DOI:
10.1039/D1MA01252E
(Paper)
Mater. Adv., 2022,
3, 3989-3993
CNFs from linseeds oil: a potential source of X-band microwave absorbers
Received
28th December 2021
, Accepted 16th March 2022
First published on 6th April 2022
Abstract
Carbon nano fibers (CNFs) were synthesized at high temperatures using natural linseeds oil. Microwave conductivity and return loss in X-band for thick films of randomly oriented population of CNFs have been explored for their ability as a potential microwave absorber in 8 GHz to 12 GHz range with return loss between −18 dB and −32 dB. Study of electrical resistance and shielding effectiveness (SE) shows that CNFs films also have the capability of obstructing the passage of microwave frequency with a shielding effectiveness of −29 dB to −40 dB.
1. Introduction
Nano carbon materials have attracted increasing attention due to their significant electrical and mechanical properties. These materials have potential for future applications. In recent times, Masahiro Tokita et al.1 has compared the capacitance of nano-fibrous carbon electrodes with various graphitic structures. However, Gehuan Wang et al.2 has reported silver nanowires/biopolymer films as a next generation flexible optoelectronic devices. Nowadays, the rapid development in information technology with extensive applications of electrical and electronic devices has caused environmental pollution in the form of electromagnetic wave radiation. A possible way to permit the coexistence of these devices and systems without harmful EMI is to develop new shielding and absorbing materials, which especially requires anti-electromagnetic interference coatings, self-concealing technology and microwave darkrooms3–6 with high performance and a large operating frequency band.7 In this context Qianqian Huang et al.8 has reported 3D printing technology to prepare metamaterial absorbers (MMAs) to manipulate electromagnetic wave absorption and use artificial patterning methods to optimize absorption capacity as well as absorption bandwidth. Nevertheless, Fan Wang et al.9 has showed that, the crystal structure and electromagnetic properties can be regulated systematically. Amongst the various absorbers, carbon materials with different morphologies have made a great impact as a radiation absorber.10–12 They have low weight, corrosion resistance and good conductivity as well as good thermal and chemical stability compared to other materials. Also, carbon materials can be coated on any geometrically complex body.13 Hence, Hongxia Jiang et al. and Yue Zhao et al.14,15 have studied shielding effectiveness and EMI of vapor-grown carbon nano-fibers and their composites in X-band. On the other hand, Jiabin Chen et al.16 has placed the focus on electromagnetic wave attenuation by carbon-based composites like FeIII-MOF-5-derived/carbon fiber composites (FMCFs). Usually, precursors for obtaining such desired carbon materials are various types of hydrocarbons derived from fossil fuels like methane etc.,17–19 which can be catalytically decomposed into carbon atoms in a chemical vapour deposition (CVD) unit. But these sources will be depleted in the near future. So, carbon rich natural sources like plant materials and oils are seen as a replacement for hydrocarbons. These materials can be reproduced when needed.20–23 Sharon et al.24,25 have tried to synthesize biomass derived carbon nanomaterials from different natural precursors and studied their microwave properties. Later on, attempts were made to utilize such carbon nanomaterials for giga hertz frequency applications.26–31
In furtherance of these previous research studies, we report the properties of CNFs from natural linseeds oil in X-band of microwave frequency region. Here, the precursor was selected on the basis of its efficiency to produce various forms of nano carbon with optimized parameters. Among various types of carbon nanomaterials, CNFs are selected due to some particular properties like light weight, corrosion resistance, good conductivity, low electrical resistance, large surface area because of longer length, good mechanical strength and easy fabrication as compared to other nano materials.
2. Experimental
2.1 Materials
Unpurified natural linseeds oil, also known as linaceae oil was used as a precursor. It was derived from dried ripe seeds of linseeds plant.
2.2 Sample preparation
Vapour deposition of linseeds oil was used to synthesize CNFs using CVD system (Fig. 1). This method is discussed in detail elsewhere.26 An alumina substrate of dimensions 1 × 3 cm2 were used for CNFs film deposition. A substrate were located in a quartz tube in the central hot zone of a split furnace maintained at 850 °C. Linseeds oil was kept in other zone of same tube sustained at the temperature of about 300 °C and adjacent to the hot zone. The flow rate of hydrogen as a carrier gas was adjusted to transfer vapour of 10 ml of linseeds oil in the hot zone as a reaction zone, in approximately 1 hr. Initially, oxygen from the system was removed by flushing entire assembly with hydrogen for 15 minutes. After the completion of reaction, thick films of CNFs deposited on an alumina substrate were taken out at normal temperature. Then, films were characterized by various techniques.
