Caramel colour pigments from black sapote (Diospyros digyna): obtention and food application
Received
28th October 2022
, Accepted 23rd May 2023
First published on 24th May 2023
Abstract
This work aimed to develop a pigment from black sapote (Diospyros digyna) using lactic acid bacteria or yeasts for fermentation for use as a pigment in gummy bear formulations. Black sapote pulp was fermented with Lactobacillus casei, Lactobacillus rhamnosus, and Saccharomyces cerevisiae. Pigments were obtained by liquid–liquid extraction with an 80% ethanol solution and fermented purée in a 1
:
1 (v/v) ratio. The extract was added with 5% of maltodextrin–mesquite gum (1
:
1 w/w) blend and microencapsulated by spray drying at 150 °C (inlet temperature). The physical characteristics (yield, moisture content, water activity (aw), colour and particle size), antioxidant capacity (AC), total phenolic compounds (TPC), and their stability at various pHs and temperatures were evaluated. The pigment powders were added at 1, 3, and 5% to the gummy bear formulations. Gummies were evaluated by colour (using a colorimeter) and some sensory characteristics. The results showed that the fermentation process increased the AC and TPC characteristics, particularly when fermentation was performed with L. rhamnosus. The AC was reduced during drying, but the light brown colour barely remained the same (L* = 61.87–67.18, a* = −0.09–4.6, and b* = 27.97–29.26). Powders obtained after fermentation with L. casei and L. rhamnosus rendered approximately 10% lower yields than the control and sample fermented with S. cerevisiae. The moisture content and aw were lower than 9.04% and 0.190, respectively, in all the powders. The particle size of the powders was in the range 0.75 to 352 μm (due to agglomerations). The stability study showed that the pH significantly (p < 0.05) changed the pigment colour parameters (L*, a*, and b*). Temperature, on the other hand, changed the colour of reconstituted powders, showing a brownish-pale green colour. Finally, by increasing the pigment concentration in the gummy bear formulations, the colour changed from light brown to almost black; however, this did not influence the acceptability of the gummy bears by consumers.
1 Introduction
Colour in foods is one of the most important appearance characteristics. The colour of foods may help in identifying the flavours and is also a quality indicator that can affect the consumer acceptability, preference, or desire over various products.1,2 On the other hand, the addition of colourants or pigments helps in standardising the colour, increase the colour intensity, or restore the colour losses during processing. Also, colouring materials are added to satisfy the consumer expectations or for colouring non coloured foods such as beverages, soft drinks, bakery products, or confectionery.3–5 In particular, the confectionary industry uses colouring materials due to the lack of colour of the main ingredients. Gummy (confectionary gels) foods are prepared with glucose syrups, starch, gelatine, water, and occasionally agar, pectin, and/or alginates,6,7 which are some texturising food materials; all these ingredients are colourless. Even though colour is added only for appearance, it is a crucial step in food production. More than 87% of candy products use synthetic colourants in their formulations.7
Food colourants are classified into artificial/synthetic, nature-identical, and natural.5 One of the most used colourant in foods and beverages is the caramel colour (CAS no. 8028-89-5), which gives red to dark-brown colours. The controlled heating of carbohydrates (caramelisation) can produce caramel colours.8–10 This type of colourant has a burnt sugar flavour and a slightly bitter taste,11 depending on the heating time and temperature. The molecule responsible for colour in caramel colourants is 4-methylimidazole, among many other chemical compounds produced during heating. Different studies have indicated that the high consumption of 4-methyl imidazole does not represent any risk to the human health in terms of carcinogenic potential characteristics because only small amounts of the caramel pigment ingredients are absorbed.11 Other studies have related the caramel colourant intake to high blood pressure and hypertension.9 However, consumer concerns about health due to the intake of colourants have increased as well as the effect of colourants after long-term consumption. Therefore, the food industry is seeking to replace synthetic colourants with novel and natural pigments. One option could be the combination of natural pigments, such as red betalains, anthocyanins, yellow β-carotene, and blue pigments from spirulina.12 Nonetheless, the combination of pigments could be a problem in obtaining the accurate colour; in addition, some of these pigments could be unstable to heat and/or pH.
Black sapote (Diospyros digyna) is a Mexican endemic fruit,13 which is mostly consumed as a seasonal fruit or as an ingredient for preparing desserts. The fruit composition includes many vitamins, minerals, and bioactive compounds.13–15 Some of these bioactive compounds are pigments that might possess nutraceutical properties that can help overcome numerous illnesses.13,15,16 The pulp of black sapote contains carotenoids (β-carotene and lycopene), xanthophylls (lutein and β-kryptoxanthin), chlorophylls, anthocyanins, and other phenolic compounds, which gives its characteristic dark brownish-black colour (by which it receives its name) when the fruit is mature.13,15,17 The addition of these pigments into food matrixes might produce highly-coloured foods with nutraceutical properties.
Thus, the objective of this study was to obtain pigments from black sapote pulp using fermentation to increase the antioxidant characteristics, evaluate the fermentation effect on the colour, and encapsulate the pigments by spray drying for use as gummy bear formulations.
2 Materials and methods
2.1. Plant material
Black sapote fruits were harvested from trees grown in Puebla, Mexico. Unripe fruits (hard texture) were collected and stored (separated) at room temperature (22 ± 2 °C) at a relative humidity of 80 ± 5% until they acquired edible characteristics (peel with deep green colour with brown spots and soft texture of the pulp when touching). Mature fruits were washed with tap water, dried, placed in polypropylene bags, sealed, and stored at −20 °C until use.
2.2. Black sapote pulp
Black sapote fruits were thawed at room temperature (22 °C). Peel and seeds were removed manually. The seedless pulp (17.11 ± 1.32 °Bx) was blended with a NutriBullet blender (Nutribullet, NB-101B, China) until obtaining a purée with a smooth texture.
2.3. Pulp fermentation and pigment extraction
2.3.1. Microorganisms.
Lactobacillus casei, Lactobacillus rhamnosus, and Saccharomyces cerevisiae were obtained from the Food Microbiology Laboratory Collection at the Universidad de las Americas Puebla (UDLAP, Cholula, Puebla, Mexico). Lactobacillus were previously seeded in 100 mL sterile de Man, Rogosa & Sharp (MRS) broth (MERCK, Germany). Yeasts were seeded in 100 mL Sabouraud Dextrose Broth (BD, Difco, USA). Microorganisms were grown at 37 °C in a shaking bath until reaching the stationary phase. Then, microorganisms were preserved in 1 mL vials in a 1
:
1 ratio with glycerol (80% v/v) at −20 °C.
2.3.2. Fermentation.
One vial of each microorganism was thawed and regrown in its respective broth for 3 days at 37 °C to obtain biomass. The broth rich in microorganisms was then centrifuged (Sorvall ST 8R centrifuge, ThermoFisher Scientific, Germany) using sterilised centrifuge tubes at 11
470×g (5 °C) for 20 min. The biomass was added to 100 g purée at 1
:
100 (w/w) ratio, mixed vigorously, and fermented for 4 days at 37 °C. Homogenisation with a vortex at high speed was made every 12 hours to ensure microorganisms growth in the purée.
2.3.3. Pigment and other bioactive compounds extraction.
Extraction was performed by the liquid–liquid procedure at a 1
:
1 ratio of ethanol (80% v/v) and fermented purée for 10 min in a shaking bath at room temperature. The mixture was then centrifugated at 11
470×g (5 °C) for 15 min. Therefore, two more extractions were made at the same conditions at room temperature. The supernatants were combined and concentrated in a rotary evaporator (R-300, Büchi, Flawil, Switzerland) at 40 °C and 70 mmHg until three-quarters of the initial volume were evaporated. The final volume was completed to 100 mL with distilled water. The total solids in the extracts were about 4.26 ± 0.63%.
