Effect of a magnetic field/electrostatic field on the quality attributes of green bell peppers during cool chain transportation
Received
6th May 2023
, Accepted 13th June 2023
First published on 13th June 2023
Abstract
Electric field and magnetic field preservation technologies are pollution-free, safe, and healthy physical means that have been widely used in recent years. The aim of this study is to evaluate the effects of different intensities of electrostatic fields and static magnetic fields on the quality of cold chain transportation of green bell peppers. During the cold storage period of green bell peppers, different intensities of an electrostatic field (3 kV cm−1 and 5 kV cm−1) and magnetic field (2 mT, 5 mT, and 8 mT) were applied, and quality testing was conducted at 0, 3, 6, 9, 12, and 15 days of cool storage. The quality of green bell peppers based on physical and chemical indicators was evaluated and was compared with that of the blank group. The results indicated that the application of electrostatic and magnetic fields is beneficial for improving the quality of green bell peppers during refrigeration. Based on various indicators, the optimal treatment conditions for this study are 4 °C and 5 kV cm−1, which can effectively improve the quality and nutrition of cold chain transportation of green peppers.
Sustainability spotlight
This study used a combination of a static magnetic field and electrostatic field combined with refrigeration treatment to preserve the freshness and nutrition of fresh green bell peppers. The processing method used is safe, non-toxic, and environmentally friendly, and can effectively improve the nutritional content of the refrigerated green bell peppers. The equipment used has a lower cost and is safe and reliable. Therefore, this study is consistent with the United Nations' sustainable development goals of food safety and improving nutritional status.
|
1. Introduction
Peppers are rich in vitamin C, carotenoids and other nutrients,1 which are widely welcomed by consumers at home and abroad and play a role in supplementing nutrition.2 Among them, green bell peppers are deeply loved by consumers because of their light green color, fat meat and light spicy taste.3 However, fruit and vegetable products are prone to quality deterioration due to temperature fluctuations during transportation.4 Generally, perishable food is stored and transported by freezing, but it easily causes high dripping loss.5,6 At present, fruits and vegetables are mostly transported by cold chain to ensure product quality. However, the development of cold chain transportation in China is relatively short, and there are still some problems.7 In addition, there are also some problems in foreign cold chain transportation. For example, the cost of refrigerated trucks is high.8,9 Many fruits and vegetables are prone to chilling injury during cold storage.10,11 According to the report of the food and Agriculture Organization of the United Nations, in North America, Australia and New Zealand, nearly 55% of food and beverage products are wasted in the supply cold chain.12
In recent years, there has been more and more research on using an electric field and magnetic field in food preservation.13,14 This is because electric and magnetic fields can affect the driving of ions, the polarization of electric dipoles, water absorption, storage and ionization, and thus affect the germination and growth of fruits and vegetables.15 A magnetic field can change the selective permeability of the cell membrane, the position of protein and lipid domains in the membrane, and the molecular position of charge.16 It can also affect related biochemical processes by changing the structure of enzymes.17 The application of a magnetic field is also beneficial to reduce the chilling injury of fruits and vegetables.18 In recent years, people have conducted extensive research on the role of a magnetic field and found that an electromagnetic field has the effect of sterilization and can delay the aging and spoiling of fruits.19–21 Wang et al.22 explored the influence of a magnetic field on liquid water and found that the friction coefficient of magnetized water is smaller than that of untreated water. Similarly, Deng et al.23 studied the changes in the properties of water under a magnetic field and found that the properties of water changed under the action of a magnetic field. The research results of NováK et al.24 showed that a 10 mT magnetic field can inhibit the growth of yeast and slow down their growth.
The principle of electrostatic field preservation is different from that of plant growth. The electrostatic field has a certain promoting effect on the germination, growth, and development of plant seeds, and the application of an electrostatic field can enhance the respiratory metabolism of plants.25 However, the principle of vegetable preservation is to slow down the postharvest respiratory intensity of vegetables to delay their aging and decay. The mechanism of electrostatic field preservation is controversial. On the one hand, some people think that the electric field changes the membrane potential of the biological cell membrane, thus reducing the physiological metabolism of vegetables; on the other hand, some people think that the external electric field has an impact on the electron transmitter of the internal electric field of vegetables, enhancing the preservation effect.14 Yan et al.26 investigated the effects of different electrostatic field intensities and action distances on crystal nucleation, and the results showed that electrostatic fields that only act on narrow surfaces can effectively nucleate at temperatures above the freezing point. This result also confirms that the effect of electrostatic fields is related to ice crystal nucleation.
The main purpose of this study was to research the effect of an electrostatic field and magnetic field on the cool chain transportation of green bell peppers. In the process of cool storage, an electrostatic field and static magnetic field with different strengths were used to evaluate according to physical and chemical indicators, including moisture content, weight loss, color, texture, etc.
2. Materials and methods
2.1 Sample preparation
Green bell peppers (Capsicum annuum L.) used in the experiment were brought from a local market and transported to the laboratory as soon as possible. Green bell peppers were put into transparent fresh-keeping bags for sealed storage, and the quality was tested 0, 3, 6, 9, 12 and 15 days after storage.
