DOI:
10.1039/D1RA08605G
(Paper)
RSC Adv., 2022,
12, 8611-8616
A novel dibenzo[a,c]phenazine-based fluorescent probe for fast and selective detection of thiophenols in environmental water†
Received
24th November 2021
, Accepted 14th March 2022
First published on 18th March 2022
Abstract
We herein report a novel dibenzo[a,c]phenazine-based fluorescent probe with fast response to thiophenols over a wide pH range from 5 to 13. The probe possesses a large Stokes shift (120 nm). More importantly, it displays a high selectivity and sensitivity for thiophenols in the presence of other analytes such as biothiols and common metal ions. A good linear relationship between the fluorescence intensity at 570 nm and the thiophenol concentration in the range of 0–20 μM was observed with a low detection limit at 40 nM. In addition, it has been successfully applied to detect thiophenols in environmental water (such as seawater, tap water and spring water) with high recovery, which confirmed its potential value in environmental monitoring.
1. Introduction
Thiophenols are widely used in the production of pesticides, dyes, and medicines.1–3 However, they are also known as volatile toxic substances, and can cause serious damage to bioorganisms and environment, especially the water environment.4 The median lethal concentration (LC50) of thiophenols to fish (0.01–0.04 mM) and mice is 0.01–0.04 mM and 46.2 mg kg−1, respectively.5,6 Inhalation or ingestion of thiophenols can cause serious health problems in humans, such as muscle atrophy, vomiting, and even death.6,7 When thiophenols are incorporated into the water ecosystem, they can be absorbed by aquatic organisms, and ultimately harm human beings.8 Therefore, there is an urgent need to develop highly sensitive and selective methods to detect thiophenols in environmental samples.
Several approaches, such as high-performance liquid chromatography (HPLC), gas chromatography (GC), and capillary electrophoresis, have been developed separation and detection of thiophenols.9–13 Among numerous detecting methods of thiophenols, fluorescent method was considered as the most powerful tool for thiophenols detection, because of the intrinsic advantages such as high selectivity and high sensitivity.14–19 In these probes, 2,4-dinitrobenzenesulfonyl or 2,4-dinitrophenyl groups were employed as recognition moieties linked to various fluorophores.20–25 However, there are still some defects, such as high pH-dependence of the fluorescence intensity, weak fluorescence enhancement after the addition of thiophenols, and relatively long response time, which limit the practical application of fluorescent method in environmental samples. Thus, it is still of great significance to explore novel applicable probes for rapid detection of thiophenols.
Dibenzo[a,c]phenazines is a kind of excellent organic fluorescent compounds with large Stokes shift, high quantum yields, good photostability, and easy synthesis, which were widely used in organic light-emitting diode (OLED) field.26–28 However, dibenzo[a,c]phenazines have so far received little attention in the field of fluorescent probe. Since the first report of 2,4-dinitrobenzenesulfonyl group as a fluorescence quencher,29 a lot of fluorescent probes with 2,4-dinitrobenzenesulfonyl group as recognition site were synthesized and reported.30–33 Herein, we employed dibenzo[a,c]phenazin-11-amine as a fluorophore and 2,4-dinitrobenzenesulfonyl group as recognition site to develop a novel fluorescent probe for fast detecting of thiophenols in water. The probe exhibits high selectivity and sensitivity for thiophenols with a large Stokes shift (120 nm). It is noteworthy that this probe can work in a wide pH range from 5 to 13 with fast response. As far as we know, this is the first thiophenols fluorescent probe which can work in such a wide pH range. Moreover, the probe demonstrated its capability to monitor thiophenols in real water samples.
2. Experimental
2.1. Materials and instrumentation
All solvents and materials were purchased in analytical grade and used as received. 1H NMR and 13C NMR spectra were based on a Bruker Avance-500 MHz NMR spectrometer (Bruker-Biospin, Billerica, MA, USA). High-resolution mass spectra were taken on WATERS MALDI SYNAPT G2 HDMS (MA, USA). The pH was measured by Ion analyzer (LEICI, China). UV-vis spectra were recorded on spectrophotometer (UV-1800, Shimadzu, Japan). Fluorescence spectra were captured on a Microplate Reader (Infinite M1000 Pro, Tecan, Switzerland). High performance liquid chromatographic (HPLC) analysis was performed on a Shimadzu HPLC system (LC-20, Shimadzu corp., Kyoto, Japan) equipped with a C18 column (Shimadzu InertSustain 4.6 I. D. × 250 mm, 5 μm, S/N 6LR98081).
