DOI:
10.1039/D1RA04013H
(Paper)
RSC Adv., 2021,
11, 26666-26671
Transition-metal-free synthesis of aryl 1-thioglycosides with arynes at room temperature†
Received
23rd May 2021
, Accepted 22nd July 2021
First published on 4th August 2021
Abstract
A mild, convenient and transition-metal-free protocol for the synthesis of aryl 1-thioglycosides is presented via arynes generated in situ combined with glycosyl thiols in the presence of TBAF(tBuOH)4. The methodology provides a general and efficient way to prepare a series of functionalized thioglycosides in good to excellent yields with a perfect control of the anomeric configuration at room temperature. In addition, the reaction conditions tolerate a variety of the pentoses and hexoses, and the reaction also performs smoothly on protected monosaccharides and disaccharides.
Introduction
Thioglycosides, as the analogue of the O-glycosides, are versatile intermediates in carbohydrate synthesis and have a wide range of potential applications,1 and are regularly used as glycosyl donors2 to construct various oligosaccharides and glycoconjugates. Additionally, they are more stable in both chemical and enzymatic degradations3 and have also been employed extensively as inhibitors in a great number of biochemical studies.4 Thioglycoside fragments are also widely used in various drugs, natural products and pharmaceutical active agents.5 Some examples of the thioglycosides derivatives include the cytotoxic Hsp90 inhibitor,6 MUS-CB,7 hSGLT1 inhibitor,8 lincomycin,9 clindamycin,10 as well as irreversible glycoside inhibitors11 (Fig. 1). On account of the great significance of S-glycosides, some elegant synthetic protocols have been developed.
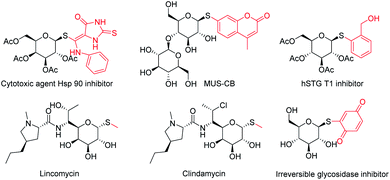 |
| Fig. 1 Examples of biologically active thioglycosides. | |
Originally, the approaches to prepare the 1-thioglycosides were that glycosyl donors reacted with sulfurs (or thiophenols) in the presence of the stoichiometric Lewis acids12 or glycosyl halides reacted with thiolate anions under strong base conditions (Scheme 1a). However, the inferior stereo-selectivity or strong base of those traditional methods limited their application. With the efforts of Sticha, Xue, Messaoudi et al., the synthesis of 1-thioglycosides achieved great advances in recent years. As shown in Scheme 1b, the functionalization of glycosyl thiols had been catalysed by the Ni,13 Cu14, Pd15 transition metal via the Buchwald–Hartwlg–Migita coupling reaction. These methods required an expensive catalyst or a high temperature (above 100 °C in some protocols of the Cu or Pd catalyst) and long reaction time, which limit the universality of the reaction to some extent. In 2019, Messaoudi and co-workers developed a protocol for the synthesis of aryl 1-thioglycosides via a Ni/photoredox dual catalyzed cross-coupling reaction16 (Scheme 1c). Recently, Messaoudi's group described the first electrochemical method for coupling various anomeric glycosyl thiols with aryl bromides17 (Scheme 1c). The reaction didn't need to perform in a strong base environment, and demonstrated superiority to synthesize highly complex thioglycosides under mild conditions. However, some functional groups are not compatible with this procedure.
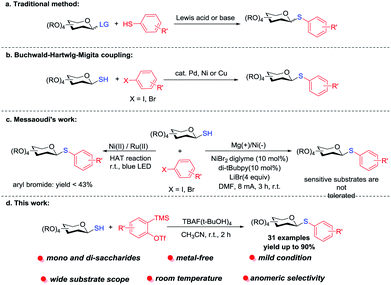 |
| Scheme 1 Methods for the synthesis of S-glycosides. | |
Arynes are highly reactive transient intermediates and are also useful synthons in organic synthesis due to the unique triple bonds, and have been extensively used in the synthesis of natural products, drug molecules and functional materials.18 Arynes have made remarkable achievements in nucleophilic reactions,19 pericyclic reactions,20 transition metal catalyzed reactions21 and multicomponent reactions.22 In recent years, N-arylation of carbohydrate amines23 and O-arylation of carbohydrates24 have been reported via the aryne insertion reactions with glycosyl thiols. The methodology of using the aryne generated in situ to realize the functionalized thioglycosides was not developed yet. On the basis of our previous research in carbohydrates,25 we report a novel protocol for the preparation of thioglycosides via a tandem intermolecular nucleophilic coupling of glycosyl thiols with arynes (Scheme 1c).