The experiment was divided into the blank group, static magnetic field group, and electrostatic field group. (1) Blank group: green bell peppers were packaged in fresh-keeping bags and stored directly in a 4 °C refrigerator; (2) static magnetic field group: green bell peppers were packaged in fresh-keeping bags and stored at 4 °C in a magnetoelectric coupling incubator, with intensities set at 2 mT, 5 mT, and 8 mT, respectively; (3) electrostatic field group: green bell peppers were packaged in fresh-keeping bags and stored at 4 °C in a magnetoelectric coupling incubator, with intensities set at 3 kV cm−1 and 5 kV cm−1, respectively. Each treatment was set up with 3 sets of parallel experiments, with samples taken every 3 days. Each indicator was repeated at least 3 times, and the results are averaged.
2.2 Water content
For fruits and vegetables with high moisture content, the detection of moisture content is very important.27 The water content of green bell pepper samples was determined by the oven atmospheric drying method. Samples were dried at 105 °C, and then taken out every hour to weigh, until they reached a constant weight. Three groups were randomly selected for each treatment method, and the average value was taken as the final result.28 |  | (1) |
2.3 Weight loss
Use an electronic scale to measure the weight loss of the sample (PB203-N electronic balance, Shanghai Balance Instrument Factory). Three green peppers in each group were weighed after 0, 3, 6, 9, 12 and 15 days of storage. The weight loss was calculated according to the following formula:29 | 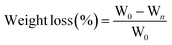 | (2) |
where W0 is the initial weight of peppers, kg; Wn is the weight of peppers after n days, kg.
2.4 Color
The color of the treated samples was evaluated. A hand-held colorimeter (Konica Minolta, CR-400, Tokyo, Japan) was used for the assessment of the color of peppers. Each sample was measured 5 times, and the average value was taken as the final result. According to the color properties of peppers, L* and a* values were selected as the indices to evaluate the color quality of samples. The L* value represents the brightness of the sample. The higher the L* value, the brighter the color. The a* value indicates the red and green degree of the sample. Positive a* indicates that the sample is red and negative a* indicates that the sample is green. In addition, the greater the absolute value of a*, the darker the corresponding color.
2.5 Textural profile analysis (TPA)
The textural characteristics of the samples were measured by using a TA-XT 2 texture analyzer (Stable Micro Systems, Surrey, UK). A portion of the green bell pepper was cut into 1 cm × 1 cm sized blocks. The parts of the green bell pepper were randomly selected and cut into small pieces before mixing them together. Five different pieces of green bell peppers were randomly selected from each group for testing, and the average value was taken as the final result. The probe of TA/45 CS was used for textural profile analysis (TPA). The speed before and after the test was 2 mm s−1, and the test speed was 1 mm s−1. The trigger force was 5 g.30
2.6 Relative conductivity
The central tissue of green bell pepper fruits was cut into small pieces of equal thickness and put into a small beaker, and 30 mL of distilled water was added into the beaker and mixed well. The conductivity was measured for the first time and the initial value was recorded as D0. After 10 minutes, the conductivity was measured for the second time and recorded as D1. Then the mixture was boiled for 1 min. After the solution was cooled to room temperature, distilled water was added to the original scale, and the conductivity D2 was measured for the third time after balancing at room temperature. This was repeated three times for each sample and the average value was taken.31 | 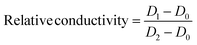 | (3) |
2.7 VC content
The content of VC in the sample was determined by potassium iodate titration. First, 10 g of thawed sample was put into a mortar, and then 2% hydrochloric acid solution (v/v) was added and ground into slurry in an ice bath. The slurry was transferred into a 100 mL volumetric flask, and the volume was adjusted to the scale with 2% hydrochloric acid solution. After 10 minutes of extraction, the suspension was filtered and the filtrate was reserved.
0.5 mL of 10 g L−1 potassium iodide solution, 2.0 mL of 5 g L−1 starch solution, 5.0 mL of extract and 2.5 mL of distilled water were added into a conical flask and mixed well. The solution was treated with 1 mmol L−1 potassium iodate until it was blue and did not fade within 30 seconds. The volume of potassium iodate solution was recorded with a repeat of three times. 5.0 mL 2% hydrochloric acid solution was taken as a blank control and titrated according to the same method. The content of VC was calculated as follows:32
|  | (4) |
where
V1 is the volume of potassium iodate solution consumed in sample titration (mL),
V0 is the volume of potassium iodate solution consumed in blank titration (mL),
Vs is the volume of sample solution taken during titration (mL),
V is the total volume of the sample extract (mL),
m is the mass of the sample (g), and 0.088 is the mass of VC equivalent to 1 mL l mmol L
−1 potassium iodate solution.