2.4. Antioxidant capacity and total phenolic compounds of extracts
2.4.1. DPPH assay.
The DPPH (2,2-diphenyl-1-picrylhydrazyl) assay was performed in a Microplate Spectrophotometer (Multiskan SkyHigh, Thermo Scientific, Singapore) following the methodology proposed by Jiménez-González & Guerrero-Beltrán18 with some modifications. Briefly, the assay involves mixing an aliquot of each sample (20 μL) with 200 μL of a proper dilution of 0.1 mM DPPH ethanolic solution. The mixture was homogenised using the shaking function of the UV-vis Multiskan Sky Microplate (Thermo Scientific, Singapore). The mixture was kept in the dark for 30 min at room temperature. Absorbances (A) were measured at 517 nm and used to calculate the inhibition percentage (I%) of the antioxidant capacity (eqn (1)). Trolox was used for quantifying the antioxidant capacity with a calibration curve: I(%) = 0.902x(0 − 100 mg L−1) + 0.763, R2 = 0.991. The results of the antioxidant capacity with the DPPH assay (DPHHAC) were expressed as mg of Trolox equivalents (TE) per g of sample on dry basis (db) (eqn (2)). All samples were analysed in triplicate. | 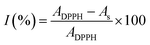 | (1) |
|  | (2) |
where ADPPH is the absorbance of the blank (free radical without sample or standard), As is the absorbance of the solutions after 30 min of reaction (for each sample, a blank was also measured), and DF is the dilution factor.
2.4.2. ABTS assay.
The ABTS assay was performed as proposed by Gómez-García et al.19 with some modifications. The ABTS˙ radical was formed by mixing 7 mM ABTS (5 mL) and 2.45 mM potassium persulfate (5 mL); the mixture was kept in the dark at room temperature for a minimum of 16 hours. A further dilution was performed to reach an absorbance value of 0.700 ± 0.020 at 754 nm. Then, 15 μL of each sample was mixed with 200 μL of the ABTS˙ solution and maintained at room temperature in the dark for 6 min before reading the absorbance at 734 nm using a microplate reader. A standard curve was made with Trolox at different concentrations: IABTS(%) = 0.899x(0 − 100 mg L−1) − 0.688, (R2 = 0.973). The results of the antioxidant capacity with the ABTS assay (ABTSAC) were expressed as mg of Trolox equivalents (TE) per g of sample in db using eqn (3) and (4). All samples were analysed in triplicate. | 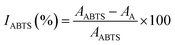 | (3) |
|  | (4) |
where AABTS is the absorbance of the blank (free radical without sample or standard), AA is the absorbance of each solution, and DF is the dilution factor.
2.4.3. Ferric reducing antioxidant power (FRAP) assay.
The FRAP microplate assay was conducted using the Bolanos de la Torre et al.20 methodology. The FRAP reagent was prepared by mixing acetate buffer (300 mM, pH 3.6), 2,4,6-tris(2-pyridyl)-s-triazine (TPTZ) solution (0.01 M in 40 mM HCl at 50 °C), and ferric chloride hexahydrate solution (0.02 M) in a 10
:
1
:
1 ratio and incubated at 37 °C for 10 min. Then, 20 μL of each sample was added to 280 mL FRAP solution and mixed thoroughly. The 96-well plate was kept in the dark for 30 min at 37 °C; then, the absorbances were measured at 593 nm using a microplate reader. All samples and standards for the standard curve y = 0.0044x(0 − 170 mg L−1) − 0.0152, (R2 = 0.944) were measured in triplicate. The results of the antioxidant capacity with FRAP (AAFRAP) were calculated and reported as mg of FeSO4·7H2O per g of sample in db using eqn (5). |  | (5) |
where AFRAP is the absorbance of each solution (sample/standard) and DF is the dilution factor.
2.4.4. Total phenolic compounds.
Total phenolic compounds (TPC) were evaluated by the Folin–Ciocalteu method using a 96-well microplate as previously reported by Ahmad et al.21 with some modifications. In a microplate, 20 μL of each sample was added, followed by 100 μL 0.1 M Folin–Ciocalteu reagent. Samples were mixed thoroughly, and after 3 min, 100 μL 2 M Na2CO3 was added. All solutions were kept in the dark for 30 min at room temperature. The absorbance was measured at 765 nm using a UV-vis Microplate. Gallic acid standard dilutions were prepared to make a calibration curve: y = 0.0045x(0 − 200 mg L−1) + 0.452, (R2 = 0.971). The results were reported as mg gallic acid equivalents (GAE per g of sample) and calculated using eqn (6). | 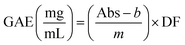 | (6) |
where Abs is the absorbance of each solution (sample/standard) and DF is the dilution factor.
2.5. Spray drying encapsulation
The pigments' encapsulation was performed by homogenising the concentrated extract with the encapsulating agents (maltodextrin and mesquite gum in a 1
:
1 w/w ratio) at a 5% (w/v) concentration. 100 mL of each mixture was passed through the spray dryer (Mini spray-dryer B290, Büchi, Switzerland) at 150 ± 3 and 95 ± 3 °C of inlet and outlet temperatures, respectively. The drying conditions were a feed flow of 10 rpm (10 ± 0.8 mL min−1), 100% aspiration, 1.05 bar air pressure, and 0.7 mm spray nozzle pressure. The powder was collected in sealed jars, covered with aluminium foil, and stored in a desiccator until analysis or used as a food pigment.
2.6. Physical properties of powders
2.6.1. Yield.
The microencapsulation yield was calculated using eqn (7). | 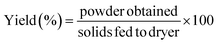 | (7) |
2.6.2. Moisture content and water activity (aw).
The moisture content was determined using a gravimetric method. About 2 g of each sample was placed in aluminium trays. Drying was performed in an oven at 105 °C for 24 h. For measuring the aw, a water activity meter (4TEV, Aqualab, USA) was used.
2.6.3. Colour.
Colour parameters (L*, a*, b*) were measured with a portable colorimeter (CR-400, Konica Minolta, Osaka, Japan) in the transmittance mode with a D65 standard illuminant, and a 2° standard observer. Hue and Chroma values were calculated using eqn (8) and (9), respectively. Hue values were corrected as proposed by Jiménez-González & Guerrero-Beltrán22. | 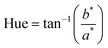 | (8) |
| 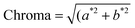 | (9) |
2.6.4. Antioxidant capacity and total phenolic compounds.
The antioxidant capacity and total phenolic compounds were measured following the methodology described previously. For the analysis, 0.02 g of each powder was weighed, dissolved in 20 mL ultrapure water (Milli-Q, Merck), and vigorously mixed with a vortex at high speed for 30 min.
2.6.5. Particle size.
The particle size distribution was measured with a Laser Diffraction Particle Size Analyser (Bluewave, MICROTRAC, USA).
2.7. Stability of pigments to heat and pH
The stability of the pigments was assessed by dissolving 0.03 g powder in 5 mL McIlvaine buffer at different pHs (2, 3, 4, 5, 6, and 7). Each solution was heated in a ramp mode from room temperature to 120 °C; once the solution reached the highest temperature, it was maintained for 20 min. Therefore, the temperature was gradually decreased until reaching 50 °C. Finally, all solutions were placed in an ice bath to stop the heat effect. The pigments stability was evaluated in terms of colour (L*, a*, b*), which was evaluated spectrophotometrically following the procedure proposed by APHA and Associations.23 Briefly, the whole visible spectrum (400–800 nm) was recorded in the transmittance mode at constant intervals of 1 nm using a microplate reader and water as a blank. Using 30 different wavelengths, the X, Y, and Z values were calculated using eqn (10). | 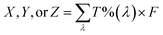 | (10) |
where T%(λ) is the transmittance value at an exact selected wavelength and F is the factor that corresponds to each coordinate: X = 0.03269, Y = 0.03333, and Z = 0.03938. The X, Y, and Z coordinates were transformed to the L*, a*, and b* values using eqn (11)–(13), respectively. | 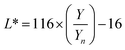 | (11) |
| 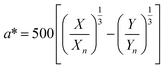 | (12) |
| 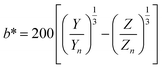 | (13) |
where Xn, Yn, and Zn are 98.041, 100.000, and 118.103, respectively, for a C illuminant, 2° observer, and X/Xn,Y/Yn and Z/Zn > 0.01.
