TOF MS. In that study, the authors isolated 57 endophytic bacteria using the MALDI MS platform (Microflex-Bruker Daltonics/BioTyper™) that categorized them into 11 bacterial species. Similarly, using Burker MALDI MS/Biotyper platform, endophytic bacterial diversity was assessed from Canola, after isolating them on Tryptone Soy Agar (TSA) medium, and MALDI MS was able to efficiently identify 94% of the isolates with high score values (2.0) in the Biotyper 3.0 database.95 The endophytes dwelling in the nodules of three legumes of the Phaseoleae tribe (cowpea, soybean or siratro) were examined by MALDI MS.96 The authors obtained bacteroids from the nodules by crushing the nodules, removing the debris and collecting the bacteroids by centrifugation (20
000g). The bacteroids were used for MALDI MS (AXIMA Simatzu corp)/SARAMIS platform-based identification by following preanalytical protocols such as: (i) the direct smear method, (ii) a suspension in 25% formic acid, or (iii) a pre-purification step for cellular proteins via extraction with acetonitrile and 70% formic acid. For the detection/identification of endophytic bacteria, researchers rely on pure bacterial cultures isolated from plant tissues and MALDI MS databases developed by the manufacturers since culturing of bacteria is a time-consuming process. Therefore, we have shortened the time of identification of endophytic bacterial analysis, using lysozyme as MALDI MS biosensors for the identification of root nodule bacteria. We have detected and identified endophytic bacteria from peanut plants (Arachis hypogaea L.) directly from a complex real-world sample. For the identification of bacteria from the root nodules of peanut plants, we crushed the roots and pre-treatment them with lysozyme biosensors and studied the supernatant using MALDI MS coupled with Biotyper platform. We have successfully identified Rhibizobial species on the inhouse extended Bruker database.59 Furthermore, Forty-two Endophytic fungal (Colletotrichum) isolates from Astrocaryum sciophilum, ommon palm trees of amazon forest were verified by Bruker MALDI MS platform coupled with Biotyper97 and the analysis grouped the isolates into 18 distinctive groups and thus the authors had elucidated the strain variability of the variousColletotrichum strains using MALDI MS tool.
4.2. Plant pathogens
Identification/detection of plant pathogens is a critical prerequisite for the management of plant disease and economic losses caused by plant pathogens. It is well known that MALDI MS is known for its speed and accuracy in the identification of clinical pathogens and various reports concerning the identification of human pathogens are adequately available. However, the application of MALDI MS related to the identification of phytopathogens is comparatively lesser than other human pathogens identified. In plant pathology, MALDI MS has become an unavoidable tool for studying plant-microbe interaction, in particular, molecular interactions through proteomics, metabolomics. Using both these techniques, protein or small molecules have been studied in MALDI MS after separation i.e., for protein separations, 2D gel electrophoresis or columns have been used. More recently, we have consolidated the role of MALDI MS in plant pathology research41 that gives the broader picture of MALDI MS and it supportive techniques in elucidating the molecular changes under different pathological conditions. Although MALDI MS is a renowned analytical technique for bacterial/fungal identification or detection, research on phytopathogen detection using MALDI MS is limited.
Intact cell MALDI MS was applied to facilitate the identification of Pantoea stewartii subsp. stewartii that causes Stewart's wilt in sweet corn.98 The authors isolated the bacteria from infected plant parts and washed them in distilled water and subjected to MALDI MS analysis after lysing them in 70% ethanol. The identity was validated on Biotyper platform using signature peak comparisons correlated with that on the database. Though the MALDI MS method identified a bacterial agent that causes Stewart's wilt, it was not extended to real sample analysis. It is crucial to identify wilt-causing plant pathogenic Clavibacter michiganensis (consisting of five subspecies), infecting a wide range of host plants such as alfalfa, tomato, corn, potato and wheat. To identify such bacteria (sub species of C. michiganensis), Zaluga et al.,99 used the protein extraction method prescribed by Bruker Daltonics [Bruker, 2019/24] and analysed them in 4800 Plus MALDI TOF/TOFTM Analyzer (AB Sciex, Belgium) and clustered the similar isolates by analysing the spectral peaks using the UPGMA (unweighted pair group method with arithmetic mean) clustering algorithm. To confirm the bacterial MALDI MS analysis, Gyr B sequence analysis was also carried out. Although the authors used AB Sciex mass spectrometer, they have used Bruker daltonics' preanalytical sample processing methods.
The pathogens Acidovorax oryzae which causes bacterial stripe in rice and Acidovorax citrulli that causes bacterial fruit blotch (BFB) in cucurbit plants are very difficult to differentiate. Therefore, Wang et al.,100 used MALDI-TOF MS in combination with FTIR to successfully differentiate both strains. The isolated cultures of both strains were subjected to MALDI MS analysis after a single water wash and 22 MALDI MS peaks were identified specifically conserved belonging to Acidovorax oryzae and 18 peaks of Acidovorax citrulli; based on the conserved spectral peaks the strains were differentiated. MALDI MS has been reported to be one of the most reliable techniques for the identification of Dickeya and Pectobacterium species, which are responsible for blackleg and soft rot diseases in a broad range of commercially important plants. In apple and pear pathogens that cause soft rot such as Erwinia amylovora and Erwinia pyrifoliae and other epiphytes of Erwnia species such as Erwinia tasmaniensis and Erwinia billingiae, MALDI MS/biotyper platform and PCR based sequencing analysis of highly conserved genes (Wensing, et al., 2012) came handy. In another study, Niu et al.,101 MALDI MS equipped with post-source decay was applied to identify the compounds isolated from a Rhizobacterium species, exhibiting strong antagonistic activity against a fire blight causing pathogen Erwinia amylovora Ea 273, and soft rot causing E. carotovora.101 The authors, based on the results of MALDI-MS analysis, reported that those compounds were polymyxin P1 and polymyxin P2.
The role of symbiotic endophytic bacterium, Enterobacter cloacae in banana plants was investigated by Macedo-Raygoza et al.,102 using intact MALDI MS cum Biotyper platform based methods. They also employed MALDI MS for studying nitrogen transfer from symbiotic endophytic bacterium to the host plant, which raises its resistance against the Black Sigatoka Pathogen, Pseudocercospora fijiensis. Recently, MALDI TOF MS was used to study rice seedlings infected with sheath blight and bacterial leaf blight pathogens Rhizoctonia solani and Xanthomonas oryzae pv. oryzae, respectively.103 The leaf proteome of rice seedlings were separated on 2DE gels and the differentially expressed proteins were identified using MALDI TOF MS.
Salplachta et al.104 had studied the feasibility of 3 analytical methods to differentiate the strains of Dickeya and Pectobacterium species. The study was conducted with 43 strains from Dickeya and Pectobacterium species which include D. dianthicola, D. dadantii, D. dieffenbachiae, D. chrysanthemi, D. zeae, D. paradisiaca, D. solani, P. carotovorum, and P. atrosepticum. The organisms were differentiated using simple mass signatures. Kajiwara,105 identified bacterial pathogens, such as Burkholderia glumae and Burkholderia gladioli pv. gladioli that cause bacterial panicle blight of rice and Erwinia chrysanthemi pv. Zeae, a causative organism for bacterial foot rot, from the extracts of infected seedlings using MALDI MS/Biotyper platform with significantly high scores. In this case, the authors claimed that the spectral peaks of the host plant tissues did not affect pathogen identification.
Other studies conducted by Fonseca-Guerra et al.;106 Oberhaensli et al.;107 Choi et al.,108 identified plant pathogens that cause disease in plants. Whole cell MALDI MS was employed for the identification of pathogen that cause blight in Miscanthus sinensis, that grows on Han River banks in Seoul, South Korea.108 The authors identified that the blight causing pathogen was Pseudomonas syringae pv. syringae (Pss), after isolating the bacterial pathogen from the infected tissues and subjecting intact cells for MALDI MS analysis with an appropriate MALDI matrix. In addition to that, Sawada et al.,109 also employed MALDI MS to identify bacteria causing rot disease in Japanese turnip. In that study, the pathogens were isolated from the infected turnip plant tissues and bacteria from the colonies were identified as Pseudomonas grimontii using MALDI MS/biotyper platform. The identity of the pathogen was also cross-verified by biochemical and physiological characterization, 16S rRNA, rpoD, gyrB, and rpoB genes sequence analysis. Furthermore, MALDI MS/Biotyper based analysis identified pathogenic strains of Pseudomonas syringae that cause brown leaf spot in Quinoa (Chenopodium quinoa).106 Their study involved isolation of the bacterial pathogen from infected quinona leaves and spectral analysis in Biotyper.