Results and discussion
We started by optimizing the reaction conditions with tetra-O-acetylated 1-thio-β-D-glucopyranose 1a (1.0 equiv.), o-(trimethylsilyl)aryl triflates 2a (1.0 equiv.) as model substrate. Phenyl-2,3,4,6-tetra-O-acetyl-1-thio-β-D-glucopyranoside 3a was isolated after 2 hours in 64% yield at room temperature (entry 1, Table 1). When the equivalent of aryne precursor increased to 1.1 equiv., the yield of desired product increased to 70% (entry 1, Table 1). In spired by this result, other fluorides, such as AgF, ZnF2, KF, more widely used tetrabutyl ammonium fluoride (TBAF) and its analogs (entries 2–7, Table 1) were tested. To our delight, the yield of 3a increased to 86% when TBAF·(tBuOH)4 (ref. 26) was used as a fluorine source (entry 7, Table 1). Next, we also investigated the solvent effect by using varies polar solvents and non-polar solvents (entries 8–13, Table 1). Nucleophilic solvents such as tetrahydrofuran, methanol, N,N-dimethylformamide and dimethyl sulfoxide exhibited low yields (26–40%), probably because the competitive reaction between nucleophilic solvents and the substrates. The yield of 3a was decreased to 60% when the temperature increased to 60 °C (entry 15). We found that the yield of the target product was not influenced with extended reaction time.
Table 1 Optimization conditions for the synthesis of S-glycosidesa

|
Entry |
F− sources |
Solvent |
Time/h |
T/°C |
Yield (%) |
Standard conditions: 1a (0.1 mmol, 1.0 equiv.), 2a (0.11 mmol, 1.1 equiv.), TBAF·(tBuOH)4 (0.2 mmol, 2.0 equiv.), CH3CN (1.5 mL) as solvent, r.t., 2 h. 1a (0.1 mmol, 1.0 equiv.), 2a (0.1 mmol, 1.0 equiv.), F− source (0.2 mmol, 2.0 equiv.), CH3CN (1.5 mL). 1a (0.1 mmol, 1.0 equiv.), 2a (0.11 mmol, 1.1 equiv.), TBAF·(tBuOH)4 (0.3 mmol, 3.0 equiv.), CH3CN (1.5 mL) as solvent, r.t., 2 h. |
1 |
CsF |
CH3CN |
2.0 |
25 |
70(64b) |
2 |
AgF |
CH3CN |
2.0 |
25 |
51 |
3 |
KF |
CH3CN |
2.0 |
25 |
42 |
4 |
ZnF2 |
CH3CN |
2.0 |
25 |
35 |
5 |
TBAF·3H2O |
CH3CN |
2.0 |
25 |
63 |
6 |
TBAF(THF) |
CH3CN |
2.0 |
25 |
67 |
7 |
TBAF·(tBuOH)4 |
CH3CN |
2.0 |
25 |
86 |
8 |
TBAF·(tBuOH)4 |
DCM |
2.0 |
25 |
78 |
9 |
TBAF·(tBuOH)4 |
THF |
2.0 |
25 |
40 |
10 |
TBAF·(tBuOH)4 |
Toluene |
2.0 |
25 |
33 |
11 |
TBAF·(tBuOH)4 |
MeOH |
2.0 |
25 |
37 |
12 |
TBAF·(tBuOH)4 |
DMSO |
2.0 |
25 |
26 |
13 |
TBAF·(tBuOH)4 |
DMF |
2.0 |
25 |
30 |
14 |
TBAF·(tBuOH)4 |
CH3CN |
2.0 |
40 |
85 |
15 |
TBAF·(tBuOH)4 |
CH3CN |
2.0 |
60 |
60 |
16 |
TBAF·(tBuOH)4 |
CH3CN |
3.0 |
25 |
83c |
17 |
TBAF·(tBuOH)4 |
CH3CN |
4.0 |
25 |
82 |
With the optimized condition in hand, the scopes of the glycosyl thiols were further investigated and the results are presented in Scheme 2. Various acetyl-protected β-glycosyl thiols 1c, 1d reacted with arynes under the standard conditions and the corresponding β-configuration thioglycosides products (3c, 3d) were obtained in good yields (85%, 75%). This conversion was also applicable for the α-configuration glycosyl thiol substrate 1b and 1e, providing corresponding arylthioglycoside products 3b and 3e in 80% and 77% yield, respectively. Moreover, triacetyl protected-pentose glycosyl thiols 1f, 1g participated in this reaction smoothly and the yields of the corresponding thioglycoside products were 82% and 81%, respectively. The reaction was not limited to monosaccharides but also extended to disaccharides. Acetyl-protected cellobiose 1h and benzoyl-protected cellobiose 1i were reacted with arynes successfully and obtained the β-disaccharide in good yields, indicated the electronic-effect of the protecting groups did not have significant influence for the reaction efficiency. Meanwhile, we also found the α-glycosyl thiols with OBn protected group can reacted with 2a when the temperature improved to 45 °C and the yield of corresponding product 3j was 78%. The sugar 1k with OPiv group provided 3k in 71% yield under optical condition. However, the reactivity of unprotected glycosyl thiol was decreased and the yield of product was only 30%.
