Elucidating the physical, morphometric, nutritional, and bioactive properties of selected highland crops viz. hull-less barley, buckwheat, and black rice for novel food formulation
Received
22nd February 2023
, Accepted 30th May 2023
First published on 14th June 2023
Abstract
Highland crops have drawn increased interest as functional foods due to the abundance of bioactive chemicals with health advantages, including antioxidant and immunomodulatory activity. Therefore, the present study aimed to investigate the selected crops of the highland, viz. hull-less barley, buckwheat and black rice, for their nutritional-functional, microstructural and immunomodulatory properties. All the selected crops possess the required protein content, which was found to be the highest in buckwheat (12.33%). Total phenolic content was found to be the highest in buckwheat (319.30 mg GAE per g), followed by hull-less barley (44.80 mg GAE per g) and black rice (77.30 mg GAE per g). The results demonstrate the potential immunomodulatory activity of all selected crops. These crops also consist of essential minerals such as iron. The results reinforce the promotion of these underutilized food grains as they are nutritionally rich, and are natural sources of antioxidants and minerals, and have potential immunomodulatory activity, which could be used in the food and pharmaceutical industries.
Sustainability spotlight
The present study highlights the nutritional-functional, microstructural, and immunomodulatory properties of selected crops of highland viz. hull-less barley, buckwheat, and black rice. These valuable underutilised grains lost their identity due to urbanisation but contain ample amount of nutrients, which is explored in this study as a potential for developing a variety of food products. Additionally, the triple burden of malnutrition, undernourishment, micronutrient deficiency, and overnutrition is affecting many social strata in both developed and developing nations. According to research, underutilised food crops might ameliorate malnutrition and “hidden hunger,” and lessen food insecurity. These targeted UN goals under SDG2 end hunger, achieve food security and improved nutrition and promote sustainable agriculture and under SDG3 ensure healthy lives and promote well-being for all at all ages.
|
Introduction
Mankind has successfully exposed nature to a greater extent through relentless efforts. India's diverse climate supports a variety of green foods, some of which are currently neglected and whose knowledge is restricted to certain tribal and ethnic tribes. Many of these are valuable as sources of food for people and meeting the needs on earth. These foods are renowned for being rich in vitamins and minerals.1 They have the potential to feed the expanding human population. Many of these plant species are hardy, adaptable, and tolerant of unfavourable climatic circumstances; however, they can still be grown in poor marginal areas at less cost. Still, due to a lack of awareness and effective methods of popularisation, they continue to be neglected. Underutilized foods are becoming more important today as a way to boost the per capita availability of food and improve health. Highland barley is a cereal crop with good cold and drought resistance, and is widely grown in high-altitude (4200–4500 m above sea level) areas.2 It is a very significant cereal crop grown in cold and high-altitude regions with traits such as resilience to cold, short growth periods, high yields, and early maturation. As compared to other crops, highland barley has a good concentration of dietary fibers, such as β-glucan and arabinoxylan. Highland barley is frequently utilised in the brewing industry, especially the food and functional food industries, and other sectors.
Nowadays, buckwheat is becoming more popular as a healthy crop. Compared to other grains such as oats, wheat, and rye, it contains a higher overall content of phenols and has better antioxidant capabilities. Indeed, buckwheat is gluten-free and can be used to make gluten-free food products, which are suitable for celiac patients.3
Black rice is a precious rice variety, and significant research in this area is available on coloured rice because it has more protein, dietary fibre, minerals, and vitamins, including more anthocyanins and trace minerals, as compared to normal rice. Moreover, black rice serves a variety of therapeutic and medical purposes, including protection against cancer, diabetes, and gastrointestinal disorders, and lowering oxidative stress to combat cardiovascular disease.4
In India, the prevalence of chronic diseases has peaked. There are different metabolic diseases, including respiratory ailments, which affect one in every five people nationwide. The primary contributing causes of this are growing urbanisation, evolving lifestyles, and unhealthy eating patterns. Additionally, India is burdened by both undernutrition and overnutrition. There is an increasing tendency toward consuming meals that would enhance health and well-being. People now evaluate food based on its ability to enhance general health as well as its taste and nutritional requirements. Hence, underutilized foods are becoming more important today as a way to boost the per capita food supply and health status. Additionally, the triple burden of malnutrition, undernourishment, micronutrient deficiency, and overnutrition is affecting many social strata in both developed and developing nations. According to research, underutilised food crops might ameliorate malnutrition and “hidden hunger,” and lessen food insecurity.5 Thus, we can consume various natural underutilised crops that have been processed into products. For flavour molecules, nutrients, and bioactive ingredients to escape the restricting food matrices and perform their functions, these structures need to degrade and dissolve inside our bodies. Hence, the function of the microstructure and the food matrix in food design and specialised product applications are important.6 There is limited information about the nutritional profiles and health benefits of these crops. Hence, keeping in view this whole scenario, the objective of this study was to determine the physical, morphometric, nutritional, and bioactive properties of selected highland crops.
Materials and methods
Grain collection
Hull-less barley and buckwheat grains were collected from Kaza, Lahual Spiti, Himachal Pradesh, whereas black rice was collected from Manipur, India. Grains were cleaned properly manually and then under running tap water to remove any unwanted dust particles. Cleaned grains were dried in a hot air oven (Oven Universal, hot air oven, NSW India) at 40 °C for 24 hours. One portion of the grains was kept aside for physical analysis and the rest were ground to flour in a cutting miller (Retsch SM 100) and then passed through a sieve (mesh 60 mm). The obtained flour was then kept in air-tight jars for further analysis.
Reagents and chemicals
The reagents and chemicals used for the study were of analytical and HPLC grade. Sodium carbonate, sodium hydroxide, folin-ciocalteu reagent, aluminium chloride, potassium persulphate, copper sulphate and other chemicals were procured from Hi-media Laboratories Private Limited, India. All standards were provided by Sigma, India.
Extraction
The flour of selected grains (10 g) was extracted with 70% ethanol (100 mL) for each sample, separately, and then filtered. The filtrate was then dried using a rotary-evaporator, followed by lyophilisation. The extract obtained was stored at −80 °C. These extracts were further used for SDS-PAGE and bioactive analysis.
The defatted flour of each grain was steeped in 0.02 mol L−1 phosphate buffer, pH 6.5 (1
:
100, g
:
mL), and then homogenised for an hour at room temperature. The solution was then centrifuged at 13
000g for 20 min at 4 °C. The supernatant was collected, passed through a 0.22 m filter (Merck Millipore, India), followed by lyophilization, and then stored at −20 °C for further free amino acid estimation.
Physical, morphometric and structure analysis
Length and width.
A vernier calliper was used to measure the physical parameters (length and width) of the selected crop grains,7 and ten consecutive readings were collected for each crop sample.
Bulk density.
According to the method explained by Soliman et al.,8 the volumetric displacement method was performed to determine the bulk density (g cm−3) of the samples.
Thousand kernel weight.
One thousand seeds of each crop were selected randomly and weights were taken in an electronic digital balance (Wensar™ IS 9001:2000).9 Ten replicates of each test's weight were conducted.
Water activity.
Samples' water activity was determined by placing them in an Aqualab 4TE water activity meter (dew point, Decagon, Inc.).10
Colour profile.
A Konica Minolta CR-400 (Chroma-Meter) colorimeter was used to assess the colour value of grains. L* value shows the brightness of the sample, a* depicts the red-green colour, whereas b* explains yellow-blue dimensions.
Scanning electron microscopy.
Scanning electron microscopy was used to determine the microstructure of dried ground samples.11 Samples were sprinkled in a carbon tab placed on an aluminium stub, followed by positioning them in an E1010 ion sputter (Hitachi, JAPAN). Samples were coated with gold to make the surface conductive, and then kept at 10 Pa vacuum for 20 s. For capturing SEM images, a Hitachi S3400N was used at 30 KV.
Fourier transform infrared spectroscopy (FT-IR) analysis.
For structural analysis, an FT-IR spectrophotometer (PerkinElmer Spectrum RX 1 [RX-1]) was used. Each powdered sample (10 mg) was determined in a spectral range noted with a resolution of 1 cm−1 from 4000 to 400 cm−1.
Thermogravimetric analysis (TGA).