2.8 Chlorophyll content (W)
The representative sample was cut and mixed, and was pounded with a tissue crusher into a homogenate for standby. 0.5 g of sample was weighed into a triangular flask, and 100 mL of extraction agent (anhydrous ethanol
:
acetone = 1
:
1) was added. The triangular flask was sealed at room temperature and kept away from light for 5 h, and then filtered. The extraction agent was used as the blank and zero, and the absorbance at 663 nm (A1) and 645 nm (A2) respectively was measured. The calculation formula of chlorophyll content is as follows:33 |  | (5) |
2.9 Statistical analysis
The data were expressed as mean ± standard deviation. Statistix 9.0 was used for LSD (least significant difference) analysis, and GraphPad Prism 8.0 was used for mapping.
3. Results and discussion
3.1 Water content
As shown in Fig. 1, as storage progressed, the water content of both groups of treated and untreated green peppers decreased. During the first six days of storage, there was no significant difference in performance between the magnetic field treated group and the blank group. However, on the ninth day of storage, differences began to appear between the blank group and the magnetic field group. Specifically, as the magnetic field intensity increased, the moisture content of green bell peppers also increased. After storage, the water content of green bell peppers stored at 4 °C and 8 mT was the highest in the magnetic field group, reaching 93%. This indicated that applying a magnetic field can be beneficial for preserving the moisture content of green bell peppers. This was related to the magnetic field effect inside vegetables. The effect of the magnetic field on the cells of green bell peppers can to some extent reduce the permeability of the biofilm, thereby reducing water loss.34
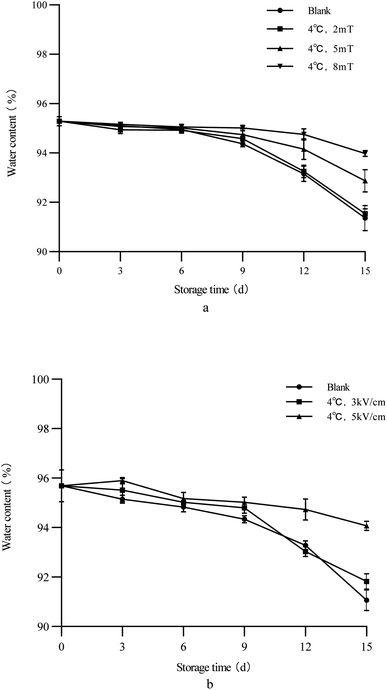 |
| Fig. 1 Changes of the water content of green bell peppers with storage time under different storage conditions ((a) magnetic field; (b) electrostatic field). | |
From Fig. 1(b), it can be seen that the group that was applied an electrostatic field to assist in preservation was different from the control group on the third day, and after storage, the difference was significant compared to the control group. Similarly, the increase in electrostatic field intensity also leads to an increase in the water content of green bell peppers. Although the fluctuation in water content during storage was greater, on the fifteenth day, the water content of green peppers treated at 4 °C and 5 kV cm−1 was still about 94%, which was also the best result compared to the magnetic field group. The preservation of green bell peppers in the electrostatic field was mainly achieved by inhibiting their respiration and physiological metabolism, thereby reducing water loss and achieving a preservation effect. Similar results have been obtained in other studies on electric field preservation, indicating that the effect of an electric field can effectively reduce water loss in fruits and vegetables.13,14
3.2 Weight loss
The weight loss is an important criterion for evaluating the loss of water content in vegetables. The reduction of water content will accelerate the aging and decay of fruit pulp, thereby reducing the commercial value of vegetables.35 As shown in Table 1, the weight loss of green bell peppers gradually increased with the increase of storage time. Compared with the blank group, the weight loss of the treatment group was lower, with the 4 °C, 8 mT group having the lowest weight loss among the magnetic field treatment groups and the 4 °C, 5 kV cm−1 group having the lowest weight loss among the electric field treatment groups. The weight loss of fruits and vegetables is related to water content. From Section 2.1, it was found that the effect of an electric field was more conducive to maintaining water content, and therefore, it was also more conducive to maintaining weight loss.36 The change in weight loss indicated that electrostatic/magnetic field treatment could help reduce the weight loss of green bell peppers, which was similar to previous reports.34
Table 1 Weight loss of green bell peppers during storage under different storage conditionsa
|
Day 3 |
Day 6 |
Day 9 |
Day 12 |
Day 15 |
Different superscripts in the same column represent significant differences in mathematical statistics (p < 0.05).