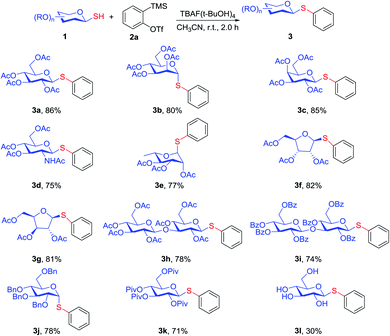 |
| Scheme 2 Scopes of glycosyl thiols 1 reacted with aryne 2a. Standard conditions: 1 (0.1 mmol, 1.0 equiv.), 2a (0.11 mmol, 1.1 equiv.), TBAF·(tBuOH)4 (0.2 mmol, 2.0 equiv.), dry CH3CN (1.5 mL). | |
Subsequently, we also investigated the electronic effects and regioselectivity of this reaction via different aryne precursors and glycosyl thiols 1. Regardless of the electron-donating or -withdrawing properties of the symmetrical aryne precursors 2, the corresponding products were obtained in good yields. The difluoro substituted aryne 2b reacted with 1 gave the thio-glycosides derivatives 4a–4h in 71–82% yields. Aryne precursor 2c reacted with a series of glycosyl thiols under standard reaction condition to afford the corresponding products 4i–4l in good yields (72–84%). Also, the dimethyl substituted aryne precursors reacted with 1a under standard condition to give corresponding aryl 1-thioglycoside 4m with 78% yield.
Meanwhile, 3-methoxy non-symmetric aryne precursor 2d exhibited excellent regioselectivity due to the steric effect and electronic effects, and single target thio-glycosides 4n, 4o were obtained in good yields (80%, 78%). However, we found that 4-methylbenzyne precursor 2e reacted with 1a and 1f gave a near equimolar mixture of two inseparable regioisomers, providing corresponding products in 82% and 90% yield, respectively. 1,2-Naphthyne precursor 2f under the same conditions also provided a near equimolar mixture of two inseparable regioisomers (Scheme 3).
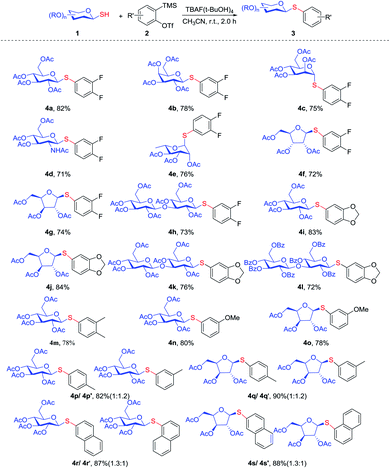 |
| Scheme 3 Scope of aryne precursors 2 reacted with glycosyl thiol. Standard condition: 1 (0.1 mmol, 1.0 equiv.), 2 (0.11 mmol, 1.1 equiv.), TBAF·(tBuOH)4 (0.2 mmol, 2.0 equiv.), dry CH3CN (1.5 mL). | |
To demonstrate the synthetic utility of this transformation, we next performed a scale-up reaction of 3a. As shown in Scheme 4a, a gram scale reaction of 1-thio-β-D-glucopyranose 1a with aryne precursor 2a proceeded to give 3a in 85% yield. A plausible mechanism was proposed in Scheme 4b, based on the basis of the experimental results and the related report by Jin and co-workers.27
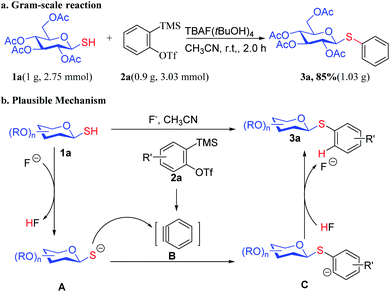 |
| Scheme 4 Gram-scale reaction and plausible mechanism. | |
Conclusions
In conclusion, we have developed a convenient method for the preparation of aryl-thioglycosides under mild and metal-catalyst-free conditions via a tandem intermolecular nucleophilic coupling of glycosyl thiols with arynes. Meanwhile, the method is applicable for various monosaccharide and disaccharide substrates, which has wide practical value between biochemistry and medicinal chemistry. In addition, 1,2-trans-thioglycosides were stereoselectively formed by the reaction of the in situ generated arynes with glycosyl thiols in good to excellent yields. Importantly, we provided a new protocol for the synthesis of functionalized thioglycosides and the possibility for further derivatization of glycosyl donors.
Experimental
General information
All reactions were carried out in dried glassware. The solvent in the reaction were dried use activated 4 Å molecular sieve, commercial reagents were used without further purification unless otherwise stated. Purification of reaction products were carried out by flash chromatography on silica gel (200–300 mesh). NMR spectra were measured in CDCl3 (with TMS as internal standard) on a Bruker AV400 (1H at 400 MHz, 13C at 100 MHz, 19F at 376 MHz) magnetic resonance spectrometer.
The general procedure for the reaction of glycosyl thiols 1 with aryne precursors 2
The glycosyl thiol 1 (50 mg, 0.14 mmol, 1.0 equiv.), aryne precursor 2 (45 mg, 0.150 mmol, 1.1 equiv.) and TBAF·(tBuOH)4 (0.274 mmol, 2.0 equiv.) were sequentially added in a clean and dry Schlenk tube, and the tube was then evacuated and backfilled with nitrogen (this sequence was repeated three times). Under nitrogen atmosphere, MeCN (1.5 mL) was added to the mixture system, then the mixture was stirred at room temperature for 2.0 hours. Saturated NaCl solution was added to dilute the system and extracted with EtOAc (3 × 2 mL). The combined organic phase was dried over anhydrous Na2SO4, filtered, and evaporated in vacuo. Finally, the crude product was purified via flash column chromatography on silica gel to give the desired product.