TGA of the samples was performed using a thermogravimetric analyser (NETZSCH STA 449 Geratebau GmbH F1 Jupiter thermal analyser). In brief, each powdered sample (2 mg) was analysed under a nitrogen atmosphere with a thermogravimetric analyzer at a heating rate of 10 °C min−1 and a temperature range of 20–400 °C.
Nutritional analysis.
Nutritional analysis was performed using standard methods of AOAC,12 which are as follows.
Moisture.
The moisture of ground grains was determined using a hot air oven. Samples were weighed in pre-weighed moisture dishes and then kept in a hot air oven at 105 °C ± 5 for 1 h. Moisture dishes were then taken out from the hot air oven and placed in a desiccator for cooling and weight was taken thereafter. This process was repeated until a constant weight was attained. The moisture content was then calculated using the following formula: moisture (%) = loss in weight (g)/weight of sample (g) × 100.
Crude protein.
Protein content was analysed using the micro-Kjeldahl method. Samples were digested on a hot plate in a Kjeldahl flask with a two-gram digestion mixture (potassium sulphate and copper sulphate) and 10 mL of sulphuric acid (96%). The digested sample was then cooled and filtered through Whatman filter paper, followed by a volume make up to 100 mL. 5 mL of digested samples were taken into a distillation flask with 10 mL of 40% sodium hydroxide and liberated ammonium borate was trapped in a boric acid solution (4%) containing 2–3 drops of indicator (bromocresol green and methyl red). The obtained distillate was titrated with 0.1 M HCl. The nitrogen content was determined using the following formula: nitrogen (%) = titration value × M of HCl × 14 × 100/sample weight × 100, and the amount of protein using the following formula: crude protein (%) = nitrogen (%) × 6.25.
Total fat.
Total fat content was determined using the Soxhlet extraction method. Weighed samples were filled in a thimble and extracted in Soxhlet apparatus with petroleum ether for 18 h. Thereafter, the fat extract was filtered through a sintered funnel in a pre-weighed beaker. Petroleum ether was then evaporated and the beaker was weighed to obtain the fat percentage with the following formula: fat (%) = amount of ether extract/weight of sample × 100.
Total ash.
Ash content was determined using incineration in a muffle furnace. Firstly, weighed samples were charred in a pre-weighed silica crucible on a hot plate. The crucible was then placed in a muffle furnace at 550 °C ± 5 for 4 h. The crucible was then kept in a desiccator for cooling, followed by weighing. Ash content in the sample was calculated using the following formula: ash (%) = weight of ash/weight of sample × 100.
Crude fiber.
The acid-alkali digestion method was used to determine the fiber content in the sample. Fat-free samples were taken and weighed, followed by acid digestion by boiling with 200 mL of sulphuric acid (1.25%) for 30 min. The residue was then washed with hot distilled water and filtered to remove the acid. The residue was then treated with alkali digestion by boiling with 200 mL of sodium hydroxide (1.25%) for 30 min. The obtained residue was then placed in a pre-weighed silica crucible and dried overnight in a hot air oven, followed by ignition in a muffle furnace at 550 °C ± 5 for 4 h. The final weight was recorded after cooling the crucible in a desiccator and crude fiber content was calculated using the following formula: crude fiber (%) = wt of the sample (before ignition) − wt of the sample (after ignition)/weight of sample × 100.
Total carbohydrate.
The total carbohydrate content of samples was analysed using the method described by Nielsen (2017).13 Digested samples were taken in a glass test tube and distilled water was used to make the final volume up to 1 mL. Then, 5% phenol (1 mL) and sulphuric acid (5 mL) were added to the sample in the test tube and stirred well. The test tube was then placed in a water bath (25–30 °C) for 20 min and absorbance was noted in the spectrometer (Bio Spectrometer, Eppendorf) at 490 nm.
Mineral content.
To ascertain the mineral content in selected crops, atomic absorption spectroscopy (iCETM 3400 AAS, Agilent Technologies) was employed. Each powdered grain (one gram) was digested using a triacid solution (9
:
3
:
1) of nitric acid, perchloric acid, and sulfuric acid. After digestion, the filtrate was diluted with Milli-Q water. Readings were recorded in triplicate.
Free amino acid determination.
A Waters Acquity UPLC-H class system with a binary solvent management system, autosampler, photodiode array detector (PDA), 600 controller pumps with an online degasser, and column heater was used to evaluate the amino acid composition.11 A column (Waters BEH C18; particle size 2.1 100 mm, 1.7 m) combined with an appropriate guard column was used for separations. Tri-ethylamine and sodium acetate (0.14 mol L−1) were used to prepare the mobile phase A, and glacial acetic acid and methanol were used to adjust the pH to 6.7. (90
:
10). Acetonitrile was utilised as the mobile phase B. Before being injected into the system, standard mix and samples with O-phthalaldehyde were derivatized and filtered with the help of a 0.45 Millipore membrane syringe filter.
SDS-PAGE analysis.
The method described by Kumar et al.14 was used to conduct the SDS PAGE analysis of the various selected grains viz. hull-less barley, buckwheat, and black rice, with minor modification. Briefly, a mixture of 62.5 mM Tris–HCl, pH 6.8, 2.5% SDS, 10% glycerol, and 5% 2-mercaptoethanol was used to homogenise 25 mg of each grain extract before cooling. Afterwards, at room temperature, the homogenate was incubated on a shaker for an hour and then centrifuged for 15 minutes at 15
000 rpm.
A resolving gel of 10%, stacking gel of 5%, and gel with a 1 mm thickness were utilised. It was stained with Coomassie brilliant blue. Biorad molecular weight marker (USA) was used.
Techno-functional analysis
Water absorption index (WAI), oil absorption index (OAI) and water solubility index (WSI).
The sample's WAI, OAI and WSI were determined using the method described by Bhatt et al.15 with minor modifications. 1 g sample was taken in pre-weighed centrifuge tubes and mixed with 10 mL distilled water and oil, separately, vortexed and then centrifuged for 15 min at 35
000 rpm. The supernatant from the water absorption index was then collected in pre-weighed Petri-plates and the residue in centrifuge tubes was weighed to determine the WAI and OAI content using the following formula: weight of gel/sample weight.
For calculating WSI, the supernatant was collected from WAI and then dried in a hot air oven at 105 °C for 1–2 h, cooled and weighed to determine the WSI content using the following formula: (weight of the Petri plate before drying − the weight of the Petri plate after drying/weight of the sample initial) × 100.
Bioactive profile
Soluble phenolic fraction (SPF) and total flavonoid content (TFC).
SPF and TFC were assessed using the method previously described by Chlopicka et al.16 with minor modifications. Briefly, for TPC, 500 μL Folin-Ciocalteu was added to 1 mg mL−1 of sample extract followed by 400 μL sodium bicarbonate (7.5%). The mixture was incubated at room temperature for 30 min in the dark and absorbance was taken at 765 nm. The standard used was gallic acid, which was measured as gallic acid equivalent (mg GAE/100 g). For TFC, 50 mL sodium nitrite (5%) and aluminium chloride (10%) were added to 1 mg mL−1 sample extract. Then 0.5 mL potassium acetate (120 mM) was added, followed by incubation at room temperature for 30 min in the dark. Afterwards, absorbance was read at 415 nm. Rutin was used as a positive control.
Antioxidant activity.
DPPH (2,2-diphenyl-1-picrylhydrazyl) and ABTS (2,2′-azinobis (3-ethylbenzothiazoline-6-sulphonic acid)) were used to determine the antioxidant potential of the sample as previously described by Kumari et al.13 Ascorbic acid was used as a standard control in both the assays.
Phenolic profiling.
A gradient method (UHPLC-DAD, Agilent Technologies, USA) diode array detector was employed to determine the concentration of phenolic compounds as described by Kumari et al.11
Antimicrobial activity.
Staphylococcus aureus (MTCC 96), Bacillus subtilis (MTCC 121), Micrococcus luteus (MTCC 2470), Escherichia coli (MTCC 43), Klebsiella pneumoniae (MTCC 109), and Pseudomonas aeruginosa (MTCC 2453) were Gram-positive and Gram-negative opportunistic pathogen strains used to test the antimicrobial activity of selected crops as described by Baliyan et al.17 Streptomycin (10 μg) was used as a control and the zone of inhibition diameter was measured after the incubation for 24 h at 37 °C.