|
Blank |
0.26 ± 0.01A |
0.46 ± 0.03A |
0.84 ± 0.01A |
1.16 ± 0.06A |
1.58 ± 0.08A |
4 °C, 2 mT |
0.19 ± 0.02B |
0.33 ± 0.02B |
0.58 ± 0.02B |
0.83 ± 0.01B |
1.18 ± 0.03B |
4 °C, 5 mT |
0.15 ± 0.01C |
0.24 ± 0.04D |
0.46 ± 0.04D |
0.59 ± 0.02EF |
0.85 ± 0.06D |
4 °C, 8 mT |
0.14 ± 0.04CD |
0.22 ± 0.01E |
0.42 ± 0.02E |
0.61 ± 0.05D |
0.81 ± 0.03E |
4 °C, 3 kV cm−1 |
0.18 ± 0.02B |
0.30 ± 0.02C |
0.52 ± 0.02C |
0.79 ± 0.01C |
0.94 ± 0.03C |
4 °C, 5 kV cm−1 |
0.13 ± 0.02D |
0.23 ± 0.02DE |
0.38 ± 0.02EF |
0.60 ± 0.01DE |
0.79 ± 0.03F |
3.3 Color
Green bell peppers belong to the respiratory climacteric type of fruit, which undergoes color changes during storage after picking, such as yellowing or turning red at maturity. This is also a more obvious visual change indicating the deterioration of green pepper quality, in addition to spoilage.37
During the observation process of this experiment, the blank group of green peppers showed significant local yellowing before the 15th day of storage. In addition, there were also some chili peppers with rotten or moldy spots on the surface, which no longer meet the edible requirements. The color changes of green peppers under different treatment methods are shown in Table 2. In the magnetic field treatment group, some green peppers in the 2 mT treatment group also showed yellowing, but the other two groups did not change color, especially the green peppers in the 8 mT treatment group. The results showed that neither the group of green peppers treated with an electrostatic field showed any yellowing or redness, nor did they show any deterioration. This indicated that static electricity/magnetic fields can slow down cell metabolism, thereby slowing down green aging and degradation.38
Table 2 Change of the color of green bell peppers with storage time under different storage conditionsa
|
Storage time per d |
Blank |
4 °C, 2 mT |
4 °C, 5 mT |
4 °C, 8 mT |
4 °C, 3 kV cm−1 |
4 °C, 5 kV cm−1 |
Different superscripts in the same line represent significant differences in mathematical statistics (p < 0.05).
|
L* |
0 |
49.27 ± 2.35A |
49.27 ± 2.35A |
49.27 ± 2.35A |
49.27 ± 2.35A |
49.27 ± 2.35A |
49.27 ± 2.35A |
3 |
48.18 ± 1.74A |
45.62 ± 2.39F |
46.20 ± 1.93B |
44.74 ± 2.86E |
46.18 ± 1.33C |
45.77 ± 1.36D |
6 |
47.27 ± 1.90C |
46.72 ± 2.05D |
47.61 ± 2.38A |
43.74 ± 1.52F |
47.54 ± 1.18B |
44.79 ± 1.22E |
9 |
46.91 ± 1.19C |
45.71 ± 1.55D |
47.53 ± 1.74A |
44.02 ± 1.02E |
47.43 ± 1.05B |
43.87 ± 0.93D |
12 |
46.74 ± 1.37A |
44.71 ± 1.45D |
45.59 ± 2.10B |
45.59 ± 1.10B |
45.39 ± 1.10C |
44.52 ± 1.13E |
15 |
42.57 ± 1.29E |
42.31 ± 1.53F |
45.41 ± 1.44A |
45.36 ± 0.87B |
43.21 ± 0.94D |
43.31 ± 0.76C |
a* |
0 |
−9.33 ± 0.52A |
−9.33 ± 0.52A |
−8.99 ± 0.52A |
−8.99 ± 0.52A |
−8.99 ± 0.52A |
−8.99 ± 0.52A |
3 |
−8.19 ± 1.42A |
−9.19 ± 0.79B |
−9.53 ± 1.27E |
−9.72 ± 1.58F |
−9.50 ± 1.08D |
−9.22 ± 0.58C |
6 |
−8.17 ± 1.24A |
−9.11 ± 0.98D |
−8.82 ± 1.25C |
−9.38 ± 0.64F |
−8.79 ± 1.21B |
−9.13 ± 0.44E |
9 |
−8.78 ± 1.74A |
−8.85 ± 0.76B |
−9.65 ± 1.21F |
−9.37 ± 0.71C |
−9.55 ± 1.19E |
−9.48 ± 1.71D |
12 |
−6.62 ± 0.95A |
−8.50 ± 1.01D |
−8.26 ± 0.74C |
−8.87 ± 0.61E |
−8.21 ± 0.84B |
−9.27 ± 0.41F |
15 |
−7.01 ± 0.97A |
−7.66 ± 0.58B |
−8.75 ± 0.43D |
−8.74 ± 1.00D |
−8.34 ± 0.23C |
−8.94 ± 1.08E |
b* |
0 |
11.20 ± 1.13A |
11.20 ± 1.13A |
11.20 ± 1.13A |
11.20 ± 1.13A |
11.20 ± 1.13A |
11.20 ± 1.13A |
3 |
10.61 ± 1.83F |
11.62 ± 1.66A |
11.19 ± 1.82D |
11.46 ± 2.89C |
11.15 ± 1.72E |
11.26 ± 0.86B |
6 |
10.51 ± 1.00D |
9.29 ± 1.37E |
11.05 ± 1.78BC |
10.64 ± 0.79C |
11.06 ± 0.78A |
11.04 ± 0.73B |
9 |
9.89 ± 1.30F |
10.39 ± 0.99D |
10.89 ± 0.81A |
10.36 ± 0.49E |
10.82 ± 1.81B |
10.56 ± 0.79C |
12 |
8.13 ± 0.91F |
9.66 ± 1.52E |
9.79 ± 0.53B |
9.89 ± 0.89C |
9.77 ± 0.73A |
9.68 ± 1.09D |
15 |
8.25 ± 1.59F |
9.12 ± 1.01E |
9.38 ± 0.47C |
9.66 ± 0.91A |
9.36 ± 1.27D |
9.51 ± 0.81B |
3.4 Textural profile analysis (TPA)
The textural changes of green bell peppers during storage for fifteen days are shown in Table 3. The hardness of green bell peppers gradually decreased throughout the refrigeration process, and the hardness of all treatment groups was significantly higher than that of the control group (p < 0.05). The textural characteristics of the blank group of green bell peppers deteriorated the fastest during storage, while the treatment group with electromagnetic field application showed improved texture characteristics, and the improvement effect became more pronounced with the increase of electric/magnetic field intensity. The lowest hardness value of green bell peppers in the blank group may be due to the rapid dehydration and atrophy of green bell pepper cells caused by strong respiration.39 At the same time, the accumulation of free radicals causes relaxation of the cell wall tissue, leading to an increase in internal microorganisms, leading to rapid cell degradation and damage to the tissue structure, ultimately resulting in a decrease in hardness values.40 In the treatment group, the effect of a static electricity/magnetic field inhibited enzyme activity, reduced cell damage, and inhibited the decrease in the hardness of green peppers as well as changes in chewiness and springiness.