Phytophthora, genus of an oomycete fungi (P. infestans) and the causative agent of potato blight (responsible for a series of famines in Europe) consists of many species. In another study by Oberhaensli et al.,107 rhizoplane bacterial diversity having antagonistic potential against Pythium sp. that cause damping-off disease in Lepidium sativum L. were studied using MALDI MS. The authors identified Aeromonas media and Enterobacter cloacae as the dominating bacteria in the plants' rhizoplane. Božik et al.,110 applied MALDI MS/Biotyper platform to study species-level variation of 43 strains of Phytopthora. For this, the authors constructed and validated a spectral library consisting of 144 main spectra (MSPs). Thus, the application potential of MALDI-TOF MS technique is more profound in plant and agricultural sciences; however, in identifying plant growth promoters, plant pathogens, and other plant-associated bacteria MALDI-MS applications are limited. Very recently, Niculau et al.,111 had developed MALDI MS-based methods to directly identify citrus canker from the infected leaves and the causative agent Xanthomonas citri subsp. citri bacterial isolate. Although MALDI MS could identify the causative organisms only upto the genus level directly from the infected spot, this technique was effective for the rapid diagnosis of citrus canker.
Further, Chowdappa et al.,112 have distinguished plant pathogenic fungi, based on protein markers expressed in intact cells using MALDI MS. The protein fingerprint-based analysis grouped 12 Alternaria species (with high similarity in spectral characteristics) out of 60 isolates of Alternaria species studied, using direct intact MALDI MS. In addition, a fungal pathogen, Phytophthora infestans, causing blight disease in tomato plants and enormous economic loss was identified using a combination of LC-MS and MALDI MS. The authors identified the expression of metabolite biomarkers such as tomatidine and saponins as infection biomarkers and early infection metabolite markers respectively and Isocoumarin as early and late asymptomatic infection marker.113 MALDI MS was also applied to discriminate Tilletia controversa from the T. caries/T. laevis complex by MALDI-TOF MS analysis of teliospores, which is a pre-requisite for treating the diseases caused by them.114 These organisms cause devastating damage in various Poaceae crops by causing different bunt diseases. The species discrimination was carried out using the MALDI MS spectra obtained using Microflex LT MALDI-TOF mass spectrometer (Bruker Daltonics) and MALDI Biotyper 3.0 software combinations. Table 1 presents a consolidated list of the various studies reported using MALDI-TOF MS-based identification of food crop-associated microbes.
Table 1 MALDI MS methodology followed for the direct identification of plant associated bacteria
No. |
Source for microbial studies |
Nature of microorganism |
MALDI MS instrument |
Matrix solution used |
Method |
Database for identification |
Organism identified |
Reference |
1 |
Roots/root nodule of Arachis hypogea |
Endophytic bacteria/bacterioids |
Microflex, (Bruker Daltonics Inc.) |
10 mg mL−1 HCCA in 50% ACN, 2.5% TFA |
Place a small portion of a single colony directly onto a clean MALDI target plate and overlay 1 μL of matrix on the sample. Analyze the sample in MALDI MS after it gets complete dryness at room temperature |
Biotyper 1.0 software |
Strains of Rhizobium sp. |
Manikandan et al.59 |
2 |
Root nodules from G. max, M. atropurpureum and V. unguiculata |
Endophytic bacteria/bacterioids |
Axima™ Confidence machine (Shimadzu-Biotech Corp.) |
A saturated solution of CHCA in 33% acetonitrile, 33% ethanol, and 3% TFA |
(i) Direct smear method, (ii) on spot extraction with 25% formic acid, and (iii) extraction with ACN and formic acid (70%). Overlaying 1 μL of matrix on samples |
SARAMIS™ Superspectrum tool |
Strains of Sinorhizobiumgenus |
Ziegler et al.96 |
3 |
Leaves of tropical palm, Astrocaryum sciophilum |
Endophytic fungi |
UltrafleXtreme mass spectrometer (Bruker Daltonics, Inc.) |
CHCA matrix (50 mg mL−1 in ACN/H2O (50/50) containing 0.1% TFA) |
Washing solution (300 μL of water and 900 μL of 100% ethanol), centrifugation (13 000 rpm). Resuspend the pellet in 40 μL of formic acid and 40 μL 100% acetonitrile. Use the supernatant after centrifugation at 13 000 rpm, as a sample. Overlaying 1 μL of CHCA on the sample spot |
Biotyper 3.0 software |
Colletotrichum strains |
Barthelemy et al.97 |
4 |
Garlic roots, stem leaves |
Endophytic bacteria |
UltrafleXtreme mass spectrometer (Bruker Daltonics, Inc.) |
CHCA matrix (50 mg mL−1 in ACN/H2O (50/50) containing 0.1% TFA) |
Bruker standard sample preparation protocol |
Biotyper 3.0 software |
Strains of Bacillus subtilis, B. marisflavi, B. koreensis, B. megaterium, Enterobacter cloacae, Pantoea dispersa, Rhizobium radiobacter, Paenibacillus massiliensis, Lactobacillus paracasei, Burkholderia cepacian, Azospirillum brasilense |
Júnior et al.93 |
5 |
Great Nettle (Urtica dioica L.) stems, leaves and roots |
Endophytic bacteria |
Microflex LT MALDI-TOF mass spectrometer (Bruker Daltonics Inc.) |
CHCA matrix (saturated solution of CHCA in 500 μL of 50% (v/v) ACN, 250 μL of 10% (v/v) TFA) and 250 μL of H2O) |
Smearing of bacterial colony and addition of 1.5 μL of the matrix solution |
Biotyper 3.0 software |
Strains of Bacillus anthracis, B. megaterium, B. pumilus, B. cereus, Escherichia coli, Pantoea agglomerans, Enterobacter amnigenus, Staphylococcus cohnii, Enterococcus faecium, Paenibacillus lautus, P. glucanolyticu |
Toubal et al.94 |
6 |
Curcuma zedoaria |
Endophytic bacteria |
MALDI-TOF MS Axima system (Shimadzu-Biotech Corp.) |
CHCA matrix 1 μL each of CHCA: ethanol : ACN : water : TFA |
Bacterial cells lysed in 20 μL formic acid (25% v/v). From the supernatant 1 μL matrix solution overlay on samples |
SARAMIS software |
Strains of Alcaligenes faecalis, Bacillus subtilis, Enterobacter aerogenes, Enterobacter, Klebsiella pneumoniae, Pantoea dispersa, Pseudomonas stutzeri, Stenotrophomonas maltophilia |
Sulistiyani and Lisdiyanti, 2018 |
7 |
Wheat roots (Triticum vulgare) |
Endophytic bacteria |
Autoflex II MALDI-TOF (Bruker Daltonics Inc.) |
CHCA matrix (10 mg mL−1) CHCA 10 mg in ACN (50% v/v) and TFA (2.5% v/v) |
Bacterial colonies on a plate and 1 μL matrix solution overlaying on samples. 40 μL of a formic acid (35%) : acetonitrile (50%) mixture and centrifuged at 25 000g for 15 min |
PECLUST (Alm et al., 2006) |
Clustering of bacterial isolates. Strains of Pantoea strains, Pseudomonas, Enterobacter, Acetnobacter |
Stets et al.89 |
8 |
Canola Roots (Brassica napus L. var. oleracea) |
Endophytic bacteria |
Autoflex III MALDI-TOF/TOF mass spectrometer (Bruker Daltonics, Inc.) |
CHCA (10 mg mL−1) in ACN (50% v/v) and TFA (2.5% v/v) |
Washing solution (300 μL of water and 900 μL of 100% ethanol), centrifugation (13 000 rpm). Resuspend the pellet in 40 μL of formic acid and 40 μL 100% acetonitrile. Use the supernatant after centrifugation at 13 000 rpm, as a sample. Overlaying 1 μL of CHCA on the sample spot |
MALDI Biotyper 3.0 software |
Strains of Bacillus subtilis, B. simplex, B. niacin, P. amylolyticus, P. glucanolyticus, P. massiliensis, P. polymyxa, Pseudomonas koreensis, P. thivervalensis |
Martínez-Hidalgo et al.95 |
9 |
Roots, stems, leaves of Astragalus bisulcatus and Stanleya pinnata |
Endophytic bacteria |
Biflex IV MALDI-TOF spectrometer (Bruker Daltonics, Inc.) |
CHCA matrix (10 mg mL−1) in ACN (50% v/v) and TFA (2.5% v/v) |
Smearing of the bacterial colony and addition of 1.0 μL of the matrix solution |
Biotyper 2.0 software |