Phenyl-2,3,5-tri-O-acetyl-1-thio-β-D-xylofuranoside (3g)
Purified by flash column chromatography Rf = 0.39 (petroleum ether/AcOEt = 3
:
1), white solid (29.8 mg, 81% yield); [α]25D = −55.4 (c = 1.0, CHCl3); 1H NMR (400 MHz, CDCl3) δ 7.55–7.46 (m, 2H, ArH), 7.40–7.28 (m, 3H, ArH), 5.18 (t, J = 8.2 Hz, 1H), 5.06–4.87 (m, 2H), 4.80 (d, J = 8.4 Hz, 1H), 4.28 (dd, J = 11.8, 4.9 Hz, 1H), 3.42 (dd, J = 11.8, 8.7 Hz, 1H), 2.09 (s, 3H), 2.05 (s, 3H), 2.04 (s, 3H); 13C NMR (100 MHz, CDCl3) δ 169.9, 169.8, 169.3, 132.7, 132.2, 129.1, 128.3, 86.2, 71.9, 69.8, 68.4, 65.2, 20.8, 20.8, 20.7; HRMS (ESI) m/z calcd for C17H20O7SNa+ (M + Na)+ 391.0822, found 391.0822.
3,4-Difluorphenyl-2,3,4,6-tetra-O-acetyl-1-thio-β-D-gluco-pyranoside (4a)
Purified by flash column chromatography Rf = 0.30 (petroleum ether/AcOEt = 3
:
1), white solid (39.1 mg, 82% yield); [α]25D = −20.7 (c = 1.0, CHCl3); 1H NMR (400 MHz, CDCl3) δ 7.42 (ddd, J = 10.2, 7.5, 2.2 Hz, 1H), 7.24–7.19 (m, 1H), 7.10 (dt, J = 10.0, 8.3 Hz, 1H), 5.21 (t, J = 9.4 Hz, 1H), 5.00 (t, J = 9.8 Hz, 1H), 4.90 (t, J = 9.6 Hz, 1H), 4.63 (d, J = 10.0 Hz, 1H), 4.19 (d, J = 3.8 Hz, 2H), 3.73 (dt, J = 10.1, 3.8 Hz, 1H), 2.09 (s, 3H), 2.08 (s, 3H), 2.01 (s, 3H), 1.98 (s, 3H); 19F {1H} NMR (376 MHz, CDCl3) δ −135.81 (dt, J = 19.7, 9.3 Hz), −136.61 (m); 19F NMR (376 MHz, CDCl3) δ −135.81 (d, J = 20.9 Hz), −136.61 (d, J = 21.0 Hz). 13C NMR (100 MHz, CDCl3) δ 170.6, 170.1, 169.3, 169.2, 150.9 (dd, J = 251.0, 12.3 Hz), 149.9 (dd, J = 251.1, 12.5 Hz), 130.3 (dd, J = 6.4, 3.6 Hz), 127.1 (dd, J = 6.2, 4.2 Hz), 122.9 (d, J = 18.2 Hz), 117.5 (d, J = 17.6 Hz), 85.1, 75.9, 73.8, 69.6, 68.0, 62.0, 20.7, 20.6, 20.5; HRMS (ESI): m/z calcd for C20H22F2O9SNa+ (M + Na)+ 499.0845, found 499.0843.
3,4-Difluorphenyl-2,3,4,6-tetra-O-acetyl-1-thio-β-D-galacto-pyranoside (4b)
Purified by flash column chromatography Rf = 0.28 (petroleum ether/AcOEt = 3
:
1), colorless oil (37.1 mg, 78% yield); [α]25D = 6.8 (c = 1.0, CHCl3); 1H NMR (400 MHz, CDCl3) δ 7.47 (ddd, J = 10.2, 7.5, 2.2 Hz, 1H), 7.24–7.19 (m, 1H), 7.11 (dt, J = 10.0, 8.4 Hz, 1H), 5.41 (dd, J = 3.3, 1.1 Hz, 1H), 5.18 (dd, J = 9.9 Hz, 1H), 5.03 (dd, J = 9.9, 3.3 Hz, 1H), 4.64 (d, J = 9.9 Hz, 1H), 4.20–4.09 (m, 2H), 3.95 (td, J = 5.8, 2.9 Hz, 1H), 2.11 (s, 3H), 2.09 (s, 3H), 2.06 (s, 3H), 1.96 (s, 3H); 13C NMR (100 MHz, CDCl3) δ 169.4, 169.1, 168.9, 168.3, 149.6 (dd, J = 250.8, 12.5 Hz), 148.9 (dd, J = 250.9, 13.0 Hz), 128.5 (dd, J = 6.2, 3.7 Hz), 127.1 (dd, J = 6.1, 4.2 Hz), 121.1 (d, J = 18.3 Hz), 116.4 (d, J = 17.7 Hz), 84.9, 73.6, 70.8, 66.2, 65.9, 60.8, 19.8, 19.6, 19.5, 19.5; 19F {1H} NMR (376 MHz, CDCl3) δ −135.92 (dt, J = 19.8, 9.3 Hz), −137.16 (m); 19F NMR (376 MHz, CDCl3) δ −135.92 (d, J = 21.0 Hz), −137.17 (d, J = 20.9 Hz). HRMS (ESI): m/z calcd for C20H22F2O9SNa+ (M + Na)+ 499.0845, found 499.0841.