Table 3 Change of the texture of green bell peppers during storagea
|
Storage time per d |
Blank |
4 °C, 2 mT |
4 °C, 5 mT |
4 °C, 8 mT |
4 °C, 3 kV cm−1 |
4 °C, 5 kV cm−1 |
Different superscripts in the same line represent significant differences in mathematical statistics (p < 0.05).
|
Hardness (N) |
0 |
1082.42 ± 1.38A |
1082.42 ± 1.38A |
1082.42 ± 1.38A |
1082.42 ± 1.38A |
1082.42 ± 1.38A |
1082.42 ± 1.38A |
3 |
910.43 ± 1.33A |
931.82 ± 1.23D |
893.33 ± 1.38E |
942.85 ± 1.34A |
968.87 ± 1.31B |
1043.58 ± 0.70C |
6 |
848.10 ± 1.21F |
876.42 ± 1.68C |
881.70 ± 0.73B |
867.20 ± 1.48D |
857.13 ± 1.02E |
966.78 ± 1.05A |
9 |
818.93 ± 0.97F |
869.14 ± 1.55B |
862.841 ± 1.62C |
852.74 ± 1.60D |
844.02 ± 1.61E |
946.12 ± 1.54A |
12 |
768.67 ± 1.37F |
861.00 ± 1.16D |
867.77 ± 0.77C |
875.38 ± 1.41B |
826.36 ± 1.28D |
942.63 ± 0.93A |
15 |
749.10 ± 0.83F |
805.84 ± 1.53E |
822.09 ± 0.80C |
859.10 ± 0.94B |
824.90 ± 0.86D |
893.65 ± 1.64A |
Chewiness (N) |
0 |
171.21 ± 1.53A |
171.21 ± 1.53A |
171.21 ± 1.53A |
171.21 ± 1.53A |
171.21 ± 1.53A |
171.21 ± 1.53A |
3 |
123.00 ± 1.34F |
149.56 ± 1.23B |
138.54 ± 0.96D |
133.04 ± 1.15E |
153.48 ± 0.80A |
138.92 ± 1.69C |
6 |
117.77 ± 1.24F |
133.93 ± 1.12C |
130.30 ± 1.37E |
132.21 ± 0.82D |
139.81 ± 0.55B |
140.05 ± 1.84A |
9 |
101.31 ± 1.78F |
109.56 ± 0.76E |
117.77 ± 1.14D |
121.79 ± 1.54C |
135.04 ± 1.14A |
135.06 ± 1.54B |
12 |
85.89 ± 1.12F |
91.48 ± 1.37E |
101.72 ± 0.83D |
114.22 ± 1.46B |
107.16 ± 0.95C |
122.06 ± 1.21A |
15 |
71.13 ± 0.97F |
90.76 ± 1.14D |
88.55 ± 1.02E |
109.98 ± 1.52B |
102.52 ± 0.58C |
112.72 ± 1.10A |
Springiness |
0 |
0.39 ± 0.09A |
0.39 ± 0.09A |
0.39 ± 0.09A |
0.39 ± 0.09A |
0.39 ± 0.09A |
0.39 ± 0.09A |
3 |
0.37 ± 0.04D |
0.39 ± 0.01A |
0.38 ± 0.03B |
0.39 ± 0.02B |
0.38 ± 0.03C |
0.39 ± 0.02AB |
6 |
0.35 ± 0.05E |
0.37 ± 0.02C |
0.36 ± 0.01D |
0.37 ± 0.02B |
0.35 ± 0.02E |
0.38 ± 0.01A |
9 |
0.33 ± 0.03B |
0.32 ± 0.01C |
0.33 ± 0.02B |
0.37 ± 0.01A |
0.30 ± 0.03D |
0.37 ± 0.01A |
12 |
0.29 ± 0.04E |
0.30 ± 0.01D |
0.30 ± 0.03D |
0.36 ± 0.01B |
0.31 ± 0.04C |
0.37 ± 0.01A |
15 |
0.26 ± 0.03E |
0.28 ± 0.01D |
0.28 ± 0.02C |
0.29 ± 0.01B |
0.25 ± 0.03F |
0.32 ± 0.01A |
3.5 Relative conductivity
In the metabolism of plant cells, the change of permeability of the cell membrane can be explored through the change of relative conductivity. External stimulation of vegetables will lead to the increase of permeability of the cell membrane, and the cell membrane changes from a liquid crystal state to a gelled state, which will lead to the increase of relative conductivity of fruits.41 Therefore, the higher the relative conductivity of vegetables, the higher the degree of damage to their cell membranes, which can evaluate the freshness of vegetables.42
Fig. 2 shows the changes in the relative conductivity of green bell peppers during storage. After storage, there was a significant difference in the relative conductivity between the treatment group and the control group. The results showed that the relative conductivity of green peppers treated with a 5 kV cm−1 electrostatic field remained the lowest during and after storage. This indicated that the electrostatic field treatment applied in this study can effectively inhibit the increase in the relative conductivity of green peppers and protect the integrity of the green pepper cell membrane and cell structure.