2.8. Pigment application
2.8.1. Gummy bear preparation.
The gummy bear formulation consisted of glucose (120 g), water for gelatine hydration (150 mL), white sugar (550 g), sodium benzoate (1 g), starch (3 g), citric acid (10 g), gelatine of 290 bloom (55 g), and water (200 mL). Three percentages of pigments powders (1, 3, and 5%) were used. A control jelly (without colourant) was also prepared. The procedure was as follows: (i) the gelatine was hydrated, (ii) water, sodium benzoate, and starch were mixed, (iii) glucose and sugar were added to the previous mixture until complete dissolution, (iv) the mixture was heated until reaching 115 °C, (v) the temperature was reduced, (vi) hydrated gelatine, citric acid, and predissolved black sapote colourant were added, and (vii) mixed gently. Finally, the liquid was poured into moulds and wait for at least 48 h to unmould the jelly samples. The samples were stored at 4 °C in sealed polyethylene metallised bags to protect them from the light.
2.8.2. Colour determination of gummies.
Colour parameters (L*, a*, b*, Hue, Chroma) of the gummy bears were determined using a colorimeter, as described previously.
2.8.3. Sensory evaluation.
An incomplete balanced paired comparison design (IBPCD) was used for determining the type of pigment and colour intensity (based on the percentage of pigment added to the gummies). This type of analysis helps to evaluate the difference in a large number of samples to avoid fatigue of the judges.24 The IBPCD design was used to determine which gummy bear jelly the judge preferred the most in each pair. The Rank Scheffé25 model with 7 points was used (3 = I strongly prefer left to right, 2 = I moderately prefer left to right, 1 = I slightly prefer left to right, 0 = no preference, and −3, −2, −1 indicate the same as positive number but changing the order “right to left”). A total of 78 combinations were made, and 30 judges evaluated different combinations. Each sample was coded with a 3-digits number and randomly given to the judges. The judges consisted of untrained men and women with ages between 20 and 60 years from the Universidad de las Americas Puebla (UDLAP). Each judge was verbally informed about the test before the analysis. Only judges who gave affirmative responses were allowed to participate. Even though the test was only visual, judges were informed that “all products were safe for consumption” and that they could leave the test at any time without a reason. To each judge, one pair of samples was given and they were asked “Which sample do you prefer the most?” Additional questions were asked: “Do you relate sample colour with a healthy product?” and “Would you consider replacing the actual caramel colourant with natural pigments?”. For additional questions, the first question was answered using a 9-point scale: number 1 representing the answer “very unhealthy” and number 9 representing the answer “very healthy”. For the second question, the judges had to answer only yes or not.
2.9. Statistical analysis
The means of data of antioxidants and physical properties were evaluated using the Statistix 8.1 software (Statistix, Analytical Software, USA). The effect of each treatment was analysed by the ANOVA test. The difference between means of samples was compared using the Tukey test at α = 0.05. Sensory analysis results were analysed using the General Linear Model with the Minitab 16 software (Minitab Inc., USA). Plots were made using the KyPlot 6.0 software (Kyenslab Inc).
3 Results and discussion
3.1. Physical characteristics of powders
Table 1 shows the physical characteristics of the microencapsulated black sapote pigments.
Table 1 Physical properties of powders of fermented black sapote pulpa
Parameter |
Samples |
Without fermentation |
L. casei
|
L. rhamnosus
|
S. cerevisiae
|
Means in the same raw with different letters are significantly different (Tukey test, p < 0.05).
|
Yield (%) |
86.55 ± 1.97 a |
78.14 ± 1.65 bc |
72.87 ± 0.16 c |
82.69 ± 1.99 ab |
Moisture (%) |
4.79 ± 0.47 b |
9.04 ± 1.05 a |
8.07 ± 0.39 a |
8.61 ± 0.56 a |
a
w (25 °C) |
0.186 ± 0.008 a |
0.158 ± 0.008 b |
0.175 ± 0.006 ab |
0.190 ± 0.001 a |
L* |
67.18 ± 0.29 a |
62.34 ± 0.83 a |
68.50 ± 1.35 a |
61.87 ± 2.96 a |
a* |
4.6 ± 0.16 a |
1.24 ± 0.10 b |
−0.09 ± 0.14 c |
3.85 ± 0.37 a |
b* |
29.6 ± 0.39 a |
27.97 ± 0.13 b |
28.76 ± 0.10 ab |
29.26 ± 0.29 a |
Chroma |
29.95 ± 0.41 a |
28.01 ± 0.12 c |
28.76 ± 0.10 bc |
29.51 ± 0.24 ab |
Hue |
81.17 ± 0.18 c |
87.46 ± 0.21 b |
90.18 ± 0.28 a |
82.50 ± 0.78 c |
3.1.1. Yield.
The yield of powders was in the range 72.87–86.55%. It was observed that the lactic acid fermentation rendered lower yields of powders compared to the control (without fermentation) and yeast fermentation (p < 0.05). Several factors influenced the spray drying yield. Specifically, in the production of powder from fruit juices, the amount and type of sugars and other compounds, such as organic acids,26 may reduce the powder's yield, which is also influenced by the low molecular weight of the compounds and low glass transition temperature (Tg).27 The low Tg of these compounds may increase the stickiness; therefore, the powders could be attached to the chamber walls of the dryer.27L. rhamnosus and L. casei are heterofermentative species;28 therefore, the main metabolites product of their fermentation are organic acids, including lactic acid, among many other compounds.29S. cerevisiae may produce a variety of metabolites, including alcohol and CO2.30 Specifically, lactic acid has a low glass transition temperature (44–51 °C),31 which may cause particle adhesion to the drying chamber reducing the yield.
3.1.2. Moisture content.
The same reason could have affected the moisture content of the powders. The sticky state of a glassy material during the drying process might make the elimination of water difficult because of an increase in its internal cohesion.32 All powders obtained after fermentation had higher moisture content compared to the unfermented (control) powder (p < 0.05). Lechanteur & Evrard33 pointed out that compounds with a low Tg are highly hygroscopic. Likewise, the solids content of the extracts after fermentation, specifically with lactic acid bacteria, was high (4.69 ± 0.31 and 4.79 ± 0.02 for L. casei and L. rhamnosus, respectively) compared to those of the S. cerevisiae fermentation (4.23 ± 0.16) and the control (3.34 ± 0.23). This could be due to the hydrolysis of the fruit cell wall by the bacteria, which could release nonfermentable sugars or organic acids with high hygroscopicity.34
3.1.3. Water activity.
On the other hand, the water activity (aw) values were lower than 0.200, indicating chemical stability. The aw values showed statistical differences (p < 0.05) depending on the type of fermentation; therefore, as the encapsulation agent was the same at the same proportion; only the metabolites produced during fermentation could explain this difference. Some of these metabolites include organic acids and/or sugars. Walker and Stewart30 have indicated that the chemical structure of chemical compounds may present different water binding properties. For instance, the presence of lactic acid could attach water molecules and form strong bonds,35 leading to a reduction in free water and aw,36 although this depends on the concentration and type of compound.