Strains of Bacillus, Pseudomonas, Pantoea, Staphylococcus, Paenibacillus, Advenella, Arthrobacter, and Variovorax |
Jong et al.92 |
10 |
Pear slices, and necrotic pear plant tissueapple flowers |
Pathogenic bactera |
Microflex LT MALDI-TOF mass spectrometer (Bruker Daltonics Inc.) |
CHCA matrix (10 mg mL−1) in ACN (50% v/v) and TFA (2.5% v/v) |
Bacteria grown in liquid medium, harvested by centrifugation, washed with water resuspending in 0–3 mL water and 0–8 mL ethanol, lysed with 40 μL of 70% formic acid and 40 μL acetonitrile. Supernatant used as samples |
Biotyper 2.0 software |
Erwinia amylovora and Erwinia pyrifoliae, Erwinia tasmaniensis and Erwinia billingiae |
Wensing et al.115 |
11 |
Specimens of intact bunt balls, mainly in the ears of wheat plant |
Pathogenic fungi |
Microflex LT MALDI-TOF mass spectrometer (Bruker Daltonics Inc.) |
CHCA matrix (10 mg mL−1) in ACN (50% v/v) and TFA (2.5% v/v) |
Mycelium/teliospores were suspended in 100 μL of formic acid (70%) and lysed by bead beating and homogenization addition of 100 μL of ACN (100%) and centrifuged at 20 000g. Supernatant used as samples |
Biotyper 3.0 software |
Tilletia controversa, T. caries, T. laevis |
Forster et al.114 |
12 |
Citrus leaves (healthy and infected) |
Pathogenic bacteria causing citrus cancer |
Autoflex speed spectrometer (Bruker Daltonics, Inc.) |
CHCA matrix (20 mg mL−1) in acetonitrile 0.2% aqueous trifluoroacetic acid (1 : 1) |
Spotted samples overlaid by 1.5 μL of the matrix solution |
Biotyper 3.1 software |
Xanthomonas citri subsp. citri |
Niculau et al.111 |
13 |
Quinoa (Chenopodium quinoa) |
Pathogenic bacteria causing leaf spot |
Microflex MALDI-TOF mass spectrometer (Bruker Daltonics Inc.) |
CHCA matrix (10 mg mL−1) in ACN (50% v/v) and TFA (2.5% v/v) |
Smearing bacteria on MALDI MS target plate 1 μL of formic acid and analyzed with 1 μL of organic matrix CHCA |
Biotyper 3.1 software |
Pseudomonas syringae |
Fonseca-Guerra et al.106 |
14 |
Miscanthus sinensis |
Pathogenic bacteria causing leaf blight |
Instrument information not provided |
Matrix information not provided |
The methodology is not provided |
Biotyper 3.0 software |
Pseudomonas syringae pv. syringae |
Choi et al.108 |
15 |
Rice seedlings |
Pathogenic bacteria causing panicle blight, and foot rot |
Ultraflex MALDI-TOF mass spectrometer (Bruker Daltonics Inc.) |
CHCA matrix (10 mg mL−1) in ACN (50% v/v) and TFA (2.5% v/v) |
Bacteria treated with 70% (v/v) formic acid and the same volume of acetonitrile, and centrifuged at 15 000 rpm for 5 min and used for the analysis with 1 μL of organic matrix CHCA |
Biotyper 3.1 software |
Burkholderia glumae, Burkholderia gladioli pv. gladioli, and Erwinia chrysanthemi pv. zeae |
Kajiwara105 |
16 |
Pathogenic cultures |
Pathogenic bacteria causing blackleg and soft rot diseases |
4700 Proteomics Analyzer (Applied Biosystems, Inc.) |
Sinapinic acid matrix solution (16 mg mL−1 in ACN/0.1% TFA, 3 : 2, v/v) in 1 : 1 ratio |
Bacteria treated with EtOH and then with ACN/FA solution (ACN/5% FA, 1 : 1, v/v). The final bacterial suspension was mixed with SA matrix solution and overlaid with the ACN/H2O solution (ACN/H2O, 3 : 2, v/v) |
Information regarding spectral analysis not provided |
Dickeya and Pectobacterium species |
Šalplachta et al.104 |
17 |
Damaged plants of different ornamental, forest and fruit woody plant species |
Pathogenic fungi (oomycetes) |
Autoflex speed MALDI-TOF mass spectrometer (Bruker Daltonics Inc.) |
CHCA matrix solution CHCA 10 mg mL−1 (acetonitrile 50%, water 47.5% and trifluoracetic acid 2.5%) |
Washing solution (300 μL of water and 900 μL of 100% ethanol), centrifugation (13 000 rpm). Resuspend the pellet in 40 μL of formic acid and 40 μL 100% acetonitrile. Use the supernatant after centrifugation at 13 000 rpm, as a sample. Overlaying 1 μL of CHCA on the sample spot |
Biotyper 3.1 software |
Strains in genus Phytophthora and Pythium |
Bozik et al.110 |
18 |
Rice seedlings |
Pathogenic bacteria causing brown stripe on rice |
Autoflex MALDI-TOF mass spectrometer (Bruker Daltonics Inc.) |
CHCA matrix solution CHCA 10 mg mL−1 (acetonitrile 50%, water 47.5% and trifluoracetic acid 2.5%) |
Washing solution (300 μL of water and 900 μL of 100% ethanol), centrifugation (13 000 rpm). Resuspend the pellet in 40 μL of formic acid and 40 μL 100% acetonitrile. Use the supernatant after centrifugation at 13 000 rpm, as a sample. Overlaying 1 μL of CHCA on the sample spot |
Information regarding spectral analysis not provided |
Acidovorax oryzae and Acidovorax citrulli |
Wang et al.100 |
19 |
Isolated from different plant parts |
Pathogen causing diseases in alfalfa, tomato, corn, potato and wheat |
4800 Plus MALDI TOF/TOFTM Analyzer (AB Sciex Inc.) |
CHCA matrix solution CHCA 10 mg mL−1 (acetonitrile 50%, water 48% and trifluoracetic acid 2%) |
Washing solution (300 μL of water and 900 μL of 100% ethanol), centrifugation (18 000 rpm). Resuspend the pellet in 50 μL of formic acid and 50 μL 100% acetonitrile. Use the supernatant after centrifugation at 18 000 rpm, as a sample. Overlaying 1 μL of CHCA on the sample spot |
BioNumerics 5.1 software (Applied Maths, Belgium) |
Clavibacter strains |
Zaluga et al.99 |
20 |
Isolated from leaves and roots |
Endophytic bacteria of banana plant |
Autoflex speed MALDI-TOF mass spectrometer (Bruker Daltonics Inc.) |
Followed manufacturers' instruction |
Followed manufacturers' instruction |
Biotyper 4.1.60. software |
Enterobacter cloacae |
Macedo-Raygoza et al.102 |
5. Future perspectives and recommendations
MALDI MS-based microbial identification platform has been extensively applied for the identification of a wide variety of microbes but is limited to the identification of clinically important microorganisms. Based on the literature survey, it became clearly evident that MALDI MS-based microbial identification tool is applied mostly for the identification of intact microorganisms cultured on media. The lack of reference spectra of food crop pathogens is the major constraint while applying MALDI MS-based microbial identification technology in agriculturally important plant pathogenic microorganism identification. The manufacturers of the database had given the option for building up the existing database with a large number of spectra belonging to various reference strains by the users thus creating an extended in-house database with a greater number of reference strains. Furthermore, the database can also be extended to reference spectra of rare species which would help the researchers to come up with precise and accurate identification strategies for any test microorganisms.
The MALDI MS-based microbial identification tool, when applied for detecting bacteria housed inside plant tissues, requires an extensive preanalytical methodology which is time-consuming, laborious work, and will not support the growth of all the endophytes present in the sample. Based on our expertise, we propose enzyme-based bacterial enrichment, i.e., treating the samples with enzymes that selectively work on plant cell wall molecules. These enriched bacteria/bacteroids can be selectively obtained through differential centrifugation for MALDI MS-based direct identification of endophytes. In other words, the bacterial signals can also be enriched directly from the plant tissues by treating samples with enzymes that specifically work on the bacterial cell wall. The major motive is to simplify the laborious procedures and induct novel techniques that involve a simple digestive process or organism-specific enrichment process that could make the biomolecules available for direct MALDI-MS analysis.