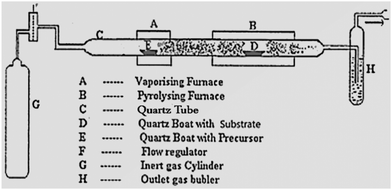 |
| Fig. 1 Schematic diagram of CVD set up. | |
2.3 Characterization
The surface morphology of films was studied using Hitachi S-4300 scanning electron microscope. A detailed study of the surface was done using JEOL-2100F field effect transmission electron microscope. Films were characterized by micro-Raman spectra using a green laser with 532 nm excitation. Van der Pauw method was used to determine the sheet resistance of films.
2.4 Microwave absorption study
Microwave conductivity, absorption and shielding effectiveness were studied using a microwave test bench in 8 GHz to 12 GHz range. Here, a silver (Ag) thick film component of width 0.635 mm was used as a simple microstrip line. This strip line component was used to characterize the microwave properties of CNFs films. Initially, the whole microstrip line component set up was calibrated without an overlay in X-band. Then, all the data was plotted as a difference in transmittance and difference in reflectance between the substrate with the CNFs film and without CNFs film. The return loss and absorption for CNFs were estimated using microwave reflectance. Transmittance of the microwaves was used to calculate the shielding effectiveness.
3. Results and Discussion
Fig. 2a shows an arbitrarily grown CNFs of length ∼40 μm with diameter ∼533 nm. The formation of these CNFs is mainly determined by breaking of C–C bonds. At this juncture, temperature plays an important role in the realization of such graphitic structures. The surface profile of fibres shows that fibres have a rough surface (Fig. 2b) which bulges at certain areas.
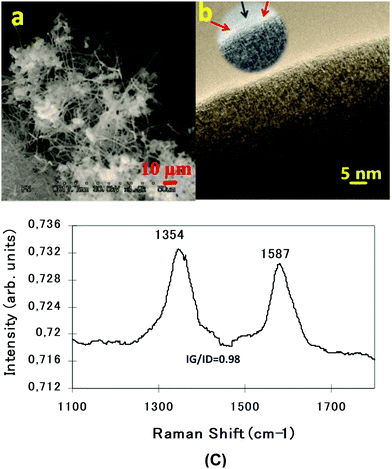 |
| Fig. 2 (a) SEM image of randomly orientated CNFs (b) TEM image of the outer surface of CNF, where the inset image shows the bulging surface, and (c) micro-Raman spectrum of CNFs film. | |
Broad peaks centred at 1354 cm−1 and 1587 cm−1 were observed in micro-Raman spectrum of CNFs films, in the range of 1100 cm−1 to 1800 cm−1 (Fig. 2c). The early peak corresponds to D-peak i.e. disordered graphite, normally observed between 1300 and 1350 cm−1 whereas, the succeeding peak corresponds to the standard graphitic G-peak commonly occurring at 1580 cm−1. A purity of CNFs films was evaluated using G-peak to D-peak intensity (IG/ID) ratio. The calculated value of this ratio was found to be 0.98, which specifies an abundance of highly ordered graphite along with a small amount of undesired carbon i.e. sp3, present in films. An amount of disorder computed for CNFs films using G-peak shift position was found to be 20.5%.
When the microwave energy is transmitted through such a material medium, the conductive surface of medium induces currents, as an electric field interacts with mobile electrons within the material. Thus, it is reported that conductivity is a key parameter in microwave frequency applications.7
Therefore, the microwave conductivity of films was calculated using an equation,
|  | (1) |
where,
σ0 is the bulk conductivity,
K =
d/
l0. Here, ‘
d’ is the film thickness and ‘
l0’ is the mean free path which is 10
−6 m for carbon nano material, and
Ei is the transmittance. Since, a thickness of thin film is in micrometer range, the skin effect can be ignored. In the measurement of microwave conductivity of CNFs film, it was placed transverse to the direction of propagation. Under such conditions the magnetic vector of the electromagnetic wave is perpendicular to the direction of propagation. Using the conductivity formula of
eqn (1) and transmittance of the bare substrate, a microwave conductivity for CNFs films was calculated.