Cell viability.
MTT (3-(4,5-dimethylthiazol-2-yl)-2,5-diphenyltetrazolium bromide) assay was used to measure the cell viability among the selected crops as described by Doskocil et al.18
Measurement of nitric oxide (NO) production.
Griess reagent (Promega) was used to assess the impact of extract/fractions and steroidal saponins on NO production. The Raw 264.7 macrophage cells were seeded in 96-well plates and left to incubate for 24 hours. Extracts of selected crops at different concentrations (25, 50, 100 and 200 μg mL−1) were used for treatment of cells, followed by 12 h of incubation. Later, cells were treated with lipopolysaccharide (LPS = 1 mg mL−1) for 24 hours and NO production was measured at 535 nm using Griess reagent in a Synergy H1 BioTek microplate reader. The experiment was carried out in triplicate.
Measurement of cytokines by ELISA.
Two different concentrations (50 and 100 μg mL−1) of each crop extract were applied to raw macrophages (1.5 × 106) for 12 hours. According to the manufacturer's instructions, the supernatant was taken and utilised to test the cytokines tumour necrosis factor alpha (TNF-α), interleukin 4 (IL-4), interleukin 6 (IL-6) and interleukin 10 (IL-10) with the help of enzyme-linked immunosorbent assay (ELISA). The absorbance was noted at 450 nm using a Synergy H1 BioTek microplate reader.
Statistical analysis.
All the analytical experimental analysis was carried out in triplicate (n = 3) and the results were presented as mean ± standard deviation followed by multiple comparisons done by using Duncan's multiple range test. The data were plotted into graphical representations using graphing software (GraphPad Prism 8.0.2.263 and OriginPro 8.5).
Results and discussion
Physical, morphometric and structure analysis
Table 1 demonstrates the physical parameters of selected crops. The length and width of grains varied from 5.64 to 6.56 mm and 1.53 to 2.70 mm, respectively. Hull-less barley showed the highest bulk density (0.78 g cm−3), followed by black rice (0.76 g cm−3) and buckwheat (0.59 g cm−3). Thousand kernel weight was recorded, highest in hull-less barley (46.83 g) then in buckwheat (20.21 g) and black rice (10.64 g). The mean weight of the buckwheat seeds in this study was within normal limits when compared to seed weights in earlier investigations.19 Similar results for physical parameters of hull-less barley coincided with the study of Bai et al.,20 whereas the average buckwheat grain dimensions found in this investigation are less than the reported dimensions observed by Kaliniewicz et al.21
Table 1 Physical and functional properties of highland grainsa,b
Grains |
Physical |
Functional |
Length (mm) |
Width (mm) |
Bulk density (g cm−3) |
1000 kernel weight (g) |
Water activity (aw) |
WAI (g g−1) |
WSI (g g−1) |
OAI (g g−1) |
All values are means of three determinations (n = 3) ± standard deviation.
WAI: water absorption index; WSI: water solubility index; OAI: oil absorption index.
|
Hull-less barley |
6.56 ± 0.08a |
2.64 ± 0.03ab |
0.78 ± 0.04a |
46.83 ± 0.04a |
0.45 ± 0.02c |
1.73 ± 0.12a |
5.9 ± 0.08b |
1.30 ± 0.14ab |
Buckwheat |
5.64 ± 0.08c |
2.70 ± 0.02a |
0.59 ± 0.08b |
20.21 ± 0.16b |
0.55 ± 0.04a |
1.54 ± 0.16b |
4.2 ± 0.06c |
1.37 ± 0.12a |
Black rice |
6.02 ± 0.06b |
1.53 ± 0.06c |
0.76 ± 0.04a |
10.64 ± 0.04c |
0.34 ± 0.05ab |
1.46 ± 0.10bc |
8.1 ± 0.04a |
1.27 ± 0.18c |
Colour profile
Fig. 1 shows the colour profile of selected highland crops, which illustrates the visual aspects of crops. The l* value was recorded highest for hull-less barley and least for black rice. Similarly, significant differences were also seen in the a* and b* values, which, respectively, exhibit colour deviations from red to green and blue to yellow. Highland crops are mostly dark in colour because of their nutritional components such as phenolics, and flavonoids, which resulted in a lower l* (brightness) value.22 The colour characteristics of various flours varied depending on the biological origin of the plant due to the various coloured pigments.23
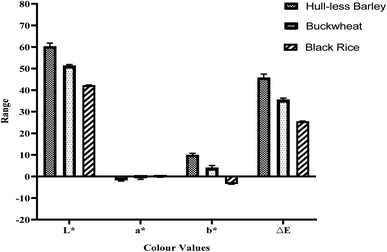 |
| Fig. 1 Colour value of highland grains. | |
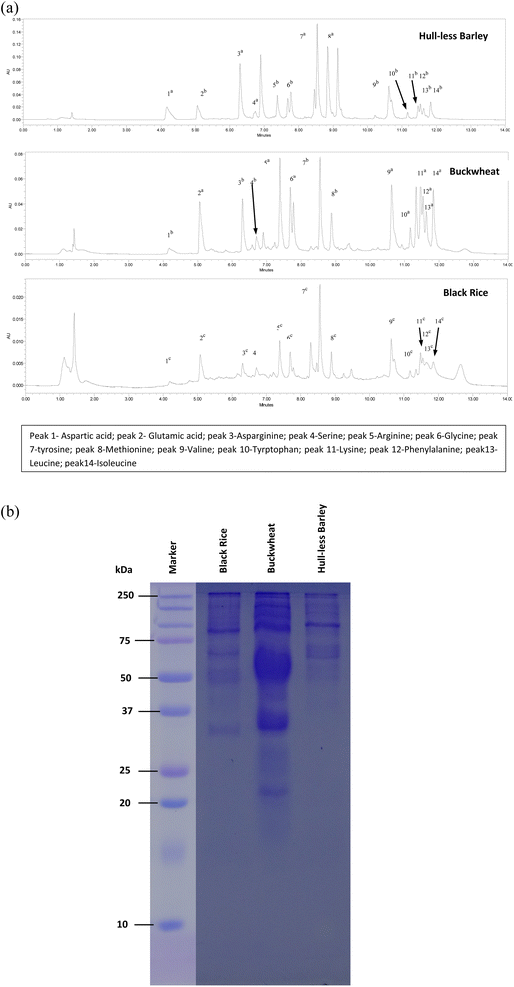 |
| Fig. 2 (a) Free amino acid profile of highland grains peak 1 – aspartic acid; peak 2 – glutamic acid; peak 3 – asparginine; peak 4 – serine; peak 5 – arginine; peak 6 – glycine; peak 7 – tyrosine; peak 8-methionine; peak 9 – valine; peak 10 – tyrptophan; peak 11 – lysine; peak 12 – phenylalanine; peak13 – leucine; peak 14 – isoleucine. (b) SDS-PAGE of highland grains. | |
Scanning electron microscopy
The microscopic morphology of selected crops was evaluated by SEM as shown in Fig. 3(a). In hull-less barley, higher concentrations of protein and tiny starch granules are related to steeliness. In Fig. 3(a) endosperm with firmly and loosely packed starches was observed in hull-less barley. It should be noted that each kernel might have a different method of starch packing. Additionally, within the kernel itself, the density of the protein in which the starch is contained might vary depending on the specific area. In buckwheat, starch granules were placed in large ridged cells, enclosed by comparatively thin cell walls. The observation of SEM in barley and buckwheat was also witnessed in a study conducted by Wijngaard et al.24 Like other rice grains, black rice granules are polyhedral and have an uneven form.25
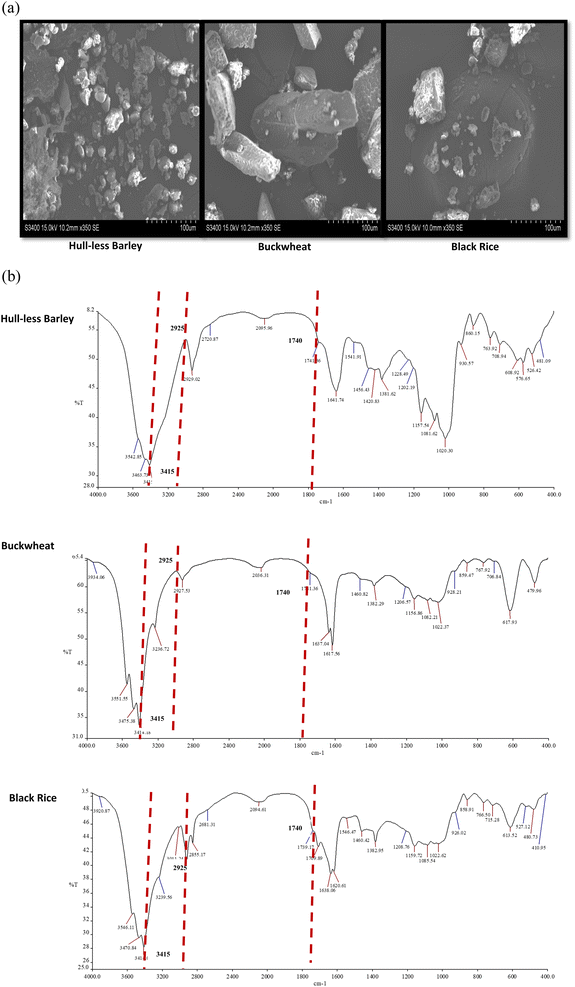 |
| Fig. 3 (a) Scanning electron microscopy of highland grains (b) FT-IR analysis of highland grains. | |
FT-IR spectra
The spectrum of FT-IR is studied to describe the functional groups' existence as well as information regarding the bond that reveals structural properties. In the present investigation, the FT-IR spectra were recorded to investigate the structural characterization of selected highland crops, as shown in Fig. 3(b). In selected highland crops, the characteristic band around 3415 cm−1 showed a similar trend of increasing wave number. This move in the peak characteristic could be attributed to the stretching of the –OH bond, which significantly weakens the hydrogen bond in polysaccharides found in cellulose and hemicellulose. Surprisingly, these findings show partial cellulose or hemicellulose degradation in selected crops. The peak near 2925 cm−1 is attributed to the stretching of the –C–H group, which describes typical polymer characteristics, as well as –H–C–H and –C–O–H conjugated bending vibrations.26 Peaks around 1740 cm−1 represent the C