In agriculture, the direct identification of pathogenic microorganisms from the infected tissues of food crops will certainly benefit the farming community from the huge monetary losses resulting from improper plant disease diagnosis and management. For the prompt diagnosis of plant diseases and their effective management, it is suggested that the MALDI MS spectral library needs to be constructed for the infected tissues at different stages of infection for a given food crop so that it can be used for the rapid identification/diagnosis of plant disease using the MALDI MS-based platform.
In a previous review, Manikandan et al.,41 have already raised a concern on the reduced research interest and direction in the area of plant pathogen detection. Compared to the extensive reports that confirm the applicability of MALDI-TOF MS as an analytical tool for analysis of human and animal pathogens, highly reduced reports are available for its plant pathogen applications. This review emphasizes that there is much scope to improvise and expand into this area of research. Speculating the plausible reasons for the reduced use of MALDI-TOF MS for plant pathogen analysis, it is recommended that there is need to collaborate with a plant pathologist or an agriculturalist to an analytical chemist or a mass spectrometrist, to discuss problems and seek solutions. This field being an interdisciplinary one, the major limitations exist in the very fact that it will require specific expertise and inputs from both disciplines. The authors proposed that these cross-domain challenges can be bridged by connecting these extremities through interdisciplinary collaborative research. This pipeline will lead to technology transfer and knowledge transfer and lead to effective solutions and eventual progress. As explained in this section, the botanist/plant biologist/microbiologist will have the technology and expert handling procedures for releasing the plant/microbe bound signature compounds that can lead to identification, on the other hand, the analytical chemist/mass expert will have the analytical hands-on expertise from the instrumentation point of view, to bring about rapid, sensitive and accurate detection of the compounds. Effective progress can only be realized through intricate co-working. This review highlights that, from the 2022 review mentioned above to date, not much progress and upgradation is evidenced. There are various sophistications and variants that have risen from MALDI-TOF-MS, these need to be tested for these plant-microbe applications too.
6. Conclusions
This review emphasizes the usefulness and analytical expertise of MALDI-TOF MS in the rapid detection and identification of microbial plant pathogens that affect valuable food crops. In an era where anything close to the health and welfare of humanity is given top priority, this review pitches on a relevant topic. Early detection is crucial in order to plan treatment strategies and MALDI-TOF MS with its ability for rapid, direct detection of microbes is a valuable asset. The gaps and the recommendations to fill these voids in this area of research have been put forth. The review clearly reinstates the fact that there is a lot more that MALDI-MS as an identification tool can offer and so more research is encouraged to exploit this technique, for the benefit of both the farmers and the consumers.
Author contributions
J. G., M. M., preparation of the original draft, and revisions; I. S., N. H., participated in the review and revisions, funding.
Conflicts of interest
The authors declare no conflict of interest.
Acknowledgements
This article was supported by the KU Research Professor Program of Konkuk University.
References
- M. Karas, D. Bachmann and F. Hillenkamp, Influence of the Wavelength in High-Irradiance Ultraviolet Laser Desorption Mass Spectrometry of Organic Molecules, Anal. Chem., 1985, 57, 2935–2939, DOI:10.1021/ac00291a042.
- F. Hillenkamp, M. Karas, R. C. Beavis and B. T. Chait, Matrix-Assisted Laser Desorption/Ionization Mass Spectrometry of Biopolymers, Anal. Chem., 1991, 63, 1193A–1203A, DOI:10.1021/ac00024a002.
- M. Baker, Mass Spectrometry for Biologists, Nat. Methods, 2010, 7, 157–161, DOI:10.1038/nmeth0210-157.
- V. Greco, C. Piras, L. Pieroni, M. Ronci, L. Putignani, P. Roncada and A. Urbani, Applications of MALDI-TOF Mass Spectrometry in Clinical Proteomics, Expert Rev. Proteomics, 2018, 15, 683–696, DOI:10.1080/14789450.2018.1505510.
- J. Webster and D. Oxley, Protein Identification by MALDI-TOF Mass Spectrometry, Methods Mol. Biol., 2012, 227–240 CrossRef CAS PubMed.
- W. M. Nadler, D. Waidelich, A. Kerner, S. Hanke, R. Berg, A. Trumpp and C. Rösli, MALDI versus ESI: The Impact of the Ion Source on Peptide Identification, J. Proteome Res., 2017, 16, 1207–1215, DOI:10.1021/acs.jproteome.6b00805.
- J. Koehbach, C. W. Gruber, C. Becker, D. P. Kreil and A. Jilek, MALDI TOF/TOF-Based Approach for the Identification of D-Amino Acids in Biologically Active Peptides and Proteins, J. Proteome Res., 2016, 15, 1487–1496, DOI:10.1021/acs.jproteome.5b01067.
- D. J. Harvey, Analysis of Carbohydrates and Glycoconjugates by Matrix-assisted Laser Desorption/Ionization Mass Spectrometry: An Update for 2003–2004, Mass Spectrom. Rev., 2009, 28, 273–361, DOI:10.1002/mas.20192.
- W.-T. Hung, S.-H. Wang, Y.-T. Chen, H.-M. Yu, C.-H. Chen and W.-B. Yang, MALDI-TOF MS Analysis of Native and Permethylated or Benzimidazole-Derivatized Polysaccharides, Molecules, 2012, 17, 4950–4961, DOI:10.3390/molecules17054950.
- J. Wang, J. Zhao, S. Nie, M. Xie and S. Li, MALDI Mass Spectrometry in Food Carbohydrates Analysis: A Review of Recent Researches, Food Chem., 2023, 399, 133968, DOI:10.1016/j.foodchem.2022.133968.
- X. Gao, B.-H. Tan, R. J. Sugrue and K. Tang, MALDI Mass Spectrometry for Nucleic Acid Analysis, Top Curr. Chem., 2012, 55–77 CrossRef CAS PubMed.
- J. C. Joyner, K. D. Keuper and J. A. Cowan, Analysis of RNA Cleavage by MALDI-TOF Mass Spectrometry, Nucleic Acids Res., 2013, 41, e2, DOI:10.1093/nar/gks811.
- P. Wang and R. W. Giese, Recommendations for Quantitative Analysis of Small Molecules by Matrix-Assisted Laser Desorption Ionization Mass Spectrometry, J. Chromatogr. A, 2017, 1486, 35–41, DOI:10.1016/j.chroma.2017.01.040.
- H. Gu, K. Ma, W. Zhao, L. Qiu and W. Xu, A General Purpose MALDI Matrix for the Analyses of Small Organic, Peptide and Protein Molecules, Analyst, 2021, 146, 4080–4086, 10.1039/D1AN00474C.
- E. P. Diamandis, Mass Spectrometry as a Diagnostic and a Cancer Biomarker Discovery Tool, Mol. Cell. Proteomics, 2004, 3, 367–378, DOI:10.1074/mcp.R400007-MCP200.
- C. Mesaros and I. A. Blair, Mass Spectrometry-Based Approaches to Targeted Quantitative Proteomics in Cardiovascular Disease, Clin. Proteomics, 2016, 13, 20, DOI:10.1186/s12014-016-9121-1.
- C. L. Bowlus, E. H. Seeley, J. Roder, J. Grigorieva, H. Roder, R. M. Caprioli and Me. Gershwin, In Situ Mass Spectrometry of Autoimmune Liver Diseases, Cell. Mol. Immunol., 2011, 8, 237–242, DOI:10.1038/cmi.2010.72.
- A. E. Clark, E. J. Kaleta, A. Arora and D. M. Wolk, Matrix-Assisted Laser Desorption Ionization–Time of Flight Mass Spectrometry: A Fundamental Shift in the Routine Practice of Clinical Microbiology, Clin. Microbiol. Rev., 2013, 26, 547–603, DOI:10.1128/CMR.00072-12.
- E. Carbonnelle, C. Mesquita, E. Bille, N. Day, B. Dauphin, J.-L. Beretti, A. Ferroni, L. Gutmann and X. Nassif, MALDI-TOF Mass Spectrometry Tools for Bacterial Identification in Clinical Microbiology Laboratory, Clin. Biochem., 2011, 44, 104–109, DOI:10.1016/j.clinbiochem.2010.06.017.
- N. Singhal, M. Kumar, P. K. Kanaujia and J. S. Virdi, MALDI-TOF Mass Spectrometry: An Emerging Technology for Microbial Identification and Diagnosis, Front. Microbiol., 2015, 6, 791, DOI:10.3389/fmicb.2015.00791.
- S. Angeletti, Matrix Assisted Laser Desorption Time of Flight Mass Spectrometry (MALDI-TOF MS) in Clinical Microbiology, J. Microbiol. Methods, 2017, 138, 20–29, DOI:10.1016/j.mimet.2016.09.003.