2.2. Synthesis of probe-dbp
Probe-dbp was synthesized according to Scheme 1. The characterization data including 1H NMR and 13C NMR were given in the ESI.† To a solution of dbp-NH2 (0.1 mmol) in dry pyridine (5 mL) at 0 °C, a solution of 2,4-dinitrobenzenesulfonyl chloride (0.15 mmol) in CH2Cl2 (5 mL) was added dropwise. The mixture was stirred at room temperature overnight. After solvent was evaporated, the crude product was purified by column chromatography to give the probe-dbp as a yellow solid (23.6 mg, yield 45%). 1H NMR (500 MHz, DMSO-d6) δ 9.20 (dd, J = 8.2 Hz, 1H), 9.18 (d, J = 8.2 Hz, 1H), 8.92 (d, J = 2.1 Hz, 1H), 8.77 (d, J = 7.2 Hz, 2H), 8.60 (dd, J = 8.8, 2.1 Hz, 1H), 8.40 (d, J = 8.8 Hz, 1H), 8.31 (d, J = 9.1 Hz, 1H), 7.95 (d, J = 2.1 Hz, 1H), 7.88 (d, J = 8.1 Hz, 1H), 7.85 (d, J = 8.1 Hz, 1H), 7.79 (m, 3H), 5.74 (s, 1H). 13C NMR (125 MHz, DMSO-d6) δ 150.73, 148.36, 142.62, 142.24, 141.45, 139.43, 136.36, 132.13, 132.04, 131.78, 131.51, 131.20, 129.74, 129.53, 128.77, 128.74, 128.03, 126.18, 125.84, 125.05, 124.10, 121.05, 116.18. HRMS (ESI) m/z calcd for C26H16N5O6S ([M + H]+): 526.0816, found 526.0810.
 |
| Scheme 1 The synthetic routes of probe-dbp. Reagents and conditions: (a) C2H5OH, CH3COOH, reflux; (b) C2H5OH, Pd–C, N2H4·H2O, reflux; (c) 2,4-dinitrobenzenesulfonyl chloride, pyridine, CH2Cl2, RT. | |
2.3. Spectral measurements
2.5 mM of probe-dbp stock solution and 5 mM p-thiocresol (a very common thiophenol) stock solution were prepared in analytical grade dimethyl sulfoxide (DMSO). The stock solutions were diluted with the mixed solvent (pH = 7.4, 10 mM PBS buffer/DMSO = 1
:
1, v/v) to desired concentration. The absorption and fluorescence spectra were carried out at room temperature (λex = 450 nm and λex = 570 nm). The stock solutions (5 mM) of p-F-C6H4SH, p-CH3O–C6H4SH, C6H5NH2 and C6H5OH were also prepared in DMSO. Other analytes such as amino acids, common metal ions and aliphatic thiols were dissolved in ultrapure water. All stock solutions were used immediately after preparation and were diluted with the mixed solvent (pH = 7.4, 10 mM PBS buffer/DMSO = 1
:
1, v/v) to desired concentration. The mixture of probe-dbp and analytes were incubated for 15 min at 37 °C before recording the fluorescence and absorption spectra. The results were reported as the mean ± standard deviation of triplicate experiments.
2.4. Preparation of real water samples
The tap water of Qingdao, spring water of Laoshan Mountain (Qingdao, Shandong province, China), and seawater from Jiaozhou Bay (Qingdao, Shandong province, China) were collected as the crude water samples. The water samples were filtered and kept at 4 °C before use. These water samples were then spiked with p-thiocresol to desired concentration (0, 2.0, 4.0, 6.0, 8.0, 10.0, 15.0, 20.0 μM). Then, the water samples were incubated with probe-dbp for 15 min before measurement, and the fluorescence intensity at 570 nm was recorded.