O carbonyl group stretching in hemicellulose, pectin, or lignin among the crops. The bands ranging from 1617.56 to 1641.74 cm−1 indicate lignin hydrocarbon stretching or bending, as well as the availability of uronic acid. In the samples, greater peak strength indicates the presence of a higher number of uronic acids. The peak shift from 1420.83 to 1460.42 cm−1 indicates a weakening of aliphatic or aromatic C–H groups, most likely lignin-derived, showing lignin breakdown.27 Further examination of the spectral data among crops revealed significantly decreased, shifted and vanished distinctive peaks in the range from 1700 to 1200 cm−1. This finding describes that cellulose, hemicellulose, pectin, lignin, and protein content in crops have been partially degraded.
Thermogravimetric analysis
A thermogravimetric analyzer was used to perform thermal disintegration in all samples (Fig. 4). TGA was performed at temperatures ranging from 50 to 400 °C, revealing alterations in the samples' weight loss and deterioration characteristics in the sample. Based on the temperature range thermal degradation can be split into three major stages. The first temperature stage ranged from 50 to 150 °C, and a significant loss in weight was noticed due to the absorbed water evaporation, degradation and devolatilization of different low-molecular-weight polysaccharides, which arises mostly at 121 °C. The second temperature stage affects loss in weight at temperatures ranging from 150 to 250 °C. The temperature at which a sudden change in deprivation characteristics was observed was around 214 °C for hull-less barley and black rice, whereas for buckwheat it was near 230 °C. In this stage, a weight loss of up to 15% was noticed with degradation temperatures for hull-less barley, buckwheat and black rice at 273.7 °C, 273.5 °C and 278.06 °C, respectively. This lessening of weight loss could be ascribed to the fragmentation of fiber via deoxylation, decarboxylation, or dihydroxylation. The third temperature stage was ranging from 250 to 400 °C and resulted in significant weight loss; up to 70% was observed among samples. At this stage, concerning the terminal degradation temperature, the mass loss was rapid, i.e., 334.10 °C, 339.45 °C and 343.91 °C, respectively. This speedy loss in mass could be attributed to hemicellulose, pectin, and lignin thermal degradation. Loss in weight, when the temperature is high, has also been linked to samples' molecular weight, with a positive correlation. Furthermore, the remaining residual mass near 400 °C for the crops hull-less barley, buckwheat, and black rice is 21.79%, 21.58%, and 24.04%, respectively. According to the literature, the weight loss in the temperature range from 400 and 600 °C has been primarily because of lignin and other complex polysaccharides.
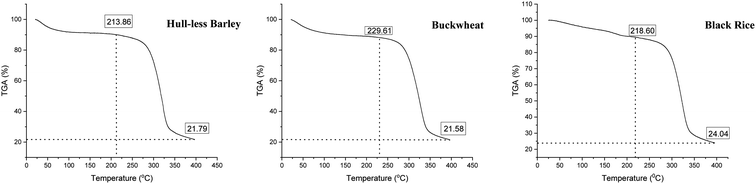 |
| Fig. 4 Thermogravimetric analysis (TGA). | |
Nutritional and mineral content
The nutritional parameters of selected crops are demonstrated in Table 2. From the results, it was observed that buckwheat contains a higher amount of crude protein content (12.33%) among the selected crops. Buckwheat grain has a high biological value and is a great source of protein.28 Protein content recorded least in black rice (7.11%) and these findings were comparable to those reported for other varieties of coloured rice.29 Similarly, the total fat, crude fiber and total ash content were also found highest in buckwheat grains, i.e., 2.56, 1.55 and 2.25%, respectively. These results were in accordance with the study conducted by Steadman et al.,30 who also observed 2.4 g ash/100 g, and 12.3 g protein/100 g in buckwheat grains. In the literature, the crude fiber content ranged between 0.92 and 1.74% in barley cultivars31 and 0.7% in buckwheat flour.32 Kaur et al.23 also recorded similar results for protein and ash content in hull-less barley in their study of comparative evaluation of different cereals. The body needs minerals for a variety of biochemical activities necessary for maintaining healthy growth and development. Insufficient intake of minerals from daily diet could lead to improper intellectual and physical development, weakened immune system, and cause other nutritional deficiency illnesses. Therefore, it is essential to examine the mineral content of food crops in order to find meals that are high in minerals. The selected crops were investigated for six minerals (copper, iron, zinc, calcium, magnesium and manganese). Table 2 describes the content of minerals in the studied highland crops. The mineral content namely copper, iron, zinc, calcium, magnesium and manganese varied from 1.39 to 3.38 ppm, 14.66 to 22.09 ppm, 5.81 to 18.99 ppm, 53.99 to 240.45 ppm, 14.73 to 134.94 ppm and 14.38 to 22.31 ppm, respectively. Thus, the minerals in the examined highland crops are comparable to those in the crops consumed in common and widely, and to some extent, they can satisfy the daily requirement for these minerals. In contrast to earlier research, the current study's buckwheat had different mineral concentrations. Similarly, the results for mineral content of black rice also differed little bit from the earlier findings and were observed to be 720, 270, 160, 54, 16, 9.7, 2.3, 2.1 and 0.47 mg kg−1 dry weight for K, Mg, Cl, Ca, Zn, Mn, Fe, Cu and Mo, respectively, as described by Tsukada et al.,33 whereas according to Podolska et al.34 Cu, Mn and Fe content of buckwheat ranged from 6.39 to 7.76, 8.90 to 9.37 and 24.22 to 29.99 mg kg−1, respectively. The mineral content of crops can be influenced by different variables, including both environmental and genetic impacts.35,36
Table 2 Nutritional and mineral content of highland grainsa,b
Grain |
Nutritional composition (%) |
Minerals (ppm) |
Moisture |
Crude protein |
Total fat |
Total ash |
Crude fiber |
Total CHO |
Cu |
Fe |
Zn |
Ca |
Mg |
Mn |
All values are means of three determinations (n = 3) ± standard deviation.