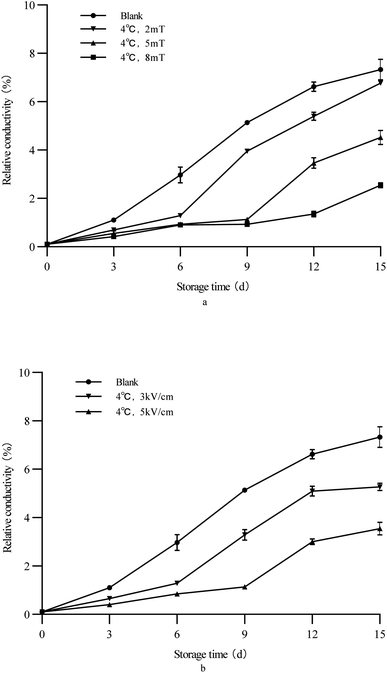 |
| Fig. 2 Change of the relative conductivity of green bell peppers with storage time under different storage conditions ((a) magnetic field; (b) electrostatic field). | |
3.6 VC content
VC is one of the most important nutritional components in green peppers. As shown in Fig. 3, with the extension of storage time, the VC content of green peppers gradually decreased, but the VC content of the treatment group green bell peppers remained at a high level. In previous studies, methods such as ultrasound treatment,43,44 microwave treatment,45 and vacuum cooling46 were commonly used to reduce the loss of VC in fruits and vegetables. In this study, the use of a static magnetic field and static electric field effectively reduced the loss of VC in green bell peppers. With the increase of electrostatic/magnetic field intensity, the retention effect of ascorbic acid in green peppers was better. Among several different electric/magnetic field intensities, the 5 kV cm−1 electric field treatment group had the highest ascorbic acid content in green peppers after 15 days of storage. For the VC content in green peppers, electrostatic field treatment can better reduce its loss and retain the nutrients in the peppers.
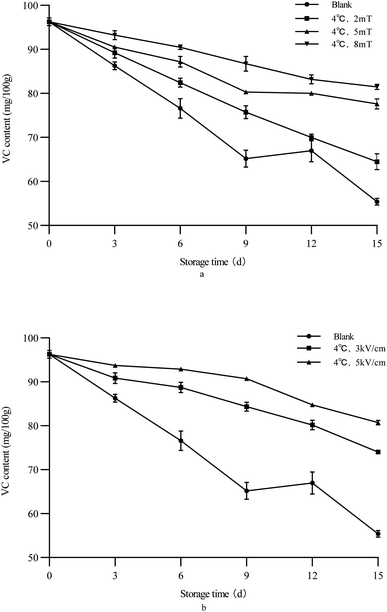 |
| Fig. 3 Changes of the VC content of green bell peppers with storage time under different storage conditions ((a) magnetic field; (b) electrostatic field). | |
3.7 Chlorophyll content
For green vegetables such as green peppers, the content of chlorophyll is very important, which also indicates the freshness of the vegetables.47 Green peppers may turn yellow or red during storage after picking, which affects their value. This phenomenon was mainly due to the degradation of chlorophyll in the skin cells of green peppers after picking, causing the color of carotenoids (yellow) to gradually appear.48
As shown in Fig. 4, as storage progresses, the chlorophyll content of green bell peppers gradually decreased, and the chlorophyll content of the treated group was consistently higher than that of the control group. The chlorophyll content in green peppers treated with an electric field was the highest, indicating that electrostatic/magnetic field treatment helps to maintain the chlorophyll content in green peppers, with the 5 kV cm−1 electric field treatment group having the best effect. Due to the differences in the principles of magnetic and electric fields, their effects also vary. But for chili peppers, the results indicate that electric field treatment is more effective.