3. Results and discussion
3.1. Synthesis of probe-dbp
Dibenzo[a,c]phenazines is a kind of excellent organic fluorescent compounds with large Stokes shift and good photostability. On the other hand, 2,4-dinitrobenzenesulfonyl group is a well-known fluorescence quencher and an effective reaction site for thiophenols recognition. Base on this fact, we designed probe-dbp with dibenzo[a,c]phenazin-11-amine as fluorophore linked to 2,4-dinitrobenzenesulfonyl group as recognition site. The synthetic routes were shown in Scheme 1. 9,10-Phenanthrenequinone and 4-nitro-o-phenylenediamine were selected as starting material. A mixture of them were refluxed in ethanol to give compound dbp-NO2. Dbp-NO2 was then reduced by Pd–C and N2H4·H2O to produce dbp-NH2. Dbp-NH2 reacted with 2,4-dinitrobenzenesulfonyl chloride to afford probe-dbp. The experimental part and spectral data can be found in the ESI.†
3.2. Photophysical properties and response towards thiophenols
The absorption and fluorescence spectroscopy of probe-dbp before and after addition of p-thiocresol were measured in aqueous medium (pH = 7.4, 10 mM PBS buffer/DMSO = 1
:
1, v/v) and depicted in Fig. S1.† The maximum absorption of probe-dbp was located at 450 nm. Before the addition of p-thiocresol, probe-dbp displayed no fluorescence. When p-thiocresol (50 μM) was added into the solution containing probe-dbp (5 μM), a strong fluorescence enhancement (∼50 fold) was observed at 570 nm (λex = 450 nm) (Fig. S2†).
The time-dependent response of probe-dbp to p-thiocresol was further evaluated. As depicted in Fig. 1A, fluorescence was observed immediately after the addition of p-thiocresol. The fluorescence intensity increased rapidly at 570 nm in the first 5 min upon the addition of the p-thiocresol, and reached equilibrium in 15 min. The results indicated that probe-dbp was a rapid and remarkable fluorescent probe for detecting thiophenols.
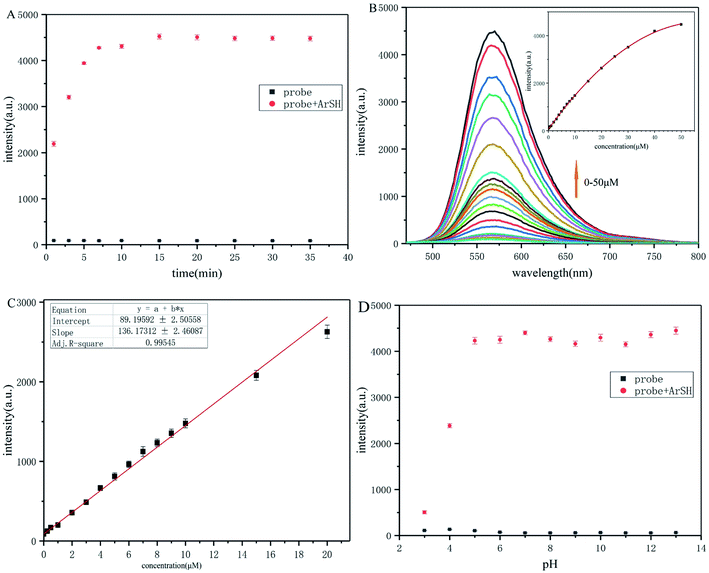 |
| Fig. 1 (A) Fluorescence intensity at 570 nm for probe-dbp with/without p-thiocresol as a function of time. (B) Fluorescence emission spectra of probe-dbp upon the addition of different concentration of p-thiocresol (0, 0.25, 0.5, 1, 2, 3, 4, 5, 6, 7, 8, 9, 10, 15, 20, 25, 30, 40, 50 μM). (C) The linear relationship between the fluorescence intensity of probe-dbp at 570 nm and the concentration of p-thiocresol. (D) The effect of pH on the fluorescence intensity of probe-dbp at 570 nm. λex = 450 nm, λem = 570 nm. Relative standard deviation (RSD), n = 3. | |
Fluorometric titration experiments of probe-dbp were carried out and the results were shown in Fig. 1B. As the thiophenol concentration increasing, the emission intensity of the probe-dbp solution grew and reached a saturation when 10 equivalents of p-thiocresol was added. In addition, a good linear relationship (Fig. 1C) between the fluorescence intensity at 570 nm and the thiophenol concentration in a range of 0–20 μM (R2 = 0.99545) was observed with a detection limit of 40 nm (3δ/k).