Total CHO: total carbohydrate; Cu: copper; Fe: iron; Zn: zinc; Ca: calcium; Mg: magnesium; Mn: manganese.
|
Hull-less barley |
7.69 ± 0.12b |
11.41 ± 0.14b |
1.24 ± 0.05c |
1.76 ± 0.06b |
0.61 ± 0.10b |
60.22 ± 0.18b |
3.38 ± 0.03b |
22.14 ± 7.44b |
15.31 ± 0.06b |
240.45 ± 0.05a |
14.73 ± 0.06b |
14.38 ± 0.05bc |
Buckwheat |
10.85 ± 0.07a |
12.33 ± 0.12a |
2.56 ± 0.10a |
2.25 ± 0.14a |
1.55 ± 0.05a |
58.43 ± 0.21c |
4.38 ± 0.02a |
19.66 ± 9.77a |
5.81 ± 0.02a |
110.26 ± 0.02b |
14.94 ± 0.04b |
15.53 ± 0.02b |
Black rice |
6.43 ± 0.08c |
7.11 ± 0.20c |
2.31 ± 0.04ab |
1.73 ± 0.08b |
0.41 ± 0.04b |
74.72 ± 0.22a |
1.39 ± 0.08c |
26.09 ± 9.78c |
18.99 ± 0.02c |
53.99 ± 0.02c |
134.94 ± 0.13a |
22.31 ± 0.02a |
Free amino acid profile and SDS-PAGE
The composition of amino acids in a crop is not only an indicator of its nutritional value, but also, this quality must be paired with protein digestibility. The free amino acid composition of selected highland crops is shown in Fig. 2(a). The results indicated that glutamic acid ranged from 25.1 to 107.3 μg g−1, methionine from 3.3 to 32.5 μg g−1, lysine from 0.3 to 12.0 μg g−1, leucine from 0.6 to 11.2 μg g−1, isoleucine from 2.5 to 48.6 μg g−1, arginine from 18.6 to 194.3 μg g−1, glycine from 1.0 to 22.1 μg g−1, tyrosine from 119.9 to 867.2 μg g−1, valine from 1.8 to 30.9 μg g−1, serine from 0.1 to 13.8 μg g−1, and tryptophan from 0.04 to 19.6 μg g−1. Most of the free amino acids are higher in buckwheat among the crops. For example, lysine was recorded highest in buckwheat (12.0 μg g−1), followed by hull-less barley (3.5 μg g−1), which is commonly deficient in most of the cereals.37 Lyu et al.38 found eighteen kinds of amino acids in highland barley, including glutamate (1.6 g/100 g), proline (0.78 g/100 g) and leucine (0.45 g/100 g), whereas Kang et al.39 observed aspartic (68.07–165.51 ng per mg rice), glutamic (138.91–232.29 ng per mg rice), and asparagines (73.55–242.89 ng per mg rice) in selected five black rice varieties.
SDS-PAGE of different highland crops viz. hull-less barley, buckwheat, and black rice was performed to obtain information on subunit molecular weight distribution patterns of protein (Fig. 2(b)). The primary grain proteins were divided into three groups with varying molecular weights (high, low and mid). Interestingly, in grains, it was seen that the mid-range molecular weight protein (37–75 kDa) was distributed well. In buckwheat, protein bands between 37 and 75 kDa were more intense, whereas in hull-less barley and black rice, most of the bands were observed between 75 and 250 kDa. A similar intense band of high molecular weight was observed by Wang et al.40 in stored buckwheat grains. Contrarily, in a study conducted by Kumar et al.14 more intense protein bands were found near 6.5 kDa and between 29 and 40 kDa in barley. Black rice showed one band near 100 kDa, which might be the specific protein band related to rice pericarp colour.41
Techno-functional analysis
The functional properties, WAI and OAI, measure how well the sample can connect with water and oil, respectively. Grains' functional characteristics provide information about the bond strengths shown in the starch granules as it helps during the development of food products.42 As demonstrated in Table 1, highland crops exhibited a WAI ranging from 1.46 to 1.73 g g−1 and OAI from 1.27 to 1.37 g g−1, respectively. The WAI and OAI results for hull-less barley closely resembled the findings of Dang et al.41 Variations in water absorption index among different crops could be due to variations in their protein concentration, water interaction levels, and structural traits.43 Buckwheat has the highest OAI (1.37 g g−1) as compared to other crops. A buckwheat protein has the capacity to bind with oil, making it suitable for use in food systems where oil absorption is crucial. The flour has a substantial amount of OAI, which is suitable for facilitating enhancement in flavour and mouthfeel when used in food formulation. The water solubility index was found highest in black rice (8.1%), followed by hull-less barley (5.9%) and buckwheat (4.2%). In the present study, the water activity ranged from 0.34 to 0.55 aw among the selected highland crops.
Bioactive profile
TPC, TFC and antioxidant properties.
Highland crops are rich in phenolic compounds, which in addition provide nourishment and have numerous health benefits. Among the studied crops, a considerable difference was found in the phenolic and flavonoid content (Table 1). The TPC ranged from 44.80 to 319.3 mg GAE per g, whereas the total flavonoid content ranged from 5.60 to 99.63 mg RU per g. Buckwheat contained the highest phenolic and flavonoid contents, followed by black rice and hull-less barley (Table 1). According to Zielinski & Kozlowska44 the whole grains of oats and barley had the highest total phenolic content when compared to wheat and rye, but were lower than buckwheat. According to Vollmannova et al.,45 the total phenolic content of five kinds of buckwheat was found to range from 138.10 to 286.99 mg GAE/100 g. The outer layer of grains' cell walls contained the highest concentration of phenolic chemicals, which are primarily esterified to the arabinose side groups of arabinoxylans.46 Selected crops were examined for their antioxidant potential using two free radical assays namely DPPH and ABTS as demonstrated in Table 1. Crops showed significant variability in their inhibitory activity against DPPH and ABTS radicals. Among the crops, in both assays, as expected, buckwheat exhibited the highest scavenging activity followed by black rice and hull-less barley. The variation in the antioxidant activity among grains was influenced by various factors viz. genetic and environmental.47 The results of the correlation analysis between the bioactive chemicals and the IC50 values for the DPPH and ABTS antioxidant assays revealed a substantial positive association between them.
Phenolic profiling.
The phenolic profiling of highland grains revealed the presence of several bioactive compounds. The presence of phenolic compounds in ingredients contributes to the quality of food products. A strong correlation between the phenolic profile and antioxidant parameters of selected highland crops was observed. Rutin was recorded highest in buckwheat (7031.8 μg g−1) followed by hull-less barley (72.1 μg g−1) and black rice (25.1 μg g−1) (Table 4). Rutin has been found to be more abundant in buckwheat than in most other plants, making it a beneficial source of flavonoids. Among the selected grains, quercetin was found only in buckwheat (118.5 μg g−1). Catechin was found to be highest in hull-less barley (71.3 μg g−1) followed by black rice (48.5 μg mL−1) and buckwheat (20.0 μg g−1). Ferulic acid was recorded at 568.2 μg g−1 in hull-less barley and 567.3 μg g−1 in black rice, whereas it was below the detection limit in buckwheat.
Antimicrobial activity.
Selected highland crops were tested for antimicrobial activity against six bacterial strains, and the outcomes are demonstrated in Table 3. Buckwheat and black rice were found to show inhibition against all six tested bacteria, whereas hull-less barley showed inhibition against five out of six studied microorganisms. Buckwheat, hull-less barley and black rice showed the highest inhibition against Escherichia coli MTCC 43, Staphylococcus Aureus MTCC 96 and Bacillus subtilis MTCC 121, respectively. Our results for barley were in agreement with a previous study conducted by Baliyan et al.,17 where the barley showed activity against similar bacterial strains. The results of the current investigation showed that the selected crops are effective at limiting the growth of tested Gram-positive and Gram-negative bacteria. Since the crops have demonstrated possible inhibitory effects against significant bacterial strains, their potential as natural antibacterial agents can be investigated.