3,4-Difluorphenyl-2-acetamido-3,4,6-tri-O-acetyl-2-deoxy-1-thio-β-D-glucopyranoside (4d)
Purified by flash column chromatography Rf = 0.28 (petroleum ether/AcOEt = 1
:
2), white solid (33.6 mg, 71% yield); [α]25D = −24.5 (c = 1.0, CHCl3); 1H NMR (400 MHz, CDCl3) δ 7.43 (ddd, J = 10.0, 7.4, 2.1 Hz, 1H), 7.25–7.19 (m, 1H), 7.09 (dt, J = 10.1, 8.4 Hz, 1H), 5.76 (d, J = 9.1 Hz, 1H), 5.19 (t, J = 9.8 Hz, 1H), 5.02 (t, J = 9.7 Hz, 1H), 4.77 (d, J = 10.4 Hz, 1H), 4.21–4.13 (m, 2H), 4.00 (q, J = 9.8 Hz, 1H), 3.77–3.67 (m, 1H), 2.08 (s, 3H), 2.02 (s, 6H), 1.98 (s, 3H); 13C NMR (100 MHz, CDCl3) δ 171.0, 170.6, 170.1, 169.3, 150.6 (dd, J = 251.0, 12.6 Hz), 149.9 (dd, J = 250.9, 12.8 Hz), 129.6 (dd, J = 6.3, 3.6 Hz), 128.2 (dd, J = 6.3, 4.2 Hz), 122.1 (d, J = 18.2 Hz), 117.5 (d, J = 17.5 Hz), 86.4, 75.9, 73.5, 68.2, 62.3, 53.2, 23.3, 20.6, 20.6, 20.5; 19F {1H} NMR (376 MHz, CDCl3) δ −135.93 (dt, J = 19.9, 9.3 Hz), −137.28 (m); 19F NMR (376 MHz, CDCl3) δ −135.93 (d, J = 21.4 Hz), −137.29 (d, J = 21.0 Hz). HRMS (ESI): m/z calcd for C20H23F2NO8SNa+ (M + Na)+ 498.1005, found 498.1006.
3,4-Difluorphenyl-2,3,4-tri-O-acetyl-1-thio-α-L-rhamno-pyranoside (4e)
Purified by flash column chromatography Rf = 0.47 (petroleum ether/AcOEt = 3
:
1), white solid (31.8 mg, 76% yield); [α]25D = −116.0 (c = 1.0, CHCl3); 1H NMR (400 MHz, CDCl3) δ 7.31 (ddd, J = 10.0, 7.3, 2.2 Hz, 1H), 7.21–7.17 (m, 1H), 7.11 (dt, J = 10.0, 8.3 Hz, 1H), 5.45 (dd, J = 3.3, 1.7 Hz, 1H), 5.35 (d, J = 1.6 Hz, 1H), 5.21 (dd, J = 10.1, 3.2 Hz, 1H), 5.14 (t, J = 9.8 Hz, 1H), 4.34–4.26 (m, 1H), 2.14 (s, 3H), 2.07 (s, 3H), 2.01 (s, 3H), 1.25 (d, J = 6.2 Hz, 3H); 13C NMR (100 MHz, CDCl3) δ 170.1, 170.0, 170.4, 150.5 (dd, J = 250.5, 12.6 Hz), 150.3 (dd, J = 251.8, 13.1 Hz), 129.4 (dd, J = 6.1, 4.1 Hz), 128.6 (dd, J = 6.3, 3.7 Hz), 121.2 (d, J = 18.2 Hz), 118.0 (d, J = 17.7 Hz), 86.0, 71.1, 71.0, 69.4, 68.1, 21.0, 20.9, 20.8, 17.4; 19F {1H} NMR (376 MHz, CDCl3) δ −135.57 (dt, J = 20.9, 9.2 Hz), −137.48 (m); 19F NMR (376 MHz, CDCl3) δ −135.57 (d, J = 20.9 Hz), −137.50 (d, J = 21.0 Hz). HRMS (ESI): m/z calcd for C18H20F2O7SNa+ (M + Na)+ 441.0790, found 441.0795.
3,4-Difluorophenyl-2,3,5-tri-O-acetyl-1-thio-β-D-xylo-furanoside (4g)
Purified by flash column chromatography Rf = 0.38 (petroleum ether/AcOEt = 3
:
1), white solid (29.9 mg, 74% yield); [α]25D = −56.9 (c = 0.5, CHCl3); 1H NMR (400 MHz, CDCl3) δ 7.35 (ddd, J = 10.0, 7.4, 2.2 Hz, 1H), 7.24–7.19 (m, 1H), 7.11 (dt, J = 10.1, 8.3 Hz, 1H), 5.17 (t, J = 8.1 Hz, 1H), 4.94–4.85 (m, 2H), 4.73 (d, J = 8.2 Hz, 1H), 4.27 (dd, J = 11.8, 4.9 Hz, 1H), 3.43 (dd, J = 11.8, 8.6 Hz, 1H), 2.10 (s, 3H), 2.04 (s, 6H); 13C NMR (100 MHz, CDCl3) δ 170.0, 169.9, 169.5, 150.9 (dd, J = 250.9, 12.5 Hz), 150.1 (dd, J = 251.7, 12.9 Hz), 130.1 (dd, J = 5.9, 3.8 Hz), 128.0–127.7 (m), 122.6 (d, J = 18.0 Hz), 117.8 (d, J = 17.6 Hz), 85.9, 71.8, 69.7, 68.3, 65.3, 20.9, 20.9, 20.8; 19F {1H} NMR (376 MHz, CDCl3) δ −135.81 (dt, J = 21.3, 9.2 Hz), −136.89 (m); 19F NMR (376 MHz, CDCl3) δ −135.81 (d, J = 21.5 Hz), −136.90 (d, J = 21.4 Hz). HRMS (ESI): m/z calcd for C17H18F2O7SNa+ (M + Na)+ 427.0634, found 427.0633.