3.3. The effect of pH
Probe-dbp itself is pH stable in a broad pH range from 3 to 13 (Fig. 1D). However, with the addition of p-thiocresol (50 μM), the probe solution showed a large fluorescence enhancement at 570 nm between pH 4.0 and 13.0. Moreover, the fluorescence enhancement at 570 nm was stable towards pH ranging from 5 to 13. As far as we know, there is no thiophenols fluorescent probe has been reported possessing such stable fluorescence enhancement over such a wide pH range (Table S1†). The results suggested that probe-dbp was able to apply for thiophenols monitoring in a wide pH range, even in a strong alkaline condition.
3.4. Selectivity for thiophenols
To explore the selectivity of probe-dbp towards thiophenols, the fluorescence change of probe-dbp towards various relevant analytes including thiophenols (p-thiocresol, 4-methoxy thiophenol, 4-fluorothiophenol), aliphatic thiols such as ethanethiol, glutathione (GSH), cysteine(Cys), homocysteine (Hcy), amino acids (Lys, His, Val, Tyr, Ala, Leu, Trp, Ile, Ser, Met), nucleophilic substances such as Ph-NH2, Ph-OH, NaSH, and other potential interfering species (Pb(AcO)2, FeCl3, NaHSO3, CuSO4, FeSO4, KI, CuI, CaCl2, MgSO4, NaCl, SnCl2, Na2SO3) were studied. As illustrated in Fig. 2A, addition of aliphatic thiols, amino acids, nucleophilic substances and other competitive species to the solution of probe-dbp did not induce remarkable fluorescence enhancements. In contrast, obvious fluorescence enhancements were observed when thiophenols were added into the solution of probe-dbp. Although NaHS caused a weak fluorescence enhancement, the enhancement is negligible compared to thiophenols. Moreover, when thiophenol was added into the solution of probe-dbp and the above-mentioned analytes (Fig. 2B), remarkable fluorescence changes could also be observed, indicating that probe-dbp possesses good anti-interference ability. Thus, it can be concluded that probe-dbp is highly selective for thiophenols over other analytes.
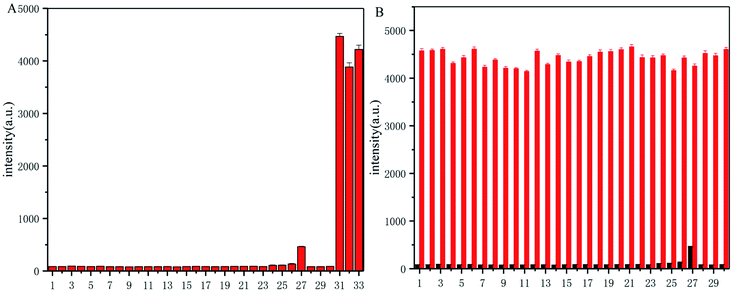 |
| Fig. 2 (A) Fluorescence intensity responses of probe-dbp (5 μM) at 570 nm in the presence of 100 μM aliquots of various analytes. (B) Fluorescence intensity responses of probe-dbp (5 μM) at 570 nm toward p-thiocresol in the presence of various analytes. (1) blank, (2) Na2SO3, (3) Pb(AcO)2, (4) FeCl3, (5) NaHSO3, (6) CuSO4, (7) FeSO4, (8) KI, (9) CuI, (10) CaCl2, (11) MgSO4, (12) NaCl, (13) SnCl2, (14) Lys, (15) His, (16) Val, (17) Tyr, (18) Ala, (19) Leu, (20) Try, (21) iso-Leu, (22) Ser, (23) Met, (24) GSH, (25) homo-Cys, (26) Cys, (27) NaHS, (28) C2H5SH, (29), aniline, (30) phenol, (31) p-thiocresol, (32) 4-methoxythiolphenol, (33) 4-fluorothiophenol. Black bar represent the addition of a single analyte (100 μM). Red bar represent the subsequent addition of p-thiocresol (100 μM) to the mixture. λex = 450 nm, λem = 570 nm. Relative standard deviation (RSD), n = 3. | |
3.5. Response mechanism
To understand the response mechanism of probe-dbp towards thiophenols, HPLC and mass spectrometry were employed to analyze probe-dbp, dbp-NH2, and the products of probe-dbp reacted with p-thiocresol. As shown in Fig. 3, probe-dbp, dbp-NH2 and p-thiocresol possess a retention time at 8.0 min, 15.1 min and 18.3 min, respectively. With the addition of p-thiocresol into probe-dbp, the peak of probe-dbp at 8.0 min decreased. Meanwhile, the peak of dbp-NH2 at 15.1 min appeared. Furthermore, we could see the m/z = 296.1176 in the mass spectrum (Fig. 4), which belong to the cleavage product dbp-NH2. From the HPLC and HRMS analysis, we can conclude that the 2,4-dinitrobenzenesulfonyl group was removed by p-thiocresol to give the fluorophore molecular dbp-NH2 (Scheme 2).