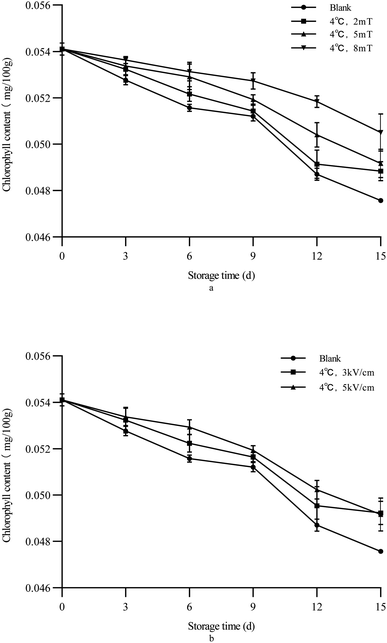 |
| Fig. 4 Change of the chlorophyll content of green bell peppers with storage time under different storage conditions ((a) magnetic field; (b) electrostatic field). | |
4. Conclusions
This study used static magnetic fields and electrostatic fields of different intensities to assist in the cold chain transportation of green peppers. Through 15 days of refrigeration and simulated transportation, the effects of static magnetic fields and electrostatic fields on the quality and nutrition of green peppers were explored. The following conclusion was drawn.
Static magnetic field/electric field assisted cold storage of green bell peppers was beneficial for improving the quality and nutrition of green peppers. Compared with the blank control group, there were improvements in the water content, texture, relative conductivity, VC, and other aspects. In addition, with the increase of magnetic field/electric field intensity, the effect of improving the quality of green pepper was enhanced, especially in terms of relative conductivity and VC content. However, considering all factors, the treatment conditions of 4 °C and 5 kV cm−1 were still the best, which can improve the nutrition and quality of cold chain transportation of green bell peppers.
Author contributions
Tianlin Feng, Min Zhang, Arun S. Mujumdar, and Lihui Zhang wrote the main manuscript text and Tianlin Feng prepared figures and tables. All authors reviewed the manuscript.
Conflicts of interest
The authors declare no conflict of interest.
Acknowledgements
We acknowledge the financial support from the National Key R&D Program of China (No. 2022YFD2100601), Jiangsu Province Key Laboratory Project of Advanced Food Manufacturing Equipment and Technology (No. FMZ202003), and National First-Class Discipline Program of Food Science and Technology (No. JUFSTR20180205). All these above enable us to carry out and promote this research smoothly. We would like to express our thanks to Mr Chaohui Yang from Yechun Food Production and Distribution Co. for his support in testing raw materials.
References
- A. Marin, J. Agric. Food Chem., 2004, 52, 3861–3869 CrossRef CAS PubMed.
- Y. Wahyuni, A.-R. Ballester, E. Sudarmonowati, R. J. Bino and A. G. Bovy, J. Nat. Prod., 2013, 76, 783–793 CrossRef CAS PubMed.
- K. Devgan, P. Kaur, N. Kumar and A. Kaur, J. Food Sci. Technol., 2019, 56, 878–888 CrossRef CAS.
- W. Liu, M. Zhang, B. Bhandari and D. Yu, LWT-Food Sci. Technol., 2020, 137, 110455 CrossRef.
- M. Kaur and M. Kumar, Food Rev. Int., 2020, 36, 761–780 CrossRef.
- X. F. Wu, M. Zhang, B. Adhikari and J. Sun, Crit. Rev. Food Sci. Nutr., 2016, 57, 3620–3631 CrossRef.
- X. Xu, X. Zhang, M. Jotham, Y. Li, Y. Chen, Q. Chen and S. Liu, Trans. Chin. Soc. Agric. Eng., 2017, 33, 308–314 Search PubMed.
- Y. Zhao, X. Zhang and X. Xu, J. Therm. Anal. Calorim., 2019, 139 CAS.
- T. Chen, M. Zhang and J. J. Chen, J. Food Sci. Biotechnol., 2020, 39, 33–40 Search PubMed.
- H. Shi and M. Zhang, J. Food Sci. Biotechnol., 2020, 39, 48–55 Search PubMed.
- Y. Fan and M. Zhang, J. Food Sci. Biotechnol., 2020, 39, 81–86 Search PubMed.
- E. S. Funcia, J. A. Gut and S. K. Sastry, Food Bioprocess Technol., 2020, 13, 1206–1214 CrossRef CAS.
- A. Morata, M. A. Bauelos, I. Loira, J. Raso and J. A. S. Lepe, Food Bioprocess Technol., 2020, 13, 1087–1094 CrossRef CAS.
- B. Saletnik, G. Zaguła, A. Saletnik, M. Bajcar, E. Słysz and C. Puchalski, Appl. Sci., 2022, 12, 3183 CrossRef CAS.
- B. Saletnik, G. Zaguła, A. Saletnik, M. Bajcar, E. Słysz and C. Puchalski, Appl. Sci., 2022, 12, 3975 CrossRef CAS.
- H. L. A. Miñano, A. C. d. S. Silva, S. Souto and E. J. X. Costa, Processes, 2020, 8, 814 CrossRef.
- S. Zhao, X. Han, B. Liu, W. Guan and Q. Dai, J. Food Process Eng., 2021, 38, 1258–1265 Search PubMed.