- A. El Khéchine, C. Couderc, C. Flaudrops, D. Raoult and M. Drancourt, Matrix-Assisted Laser Desorption/Ionization Time-of-Flight Mass Spectrometry Identification of Mycobacteria in Routine Clinical Practice, PLoS One, 2011, 6, e24720, DOI:10.1371/journal.pone.0024720.
- M. Manikandan, A. Deenadayalan, A. Vimala, J. Gopal and S. Chun, Clinical MALDI Mass Spectrometry for Tuberculosis Diagnostics: Speculating the Methodological Blueprint and Contemplating the Obligation to Improvise, TrAC, Trends Anal. Chem., 2017, 94, 190–199, DOI:10.1016/j.trac.2017.06.014.
- M. Muthu, A. Deenadayalan, D. Ramachandran, D. Paul, J. Gopal and S. Chun, A State-of-Art Review on the Agility of Quantitative Proteomics in Tuberculosis Research, TrAC, Trends Anal. Chem., 2018, 102, 369–378, DOI:10.1016/j.trac.2018.02.004.
- M. Manikandan, H.-F. Wu and N. Hasan, Cell Population Based Mass Spectrometry Using Platinum Nanodots for Algal and Fungal Studies, Biosens. Bioelectron., 2012, 35, 493–497, DOI:10.1016/j.bios.2012.03.020.
- M. Manikandan and H.-F. Wu, Probing the Fungicidal Property of CdS Quantum Dots on Saccharomyces Cerevisiae and Candida Utilis Using MALDI-MS, J. Nanopart. Res., 2013, 15, 1728, DOI:10.1007/s11051-013-1728-9.
- M. I. L. Sjöholm, J. Dillner and J. Carlson, Multiplex Detection of Human Herpesviruses from Archival Specimens by Using Matrix-Assisted Laser Desorption Ionization-Time of Flight Mass Spectrometry, J. Clin. Microbiol., 2008, 46, 540–545, DOI:10.1128/JCM.01565-07.
- I. Sivanesan, J. Gopal, R. Surya Vinay, E. H. Luke, J.-W. Oh and M. Muthu, Consolidating the Potency of Matrix-Assisted Laser Desorption/Ionization-Time of Flight Mass Spectrometry (MALDI-TOF MS) in Viral Diagnosis: Extrapolating Its Applicability for COVID Diagnosis?, TrAC, Trends Anal. Chem., 2022, 150, 116569, DOI:10.1016/j.trac.2022.116569.
- A. Yssouf, L. Almeras, D. Raoult and P. Parola, Emerging Tools for Identification of Arthropod Vectors, Future Microbiol., 2016, 11, 549–566, DOI:10.2217/fmb.16.5.
- A. Vega-Rúa, N. Pagès, A. Fontaine, C. Nuccio, L. Hery, D. Goindin, J. Gustave and L. Almeras, Improvement of Mosquito Identification by MALDI-TOF MS Biotyping Using Protein Signatures from Two Body Parts, Parasites Vectors, 2018, 11, 574, DOI:10.1186/s13071-018-3157-1.
- Y.-X. Liu, Y. Qin, T. Chen, M. Lu, X. Qian, X. Guo and Y. Bai, A Practical Guide to Amplicon and Metagenomic Analysis of Microbiome Data, Protein Cell, 2021, 12, 315–330, DOI:10.1007/s13238-020-00724-8.
- J. Gopal, M. Muthu, S.-C. Chun and H.-F. Wu, State-of-the-Art Nanoplatform-Integrated MALDI-MS Impacting Resolutions in Urinary Proteomics, Proteomics: Clin. Appl., 2015, 9, 469–481, DOI:10.1002/prca.201400182.
- S. B. Barbuddhe, T. Maier, G. Schwarz, M. Kostrzewa, H. Hof, E. Domann, T. Chakraborty and T. Hain, Rapid Identification and Typing of Listeria Species by Matrix-Assisted Laser Desorption Ionization-Time of Flight Mass Spectrometry, Appl. Environ. Microbiol., 2008, 74, 5402–5407, DOI:10.1128/AEM.02689-07.
- E. Bessède, O. Solecki, E. Sifré, L. Labadi and F. Mégraud, Identification of Campylobacter Species and Related Organisms by Matrix Assisted Laser Desorption Ionization–Time of Flight (MALDI-TOF) Mass Spectrometry, Clin. Microbiol. Infect., 2011, 17, 1735–1739, DOI:10.1111/j.1469-0691.2011.03468.x.
- R. Dieckmann and B. Malorny, Rapid Screening of Epidemiologically Important Salmonella Enterica Subsp. Enterica Serovars by Whole-Cell Matrix-Assisted Laser Desorption Ionization–Time of Flight Mass Spectrometry, Appl. Environ. Microbiol., 2011, 77, 4136–4146, DOI:10.1128/AEM.02418-10.
- R. Dieckmann, E. Strauch and T. Alter, Rapid Identification and Characterization of Vibrio Species Using Whole-cell MALDI-TOF Mass Spectrometry, J. Appl. Microbiol., 2010, 109, 199–211, DOI:10.1111/j.1365-2672.2009.04647.x.
- T. H. Hazen, R. J. Martinez, Y. Chen, P. C. Lafon, N. M. Garrett, M. B. Parsons, C. A. Bopp, M. C. Sullards and P. A. Sobecky, Rapid Identification of Vibrio Parahaemolyticus by Whole-Cell Matrix-Assisted Laser Desorption Ionization-Time of Flight Mass Spectrometry, Appl. Environ. Microbiol., 2009, 75, 6745–6756, DOI:10.1128/AEM.01171-09.
- R. E. Mandrell, L. A. Harden, A. Bates, W. G. Miller, W. F. Haddon and C. K. Fagerquist, Speciation of Campylobacter Coli, C. Jejuni , C. Helveticus , C. Lari , C. Sputorum , and C. Upsaliensis by Matrix-Assisted Laser Desorption Ionization-Time of Flight Mass Spectrometry, Appl. Environ. Microbiol., 2005, 71, 6292–6307, DOI:10.1128/AEM.71.10.6292-6307.2005.
- K. Sparbier, U. Weller, C. Boogen and M. Kostrzewa, Rapid Detection of Salmonella Sp. by Means of a Combination of Selective Enrichment Broth and MALDI-TOF MS, Eur. J. Clin. Microbiol. Infect. Dis., 2012, 31, 767–773, DOI:10.1007/s10096-011-1373-0.
- R. Stephan, D. Ziegler, V. Pflüger, G. Vogel and A. Lehner, Rapid Genus- and Species-Specific Identification of Cronobacter Spp. by Matrix-Assisted Laser Desorption Ionization-Time of Flight Mass Spectrometry, J. Clin. Microbiol., 2010, 48, 2846–2851, DOI:10.1128/JCM.00156-10.
- S. Chun, J. Gopal and M. Muthu, A Consolidative Synopsis of the MALDI-TOF MS Accomplishments for the Rapid Diagnosis of Microbial Plant Disease Pathogens, TrAC, Trends Anal. Chem., 2022, 156, 116713, DOI:10.1016/j.trac.2022.116713.
- J. R. Yates, Mass Spectrometry and the Age of the Proteome, J. Mass Spectrom., 1998, 33, 1–19, DOI:10.1002/(SICI)1096-9888(199801)33:1<1::AID-JMS624>3.0.CO;2-9.
- D. J. Harvey, Matrix-Assisted Laser Desorption/Ionization Mass Spectrometry of Carbohydrates and Glycoconjugates, Int. J. Mass Spectrom., 2003, 226, 1–35, DOI:10.1016/S1387-3806(02)00968-5.
- E. De Carolis, A. Vella, L. Vaccaro, R. Torelli, T. Spanu, B. Fiori, B. Posteraro and M. Sanguinetti, Application of MALDI-TOF Mass Spectrometry in Clinical Diagnostic Microbiology, J. Infect. Dev. Countries, 2014, 8, 1081–1088, DOI:10.3855/jidc.3623.
- C. Benagli, A. Demarta, A. Caminada, D. Ziegler, O. Petrini and M. Tonolla, A Rapid MALDI-TOF MS Identification Database at Genospecies Level for Clinical and Environmental Aeromonas Strains, PLoS One, 2012, 7, e48441, DOI:10.1371/journal.pone.0048441.
- N. T. Popović, S. P. Kazazić, I. Strunjak-Perović and R. Čož-Rakovac, Differentiation of Environmental Aquatic Bacterial Isolates by MALDI-TOF MS, Environ. Res., 2017, 152, 7–16, DOI:10.1016/j.envres.2016.09.020.