3.1.4. Colour characteristics.
The presence of carotenoids, xanthophylls, chlorophyll, anthocyanins, and other phenolic compounds in the extract give a deep brown colour, characteristic of the fruit.13 The powder had a milky light brown colour due to the white or almost white colour of the encapsulation agents.37 The L* colour parameter shows values in the light zone of the colour space. Both the a* and b* colour parameters values were located in the first and second quadrants of the colour space; however, the values were close to 90°, which indicates yellow hue.22 Lower colour saturations (Chroma) values were observed for powders from the L. casei and L. rhamnosus fermentations. As previously mentioned, the presence of certain compounds may increase the attachment of powders to the drying chamber and cyclone, which could induce chemical reactions such as oxidation, cleavage, or hydrolysis due to temperature, resulting in a reduction of the pigments colour or even induce the formation of other compounds.38–40
3.1.5. Particle size.
Concerning the particle sizes of the powders, values were in the range of 0.75–352 μm for the powder without fermentation (Fig. 1a). For powders from fermentation, the particle sizes were in the range of 1.06–15.56 μm for L. casei (Fig. 1b), 0.892–13.08 μm for L rhamnosus (Fig. 1c), and 0.75–12.0 μm for S. cerevisiae (Fig. 1d). Small particles were observed in the control powders (without fermentation) and powders from fermentation with S. cerevisiae. However, the presence of a great number of small particles could induce agglomerations by physical forces41 after drying; for example, in the unfermented sample. A possible reason for nonagglomerated particles in powders from S. cerevisiae fermentation could be the breakdown of larger chemical compounds due to fermentation, such as some sugars or compounds of the cell wall. On the other hand, during drying, bridges between particles could be formed due to collisions when particles are not completely dry or when they reach their sticky state due to low Tg,42 producing larger particles and reducing the yields, for example, in powders from the lactic acid fermentation.
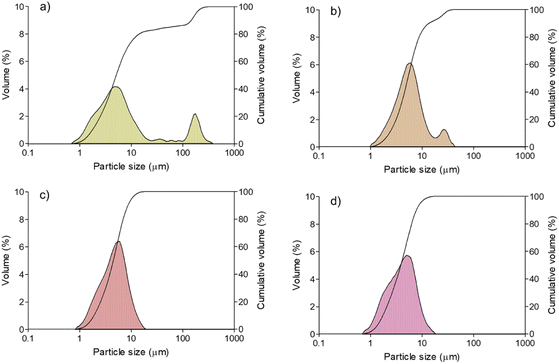 |
| Fig. 1 Particle size distribution of spray dried microcapsules of black sapote pulp: (a) unfermented and fermented with (b) L. casei, (c) L. rhamnosus, and (d) S. cerevisiae. | |
3.2. Antioxidant characteristics
The antioxidant capacity and total phenolic compounds results are shown in Fig. 2. To observe the response of different methods of analysis of antioxidant compounds in the extract, different antioxidant capacity assays were conducted. As mentioned by other authors, DPPH, ABTS, and FRAP may provide comparable results for the antioxidant capacity.43
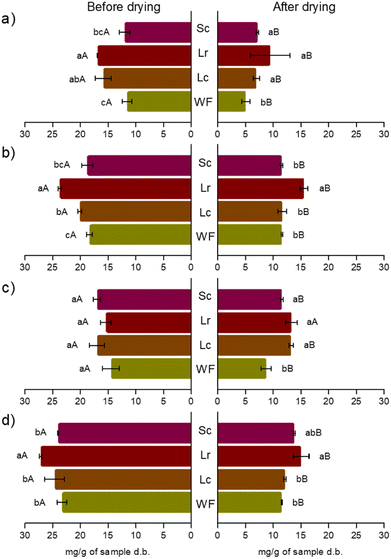 |
| Fig. 2 Antioxidant capacity: (a) DPPH (mg TE per g db), (b) FRAP (mg Fe(II) per g db), (c) ABTS (mg TE per g db), and (d) total phenolic compounds (mg GAE per g db) content of fermented black sapote pulp before (left) and after (right) spray drying. WF was the control without fermentation. Lc, Lr, and Sc correspond to the fermented samples with L. casei, L. rhamnosus, and S. cerevisiae, respectively. Different lowercase letters at the same moment (before or after drying, separately) indicate statistical differences (p < 0.05) between samples for the same antioxidant capacity test or total phenolic compounds. Different capital letters between the same sample for the same antioxidant capacity test or TPC indicate statistical differences (p < 0.05) between before and after drying. | |
3.2.1. Extracts from fermented purées.
3.2.1.1 Antioxidant capacity.
The antioxidant capacity of the extracts from fermented purées, performed with L. rhamnosus was significantly higher (p < 0.05) than the AC of the control and the S. cerevisiae extracts analysed with DPPH and FRAP assays; however, they were similar to the values of AC of the extracts fermented with L. casei. It has been reported that the lactic acid bacteria could transform glycosides to aglycones with higher biological activity, which may help to release covalent phenolics attached to the cell wall.44 On the other hand, no significant differences (p > 0.05) were observed in the antioxidant capacity of extracts analysed with the ABTS method. Different factors could affect the determination of AC in food matrixes. It was already mentioned that the reproducibility of the ABTS method is affected by the ABTS˙ radical solution because it requires a period to the radical formation.45 It is also sensitive to certain antioxidants present in the food matrix, depending on its chemical diversity;46 specifically, the ABTS assay is sensitive to hydrophilic antioxidants.47 Total phenolic compounds. A similar effect was observed for TPC. Extracts from the L. rhamnosus fermentation showed the highest TPC values compared to the other treatments. The values of TPC were in the range from 23.33 ± 0.84 to 27.20 ± 0.22 mg GAE per g of the sample in db. An increase in the total phenolic compounds after fermentation was observed in extracts of myrtle (Myrtus communis) berries fermented with L. plantarum; the TPC content was five times higher than that of the control.48 The increase in TPC and AC has also been observed in papaya and pineapple (both tropical fruits) products after lactic fermentation.49
3.2.2. Powders.
3.2.2.1 Antioxidant capacity.
In general, the drying process decreased the AC once the different extracts passed through the spray drying equipment. The effect of temperature on the AC tended to decrease, which has been observed by other authors.50,51 However, the compounds quantified and unquantified produced or released by fermentation with L. rhamnosus remained higher compared to the other treatments (Fig. 2), specially for the evaluation of AC with FRAP. The FRAP method is based on the ability of compounds to reduce ferric ions and measure the antioxidant species, not the ability to inhibit free radicals.52 On the other hand, the DPPH and ABTS assays indicate that the fermented samples retained better antioxidant compounds and increase bioavailability53 and stability. However, further research is needed to determine the effect of fermentation on the chemical compounds responsible for antioxidant capacity and how these modifications/interactions increase their stability. Total phenolic compounds. Similar behaviour to the antioxidant capacity was observed for TPC in the powders. This might indicate that the TPC, produced by fermentation as products of the normal metabolism of microorganisms and/or the release from the cell walls of the vegetable material (among other unquantified compounds) content, could be the main reason for the antioxidant capacity (free radical scavenging and ferric reducing capacity) observed in the powders.
3.3. Colour stability of reconstituted powders
3.3.1. Reconstituted powders.
The colour of the dissolved powders was brown at the naked eye for solutions of all pHs and all treatments: without fermentation (WF), L. casei fermentation (Lc), L. rhamnosus fermentation (Lr), and S. cerevisiae fermentation (Sc). However, the colour parameter values were slightly different, depending on the pH from where the powder came from for making the solution. In general, the colour values were in the ranges 66.77–84.84 for L*, −6.21–7.98 for a*, and 39.41–67.71 for b* (Table 2). The WF and Sc solutions were browner than the other solutions, with values found in the first quadrant of the colour space.