- S. Zhao, Z. Yang, L. Zhang, N. Luo and X. Li, J. Food Eng., 2018, 217, 24–33 CrossRef CAS.
- Z. Yang, L. Zhang, S. Zhao, N. Luo and Q. Deng, J. Food Process Eng., 2020, 43 CAS.
- T. Kang, Y. You, R. Hoptowit, M. M. Wall and S. Jun, J. Food Eng., 2021, 110541 CrossRef CAS.
- L. Zhang, Z. Yang, S. Zhao, N. Luo and Q. Deng, Food Bioprocess Technol., 2020, 13, 732–738 CrossRef CAS.
- Y. Wang, B. Zhang, Z. Gong, K. Gao, Y. Ou and J. Zhang, J. Mol. Struct., 2013, 1052, 102–104 CrossRef CAS.
- B. Deng and X. F. Pang, Dianzi Keji Daxue Xuebao/J. Univ. Electron. Sci. Technol. China, 2008, 37, 959–962 CAS.
- J. Novák, L. k. Straák, L. Fojt, I. Slaninová and V. Vetterl, Bioelectrochemistry, 2007, 70, 115–121 CrossRef PubMed.
- A. I. Wenjia, Y. Fang, S. Xiang, X. Yao and G. O. Phillips, J. Nutr. Sci. Vitaminol., 2015, 61, S168 CrossRef.
- J. Yan and G. Patey, J. Phys. Chem. A, 2012, 116, 7057–7064 CrossRef CAS PubMed.
- L. Qiu, M. Zhang, J. Tang, B. Adhikari and P. Cao, Food Res. Int., 2019, 116, 90–102 CrossRef CAS.
- M. Zhang, J. Tang, A. S. Mujumdar and S. Wang, Trends Food Sci. Technol., 2006, 17, 524–534 CrossRef CAS.
- D. Vázquez-Celestino, H. Ramos-Sotelo, D. M. Rivera-Pastrana, M. Vázquez-Barrios and E. M. Mercado-Silva, Postharvest Biol. Technol., 2015, 111, 398–405 CrossRef.
- L. Li, M. Zhang, B. Bhandari and L. Zhou, Drying Technol., 2018, 1–10 CrossRef.
- B.-g. Xu, M. Zhang, B. Bhandari, X.-f. Cheng and J. Sun, Food Bioprocess Technol., 2015, 8, 1366–1376 CrossRef.
- H. Qing-Guo, Z. Min, A. S. Mujumdar, D. Wei-Hua and S. Jin-Cai, Drying Techonlogy, 2006, 24, 1025–1032 CrossRef.
- Z. Wu, M. Zhang and S. Wang, Food Control, 2012, 23, 120–127 CrossRef CAS.
- Z. Ayhan and O. Estuerk, J. Food Sci., 2009, 74, 55–61 CrossRef.
- K. Chitravathi, O. P. Chauhan and P. S. Raju, Food Packag. Shelf Life, 2015, 4, 1–9 CrossRef.
- L. Wang, M. Zhang, B. Bhandari and Z. Gao, Food Res. Int., 2016, 86, 131–139 CrossRef CAS.
- R. Kosson and A. Stepowska, Veg. Crops Res. Bull., 2006, 19–27 Search PubMed.
- Q. Wang, Y. Li, D. W. Sun and Z. Zhu, Crit. Rev. Food Sci. Nutr., 2018, 58, 2285–2298 CrossRef CAS PubMed.
- C. Barry-Ryan, J. M. Pacussi and D. O’Beirne, J. Food Sci., 2000, 65, 726–730 CrossRef CAS.
- S. H. Mirdehghan, M. Rahemi, D. Martínez-Romero, F. Guillén, J. M. Valverde, P. J. Zapata, M. Serrano and D. Valero, Postharvest Biol. Technol., 2007, 44, 19–25 CrossRef CAS.
- J. An, M. Zhang and Q. Lu, J. Food Eng., 2007, 78, 340–344 CrossRef CAS.
- X. Meng, Z. Min and B. Adhikari, Postharvest Biol. Technol., 2012, 71 Search PubMed.
- K. Fan, M. Zhang and F. Jiang, Ultrason. Sonochem., 2019, 54, 162–170 CrossRef CAS.
- X.-f. Cheng, M. Zhang, B. Adhikari, M. N. Islam and B.-g. Xu, Int. J. Refrig., 2014, 44, 49–55 CrossRef.
- X. Duan, M. Zhang and A. S. Mujumdar, Drying Technol., 2007, 25, 1725–1731 CrossRef.
- F. Tao, M. Zhang and H.-q. Yu, J. Food Eng., 2007, 79, 1302–1309 CrossRef CAS.
- H.-z. Chen, M. Zhang, B. Bhandari and Z. Guo, Postharvest Biol. Technol., 2018, 140, 85–92 CrossRef CAS.
- F. Zhang, J. Chai, L. Zhao, Y. Wang and X. Liao, Foods, 2021, 10, 508 CrossRef CAS.
|
This journal is © The Royal Society of Chemistry 2023 |