- T. R. Sandrin, J. E. Goldstein and S. Schumaker, MALDI TOF MS Profiling of Bacteria at the Strain Level: A Review, Mass Spectrom. Rev., 2013, 32, 188–217, DOI:10.1002/mas.21359.
- J. Wang, H. Wang, K. Cai, P. Yu, Y. Liu, G. Zhao, R. Chen, R. Xu and M. Yu, Evaluation of Three Sample Preparation Methods for the Identification of Clinical Strains by Using Two MALDI-TOF MS Systems, J. Mass Spectrom., 2021, 56(2), e4696, DOI:10.1002/jms.4696.
- P. Rahi, O. Prakash and Y. S. Shouche, Matrix-Assisted Laser Desorption/Ionization Time-of-Flight Mass-Spectrometry (MALDI-TOF MS) Based Microbial Identifications: Challenges and Scopes for Microbial Ecologists, Front. Microbiol., 2016, 7, 1359, DOI:10.3389/fmicb.2016.01359.
- Y. Li, T. Wang and J. Wu, Capture and Detection of Urine Bacteria Using a Microchannel Silicon Nanowire Microfluidic Chip Coupled with MALDI-TOF MS, Analyst, 2021, 146, 1151–1156, 10.1039/D0AN02222E.
- W. Cheng, H. Shi, M. Teng, M. Yu, B. Feng, C. Ding, S. Yu and F. Yang, Rapid Identification of Bacterial Mixtures in Urine Using MALDI-TOF MS-Based Algorithm Profiling Coupled with Magnetic Enrichment, Analyst, 2022, 147, 443–449, 10.1039/D1AN02098F.
- L. V. da Costa, R. V. d. S. L. de Miranda, C. M. F. dos Reis, J. M. de Andrade, F. V. Cruz, A. M. Frazão, E. L. da Fonseca, J. N. Ramos, M. L. L. Brandão and V. V. Vieira, MALDI-TOF MS Database Expansion for Identification of Bacillus and Related Genera Isolated from a Pharmaceutical Facility, J. Microbiol. Methods, 2022, 203, 106625, DOI:10.1016/j.mimet.2022.106625.
- P. O. Barth, E. W. Roesch, L. Lutz, Â. C. de Souza, L. Z. Goldani and D. C. Pereira, Rapid Bacterial Identification by MALDI-TOF MS Directly from Blood Cultures and Rapid Susceptibility Testing: A Simple Approach to Reduce the Turnaround Time of Blood Cultures, Braz. J. Infect. Dis., 2023, 27, 102721, DOI:10.1016/j.bjid.2022.102721.
- H. Liu, Z. Du, J. Wang and R. Yang, Universal Sample Preparation Method for Characterization of Bacteria by Matrix-Assisted Laser Desorption Ionization-Time of Flight Mass Spectrometry, Appl. Environ. Microbiol., 2007, 73, 1899–1907, DOI:10.1128/AEM.02391-06.
- A. Freiwald and S. Sauer, Phylogenetic Classification and Identification of Bacteria by Mass Spectrometry, Nat. Protoc., 2009, 4, 732–742, DOI:10.1038/nprot.2009.37.
- N. Matsuda, M. Matsuda, S. Notake, H. Yokokawa, Y. Kawamura, K. Hiramatsu and K. Kikuchi, Evaluation of a Simple Protein Extraction Method for Species Identification of Clinically Relevant Staphylococci by Matrix-Assisted Laser Desorption Ionization–Time of Flight Mass Spectrometry, J. Clin. Microbiol., 2012, 50, 3862–3866, DOI:10.1128/JCM.01512-12.
- A. A. Alatoom, S. A. Cunningham, S. M. Ihde, J. Mandrekar and R. Patel, Comparison of Direct Colony Method versus Extraction Method for Identification of Gram-Positive Cocci by Use of Bruker Biotyper Matrix-Assisted Laser Desorption Ionization–Time of Flight Mass Spectrometry, J. Clin. Microbiol., 2011, 49, 2868–2873, DOI:10.1128/JCM.00506-11.
- C. Cassagne, S. Ranque, A.-C. Normand, P. Fourquet, S. Thiebault, C. Planard, M. Hendrickx and R. Piarroux, Mould Routine Identification in the Clinical Laboratory by Matrix-Assisted Laser Desorption Ionization Time-Of-Flight Mass Spectrometry, PLoS One, 2011, 6, e28425, DOI:10.1371/journal.pone.0028425.
- M. Manikandan, P.-Y. Hua and H.-F. Wu, Rapid Endophytic Bacterial Detection by Enzyme Incorporated MALDI MS, RSC Adv., 2014, 4, 50233–50240, 10.1039/C4RA05604C.
- M. Welker, Proteomics for Routine Identification of Microorganisms, Proteomics, 2011, 11, 3143–3153, DOI:10.1002/pmic.201100049.
- J. Rychert, C.-A. D. Burnham, M. Bythrow, O. B. Garner, C. C. Ginocchio, R. Jennemann, M. A. Lewinski, R. Manji, A. B. Mochon and G. W. Procop, et al., Multicenter Evaluation of the Vitek MS Matrix-Assisted Laser Desorption Ionization–Time of Flight Mass Spectrometry System for Identification of Gram-Positive Aerobic Bacteria, J. Clin. Microbiol., 2013, 51, 2225–2231, DOI:10.1128/JCM.00682-13.
- S. Sauer, A. Freiwald, T. Maier, M. Kube, R. Reinhardt, M. Kostrzewa and K. Geider, Classification and Identification of Bacteria by Mass Spectrometry and Computational Analysis, PLoS One, 2008, 3, e2843, DOI:10.1371/journal.pone.0002843.
- S. Emonet, H. N. Shah, A. Cherkaoui and J. Schrenzel, Application and Use of Various Mass Spectrometry Methods in Clinical Microbiology, Clin. Microbiol. Infect., 2010, 16, 1604–1613, DOI:10.1111/j.1469-0691.2010.03368.x.
- M. Y. Ashfaq, D. A. Da’na and M. A. Al-Ghouti, Application of MALDI-TOF MS for Identification of Environmental Bacteria: A Review, J. Environ. Manage., 2022, 305, 114359, DOI:10.1016/j.jenvman.2021.114359.
- B. Schulthess, R. Ledermann, F. Mouttet, A. Zbinden, G. V. Bloemberg, E. C. Böttger and M. Hombach, Use of the Bruker MALDI Biotyper for Identification of Molds in the Clinical Mycology Laboratory, J. Clin. Microbiol., 2014, 52, 2797–2803, DOI:10.1128/JCM.00049-14.
- S.-S. Han, Y.-S. Jeong and S.-K. Choi, Current Scenario and Challenges in the Direct Identification of Microorganisms Using MALDI TOF MS, Microorganisms, 2021, 9, 1917, DOI:10.3390/microorganisms9091917.
- L. Ferreira, F. Sánchez-Juanes, M. González-Ávila, D. Cembrero-Fuciños, A. Herrero-Hernández, J. M. González-Buitrago and J. L. Muñoz-Bellido, Direct Identification of Urinary Tract Pathogens from Urine Samples by Matrix-Assisted Laser Desorption Ionization-Time of Flight Mass Spectrometry, J. Clin. Microbiol., 2010, 48, 2110–2115, DOI:10.1128/JCM.02215-09.
- H. L. Köhling, A. Bittner, K.-D. Müller, J. Buer, M. Becker, H. Rübben, A. W. Rettenmeier and F. Mosel, Direct Identification of Bacteria in Urine Samples by Matrix-Assisted Laser Desorption/Ionization Time-of-Flight Mass Spectrometry and Relevance of Defensins as Interfering Factors, J. Med. Microbiol., 2012, 61, 339–344, DOI:10.1099/jmm.0.032284-0.
- A. Burillo, B. Rodríguez-Sánchez, A. Ramiro, E. Cercenado, M. Rodríguez-Créixems and E. Bouza, Gram-Stain Plus MALDI-TOF MS (Matrix-Assisted Laser Desorption Ionization-Time of Flight Mass Spectrometry) for a Rapid Diagnosis of Urinary Tract Infection, PLoS One, 2014, 9, e86915, DOI:10.1371/journal.pone.0086915.