Table 3 Antimicrobial properties of highland grainsa
Grain |
Zone of inhibition (mm) |
Salmonella typhi MTCC 733 |
Staphylococcus aureus MTCC 96 |
Bacillus subtilis MTCC 121 |
Escherichia coli MTCC 43 |
Pseudomonas aeruginosa MTCC 2453 |
Micrococus luteus MTCC 2470 |
All values are means of three determinations (n = 3) ± standard deviation.
|
Hull-less barley |
— |
8.0 ± 0.12c |
2.0 ± 0.08c |
4.0 ± 0.14c |
2.0 ± 0.08c |
4.0 ± 0.03c |
Buckwheat |
16.0 ± 0.04a |
16.0 ± 0.16a |
15.0 ± 0.06b |
20.0 ± 0.12a |
17.0 ± 0.08a |
17.0 ± 0.02a |
Black rice |
12.0 ± 0.05b |
11.0 ± 0.10b |
18.0 ± 0.04a |
15.0 ± 0.18b |
14.0 ± 0.06b |
12.0 ± 0.06b |
Table 4 Phenolic profile of highland grainsa,b
Grain |
SPF (mg GAE per g) |
TFC (mg RU per g) |
IC50 of free radical scavenging activities (mg mL−1) |
Chlorogenic acid (μg g−1) |
Catechin (μg g−1) |
Rutin (μg g−1) |
Vanillic acid (μg g−1) |
Quercetin (μg g−1) |
Ferulic acid (μg g−1) |
DPPH |
ABTS |
All values are means of three determinations (n = 3) ± standard deviation.
SPF: soluble phenolic fraction; TFC: total flavonoid content; DPPH: (1,1-dicrylhydrazyl); ABTS: (2,2′-azinobis (3-ethylbenzothiazoline-6-sulphonic acid).
|
Hull-less barley |
44.80 ± 0.21c |
5.60 ± 0.09c |
165.21 ± 0.34a |
231.05 ± 0.67a |
59.0 ± 0.21a |
71.3 ± 0.11a |
72.1 ± 0.04b |
12.0 ± 0.10a |
— |
568.2 ± 0.15a |
Buckwheat |
319.3 ± 0.34a |
99.63 ± 0.16a |
50.51 ± 0.22c |
115.54 ± 0.25c |
32.5 ± 0.20b |
20.0 ± 0.46c |
7031.8 ± 3.51a |
— |
118.5 ± 0.04a |
— |
Black rice |
77.3 ± 0.12b |
32.00 ± 0.06b |
106.24 ± 0.33b |
188.12 ± 0.27b |
— |
48.5 ± 0.02b |
25.1 ± 0.07c |
— |
— |
567.3 ± 0.11b |
Cell viability.
Cell viability was assessed by measuring the capacity of mitochondrial dehydrogenases to catalyse MTT reduction to formazan crystals. The results of the cell viability assay demonstrated the safety of the process. From the results, it was observed that all samples were determined to be safe up to 400 μg mL−1. Therefore, even at the greatest dose, no detectable cell damage was seen (Fig. 5(a)).
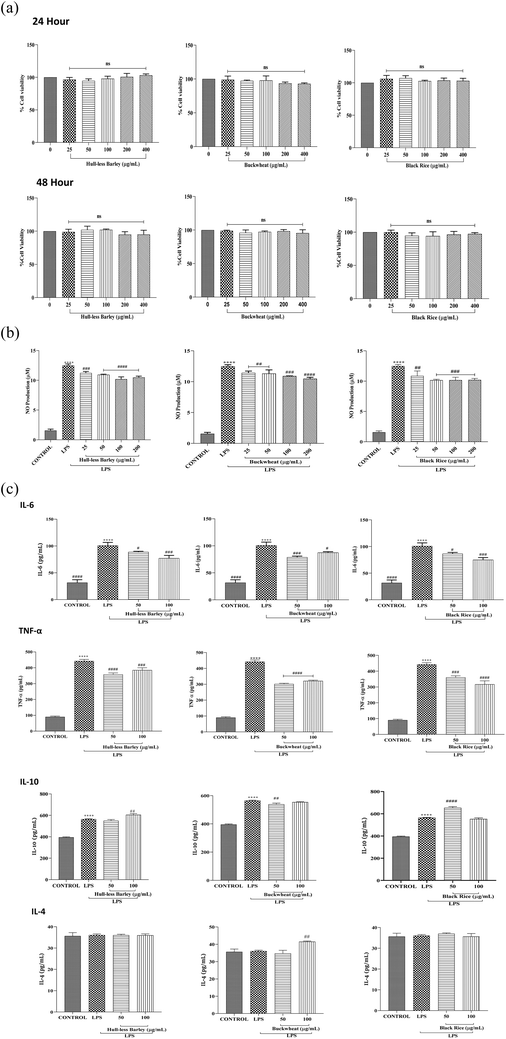 |
| Fig. 5 (a) Cell viability of highland grains (b) NO production of highland grains (c) cytokine expression of highland grains. Data are mean ± SD from the experiment. Here, ##p < 0.01, ###p < 0.001, and ####p < 0.0001 indicate significant difference from the LPS group, whereas ****p < 0.0001 indicates significant difference from the control. | |
Effect on NO production and cytokine expression.
The development of inflammation is significantly influenced by the signalling molecule NO. Hence, foods that prevent NO formation are also thought of as therapeutic agents for treating diseases linked to inflammation. Macrophages release NO in the activated state, which is frequently employed to assess the immunomodulatory activity of substances. Therefore, the activated macrophages were treated with various concentrations of crop extracts to analyse the impact of highland crops on NO production (Fig. 5(b)). The results of the NO investigation demonstrated that LPS-stimulated cells had a substantial rise in NO production levels. As seen in Fig. 5(b), LPS stimulation led to a significant (p ≤ 0.05) increase in NO levels as compared to the control. In contrast, each crop extract (25, 50, 100, and 200 μg mL−1) significantly (p ≤ 0.05) yet concentration independently inhibited NO generation in LPS-stimulated macrophages. The data obtained from black rice in the present investigation was consistent with the study done by Petron et al.,48 in which they demonstrated that extracts from integral, black, red, and bran rice particularly decreased the expression of iNOS, which is in charge of producing NO, in response to an inflammatory stimulus without changing the secretion of cytokines.
In several acute or chronic inflammation-related illnesses, dysregulation of pro-inflammatory cytokines such as TNF-α and IL-6 has been found. Therefore, further, we elucidate the effects of the selected crops on the secretion of TNF-α, IL-10, IL-4 and IL-6 by LPS-stimulated macrophages (Fig. 5(c)). In the current investigation, we found that LPS treatment resulted in higher levels of cytokine (TNF-α and IL-6) expression as compared to the control and simultaneously IL-10 and IL-4 expression declined. However, remarkably much more IL-10 and IL-4 productions were seen in the presence of highland crops, indicating that these crops might have immunomodulatory attributes. These findings demonstrated that these crops have immunosuppressive as well as both anti-inflammatory and immunoregulatory functions as they can effectively modulate the Th2 cytokine profile that contributes to the progression of spleen inflammation and injury. Additionally, highland crop extract treatment reversed the effects of LPS with a notable reduction in the expression of inflammatory cytokines (TNF-α and IL-6) and a concurrent increase in the expression of IL-10 and IL-4. IL-10 is also involved in the regulation of autoimmune diseases, allergies, and inflammatory conditions. Thus, these crops can be a therapeutic target for various inflammatory disorders. These observations suggested that these crops have a modulatory effect on both regulatory molecules and effector inflammatory molecule production, indicating their efficacy in preserving inflammatory homeostasis. The present findings are similar to a previous study conducted by Ishii et al.,49 where LPS intake was reduced by combinatory treatment with the extract of buckwheat, as it nearly inhibited all of the gene expression produced by LPS treatment. Indeed, these results were also in accordance with a study conducted by Kim et al.,50 who observed the inhibitory potential of buckwheat extract on mast cells. Additionally, black rice extracts have also been proven to reduce the formation of lipopolysaccharide (LPS), and production of cytokine signalling molecules including TNF-α, IL-6 and NO.51
Conclusions
The current study explored the nutritional, functional, morphological, and immunomodulatory potential of some high-valued cereal crops of highland, in order to encourage their consumption and to conserve their populations. The findings showed that these crops are rich in significant nutritive value and antioxidant properties, making them suitable for use in diets as nutraceuticals or functional foods to enhance the health and quality of human life. Moreover, these crops exhibited strong in vitro anti-inflammatory action (immunomodulatory attributes), suggesting that they could be a potential candidate for the development of various food products and for the treatment or slowing of the progression of inflammatory illnesses. Therefore, to fulfil the nutritional need on a commercial scale, it is advised to plant and use these high-valued crops on a big scale for the sustainable use of natural resources.