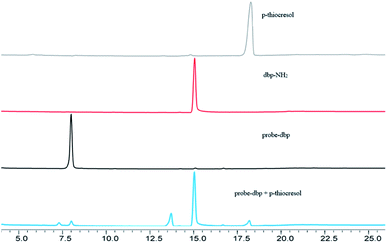 |
| Fig. 3 HPLC chromatograms of p-thiocresol, dbp-NH2, probe-dbp, probe-dbp + p-thiocresol. | |
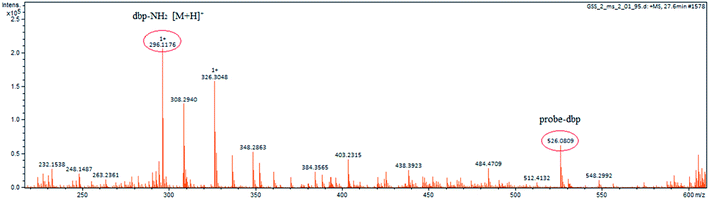 |
| Fig. 4 Mass spectrum of the reaction solution of probe-dbp and p-thiocresol. | |
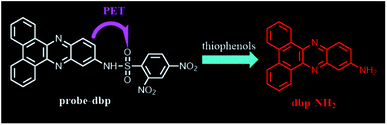 |
| Scheme 2 The proposed detection mechanism towards thiophenol. | |
To further verify the response mechanism of probe-dbp for thiophenols, the density functional theory (DFT) was applied using the Gaussian 09 program. As shown in Fig. 5, the highest occupied molecular orbital (HOMO) of probe-dbp is primarily distributed in dibenzo[a,c]phenazin-11-amine group, while the lowest unoccupied molecular orbital (LUMO) is mainly distributed in 2,4-dinitrobenzenesulfonyl group. The calculated results demonstrate the possible photoinduced electron transfer (PET) processes in probe-dbp. Electron transfer from dibenzo[a,c]phenazin-11-amine group (PET donor) to 2,4-dinitrobenzenesulfonyl group (PET acceptor) attenuates the original fluorescence of dibenzo[a,c]phenazin-11-amine. When 2,4-dinitrobenzenesulfonyl group was removed, the fluorescence of dbp-NH2 can be restored because of the removal of 2,4-dinitrobenzenesulfonyl group. The calculated results exhibit good consistency with the experimental results.
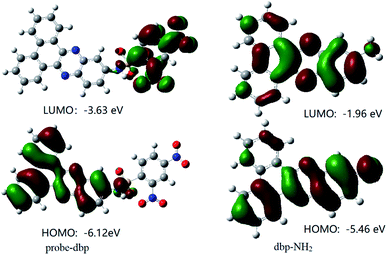 |
| Fig. 5 Frontier orbital diagrams of probe-dbp and dbp-NH2. | |
3.6. Application in real water samples
Considering the potential damage of thiophenols to the water ecosystem, probe-dbp was employed to evaluate thiophenols concentration in real water samples. Tap water of Qingdao city, spring water from Laoshan Mountain (Qingdao, Shandong province, China) and seawater from Jiaozhou Bay (Qingdao, Shandong province, China) were collected and filtered for test. A variety of concentrations of p-thiocresol (0–20 μM) were spiked into these water samples. Then, these water samples were incubated with probe-dbp. The fluorescence intensity at 570 nm were recorded. It shows good relationship between the fluorescence intensity and thiophenol concentrations in a range of 0–20 μM (Fig. 6) in all water samples. As shown in Table S2,† the calculated thiophenol recoveries for each water samples ranged from 93% to 108%, suggesting that probe-dbp is specific for thiophenols detection in real water samples. The method described above might provide a new way for quantitative detection of thiophenols in both freshwater and seawater samples.