- G. A. March Rosselló, M. P. Gutiérrez Rodríguez, R. Ortiz de Lejarazu Leonardo, A. Orduña Domingo and M. Á. Bratos Pérez, New Procedure for Rapid Identification of Microorganisms Causing Urinary Tract Infection from Urine Samples by Mass Spectrometry (MALDI-TOF), Enferm. Infecc. Microbiol. Clin., 2015, 33, 89–94, DOI:10.1016/j.eimc.2014.02.022.
- M. L. DeMarco and C.-A. D. Burnham, Diafiltration MALDI-TOF Mass Spectrometry Method for Culture-Independent Detection and Identification of Pathogens Directly From Urine Specimens, Am. J. Clin. Pathol., 2014, 141, 204–212, DOI:10.1309/AJCPQYW3B6JLKILC.
- E. Svetličić, L. Dončević, L. Ozdanovac, A. Janeš, T. Tustonić, A. Štajduhar, A. L. Brkić, M. Čeprnja and M. Cindrić, Direct Identification of Urinary Tract Pathogens by MALDI-TOF/TOF Analysis and De Novo Peptide Sequencing, Molecules, 2022, 27, 5461, DOI:10.3390/molecules27175461.
- C. Franco-Paredes, Bloodstream Infections, in Core Concepts in Clinical Infectious Diseases (CCCID), Elsevier, 2016, pp. 9–15 Search PubMed.
- B. La Scola and D. Raoult, Direct Identification of Bacteria in Positive Blood Culture Bottles by Matrix-Assisted Laser Desorption Ionisation Time-of-Flight Mass Spectrometry, PLoS One, 2009, 4, e8041, DOI:10.1371/journal.pone.0008041.
- A. G. W. Foster, Rapid Identification of Microbes in Positive Blood Cultures by Use of the Vitek MS Matrix-Assisted Laser Desorption Ionization–Time of Flight Mass Spectrometry System, J. Clin. Microbiol., 2013, 51, 3717–3719, DOI:10.1128/JCM.01679-13.
- J. D. Haigh, I. M. Green, D. Ball, M. Eydmann, M. Millar and M. Wilks, Rapid Identification of Bacteria from BioMerieux BacT/ALERT Blood Culture Bottles by MALDI-TOF MS, Br. J. Biomed. Sci., 2013, 70, 149–155, DOI:10.1080/09674845.2013.11669949.
- M. Tadros and A. Petrich, Evaluation of MALDI-TOF Mass Spectrometry and Sepsityper Kit™ for the Direct Identification of Organisms from Sterile Body Fluids in a Canadian Pediatric Hospital, Can. J. Infect. Dis. Med. Microbiol., 2013, 24, 191–194, DOI:10.1155/2013/701093.
- E. A. Idelevich, I. Schüle, B. Grünastel, J. Wüllenweber, G. Peters and K. Becker, Rapid Identification of Microorganisms from Positive Blood Cultures by MALDI-TOF Mass Spectrometry Subsequent to Very Short-Term Incubation on Solid Medium, Clin. Microbiol. Infect., 2014, 20, 1001–1006, DOI:10.1111/1469-0691.12640.
- R. Kohlmann, A. Hoffmann, G. Geis and S. Gatermann, MALDI-TOF Mass Spectrometry Following Short Incubation on a Solid Medium Is a Valuable Tool for Rapid Pathogen Identification from Positive Blood Cultures, Int. J. Med. Microbiol., 2015, 305, 469–479, DOI:10.1016/j.ijmm.2015.04.004.
- N. J. Froböse, E. A. Idelevich and F. Schaumburg, Short Incubation of Positive Blood Cultures on Solid Media for Species Identification by MALDI-TOF MS: Which Agar Is the Fastest?, Microbiol. Spectrum, 2021, 9(1), e0003821, DOI:10.1128/Spectrum.00038-21.
- I. Neonakis and D. Spandidos, Rapid Identification of Gram-negative Pathogens from Positive Blood Cultures Using MALDI-TOF MS Following Short-term Incubation on Solid Media, Biomed. Rep., 2022, 16, 47, DOI:10.3892/br.2022.1530.
- J. A. Branda, R. P. Markham, C. D. Garner, J. A. Rychert and M. J. Ferraro, Performance of the Vitek MS v2.0 System in Distinguishing Streptococcus Pneumoniae from Nonpneumococcal Species of the Streptococcus Mitis Group, J. Clin. Microbiol., 2013, 51, 3079–3082, DOI:10.1128/JCM.00824-13.
- C. W. Svarrer and S. A. Uldum, The Occurrence of Legionella Species Other than Legionella Pneumophila in Clinical and Environmental Samples in Denmark Identified by Mip Gene Sequencing and Matrix-Assisted Laser Desorption Ionization Time-of-Flight Mass Spectrometry, Clin. Microbiol. Infect., 2012, 18, 1004–1009, DOI:10.1111/j.1469-0691.2011.03698.x.
- I. D. Nix, E. A. Idelevich, A. Schlattmann, K. Sparbier, M. Kostrzewa and K. Becker, MALDI-TOF Mass Spectrometry-Based Optochin Susceptibility Testing for Differentiation of Streptococcus Pneumoniae from Other Streptococcus Mitis Group Streptococci, Microorganisms, 2021, 9, 2010, DOI:10.3390/microorganisms9102010.
- C. Yo, Y. Shen, W. Hsu, R. A. Mekary, Z. R. Chen, W. J. Lee, S. Chen and C. Lee, MALDI-TOF Mass Spectrometry Rapid Pathogen Identification and Outcomes of Patients with Bloodstream Infection: A Systematic Review and Meta-analysis, Microb. Biotechnol., 2022, 15, 2667–2682, DOI:10.1111/1751-7915.14124.
- B. Bishop, Y. Geffen, A. Plaut, O. Kassis, R. Bitterman, M. Paul and A. Neuberger, The Use of Matrix-Assisted Laser Desorption/Ionization Time-of-Flight Mass Spectrometry for Rapid Bacterial Identification in Patients with Smear-Positive Bacterial Meningitis, Clin. Microbiol. Infect., 2018, 24, 171–174, DOI:10.1016/j.cmi.2017.05.014.
- S. Segawa, S. Sawai, S. Murata, M. Nishimura, M. Beppu, K. Sogawa, M. Watanabe, M. Satoh, T. Matsutani and M. Kobayashi, et al., Direct Application of MALDI-TOF Mass Spectrometry to Cerebrospinal Fluid for Rapid Pathogen Identification in a Patient with Bacterial Meningitis, Clin. Chim. Acta, 2014, 435, 59–61, DOI:10.1016/j.cca.2014.04.024.
- Y. Li, M. Shan, Z. Zhu, X. Mao, M. Yan, Y. Chen, Q. Zhu, H. Li and B. Gu, Application of MALDI-TOF MS to Rapid Identification of Anaerobic Bacteria, BMC Infect. Dis., 2019, 19, 941, DOI:10.1186/s12879-019-4584-0.
- M. I. Stets, A. S. Pinto, L. F. Huergo, E. M. de Souza, V. F. Guimarães, A. C. Alves, M. B. R. Steffens, R. A. Monteiro, F. d. O. Pedrosa and L. M. Cruz, Rapid Identification of Bacterial Isolates from Wheat Roots by High Resolution Whole Cell MALDI-TOF MS Analysis, J. Biotechnol., 2013, 165, 167–174, DOI:10.1016/j.jbiotec.2013.04.001.
- R. Alm, P. Johansson, K. Hjernø, C. Emanuelsson, M. Ringnér and J. Häkkinen, Detection and Identification of Protein Isoforms Using Cluster Analysis of MALDI−MS Mass Spectra, J. Proteome Res., 2006, 5, 785–792, DOI:10.1021/pr050354v.
- A. Tani, N. Sahin, Y. Matsuyama, T. Enomoto, N. Nishimura, A. Yokota and K. Kimbara, High-Throughput Identification and Screening of Novel Methylobacterium Species Using Whole-Cell MALDI-TOF/MS Analysis, PLoS One, 2012, 7, e40784, DOI:10.1371/journal.pone.0040784.
- M. Sura-de Jong, R. J. B. Reynolds, K. Richterova, L. Musilova, L. C. Staicu, I. Chocholata, J. J. Cappa, S. Taghavi, D. van der Lelie and T. Frantik, et al., Selenium Hyperaccumulators Harbor a Diverse Endophytic Bacterial Community Characterized by High Selenium Resistance and Plant Growth Promoting Properties, Front. Plant Sci., 2015, 6, 113, DOI:10.3389/fpls.2015.00113.