From the microwave conductivity curve of CNFs films in X-band (Fig. 3a), the ratio of σ/σ0 for films was found in the range of 0.81 S cm−1 to 0.92 S cm−1. At this point, peaks in the conductivity curve (Fig. 3a) suggest that, the maximum absorption would be possible at these frequencies. With such an assumption, the return loss for films was measured in 8–12 GHz range. From the curve plotted for return loss and microwave absorption against frequency in Fig. 3b, it is observed that, the CNFs films have an ability to absorb X-band microwaves up to ∼80% to 99% with a return loss between −18 dB and −32 dB on overall frequency range. A maximum absorption of 95% to 99% with a return loss from −27 dB to −32 dB was seen in frequency range of 9.40 GHz to 10.40 GHz with a bandwidth of 1 GHz. Such appreciable absorption of microwaves in CNFs is because of microwave energy during its transmission through the material interacts with free charge carries present inside the material. This process dissipates microwave energy as a heat. So, more conductive material means there are more free charges available for its interaction with microwaves. This will result in the loss of microwaves inside the material. Measurement of electrical resistance using van der Pauw method shows that, CNFs films has resistance of 0.033 × 10−3 Ohm cm (Fig. 3a). A plot shows an Ohmic nature, where low resistance in CNFs films may be due to the higher concentration of sp2 bonded carbon present in films. Therefore, besides structural properties of the material, microwave loss may also come from the resistive part. A lower resistance of material may induce larger dielectric losses that can cause more absorption or transmission of microwave frequencies. In addition, multiple internal reflections from randomly grown CNFs are also responsible for increased absorption of microwaves. Such reflections give rise to a series of emergent waves. This causes an absorption by destructive interference.
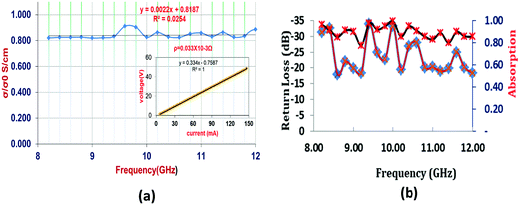 |
| Fig. 3 (a) Microwave conductivity against frequency along with electrical resistance plot for CNFs film (b) Microwave absorption and return loss vs. frequency curve for CNFs film. | |
Furthermore, the microwave wave absorption properties of material can be tuned by optimizing the length, diameter and conductivity of CNFs.
The ability of CNFs to block the passage of microwaves was confirmed by shielding effectiveness (SE) study of films in X-band.
Here, the effectiveness of shielding microwaves was calculated using eqn (2),
| SE = −20 log−T | (2) |
where
T is the electromagnetic radiation transmittance.
A plot of shielding effectiveness vs. frequency (Fig. 4), shows that CNFs films have potential to block the passage of microwaves through the system with SE between −29 dB and −40 dB over whole X-band frequency range.
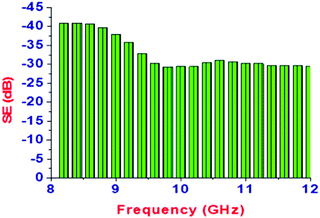 |
| Fig. 4 Shielding effectiveness vs. frequency plot for CNFs. | |
4. Summary
Carbon nano fibers were synthesized using natural linseeds oil as a precursor at 850 °C. The randomly grown population of nanofibers was found with an almost uniform diameter of ∼533 nm and length ∼40 μm. An electrical resistance and microwave conductivity study indicates that the as prepared CNFs films have good microwave conductivity between 0.82 S cm−1 and 1.05 S cm−1 in 8 GHz to12 GHz frequency range. SE between −29 dB and −40 dB over the whole frequency range showed the capability of CNFs films to block the passage of microwaves through the system. These results support the possible use of CNFs films obtained from linseeds oil for applications in communication, space, remote sensing etc. as a microwave absorber as well as an SE material.
Conflicts of interest
The authors have certify that they have no affiliations with or involvement in any organization or entity with any financial interest (such as honoraria; educational grants; participation in speakers’ bureaus; membership, employment, consultancies, stock ownership, or other equity interest; and expert testimony or patent-licensing arrangements), or non-financial interest (such as personal or professional relationships, affiliations, knowledge or beliefs) in the subject matter or materials discussed in this manuscript.