Author contributions
Rashim Kumari: methodology, validation, data curation, and writing-original draft; Vijay Singh: experimental work; Yogendra Shantaram Padwad: reviewing and editing; Mahesh Gupta: conceptualization, supervision, writing-reviewing and editing.
Conflicts of interest
There are no conflicts to declare.
Acknowledgements
We state our gratitude to the Director, CSIR-Institute of Himalayan Bioresource Technology for their precious implications and support. I am thankful to DST WOS-B Scheme (DST/WOS/2018/1289-HFN) for awarding fellowship. The authors acknowledge Dr Avnesh Kumari, senior technical officer, biotechnology division, CSIR-IHBT for carrying out SEM analysis. This manuscript represents CSIR-IHBT communication number 5266.
Notes and references
- K. Sheela, K. G. Nath, D. Vijayalakshmi, G. M. Yankanchi and R. B. Patil, Proximate composition of underutilized green leafy vegetables in Southern Karnataka, J. Hum. Ecol., 2004, 15(3), 227–229, DOI:10.1080/09709274.2004.11905698.
- B. Liu, G. Ma, R. W. Bussmann, K. Bai, J. Li, W. Cao and C. Long, Determining factors for the diversity of hulless barley agroecosystem in the himalaya region—A case study from Northwest Yunnan, China, Global Ecology and Conservation, 2019, 18, e00600, DOI:10.1016/j.gecco.2019.e00600.
- S. M. Hosseini, N. Soltanizadeh, P. Mirmoghtadaee, P. Banavand, L. Mirmoghtadaie and S. Shojaee-Aliabadi, Gluten-free products in celiac disease: Nutritional and technological challenges and solutions, J. Res. Med. Sci., 2018, 23, 1–11, DOI:10.4103/jrms.JRMS_666_18.
- S. Arora, I. K. Virdi, V. S. Sharanagat, A. Kheto, S. Dhua, R. Suhag, R. Kumar, Y. Kumar and A. Patel, Roasting of black rice (Oryza sativa L.): Change in physico-functional, thermo-pasting, antioxidant and anthocyanin content, Journal of Food Measurement and Characterization, 2021, 15(3), 2240–2250, DOI:10.1007/s11694-021-00828-7.
- X. Li, R. Yadav and K. H. Siddique, Neglected and underutilized crop species: the key to improving dietary diversity and fighting hunger and malnutrition in Asia and the Pacific, Front. Nutr., 2020, 7, 593711, DOI:10.3389/fnut.2020.593711.
- J. M. Aguilera, Rational food design and food microstructure, Trends Food Sci. Technol., 2022, 122, 256–264, DOI:10.1016/j.tifs.2022.02.006.
- A. Rani, S. Sood and F. M. Bhat, Physico-chemical and Functional Properties of Three Hull-less Barley (Hordeum vulgare) Varieties Grown in the High Altitude Region, Int. J. Curr. Microbiol. Appl. Sci., 2020, 9(9), 2069–2077, DOI:10.20546/ijcmas.2020.909.258.
- N. S. Soliman, M. A. Abd El Maksoud, G. R. Gamea and Y. A. Qaid, Physical characteristics of wheat grains, Misr J. Agric. Eng., 2009, 26(4), 1855–1877, DOI:10.21608/MJAE.2009.107570.
- M. Nádvorníková, J. Banout, D. Herák and V. Verner, Evaluation of physical properties of rice used in traditional Kyrgyz Cuisine, Food Sci. Nutr., 2018, 6(6), 1778–1787, DOI:10.1002/fsn3.746.
- R. Kumari, V. Abhishek and M. Gupta, In-vitro starch digestibility, nutritional-functional and texture properties of hull less barley incorporated extruded noodles, Vegetos, 2021, 34(1), 205–211, DOI:10.1007/s42535-021-00187-0.
- R. Kumari and M. Gupta, Elucidating the techno-functional, morphological and phenolic properties of hull less barley and buckwheat incorporated pasta, Food Chem. Adv., 2022, 1, 100055, DOI:10.1016/j.focha.2022.100055.
-
AOAC, AOAC Official Methods of Analysis, Association of Official Analytical Chemists, Arlington, 2005 Search PubMed.
-
S. S. Nielsen, Total Carbohydrate by Phenol-Sulfuric Acid Method, in Food Analysis Laboratory Manual, Food Science Text Series, Springer, 2017, pp. 137–141, DOI:10.1007/978-3-319-44127-6_14.
- K. A. Kumar, G. K. Sharma and K. R. Anilakumar, Influence of multigrain premix on nutritional, in-vitro and in-vivo protein digestibility of multigrain biscuit, J. Food Sci. Technol., 2019, 56(2), 746–753, DOI:10.1007/s13197-018-3533-z.
- S. Bhatt and M. Gupta, Exploration of soluble dietary fiber extraction technique for enhancing physicochemical and structural properties of mango and pomegranate peel, Biomass Convers. Biorefin., 2022, 9(1), 1–16, DOI:10.1007/s13399-022-02545-7.
- J. Chlopicka, P. Pasko, S. Gorinstein, A. Jedryas and P. Zagrodzki, Total phenolic and total flavonoid content, antioxidant activity and sensory evaluation of pseudocereal breads, LWT–Food Sci. Technol., 2012, 46(2), 548–555, DOI:10.1016/j.lwt.2011.11.009R; V. A. Kumari and M. Gupta, In-vitro starch digestibility, nutritional-functional and texture properties of hull less barley incorporated extruded noodles, Vegetos, 2021, 34(1), 205–211, DOI:10.1007/s42535-021-00187-0.
- N. Baliyan, K. Dindhoria, A. Kumar, A. Thakur and R. Kumar, Comprehensive Substrate-Based Exploration of Probiotics from Undistilled Traditional Fermented Alcoholic Beverage ‘Lugri, Front. Microbiol., 2021, 12, 626964, DOI:10.3389/fmicb.2021.626964.
- I. Doskocil, J. Havlik, R. Verlotta, J. Tauchen, L. Vesela, K. Macakova, L. Opletal, L. Kokoska and V. Rada, In vitro immunomodulatory activity, cytotoxicity and chemistry of some central European polypores, Pharm. Biol., 2016, 54(11), 2369–2376, DOI:10.3109/13880209.2016.1156708.
- H. Unal, G. Izli, N. Izli and B. B. Asik, Comparison of some physical and chemical characteristics of buckwheat (Fagopyrum esculentum Moench) grains, CyTA–J. Foodd, 2017, 15(2), 257–265, DOI:10.1080/19476337.2016.1245678.
- Y. P. Bai, H. M. Zhou, K. R. Zhu and Q. Li, Effect of thermal treatment on the physicochemical, ultrastructural and nutritional characteristics of whole grain highland barley, Food Chem., 2021, 346, 128657, DOI:10.1016/j.foodchem.2020.128657.
- Z. Kaliniewicz, P. Markowski, A. Anders and K. Jadwisieńczak, Frictional properties of selected seeds, Tech. Sci., 2015, 18, 85–101 Search PubMed.
- L. T. G. F. Brites, F. Ortolan, D. W. D. Silva, F. R. Bueno, T. D. S. Rocha, Y. K. Chang and C. J. Steel, Gluten-free cookies elaborated with buckwheat flour, millet flour and chia seeds, Food Sci. Technol., 2018, 39, 458–466, DOI:10.1590/fst.30416.
- H. Kaur, B. S. Gill and B. L. Karwasra, Physicochemical, functional, pasting and antioxidant properties of flours from different cereals: A comparative evaluation, Int. J. Adv. Biotechnol. Res., 2017, 8(2), 1220–1231 CAS.
- H. H. Wijngaard, S. Renzetti and E. K. Arendt, Microstructure of buckwheat and barley during malting observed by confocal scanning laser microscopy and scanning electron microscopy, J. Inst. Brew., 2007, 113(1), 34–41, DOI:10.1002/j.2050-0416.2007.tb00253.x.