6-Benzo[d][1,3]dioxolyl-2,3,6,2′,3′,4′,6′-hepta-O-acetyl-1-thio-β-cellobioside (4k)
Purified by flash column chromatography Rf = 0.41 (petroleum ether/AcOEt = 1
:
1), white solid (58.6 mg, 76% yield); [α]25D = −24.5 (c = 0.5, CHCl3); 1H NMR (400 MHz, CDCl3) δ 7.01 (d, J = 1.8 Hz, 1H), 6.95 (dd, J = 8.0, 1.8 Hz, 1H), 6.73 (d, J = 8.0 Hz, 1H), 5.97 (s, 2H), 5.14 (dt, J = 14.9, 9.3 Hz, 2H), 5.05 (t, J = 9.6 Hz, 1H), 4.90 (dd, J = 9.2, 7.9 Hz, 1H), 4.82 (t, J = 9.7 Hz, 1H), 4.58 (dd, J = 11.9, 2.0 Hz, 1H), 4.50 (dd, J = 13.5, 9.0 Hz, 2H), 4.37 (dd, J = 12.5, 4.2 Hz, 1H), 4.07 (dd, J = 11.9, 5.3 Hz, 1H), 4.01 (dd, J = 12.5, 2.3 Hz, 1H), 3.69 (t, J = 9.5 Hz, 1H), 3.65–3.56 (m, 2H), 2.12 (s, 3H), 2.08 (s, 3H), 2.06 (s, 3H), 2.01 (s, 3H), 1.99 (s, 3H), 1.99 (s, 3H), 1.97 (s, 3H); 13C NMR (100 MHz, CDCl3) δ 170.6, 170.4, 170.4, 169.9, 169.6, 169.4, 169.1, 148.6, 147.9, 128.8, 122.8, 114.8, 108.6, 101.6, 100.9, 85.8, 76.4, 73.8, 73.0, 72.1, 71.7, 70.0, 67.8, 61.9, 61.6, 20.9, 20.8, 20.7, 20.6.
Conflicts of interest
There are no conflicts to declare.
Acknowledgements
We thank the Natural Science Foundation of China (21967011) and the Natural Science Foundation of Jiangxi Province (20181BBE50004), and the State Key Laboratory of Catalytic Materials and Reaction Engineering (ZC06070008).
Notes and references
- For selected reviews for thioglycosides:
(a) R. Caraballo, M. Sakulsombat and O. Ramstrom, ChemBioChem, 2010, 11, 1600–1606 CrossRef CAS PubMed;
(b) N. Ibrahim, M. Alami and S. Messaoudi, Asian J. Org. Chem., 2018, 7, 2026–2038 CrossRef CAS;
(c) G. Lian, X. Zhang and B. Yu, Carbohydr. Res., 2015, 403, 13–22 CrossRef CAS;
(d) K. Pachamuthu and R. R. Schmidt, Chem. Rev., 2006, 106, 160–187 CrossRef CAS;
(e) A. Borbás, Chem.–Eur. J., 2020, 26, 6090–6101 CrossRef PubMed;
(f) B. Aguilera, J. Jiménez-Barbero and A. Fernández-Mayoralas, Carbohydr. Res., 1998, 308, 19–27 CrossRef CAS.
-
(a) Y. Geng, Q. Qin and X.-S. Ye, J. Org. Chem., 2012, 77, 5255–5270 CrossRef CAS PubMed;
(b) C.-C. Liu, C. Zhai, X.-J. Zheng and X.-S. Ye, ACS Chem. Biol., 2016, 11, 1702–1709 CrossRef CAS;
(c) X. Xiao, Y. Zhao, P. Shu, X. Zhao, Y. Liu, J. Sun, Q. Zhang, J. Zeng and Q. Wan, J. Am. Chem. Soc., 2016, 138, 13402–13407 CrossRef CAS;
(d) L. Yang, Q. Qin and X.-S. Ye, Asian J. Org. Chem., 2013, 2, 30–49 CrossRef CAS;
(e) L. Yang and X.-S. Ye, Carbohydr. Res., 2010, 345, 1713–1721 CrossRef CAS;
(f) N. Basu, S. Kumar Maity and R. Ghosh, RSC Adv., 2012, 2, 12661–12664 RSC;
(g) Y. Zeng, Z. Wang, D. Whitfield and X. Huang, J. Org. Chem., 2008, 73, 7952–7962 CrossRef CAS;
(h) D. Budhadev and B. Mukhopadhyay, Carbohydr. Res., 2014, 384, 51–55 CrossRef CAS.