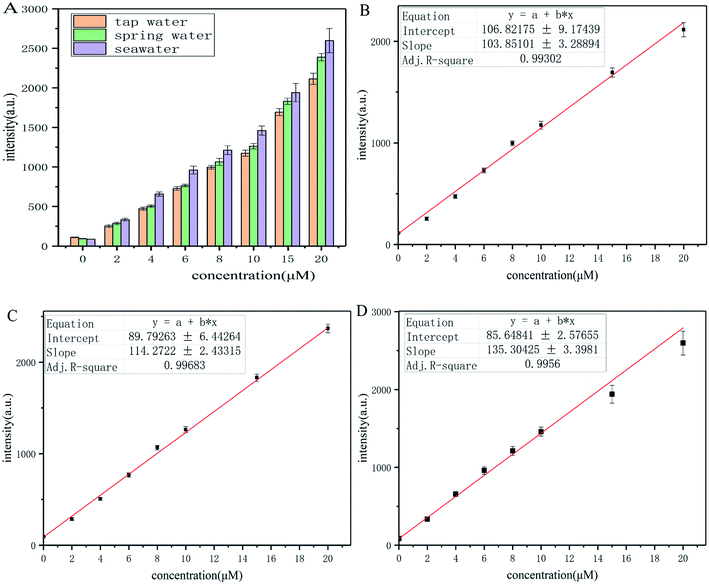 |
| Fig. 6 (A) Fluorescence response of probe-dbp (5 μM) towards various concentration of p-thiocresol in different water samples, including tap water, spring water and seawater. The linear relationship between the fluorescence intensity of probe-dbp at 570 nm and the concentration of p-thiocresol in tap water (B), spring water (C) and seawater (D). | |
4. Conclusion
In this work, we have designed and synthesized probe-dbp with dibenzo[a,c]phenazin-11-amine as fluorophore linked to 2,4-dinitrobenzenesulfonyl group as recognition site. Probe-dbp exhibited high selectivity and sensitivity towards thiophenols over a wide pH range from 5 to 13. Moreover, the probe can be used for the quantitative detection of thiophenols in both freshwater and seawater samples. It can be concluded that probe-dbp possesses a great potential for detection and quantification of thiophenols in environmental systems, even in a strong alkaline condition.
Conflicts of interest
There are no conflicts of interest to declare.
Acknowledgements
This work was supported by NSFC-Shandong Joint Fund (U1706213).
References
- A. Eychmuller and A. L. Rogach, Pure Appl. Chem., 2000, 72, 179–188 CrossRef CAS.
- K. Shimada and K. Mitamura, J. Chromatogr. B: Biomed. Sci. Appl., 1994, 659, 227–241 CrossRef CAS.
- J. C. Love, L. A. Estroff, J. K. Kriebel, R. G. Nuzzo and G. M. Whitesides, Chem. Rev., 2005, 105, 1103–1169 CrossRef CAS PubMed.
- I. C. Popoff, C. B. Thanawalla, R. A. Creager and J. R. Frank, J. Agric. Food Chem., 1972, 20, 80 CrossRef CAS.
- T. P. Heil and R. C. Lindsay, J. Environ. Sci. Health - Part B Pesticides, Food Contam. Agric. Wastes, 1989, 24, 349–360 CrossRef CAS PubMed.
- P. Amrolia, S. G. Sullivan, A. Stern and R. Munday, J. Appl. Toxicol., 1989, 9, 113–118 CrossRef CAS PubMed.
- T. R. Juneja, R. L. Gupta and S. Samanta, Toxicol. Lett., 1984, 21, 185–189 CrossRef CAS PubMed.
- P. V. R. Snelgrove, Bioscience, 1999, 49, 129–138 CrossRef.
- Y. Sun, Z. Lv, Z. Sun, C. Wu, Z. Ji and J. You, Anal. Bioanal. Chem., 2016, 408, 3527–3536 CrossRef CAS PubMed.
- K. Beiner, P. Popp and R. Wennrich, J. Chromatogr. A, 2002, 968, 171–176 CrossRef CAS PubMed.