Table 2 Colour stability of fermented and dried sapote pigments at different pH and heat treatments (120 °C) for 1 houra
Sample |
pH |
Colour parameters |
Recently dispersed at 22 ± 1 °C |
After 120 °C treatment |
L* |
a* |
b* |
L* |
a* |
b* |
Different lowercase letters in the same column for the same treatment (WF, Lc, Lr, Sc, correspond to the unfermented and fermented samples with L. casei, L. rhamnosus, and S. cerevisiae, respectively) indicate significant differences according to Tukey's test (p < 0.05). Different capital letters in the same row for the same colour parameter (L*, a*, b*) between just dispersed and after heat treatment denote significant differences according to Tukey's test (p < 0.05).
|
WF |
2.00 |
73.79 ± 0.34 aB |
2.47 ± 0.29 dA |
58.36 ± 0.69 dA |
88.46 ± 0.10 aA |
−2.04 ± 0.02 dB |
18.31 ± 0.27 cB |
|
3.00 |
66.77 ± 0.07 fA |
7.98 ± 0.10 aA |
67.71 ± 0.22 aA |
64.38 ± 0.10 eB |
3.53 ± 0.01 aB |
56.00 ± 0.09 aB |
|
4.00 |
73.07 ± 0.14 bA |
4.40 ± 0.05 cA |
59.89 ± 0.24 cA |
65.58 ± 0.23 dB |
3.33 ± 0.15 aB |
56.79 ± 0.21 aB |
|
5.00 |
71.67 ± 0.02 cA |
6.56 ± 0.14 bA |
63.40 ± 0.33 bA |
68.56 ± 0.12 cB |
2.09 ± 0.01 bB |
56.44 ± 0.08 aB |
|
6.00 |
70.97 ± 0.14 dA |
7.42 ± 0.14 aA |
63.73 ± 0.23 bA |
70.17 ± 0.07 bB |
1.15 ± 0.04 cB |
53.54 ± 0.06 bB |
|
7.00 |
69.73 ± 0.01 eA |
7.87 ± 0.10 aA |
63.31 ± 0.16 bA |
68.81 ± 0.45 cB |
1.68 ± 0.27 bB |
53.51 ± 0.43 bB |
Lc |
2.00 |
84.71 ± 0.68 aA |
−5.69 ± 0.01 eB |
39.41 ± 0.96 cA |
75.11 ± 0.19 cB |
−0.11 ± 0.04 aA |
37.3 ± 0.26 dB |
|
3.00 |
84.50 ± 0.07 aA |
−5.19 ± 0.02 dB |
40.43 ± 0.17 bcB |
72.89 ± 0.25 eB |
−1.11 ± 0.10 bA |
49.74 ± 0.25 abA |
|
4.00 |
83.69 ± 0.08 abA |
−4.37 ± 0.03 cB |
41.85 ± 0.1 abcB |
76.72 ± 0.34 abB |
−3.58 ± 0.09 eA |
50.25 ± 0.37 aA |
|
5.00 |
82.61 ± 0.26 bA |
−2.34 ± 0.01 bA |
40.69 ± 0.36 bcB |
77.50 ± 0.22 aB |
−3.34 ± 0.15 deB |
48.67 ± 0.54 bcA |
|
6.00 |
80.57 ± 0.55 cA |
−0.63 ± 0.23 aA |
42.94 ± 1.15 abB |
76.51 ± 0.06 bB |
−3.15 ± 0.02 dB |
47.96 ± 0.04 cA |
|
7.00 |
80.27 ± 0.01 cA |
−0.94 ± 0.01 aA |
43.37 ± 0.10 aB |
74.21 ± 0.16 dB |
−1.65 ± 0.11 cB |
49.45 ± 0.39 abA |
Lr |
2.00 |
84.19 ± 0.43 aA |
−6.21 ± 0.03 fB |
42.52 ± 0.43 bA |
83.13 ± 0.20 aB |
−2.47 ± 0.03 bA |
26.86 ± 0.30 cB |
|
3.00 |
84.84 ± 0.03 aA |
−5.82 ± 0.08 eB |
42.28 ± 0.19 bB |
77.19 ± 0.40 bB |
−3.39 ± 0.11 cA |
45.76 ± 0.67 dA |
|
4.00 |
84.11 ± 0.51 aA |
−5.27 ± 0.11 dB |
43.43 ± 0.18 bB |
77.82 ± 0.10 bB |
−4.16 ± 0.05 dA |
49.39 ± 0.44 aA |
|
5.00 |
82.39 ± 0.07 bA |
−3.29 ± 0.01 cA |
44.82 ± 0.14 aB |
77.82 ± 0.15 bB |
−3.46 ± 0.06 cB |
49.35 ± 0.33 aA |
|
6.00 |
80.36 ± 0.26 cA |
−1.04 ± 0.09 bA |
45.81 ± 0.52 aB |
76.32 ± 0.06 bB |
−3.33 ± 0.02 cB |
49.23 ± 0.21 aA |
|
7.00 |
79.88 ± 0.13 cA |
−0.72 ± 0.02 aA |
45.75 ± 0.24 aB |
73.99 ± 0.86 cB |
−1.37 ± 0.36 aB |
50.48 ± 1.07 aA |
Sc |
2.00 |
69.50 ± 0.06 bcB |
4.24 ± 0.03 cA |
61.54 ± 0.40 aA |
88.57 ± 0.04 aA |
−2.18 ± 0.01 eB |
19.05 ± 0.01 dB |
|
3.00 |
73.32 ± 0.65 aB |
2.33 ± 0.37 dA |
57.04 ± 1.02 bA |
75.77 ± 0.28 bA |
−1.16 ± 0.08 dB |
43.88 ± 0.31 cB |
|
4.00 |
70.81 ± 0.50 bA |
4.46 ± 0.36 bcA |
61.79 ± 0.65 aA |
67.45 ± 0.02 eB |
2.03 ± 0.03 aB |
56.98 ± 0.32 aB |
|
5.00 |
70.17 ± 0.01 bB |
5.52 ± 0.07 bA |
62.46 ± 0.11 aA |
71.07 ± 0.67 cA |
0.39 ± 0.30 cB |
55.03 ± 0.75 bB |
|
6.00 |
68.03 ± 0.26 cdB |
7.26 ± 0.19 aA |
63.85 ± 0.31 aA |
69.51 ± 0.36 cdA |
1.10 ± 0.11 bB |
54.77 ± 0.30 bB |
|
7.00 |
67.08 ± 0.49 dA |
7.58 ± 0.46 aA |
63.01 ± 0.58 aA |
68.04 ± 0.57 deA |
1.43 ± 0.27 abB |
53.81 ± 0.50 bB |
The a* and b* colour values increased as the pH increased. Similar changes were observed for the Lr and Lc solutions. However, the colour values of the lactic acid bacteria solutions were greenish, corresponding to negative a* values (found in the second quadrant of the colour space). This could be due to the change in the green of chlorophylls to the green-brownish of pheophytins' olive green colour.54 In addition, the colour saturation (Chroma) of the dispersed pigments was low, as indicated by the a* colour parameter. On the other hand, the L* colour values decreased slightly as the pH increased. For both Lc and Lr solutions from powders of pH in the range of 3–7, the a* and b* values increased.
3.3.2. Remarks.
After the heat treatment of the reconstituted solutions for all pHs, the colour observed by the naked eye remained the same as before heating (brown). However, the L*, a*, and b* values of all solutions changed for all pH values (p < 0.05). In case of solutions from L. casei and L. rhamnosus fermentation, the a* values at pH 2, 3, and 4 increased; however, these values were still negative.