-
(a) C. S. Rye and S. G. Withers, Carbohydr. Res., 2004, 339, 699–703 CrossRef CAS;
(b) C. Aydillo, I. Compañón, A. Avenoza, J. H. Busto, F. Corzana, J. M. Peregrina and M. M. Zurbano, J. Am. Chem. Soc., 2014, 136, 789–800 CrossRef CAS;
(c) B. B. Metaferia, B. J. Fetterolf, S. Shazad-ul-Hussan, M. Moravec, J. A. Smith, S. Ray, M.-T. Gutierrez-Lugo and C. A. Bewley, J. Med. Chem., 2007, 50, 6326–6336 CrossRef CAS;
(d) H. Driguez and J. Thiem, Top. Curr. Chem., 1997, 187, 85–116 CrossRef CAS.
-
(a) K. Pachamuthu and R. R. Schmidt, Chem. Rev., 2006, 106, 160–187 CrossRef CAS;
(b) S. Sattin and A. Bernardi, Carbohydr. Chem., 2016, 41, 1–25 CAS;
(c) K. Qin, H. Zhang, Z. Zhao and X. Chen, J. Am. Chem. Soc., 2020, 142, 9382–9388 CrossRef CAS.
-
(a) S. V. Pestova, E. S. Izmest'ev, S. A. Rubtsova, A. V. Polukeev and A. V. Kutchin, Russ. J. Org. Chem., 2018, 54, 1041–1044 CrossRef CAS;
(b) S. Biswas, C. V. Garcia De Gonzalo, L. M. Repka and W. A. van der Donk, ACS Chem. Biol., 2017, 12, 2965–2969 CrossRef CAS PubMed;
(c) T. J. Oman, J. M. Boettcher, H. Wang, X. N. Okalibe and W. A. van der Donk, Nat. Chem. Biol., 2011, 7, 78–80 CrossRef CAS PubMed.
-
(a) A. Mielczarek-Lewandowska, M. L. Hartman and M. Czyz, Apoptosis, 2020, 25, 12–28 CrossRef CAS;
(b) L. Li, N.-N. Chen, Q.-D. You and X.-L. Xu, Expert Opin. Ther. Pat., 2021, 31, 67–80 CrossRef CAS PubMed.
- B. K. Barr and R. J. Holewinski, Biochemistry, 2002, 41, 4447–4452 CrossRef CAS.
- A. Burse, F. Castaneda, W. Boland and R. K.-H. Kinne, Int. J. Med. Sci., 2007, 4, 131–139 Search PubMed.
- Z. Kamenik, S. Kadlcik, B. Radojevic, P. Jiraskova, M. Kuzma, R. Gazak, L. Najmanova, J. Kopecky and J. Janata, Chem. Sci., 2016, 7, 430–435 RSC.
- B. Keith and S. P. Graham, J. Serb. Chem. Soc., 2000, 65, 691–694 CrossRef.
- M. Schnabelrauch, A. Vasella and S. G. Withers, Helv. Chim. Acta, 1994, 77, 778–799 CrossRef CAS.
-
(a) S. K. Das, J. Roy, K. A. Reddy and C. Abbineni, Carbohydr. Res., 2003, 338, 2237–2240 CrossRef CAS;
(b) S. Escopy, Y. Singh and A. V. Demchenko, Org. Biomol. Chem., 2019, 17, 8379–8383 RSC.
- E. Brachet, J. D. Brion, M. Alami and S. Messaoudi, Chem.–Eur. J., 2013, 19, 15276–15280 CrossRef CAS.
-
(a) A. Chabrier, A. Bruneau, S. Benmahdjoub, B. Benmerad, S. Belaid, J. D. Brion, M. Alami and S. Messaoudi, Chem.–Eur. J., 2016, 22, 15006–15010 CrossRef CAS;
(b) P. Nauš, L. Lešetický, S. Smrček, I. Tišlerová and M. Štícha, Synlett, 2003, 2117–2122 CrossRef;
(c) X. Yuan, Y. Kou, L. Yu, Z.-X. Zhang and W. Xue, Org. Chem. Front., 2015, 2, 1604–1607 RSC;
(d) F. Zhu, E. Miller, S. Q. Zhang, D. Yi, S. O'Neill, X. Hong and M. A. Walczak, J. Am. Chem. Soc., 2018, 140, 18140–18150 CrossRef CAS PubMed.
-
(a) R. A. A. Al-Shuaeeb, D. Montoir, M. Alami and S. Messaoudi, J. Org. Chem., 2017, 82, 6720–6728 CrossRef CAS;
(b) S. Benmahdjoub, N. Ibrahim, B. Benmerad, M. Alami and S. Messaoudi, Org. Lett., 2018, 20, 4067–4071 CrossRef CAS PubMed;
(c) E. Brachet, J.-D. Brion, S. Messaoudi and M. Alami, Adv. Synth. Catal., 2013, 355, 477–490 CrossRef CAS;
(d) A. L.-S. RA, G. Galvani, G. Bernadat, J. D. Brion, M. Alami and S. Messaoudi, Org. Biomol. Chem., 2015, 13, 10904–10916 RSC.