- D. R. Dreyer, H.-P. Jia, A. D. Todd, J. Geng and C. W. Bielawski, Org. Biomol. Chem., 2011, 9, 7292–7295 RSC.
- H. Li, J. Fan, J. Wang, M. Tian, J. Du, S. Sun, P. Sun and X. Peng, Chem. Commun., 2009, 5904–5906, 10.1039/b907511a.
- O. Pluchery, C. Humbert, M. Valamanesh, E. Lacaze and B. Busson, Phys. Chem. Chem. Phys., 2009, 11, 7729–7737 RSC.
- H. Liu, C. Guo, S. Guo, J. Fan, L. Wang and D. Shi, Talanta, 2019, 201, 301–308 CrossRef CAS PubMed.
- F. Wu, H. Wang, J. Xu, H.-Q. Yuan, L. Zeng and G.-M. Bao, Sens. Actuators, B, 2018, 254, 21–29 CrossRef CAS.
- Y. Zhang, Y. Hao, X. Ma, S. Chen and M. Xu, Environ. Pollut., 2020, 265, 114958 CrossRef CAS PubMed.
- S. Chen, H. Li and P. Hou, Anal. Chim. Acta, 2017, 993, 63–70 CrossRef CAS PubMed.
- X. Xie, M. Li, F. Tang, Y. Li, L. Zhang, X. Jiao, X. Wang and B. Tang, Anal. Chem., 2017, 89, 3015–3020 CrossRef CAS PubMed.
- L. Xiong, J. Ma, Y. Huang, Z. Wang and Z. Lu, ACS Sens., 2017, 2, 599–605 CrossRef CAS PubMed.
- L. Dong, G.-R. Chen, X.-P. He and S. Vidal, Org. Biomol. Chem., 2019, 17, 9251–9256 RSC.
- J. Gai, C. Chen, J. Huang, J. Sheng, W. Chen and X. Song, Tetrahedron Lett., 2020, 61, 152038 CrossRef CAS.
- Y. Geng, H. Tian, L. Yang, X. Liu and X. Song, Sens. Actuators, B, 2018, 273, 1670–1675 CrossRef CAS.
- Y. Guo, D. Zhang, J. Wang, H. Lu and S. Pu, Dyes Pigm., 2020, 175, 108191 CrossRef CAS.
- K.-R. Kim, H. J. Kim and J.-I. Hong, Anal. Chem., 2019, 91, 1353–1359 CrossRef CAS PubMed.
- J. Niu, Y. Li, R. Zhang, J. Zhang, X. Wang, X. Xie, X. Jiao and B. Tang, Chem. Commun., 2021, 57, 2800–2803 RSC.
- T. Yamamoto, K. Sugiyama, T. Kushida, T. Inoue and T. Kanbara, J. Am. Chem. Soc., 1996, 118, 3930–3937 CrossRef CAS.
- A. Samadi-Maybodi, R. Akhoondi and M. J. Chaichi, J. Fluoresc., 2010, 20, 671–679 CrossRef CAS PubMed.
- T. Yamamoto, Macromol. Rapid Commun., 2002, 23, 583–606 CrossRef CAS.
- W. Jiang, Q. Q. Fu, H. Y. Fan, J. Ho and W. Wang, Angew. Chem. Int. Ed., 2007, 46, 8445–8448 CrossRef CAS PubMed.
- W. Lv, Y. Chen, L. Bian and X. Chen, Talanta, 2019, 197, 204–210 CrossRef CAS PubMed.
- J. J. Wu, D. D. Su, C. Q. Qin, W. Li, J. Rodrigues, R. L. Sheng and L. T. Zeng, Talanta, 2019, 201, 111–118 CrossRef CAS PubMed.
- W. Y. Zhang, X. J. Liu, H. Y. Zhang, C. C. Feng, C. X. Liu, M. M. Yu, L. H. Wei and Z. X. Li, J. Mater. Chem. C, 2015, 3, 8248–8254 RSC.
- S. Pagidi, N. K. Kalluvettukuzhy and P. Thilagar, Langmuir, 2018, 34, 8170–8177 CrossRef CAS PubMed.
Footnote |
† Electronic supplementary information (ESI) available. See DOI: 10.1039/d1ra08605g |
|
This journal is © The Royal Society of Chemistry 2022 |
Click here to see how this site uses Cookies. View our privacy policy here.