Acknowledgements
The authors would like to thank Dr Naresh Chandra, Dr Avinash Patil and management authorities of B. K. Birla College, Kalyan, for their support.
References
- M. Tokita, M. Egashira, N. Yoshimoto and M. Morita, The influence of graphitic structure of carbon electrode on aging behavior of electric double layer Capacitor, Electrochemistry, 2012, 80(10), 752–754 CrossRef CAS.
- G. Wang, L. Hao, X. Zhang, S. Tan and G. Ji, Flexible and transparent silver nanowires/biopolymer film for high-efficient electromagnetic interference shielding, J. Colloid Interface Sci., 2022, 607(1), 89–99 CrossRef CAS PubMed.
- G. Li, G. G. Hu, H. D. Zhou, X. J. Fan and X. G. Li, Attractive microwave-absorbing properties of La1-xSrxMnO3 manganite powders, Mater. Chem. Phys., 2002, 75, 101–104 CrossRef CAS.
- H. S. Cho and S. S. Kim, M-hexaferrites with planar magnetic anisotropy and their application to high-frequency microwave absorbers, IEEE Trans. Magn., 1999, 15, 3151–3153 Search PubMed.
- S. P. Ruan, B. K. Xu, H. Suo, F. Q. Wu, S. Q. Xiang and M. Y. Zhao, Microwave absorptive behavior of ZnCo-substi-tuted W-type Ba hexaferrite nano crystalline composite material, J. Magn. Magn. Mater., 2000, 212, 175–177 CrossRef CAS.
- M. R. Anantharaman, S. Sindhu, S. Jagatheesan, K. A. Malini and P. Kurian, Dielectric properties of rubber ferrite composites containing mixed ferrites, J. Phys. D: Appl. Phys., 1999, 32, 1801–1810 CrossRef CAS.
- A. Saib, L. Bednarz, R. Daussin, C. Bailly, L. Xudong, J.-M. Thomassin, C. Pagnoulle, C. Detrembleur, R. Jérôme and I. Huynen, Carbon nanotube composites for broadband microwave absorbing materials, IEEE Trans. Microwave Theory Tech., 2006, 54(6), 2745–2754 CAS.
- Q. Huang, G. W. M. Zhou, J. Zheng, S. Tang and G. Ji, Metamaterial electromagnetic wave absorbers and devices: Design and 3D microarchitecture, J. Mater. Sci. Technol., 2022, 108, 90–101 CrossRef.
- F. Wang, W. Gu, J. Chen, Y. Wu, M. Zhou, S. Tang, X. Cao, P. Zhang and G. Ji, The point defect and electronic structure of K doped LaCo0.9Fe0.1O3 perovskite with enhanced microwave absorbing ability, Nano Res., 2021, 85, 3955, DOI:10.1007/s12274-021-3955-1.
- M. S. Pinho and M. L. Gregori, Performance of radar absorbing materials by waveguide measurements for X- and Ku-band frequencies, Eur. Polym. J., 2002, 38(11), 2321–2327 CrossRef CAS.
- J.-H. Du and C. Sun, Microwave Electromagnetic Characteristics of a Microcoiled Carbon Fibers/paraffin Wax Composite in Ku Band, J. Mater. Res., 2002, 17(5), 1232–1236 CrossRef CAS.
- K. Kaneto and M. Tsurute, Electrical properties of carbon micro coils, Synth. Met., 1999, 103, 2578–2779 CrossRef CAS.
- S. Maheshwar, P. Debabrata, Z. Renju and P. Vijaya, Application of carbon nanomaterial as a microwave absorber, J. Nanosci. Nanotechnol., 2005, 5(12), 2117–2120 CrossRef PubMed.
- H. Jiang, Q. Ni and H. Wang, Preparation and electrical property evaluation of vapor-grown carbon nanofibers reinforced natural rubber composites, J. Ind. Text., 2013, 42(3), 340–350 CrossRef CAS.
- Y. Zhao, L. Hao, X. Zhang, S. Tan, H. Li, J. Zheng and G. Ji, A novel strategy in electromagnetic wave absorbing and shielding materials design: multi-responsive field effect, Small Sci., 2021, 2100077, 1–23 Search PubMed.