In general, the three colour coordinates (L*, a*, b*) of the solutions after heat treatment decreased. It is well known that heat may induce changes in the molecular structures of many pigments, which could result in changes or losses of colour. However, in this study, all pigments showed high stability over a wide range of pH; this may indicate that the pigments from black sapote could be used in different foods to replace the caramel colourant commonly used in brown coloured foods. The pigments from black sapote could have a positive impact on the human health and the environment. Also, the interest in consuming black sapote might increase.13
3.4. Black sapote pigments added to gummy bear formulations
3.4.1. Colour.
A gummy bear model formulation was selected to evaluate the black sapote pigments added as the colourant. The food preparation required heat treatment and acidity conditions due to citric acid; both conditions could affect the colour of the formulation. The pH of the gummy bears was in the range of 3.38–3.43; this pH range was evaluated in the stability test. The gummy formulations presented good stability to the heat treatment.
Fig. 3 illustrate the colour of gummy bears added with WF, Lc, Lr, and Sc pigment powders (1, 3, and 5%) and without the addition of pigments (control).
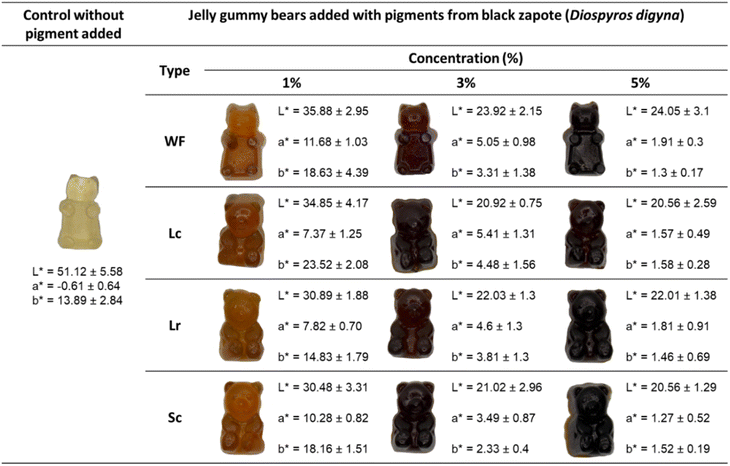 |
| Fig. 3 Gummy bears without pigment (control) and with pigments from black sapote pulp: without fermentation (WF) and after fermentation with L. casei (Lc), L. rhamnosus (Lr), and S. cerevisiae (Sc) at distinct levels (1, 3, 5%) of pigment. | |
The control gummy bears presented a green-sandy translucid colour. Once the powder was added to the gummy bear formulation, the colour of the food darkened (L* value decreased). The a* and b* colour values were different compared to the control. The final colour of the food differed, depending on the quantity of powder added. Increasing the amount of powder (pigment) decreased the lightness (L*), redness (a*), and yellowness (b*) of the gummy food. The pigment increased the tone (Hue) of the gummies (Fig. 4) by approximately 35°. The initial hue values were 59.10 ± 8.51°, 73.24 ± 2.79°, 62.10 ± 1.51°, and 58.44 ± 4.40° for WF, Lc, Lr, and Sc, respectively. Saturation (Chroma) was higher in the gummy bears with low pigments content (Fig. 4).
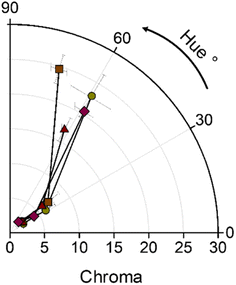 |
| Fig. 4 Hue and Chroma parameters for ●WF, ■Lc, ▲Lr, ♦Sc at three levels of pigment addition in gummy bears. | |
The colour observed in gummy bears can be described to be similar to the colour of maple syrup for 1% of powder and similar to coke beverages for 3% of powder. On the other hand, based on the stability analysis (Table 2), the colour gummy bears (Fig. 3) may persist during processing. If the intention of adding pigments to the food is to increase the antioxidant capacity, previous fermentation can be performed before obtaining the powder to be added to the food (Fig. 2). Other studies have demonstrated the use of different pigments in gummy foods (candy)7,55–58 but none has focused on obtaining brown or caramel-coloured pigments.
3.4.2. Sensory.
The preference test was used only to evaluate visual appearance. The judges were asked only to observe the gummies and give their opinions. The ANOVA test revealed no significant differences (p < 0.05) in preference among the gummy bears (type and amount of pigment added). The results indicated the good acceptability for all the samples. The amount of pigment added did not affect the gummy bear preferences; therefore, the pigment could be added to different food systems.
Regarding the questions made to judges about the relationship between colour and healthfulness, no significant differences (p < 0.05) were observed due to the large quantity of data that is common when untrained judges are used in sensory analysis.59
Fig. 5 illustrates the sensory scores for all gummy bear formulations. The question was to answer over a 9-point scale, where 1 was “equal to unhealthy” and 9 was “equivalent to extremely healthy”. Most of the judges evaluated the colour of the gummies at about 5 points, which indicates “neither healthy nor unhealthy”. The gummies containing low amounts of the sapote pigments were evaluated by judges as healthier (according to the median of the obtained data) than those containing high amounts of pigments, except for the gummies containing 3% of Sc powder, in which most of the answers were below 5. Finally, approximately 95% of the judges, once they knew that the pigments came from natural sources, showed increased interest and accepted changing artificial colourants for natural pigments.
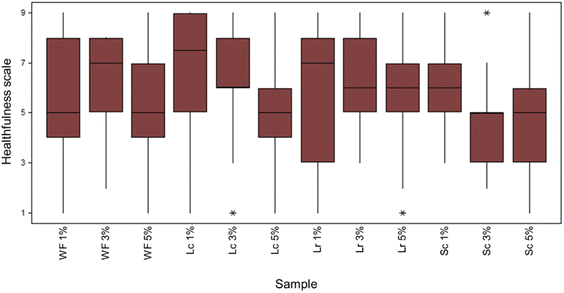 |
| Fig. 5 Boxplot for the relationship between the colour and healthiness of gummy bears coloured with pigments of black sapote. Control or unfermented (WF), and/or fermented with L. casei (Lc), L. rhamnosus (Lr), and S. cerevisiae (Sc) at different pigments levels (1, 3, and 5%). | |
4 Conclusion
The present work shows that the use of black sapote pulp as a natural colorant in food products is a promising alternative to synthetic colorants obtained from sugar. The fermentation process, especially with Lactobacillus rhamnosus, was done to increase the content of bioactive compounds, which may have potential health benefits. Despite the encapsulation process decreasing the antioxidant capacity, the stability test showed that the encapsulated pigments under different pH and thermal conditions can be used in a wide range of food applications. The addition of pigments to the gummy bear formulations resulted in different hues and did not affect the overall preference of the judges. Once the judges were informed that the colorant was natural, it was better accepted. Further studies should be conducted to evaluate the potential impact of fermentation on the flavour of the final product. Overall, black sapote pigments offer a natural and healthy alternative to synthetic colorants in food products.
Ethical statement
This manuscript does not contain any studies with human participants or animals performed by any of the authors. Ethics approval or patient consent was not required for this study.
Author contributions
All authors contributed to design and plan the experiments, to make the technical part (measurements, procedure, and analysis), and draft the manuscript including the design of figures and tables. All authors discussed the results and wrote the manuscript. Jiménez-González, Oscar: conceptualization, data curation, formal analysis, methodology, writing original draft, review and editing; González-Perez, Julio: data curation, formal analysis, and methodology; Mejía-Garibay, Beatriz: conceptualization, and methodology; Lopez-Malo, Aurelio: conceptualization, and methodology, and Guerrero-Beltrán, José Angel: supervision, conceptualization, methodology, writing original draft, review and editing.
Conflicts of interest
The authors declared no potential conflicts of interest with respect to the research, authorship, and/or publication of this article.