- P. S. P. Costa Júnior, F. P. Cardoso, A. D. Martins, V. H. Teixeira Buttrós, M. Pasqual, D. R. Dias, R. F. Schwan and J. Dória, Endophytic Bacteria of Garlic Roots Promote Growth of Micropropagated Meristems, Microbiol. Res., 2020, 241, 126585, DOI:10.1016/j.micres.2020.126585.
- S. Toubal, O. Bouchenak, D. Elhaddad, K. Yahiaoui, S. Boumaza and K. Arab, MALDI-TOF MS Detection of Endophytic Bacteria Associated with Great Nettle (Urtica Dioica L.), Grown in Algeria, Pol. J. Microbiol., 2018, 67, 67–72, DOI:10.5604/01.3001.0011.6145.
- P. Martínez-Hidalgo, J. D. Flores-Félix, F. Sánchez-Juanes, R. Rivas, P. F. Mateos, I. Santa Regina, Á. Peix, E. Martínez-Molina, J. M. Igual and E. Velázquez, Identification of Canola Roots Endophytic Bacteria and Analysis of Their Potential as Biofertilizers for Canola Crops with Special Emphasis on Sporulating Bacteria, Agronomy, 2021, 11, 1796, DOI:10.3390/agronomy11091796.
- D. Ziegler, A. Mariotti, V. Pflüger, M. Saad, G. Vogel, M. Tonolla and X. Perret, In Situ Identification of Plant-Invasive Bacteria with MALDI-TOF Mass Spectrometry, PLoS One, 2012, 7, e37189, DOI:10.1371/journal.pone.0037189.
- M. Barthélemy, V. Guérineau, G. Genta-Jouve, M. Roy, J. Chave, R. Guillot, L. Pellissier, J.-L. Wolfender, D. Stien and V. Eparvier, et al., Identification and Dereplication of Endophytic Colletotrichum Strains by MALDI TOF Mass Spectrometry and Molecular Networking, Sci. Rep., 2020, 10, 19788, DOI:10.1038/s41598-020-74852-w.
- A. Wensing, S. Zimmermann and K. Geider, Identification of the Corn Pathogen Pantoea Stewartii by Mass Spectrometry of Whole-Cell Extracts and Its Detection with Novel PCR Primers, Appl. Environ. Microbiol., 2010, 76, 6248–6256, DOI:10.1128/AEM.01032-10.
- J. Zaluga, K. Heylen, K. Van Hoorde, B. Hoste, J. Van Vaerenbergh, M. Maes and P. De Vos, GyrB Sequence Analysis and MALDI-TOF MS as Identification Tools for Plant Pathogenic Clavibacter, Syst. Appl. Microbiol., 2011, 34, 400–407, DOI:10.1016/j.syapm.2011.05.001.
- Y. Wang, Q. Zhou, B. Li, B. Liu, G. Wu, M. Ibrahim, G. Xie, H. Li and G. Sun, Differentiation in MALDI-TOF MS and FTIR Spectra between Two Closely Related Species Acidovorax Oryzae and Acidovorax Citrulli, BMC Microbiol., 2012, 12, 182, DOI:10.1186/1471-2180-12-182.
- B. Niu, J. Vater, C. Rueckert, J. Blom, M. Lehmann, J.-J. Ru, X.-H. Chen, Q. Wang and R. Borriss, Polymyxin P Is the Active Principle in Suppressing Phytopathogenic Erwinia Spp. by the Biocontrol Rhizobacterium Paenibacillus Polymyxa M-1, BMC Microbiol., 2013, 13, 137, DOI:10.1186/1471-2180-13-137.
- G. M. Macedo-Raygoza, B. Valdez-Salas, F. M. Prado, K. R. Prieto, L. F. Yamaguchi, M. J. Kato, B. B. Canto-Canché, M. Carrillo-Beltrán, P. Di Mascio and J. F. White, et al., Enterobacter Cloacae, an Endophyte That Establishes a Nutrient-Transfer Symbiosis With Banana Plants and Protects Against the Black Sigatoka Pathogen, Front. Microbiol., 2019, 10, 804, DOI:10.3389/fmicb.2019.00804.
- C. B. Sruthilaxmi and S. Babu, Proteome Responses to Individual Pathogens and Abiotic Conditions in Rice Seedlings, Phytopathology, 2020, 110, 1326–1341, DOI:10.1094/PHYTO-11-19-0425-R.
- J. Šalplachta, A. Kubesová, J. Horký, H. Matoušková, M. Tesařová and M. Horká, Characterization of Dickeya and Pectobacterium Species by Capillary Electrophoretic Techniques and MALDI-TOF MS, Anal. Bioanal. Chem., 2015, 407, 7625–7635, DOI:10.1007/s00216-015-8920-y.
- H. Kajiwara, Direct Detection of the Plant Pathogens Burkholderia Glumae, Burkholderia Gladioli Pv. Gladioli, and Erwinia Chrysanthemi Pv. Zeae in Infected Rice Seedlings Using Matrix Assisted Laser Desorption/Ionization Time-of-Flight Mass Spectrometry, J. Microbiol. Methods, 2016, 120, 1–5, DOI:10.1016/j.mimet.2015.08.014.
- I. Fonseca-Guerra, C. Chiquillo, M. J. Padilla and M. Benavides-Rozo, First Report of Bacterial Leaf Spot on Chenopodium Quinoa Caused by Pseudomonas Syringae in Colombia, J. Plant Dis. Prot., 2021, 128, 871–874, DOI:10.1007/s41348-021-00435-0.
- T. Oberhaensli, V. Hofer, L. Tamm, J. G. Fuchs, M. Koller, J. Herforth-Rahmé, M. Maurhofer and B. Thuerig, Aeromonas Media in Compost Amendments Contributes to Suppression of Pythium Ultimum in Cress, Acta Hortic., 2017, 353–360, DOI:10.17660/ActaHortic.2017.1164.45.
- O. Choi, B. Kang, S. K. Cho, J. Park, Y. Lee, W.-I. Kim, J. Marunga, I. Hwang and J. Kim, Identification of Pseudomonas Syringae Pv. Syringae Causing Bacterial Leaf Blight of Miscanthus Sinensis, J. Plant Dis. Prot., 2017, 124, 97–100, DOI:10.1007/s41348-016-0058-4.
- H. Sawada, H. Horita, T. Misawa and Y. Takikawa, Pseudomonas Grimontii, Causal Agent of Turnip Bacterial Rot Disease in Japan, J. Gen. Plant Pathol., 2019, 85, 413–423, DOI:10.1007/s10327-019-00869-3.
- M. Božik, M. Mrázková, K. Novotná, M. Hrabětová, P. Maršik, P. Klouček and K. Černý, MALDI-TOF MS as a Method for Rapid Identification of Phytophthora de Bary, 1876, PeerJ, 2021, 9, e11662, DOI:10.7717/peerj.11662.
- E. d. S. Niculau, D. Ferreira, E. Rodrigues-Filho, F. Behlau, R. F. Magnani, L. Toffano, E. L. Prieto, J. B. Fernandes and M. F. d. G. F. da Silva, MALDI-TOF Mass Spectrometry for the Diagnosis of Citrus Canker Caused by Xanthomonas Citri Subsp. Citri, Molecules, 2022, 27, 8947, DOI:10.3390/molecules27248947.
- P. Chowdappa, M. J. Lakshmi and S. Madhura, Matrix Assisted Laser Desorption Ionization–Time of Flight (MALDI-TOF) Mass Spectrometry for Identification of Plant Pathogenic Alternaria Species, Phytoparasitica, 2013, 41, 169–179, DOI:10.1007/s12600-012-0276-7.
- P. Galeano Garcia, F. Neves dos Santos, S. Zanotta, M. Eberlin and C. Carazzone, Metabolomics of Solanum Lycopersicum Infected with Phytophthora Infestans Leads to Early Detection of Late Blight in Asymptomatic Plants, Molecules, 2018, 23, 3330, DOI:10.3390/molecules23123330.
- M. K. Forster, S. Sedaghatjoo, W. Maier, B. Killermann and L. Niessen, Discrimination of Tilletia Controversa from the T. Caries/T. Laevis Complex by MALDI-TOF MS Analysis of Teliospores, Appl. Microbiol. Biotechnol., 2022, 106, 1257–1278, DOI:10.1007/s00253-021-11757-2.
- A. Wensing, M. Gernold and K. Geider, Detection of Erwinia Species from the Apple and Pear Flora by Mass Spectroscopy of Whole Cells and with Novel PCR Primers, J. Appl. Microbiol., 2012, 112, 147–158, DOI:10.1111/j.1365-2672.2011.05165.x.
|
This journal is © The Royal Society of Chemistry 2023 |