- J. Chen, J. Zheng, F. Wang, Q. Huang and G. Ji, Carbon fibers embedded with FeIII-MOF-5-derived composites for enhanced microwave absorption, Carbon, 2021, 174, 509–517 CrossRef CAS.
- A. G. Tkachev, S. V. Blinov and N. R. Memetov, Carbon nanomaterials on the base of catalytic hudrocarbon pyrolysis: Development and Perspective Use, Hydrogen Mater. Sci. Chem. Carbon Nanomater., 2007, l3, 515–519 Search PubMed.
- A. D. Rud, A. E. Perekos, V. M. Ogenko, A. P. Shpak, V. N. Uvarov, K. V. Chuistov, A. M. Lakhnik, V. Z. Voynash and L. I. Ivaschuk, Different states of carbon produced by high-energy plasmochemistry synthesis, J. Non-Cryst. Solids, 2007, 353, 3650–3654 CrossRef CAS.
- D. A. Makeiff and T. Huber, Microwave absorption by polyaniline-carbon nanotube composites, Synth. Met., 2006, 156, 497–505 CrossRef CAS.
- M. Sharon and M. Sharon, Carbon Nanomaterials and their Synthesis from Plant-Derived Precursors, Synth. React. Inorg., Met.-Org., Nano-Met. Chem., 2006, 36, 1–15 CrossRef.
- M. Sharon, Cabon Nanomaterials Encyliopedia, J. Nanosci. Nanotechnol., 2004, 1, 517–546 CAS.
- M. Kumar, X. Zhao, Y. Ando, S. Iijima, M. Sharon and K. Hirahara, Carbon nanotubes from camphor by catalytic CVD, Mol. Cryst. Liq. Cryst., 2002, 387, 341–345 Search PubMed.
- M. Sharon and M. Sharon, Carbon Nanomaterials: Applications in Physico-chemical and Bio-Systems, Def. Sci. J., 2008, 58(4), 460–485 CrossRef CAS.
- H. K. Dubey, D. E. Kshirsagar, L. P. Deshmukh, M. Sharon and M. Sharon, A New Carbon Material Synthesized from Coconut Shell, Adv. Sci., Eng. Med., 2011, 3, 1–4 CrossRef.
- M. Sharon, D. Pradhan, R. Zacharia and V. Puri, Application of carbon nanomaterials as a microwave absorber, J. Nanosci. Nanotechnol., 2005, 5(12), 2117–2120 CrossRef CAS PubMed.
- D. E. Kshirsagar, V. Puri, M. Sharon and M. Sharon, Microwave Absorption Study of Carbon Nano Materials Synthesized from Natural Oils, Carbon: Sci., 2006, 7(4), 245–248 Search PubMed.
- D. E. Kshirsagar, V. Puri, M. Sharon and M. Sharon, Electromagnetic Wave-Absorbing Properties of Pongamia Glabra Based-CNMs in the 8–12 GHz Range, Synth. React. Inorg., Met.-Org., Nano-Met. Chem., 2007, 37, 477–479 CrossRef CAS.
-
M. Sharon, R. R. Vishwakarma, D. E. Kshirsagar and M. Sharon, Nanotechnology, Carbon for Microwave Absorption, Defence Application, Studium Press, LLC, Houston USA, 2013, vol. 5, ch. 12, 231–256 Search PubMed.
- J. H. Du, C. Sun, S. Bai, G. Su, Z. Ying and H. M. Cheng, Microwave electromagnetic characteristics of a microcoiled carbon fibers/paraffin wax composite in Ku band, J. Mater. Res., 2002, 17, 1232–1236 CrossRef CAS.
- S. K. Kwon, J. M. Ahn, G. H. Kim, C. H. Chun, J. S. Hwang and J. H. Lee, Microwave absorbing properties of carbon black/silicone rubber blend, Polym. Eng. Sci., 2002, 42, 2165–2171 CrossRef CAS.
- D. E. Kshirsagar, V. Puri, H. Dubey and M. Sharon, Giga hertz frequency absorber carbon nano fibers synthesized using linseed oil, Mater. Today Commun., 2017, 13, 23–25 CrossRef CAS.
|
This journal is © The Royal Society of Chemistry 2022 |
Click here to see how this site uses Cookies. View our privacy policy here.