Acknowledgements
Authors Jiménez-González and González-Perez thank Universidad de las Americas Puebla (UDLAP) and the National Council for Science and Technology (CONACyT) of Mexico for the financial support for their PhD studies.
References
- L. L. Garber, E. M. Hyatt and L. Nafees, The effects of analogous food color on perceived flavor: A factorial investigation, J. Food Prod. Mark., 2016, 22, 486–500 CrossRef.
- S. Sukkwai, K. Kijroongrojana, P. Chonpracha, K. D. Pujols, J. R. Alonso-Marenco and R. Ardoin,
et al.
, Effects of colorant concentration and ‘natural colour’ or ‘sodium content’ claim on saltiness perception, consumer liking and emotion, and purchase intent of dipping sauces, Int. J. Food Sci. Technol., 2018, 53, 1246–1254 CrossRef CAS.
- S. Lehto, M. Buchweitz, A. Klimm, R. Straßburger, C. Bechtold and F. Ulberth, Comparison of food colour regulations in the EU and the US: a review of current provisions, Food Addit. Contam.: Part A, 2017, 34, 335–355 CrossRef CAS PubMed.
- N. Martins, C. L. Roriz, P. Morales, L. Barros and I. C. F. R. Ferreira, Food colorants: Challenges, opportunities and current desires of agro-industries to ensure consumer expectations and regulatory practices, Trends Food Sci. Technol., 2016, 52, 1–15 CrossRef CAS.
- M. J. Scotter, Emerging and persistent issues with artificial food colours: natural colour additives as alternatives to synthetic colours in food and drink, Qual. Assur. Saf. Crops Foods, 2011, 3, 28–39 CrossRef CAS.
- P. Burey, B. R. Bhandari, R. P. G. Rutgers, P. J. Halley and P. J. Torley, Confectionery gels: A Review on formulation, rheological and structural aspects, Int. J. Food Prop., 2009, 12, 176–210 CrossRef CAS.
- E. N. Dewi, R. A. Kurniasih and L. Purnamayati, The application of microencapsulated phycocyanin as a blue natural colorant to the quality of jelly candy, IOP Conf. Ser.: Earth Environ. Sci., 2018, 116, 012047 CrossRef.
-
T. Krahl, H. Fuhrmann and S. Dimassi, Ice Cream, in Handbook on Natural Pigments in Food and Beverages, Elsevier, 2016, pp. 197–207 Search PubMed.
-
M. Ramesh and A. Muthuraman, Flavoring and Coloring Agents: Health Risks and Potential Problems, in Natural and Artificial Flavoring Agents and Food Dyes, Elsevier, 2018, pp. 1–28 Search PubMed.
-
E. Stich, Food Color and Coloring Food, in Handbook on Natural Pigments in Food and Beverages, Elsevier, 2016, pp. 3–27 Search PubMed.
- T. A. Vollmuth, Caramel color safety – An update, Food Chem. Toxicol., 2018, 111, 578–596 CrossRef CAS PubMed.
-
K. Brimmer, Replacement Strategies for Caramel Coloring Using Natural Ingredients, Sensient Food Colors, 2017, https://sensientfoodcolors.com/en-us/dry-grocery/replacement-strategies-caramel-coloring/, accessed 18 Oct2022 Search PubMed.
- O. Jiménez-González and J. Á. Guerrero-Beltrán,
Diospyros digyna (black sapote), an Undervalued Fruit: A Review, ACS Food Sci. Technol., 2021, 1, 3–11 CrossRef.
-
E. M. Yahia, J. De Jesus Ornelas-Paz and G. A. Gonzalez-Aguilar, Nutritional and health-promoting properties of tropical and subtropical fruits, in Postharvest Biology and Technology of Tropical and Subtropical Fruits, Elsevier, 2011, pp. 21–78 Search PubMed.
- E. M. Yahia, F. Gutierrez-Orozco and C. A. Leon, Phytochemical and antioxidant characterization of the fruit of black sapote (Diospyros digyna Jacq.), Food Res. Int., 2011, 44, 2210–2216 CrossRef CAS.
- G. Mannino, G. Serio, C. M. Bertea, R. Chiarelli, A. Lauria and C. Gentile, Phytochemical profile and antioxidant properties of the edible and non-edible portions of black sapote (Diospyros digyna Jacq.), Food Chem., 2022, 380, 132137 CrossRef CAS PubMed.
- L. A. Arellano-Gómez, C. Saucedo-Veloz and M. L. Arévalo-Galarza, Cambios bioquímicos y fisiológicos durante la maduración de frutos de zapote negro (Diospyros digyna jacq.), Agrociencia, 2005, 39, 173–181 Search PubMed.
- O. Jiménez-González and J. Á. Guerrero-Beltrán, Microencapsulates by spray of Lacticaseibacillus rhamnosus GG from fermented whole or skimmed cow's milk added with Mexican honeysuckle (Justicia spicigera) extract using mesquite gum as carrier agent, Heliyon, 2022, 8, e10733 CrossRef PubMed.
- R. Gómez-García, A. A. Vilas-Boas, M. Machado, D. A. Campos, C. N. Aguilar and A. R. Madureira,
et al.
, Impact
of simulated in vitro gastrointestinal digestion on bioactive compounds, bioactivity and cytotoxicity of melon (Cucumis melo L. inodorus) peel juice powder, Food Biosci., 2022, 47, 101726 CrossRef.
- A. A. S. Bolanos de la Torre, T. Henderson, P. S. Nigam and R. K. Owusu-Apenten, A universally calibrated microplate ferric reducing antioxidant power (FRAP) assay for foods and applications to Manuka honey, Food Chem., 2015, 174, 119–123 CrossRef CAS PubMed.
- I. Ahmad, A. Yanuar, K. Mulia and A. Mun'im, Optimization of ionic liquid-based microwave-assisted extraction of polyphenolic content from Peperomia pellucida (L) kunth using response surface methodology, Asian Pac. J. Trop. Biomed., 2017, 7, 660–665 CrossRef.
- O. Jiménez-González and J. Á. Guerrero-Beltrán, Extraction, microencapsulation, color properties, and experimental design of natural pigments obtained by spray drying, Food Eng. Rev., 2021, 13, 769–811 CrossRef.
-
APHA (American Public Health Association), AWWA (American Water Works Association) and WEF (Water Environment Federation), 2120 COLOR, in Standard Methods for the Examination of Water and Wastewater, ed. Franson M. A. H., American Public Health Association, Washington, D.C., 1992, DOI:10.2105/SMWW.2882.017.
-
M. C. Gacula and J. Singh, Statistical Methods in Food and Consumer Research, Academic Press, 1984, DOI:10.1016/C2009-0-02978-X.
- H. Scheffé, An Analysis of Variance for Paired Comparisons, J. Am. Stat. Assoc., 1952, 47, 381–400 Search PubMed.
- B. R. Bhandari, A. Senoussi, E. D. Dumoulin and A. Lebert, Spray drying of concentrated fruit juices, Drying Technol., 1993, 11, 1081–1092 CrossRef CAS.
- L. Zhang, X. Zeng, N. Fu, X. Tang, Y. Sun and L. Lin, Maltodextrin: A consummate carrier for spray-drying of xylooligosaccharides, Food Res. Int., 2018, 106, 383–393 CrossRef CAS PubMed.
- D. Hill, I. Sugrue, C. Tobin, C. Hill, C. Stanton and R. P. Ross, The Lactobacillus casei Group: History and Health Related Applications, Front. Microbiol., 2018, 9, 2107 CrossRef PubMed.
- S.-J. Lee, H.-S. Jeon, J.-Y. Yoo and J.-H. Kim, Some Important Metabolites Produced by Lactic Acid Bacteria Originated from Kimchi, Foods, 2021, 10, 2148 CrossRef CAS PubMed.