- C. K. Reddy, L. Kimi and S. Haripriya, Variety difference in molecular structure, functional properties, phytochemical content and antioxidant capacity of pigmented rice, Journal of Food Measurement and Characterization, 2016, 10(3), 605–613, DOI:10.1007/s11694-016-9344-x.
- Y. Zhang, J. Liao and J. Qi, Functional and structural properties of dietary fiber from citrus peel affected by the alkali combined with high-speed homogenization treatment, LWT–Food Sci. Technol., 2020, 128, 109397, DOI:10.1016/j.lwt.2020.109397.
- W. Dong, D. Wang, R. Hu, Y. Long and L. Lv, Chemical composition, structural and functional properties of soluble dietary fiber obtained from coffee peel using different extraction methods, Food Res. Int., 2020, 136, 109497, DOI:10.1016/j.foodres.2020.109497.
- G. Bonafaccia, M. Marocchini and I. Kreft, Composition and technological properties of the flour and bran from common and tartary buckwheat, Food Chem., 2003, 80(1), 9–15, DOI:10.1016/S0308-8146(02)00228-5.
- J. L. F. Monks, N. L. Vanier, J. Casaril, R. M. Berto, M. de Oliveira, C. B. Gomes, M. P. de Carvalho, A. R. G. Dias and M. C. Elias, Effects of milling on proximate composition, folic acid, fatty acids and technological properties of rice, J. Food Compos. Anal., 2013, 30(2), 73–79, DOI:10.1007/s10068-017-0259-z.
- K. J. Steadman, M. S. Burgoon, B. A. Lewis, S. E. Edwardson and R. L. Obendorf, Minerals, phytic acid, tannin and rutin in buckwheat seed milling fractions, J. Sci. Food Agric., 2001, 81(11), 1094–1100, DOI:10.1002/jsfa.914.
- P. Sharma and H. S. Gujral, Milling behavior of hulled barley and its thermal and pasting properties, J. Food Eng., 2010, 97(3), 329–334, DOI:10.1016/j.jfoodeng.2009.10.025.
- L. Sinkovič, D. K. Sinkovič and V. Meglič, Milling fractions
composition of common (Fagopyrum esculentum Moench) and Tartary (Fagopyrum tataricum (L.) Gaertn.) buckwheat, Food Chem., 2021, 365, 130459, DOI:10.1016/j.foodchem.2021.130459.
- H. Tsukada, H. Hasegawa, A. Takeda and S. Hisamatsu, Concentrations of major and trace elements in polished rice and paddy soils collected in Aomori, Japan, J. Radioanal. Nucl. Chem., 2007, 273(1), 199–203, DOI:10.1007/s10967-007-0736-6.
- G. Podolska, E. Gujska, J. Klepacka and E. Aleksandrowicz, Bioactive compounds in different buckwheat species, Plants, 2021, 10(5), 961, DOI:10.3390/plants10050961.
- L. X. Peng, Y. F. Huang, L. I. U. Yuan, Z. F. Zhang, L. Y. Lu and Z. H. A. O. Gang, Evaluation of essential and toxic element concentrations in buckwheat by experimental and chemometric approaches, J. Integr. Agric., 2014, 13(8), 1691–1698, DOI:10.1016/S2095-3119(13)60724-8.
- F. E. Prado, J. L. Fernández-Turiel, M. Tsarouchi, G. K. Psaras and J. A. González, Variation of seed mineral concentrations in seven quinoa cultivars grown in two agroecological sites, Cereal Chem., 2014, 91(5), 453–459, DOI:10.1094/CCHEM-08-13-0157-R.
- P. Ratan and P. Kothiyal, Fagopyrum esculentum Moench (common buckwheat) edible plant of Himalayas: A Review, Asian J. Pharm. Life Sci., 2011, 2231, 4423 Search PubMed.
- Y. Lyu, S. Ma, J. Liu and X. Wang, A systematic review of highland barley: Ingredients, health functions and applications, Grain Oil Sci. Technol., 2022, 5(1), 35–43, DOI:10.1016/j.gaost.2021.12.002.
- M. Y. Kang, J. H. Kim, C. W. Rico and S. H. Nam, A comparative study on the physicochemical characteristics of black rice varieties, Int. J. Food Prop., 2011, 14(6), 1241–1254, DOI:10.1080/10942911003637350.
- L. Wang, L. Wang, A. Wang, J. Qiu and Z. Li, Inhibiting effect of superheated steam processing on milling characteristics deterioration induced by storage of common buckwheat, LWT–Food Sci. Technol., 2021, 145, 111375, DOI:10.1016/j.lwt.2021.111375.
- D. R. T. Sari, A. Safitri, J. R. K. Cairns and F. Fatchiyah, Protein profiling of coloring rice (Oryza sativa L.) using SDS-PAGE and experionTM260 analysis, J. Phys.: Conf. Ser., 2019, 1146(1), 012038, DOI:10.1088/1742-6596/1146/1/012038.
- B. Dang, W. G. Zhang, J. Zhang, X. J. Yang and H. D. Xu, Effect of thermal treatment on the internal structure, physicochemical properties and storage stability of whole grain highland barley flour, Foods, 2022, 11(14), 2021, DOI:10.3390/foods11142021.
- M. S. Butt and B. Rizwana, Nutritional and functional properties of some promising legumes protein isolates, Pak. J. Nutr., 2010, 9(4), 373–379, DOI:10.3923/pjn.2010.373.379.
- H. Zieliński and H. Kozłowska, Antioxidant activity and total phenolics in selected cereal grains and their different morphological fractions, J. Agric. Food Chem., 2000, 48(6), 2008–2016, DOI:10.1021/jf990619o.
- A. Vollmannova, E. Margitanova, T. Tóth, M. Timoracka, D. Urminska, T. Bojňanská and I. Čičová, Cultivar influence on total polyphenol and rutin contents and total antioxidant capacity in buckwheat, amaranth, and quinoa seeds, Czech J. Food Sci., 2013, 31(6), 589–595, DOI:10.17221/452/2012-cjfs.
- P. Sharma and H. S. Gujral, Antioxidant and polyphenol oxidase activity of germinated barley and its milling fractions, Food Chem., 2010, 120(3), 673–678, DOI:10.1016/j.foodchem.2009.10.059.
- L. Yu, J. Perret, M. Harris, J. Wilson and S. Haley, Antioxidant properties of bran extracts from “Akron” wheat grown at different locations, J. Agric. Food Chem., 2003, 51(6), 1566–1570, DOI:10.1021/jf020950z.
- K. Petroni, M. Landoni, F. Tomay, V. Calvenzani, C. Simonelli and M. Cormegna, Proximate composition, polyphenol content and anti-inflammatory
properties of white and pigmented Italian rice varieties, Univers. J. Agric. Res., 2017, 5(5), 312–321, DOI:10.13189/ujar.2017.050509.
- S. Ishii, T. Katsumura, C. Shiozuka, K. Ooyauchi, K. Kawasaki, S. Takigawa, T. Fukushima, Y. Tokuji, M. Kinoshita, M. Ohnishi and M. Kawahara, Anti-inflammatory effect of buckwheat sprouts in lipopolysaccharide-activated human colon cancer cells and mice, Biosci., Biotechnol., Biochem., 2008, 72(12), 3148–3157, DOI:10.1271/bbb.80324.
- C. D. Kim, W. K. Lee, K. O. No, S. K. Park, M. H. Lee, S. R. Lim and S. S. Roh, Anti-allergic action of buckwheat (Fagopyrum esculentum Moench) grain extract, Int. Immunopharmacol.y, 2003, 3(1), 129–136, DOI:10.1016/s1567-5769(02)00261-8.
- V. Shalini, S. Bhaskar, K. S. Kumar, S. Mohanlal, A. Jayalekshmy and A. Helen, Molecular mechanisms of anti-inflammatory action of the flavonoid, tricin from Njavara rice (Oryza sativa L.) in human peripheral blood mononuclear cells: possible role in the inflammatory signaling, Int. Immunopharmacol., 2012, 14(1), 32–38, DOI:10.1016/j.intimp.2012.06.005.
|
This journal is © The Royal Society of Chemistry 2023 |
Click here to see how this site uses Cookies. View our privacy policy here.