- G. Walker and G. Stewart, Saccharomyces cerevisiae in the Production of Fermented Beverages, Beverages, 2016, 2, 30 CrossRef.
- R. Zhang, F. Du, K. Jariyavidyanont, E. Zhuravlev, C. Schick and R. Androsch, Glass transition temperature of poly(d,l-lactic acid) of different molar mass, Thermochim. Acta, 2022, 718, 179387 CrossRef CAS.
- J. J. Fitzpatrick, M. Hodnett, M. Twomey, P. S. M. Cerqueira, J. O'Flynn and Y. H. Roos, Glass transition and the flowability and caking of powders containing amorphous lactose, Powder Technol., 2007, 178, 119–128 CrossRef CAS.
- A. Lechanteur and B. Evrard, Influence of Composition and Spray-Drying Process Parameters on Carrier-Free DPI Properties and Behaviors in the Lung: A review, Pharmaceutics, 2020, 12, 55 CrossRef CAS PubMed.
-
G. Ramis-Ramos, Antioxidants|Synthetic Antioxidants, in Encyclopedia of Food Sciences and Nutrition, Elsevier, 2003, pp. 265–275 Search PubMed.
- R. Wijayasinghe, T. Vasiljevic and J. Chandrapala, Water-lactose behavior as a function of concentration and presence of lactic acid in lactose model systems, J. Dairy Sci., 2015, 98, 8505–8514 CrossRef CAS PubMed.
- C. E. Velezmoro and A. J. A. Meirelles, Water Activity In Solutions Containing Organic Acids, Drying Technol., 1998, 16, 1789–1805 CrossRef CAS.
- Z. Xiao, J. Xia, Q. Zhao, Y. Niu and D. Zhao, Maltodextrin as wall material for microcapsules: A review, Carbohydr. Polym., 2022, 298, 120113 CrossRef CAS PubMed.
- S. Ghosh, T. Sarkar, A. Das and R. Chakraborty, Natural colorants from plant pigments and their encapsulation: An emerging window for the food industry, LWT, 2022, 153, 112527 CrossRef CAS.
- D. B. Rodriguez-Amaya, Update on natural food pigments – A mini-review on carotenoids, anthocyanins, and betalains, Food Res. Int., 2019, 124, 200–205 CrossRef CAS PubMed.
- D. B. Rodriguez-Amaya, Natural food pigments and colorants, Curr. Opin. Food Sci., 2016, 7, 20–26 CrossRef.
- I. Zbicinski, K. Ciesielski and B. Ge, Mechanism of particle agglomeration for single and multi-nozzle atomization in spray drying: A review, Processes, 2022, 10, 727 CrossRef.
- A. Gianfrancesco, C. Turchiuli and E. Dumoulin, Powder agglomeration during the spray-drying process: measurements of air properties, Dairy Sci. Technol., 2008, 88, 53–64 CrossRef.
- S. Dudonné, X. Vitrac, P. Coutière, M. Woillez and J.-M. Mérillon, Comparative Study of Antioxidant Properties and Total Phenolic Content of 30 Plant Extracts of Industrial Interest Using DPPH, ABTS, FRAP, SOD, and ORAC Assays, J. Agric. Food Chem., 2009, 57, 1768–1774 CrossRef PubMed.
- P. Filannino, R. Di Cagno and M. Gobbetti, Metabolic and functional paths of lactic acid bacteria in plant foods: get out of the labyrinth, Curr. Opin. Biotechnol., 2018, 49, 64–72 CrossRef CAS PubMed.
- K. Thaipong, U. Boonprakob, K. Crosby, L. Cisneros-Zevallos and D. Hawkins Byrne, Comparison of ABTS, DPPH, FRAP, and ORAC assays for estimating antioxidant activity from guava fruit extracts, J. Food Compos. Anal., 2006, 19, 669–675 CrossRef CAS.
- F. Xiao, T. Xu, B. Lu and R. Liu, Guidelines for antioxidant assays for food components, Food Front., 2020, 1, 60–69 CrossRef.
- A. Floegel, D.-O. Kim, S.-J. Chung, S. I. Koo and O. K. Chun, Comparison of ABTS/DPPH assays to measure antioxidant capacity in popular antioxidant-rich US foods, J. Food Compos. Anal., 2011, 24, 1043–1048 CrossRef CAS.
- J. A. Curiel, D. Pinto, B. Marzani, P. Filannino, G. A. Farris and M. Gobbetti,
et al.
, Lactic acid fermentation as a tool to enhance the antioxidant properties of Myrtus communis berries, Microb. Cell Fact., 2015, 14, 67 CrossRef PubMed.
- A. Fessard, A. Kapoor, J. Patche, S. Assemat, M. Hoarau and E. Bourdon,
et al.
, Lactic fermentation as an efficient tool to enhance the antioxidant activity of tropical fruit juices and teas, Microorganisms, 2017, 5, 23 CrossRef PubMed.
- K. Bigetti Guergoletto, K. Landucci Bonifácio, D. Sabbatini Barbosa, D. Farinha Valezi, A. Salviato and E. Di Mauro,
et al.
, Influence of spray-drying and room temperature storage on the anti- and prooxidant properties of fermented Juçara Pulp, Food Technol. Biotechnol., 2020, 58, 29–37 Search PubMed.
- N. M. Lakshmi, K. Umashankar, A. Chandralekha, K. Raghavarao, N. M. Jayan and C. Salini,
et al.
, Spray drying as effective encapsulation method for phenol degrading bacteria, Indian J. Exp. Biol., 2017, 55, 8 Search PubMed.
-
S. C. Litescu, S. Eremia and G. L. Radu, Methods for the Determination of Antioxidant Capacity in Food and Raw Materials, in Bio-Farms for Nutraceuticals, ed. Giardi M. T., Rea G. and Berra B., Springer US, Boston, MA, 2010, pp. 241–249 Search PubMed.
- O. A. Adebo and I. Gabriela Medina-Meza, Impact of Fermentation on the Phenolic Compounds and Antioxidant Activity of Whole Cereal Grains: A Mini Review, Molecules, 2020, 25, 927 CrossRef CAS PubMed.
- A. Andrés-Bello, V. Barreto-Palacios, P. García-Segovia, J. Mir-Bel and J. Martínez-Monzó, Effect of pH on Color and Texture of Food Products, Food Eng. Rev., 2013, 5, 158–170 CrossRef.
- S. Akhavan Mahdavi, S. M. Jafari, E. Assadpour and M. Ghorbani, Storage stability of encapsulated barberry's anthocyanin and its application in jelly formulation, J. Food Eng., 2016, 181, 59–66 CrossRef CAS.
- J. M. Obón, M. R. Castellar, M. Alacid and J. A. Fernández-López, Production of a red–purple food colorant from Opuntia stricta fruits by spray drying and its application in food model systems, J. Food Eng., 2009, 90, 471–479 CrossRef.
- M. C. Otálora, H. de Jesús Barbosa, J. E. Perilla, C. Osorio and M. A. Nazareno, Encapsulated betalains (Opuntia ficus-indica) as natural colorants. Case study: Gummy candies, LWT, 2019, 103, 222–227 CrossRef.
- J. A. Rodríguez-Sánchez, M. T. Cruz y Victoria and B. E. Barragán-Huerta, Betaxanthins and antioxidant capacity in Stenocereus pruinosus: Stability and use in food, Food Res. Int., 2017, 91, 63–71 CrossRef PubMed.
- V. Losó, A. Gere, A. Györey, Z. Kókai and L. Sipos, Comparison of the performance of a trained and an untrained sensory panel on sweetcorn varieties with the panelcheck software, Appl. Stud. Agribus. Commer., 2012, 6, 77–83 CrossRef.
|
This journal is © The Royal Society of Chemistry 2023 |