- M. Zhu, G. Dagousset, M. Alami, E. Magnier and S. Messaoudi, Org. Lett., 2019, 21, 5132–5137 CrossRef CAS PubMed.
- M. Zhu, M. Alami and S. Messaoudi, Chem. Commun., 2020, 56, 4464–4467 RSC.
- For selected reviews of arynes:
(a) V. P. Boyarskiy, N. A. Bokach, K. V. Luzyanin and V. Y. Kukushkin, Chem. Rev., 2015, 115, 2698–2779 CrossRef CAS;
(b) A. V. M. Dubrovskiy, N. A. Markina and R. C. Larock, Org. Biomol. Chem., 2013, 11, 191–218 RSC;
(c) C. M. Gampe and E. M. Carreira, Angew. Chem., Int. Ed., 2012, 51, 3766–3778 CrossRef CAS;
(d) M. Giustiniano, A. Basso, V. Mercalli, A. Massarotti, E. Novellino, G. C. Tron and J. Zhu, Chem. Soc. Rev., 2017, 46, 1295–1357 RSC;
(e) J. He, D. Qiu and Y. Li, Acc. Chem. Res., 2020, 53, 508–519 CrossRef CAS;
(f) P. M. Tadross and B. M. Stoltz, Chem. Rev., 2012, 112, 3550–3577 CrossRef CAS;
(g) H. H. Wenk, M. Winkler and W. Sander, Angew. Chem., Int. Ed., 2003, 42, 502–528 CrossRef CAS;
(h) E. Yoshioka and H. Miyabe, Tetrahedron, 2012, 68, 179–189 CrossRef CAS;
(i) J. Shi, Y. Li and Y. Li, Chem. Soc. Rev., 2017, 46, 1707–1719 RSC;
(j) I. Pozo, E. Guitián, D. Pérez and D. Peña, Acc. Chem. Res., 2019, 52, 2472–2481 CrossRef CAS;
(k) J. Shi, L. Li and Y. Li, Chem. Rev., 2021, 121, 3892–4044 CrossRef CAS PubMed;
(l) D. B. Werz and A. T. Biju, Angew. Chem., Int. Ed., 2020, 59, 3385–3398 CrossRef CAS.
-
(a) H. Yoshida, Comprehensive Organic Synthesis II, ed. P. Knochel, Elsevier, Amsterdam, 2nd edn, 2014, pp. 517–579 Search PubMed;
(b) S. Ghorai and D. Lee, Org. Lett., 2019, 21, 7390–7393 CrossRef CAS;
(c) Y. Sumida, T. Sumida, D. Hashizume and T. Hosoya, Org. Lett., 2016, 18, 5600–5603 CrossRef CAS;
(d) K. N. Singh, P. Singh, M. Kaur and E. Sharma, ChemistrySelect, 2017, 2, 2213–2218 CrossRef CAS.
-
(a) A. Criado, D. Peña, A. Cobas and E. Guitián, Chem.–Eur. J., 2010, 16, 9736–9740 CrossRef CAS;
(b) T. Hosoya, T. Hamura, Y. Kuriyama, M. Miyamoto, T. Matsumoto and K. Suzuki, Synlett, 2000, 520–522 CAS;
(c) J. Zhang, A. C. S. Page, V. Palani, J. Chen and T. R. Hoye, Org. Lett., 2018, 20, 5550–5553 CrossRef CAS PubMed;
(d) C.-D. Wang and R.-S. Liu, Org. Biomol. Chem., 2012, 10, 8948–8952 RSC;
(e) M. Sarmah, A. Sharma and P. Gogoi, Org. Biomol. Chem., 2021, 19, 722–737 RSC.
-
(a) S. Cacchi, G. Fabrizi and A. Goggiamani, Org. Biomol. Chem., 2011, 9, 641–652 RSC;
(b) R. Karmakar and D. Lee, Chem. Soc. Rev., 2016, 45, 4459–4470 RSC;
(c) R. A. Dhokale and S. B. Mhaske, Synthesis, 2018, 50, 1–16 CrossRef CAS;
(d) H. Tanaka, H. Kuriki, T. Kubo, I. Osaka and H. Yoshida, Chem. Commun., 2019, 55, 6503–6506 RSC;
(e) M. Feng and X. Jiang, Synthesis, 2017, 49, 4414–4433 CAS.
-
(a) H. Yoshida, Y. Ito and J. Ohshita, Chem. Commun., 2011, 47, 8512–8514 RSC;
(b) S. Ghorai and D. Lee, Synlett, 2020, 31, 750–771 CrossRef CAS.
- K. B. Pal, M. Mahanti and U. J. Nilsson, Org. Lett., 2018, 20, 616–619 CrossRef CAS PubMed.
- M. Bhardwaj, N. Hussain, I. A. Zargar, A. K. Dash and D. Mukherjee, Org. Biomol. Chem., 2020, 18, 4174–4177 RSC.
- N. Yan, Z.-W. Lei, J.-K. Su, W.-L. Liao and X.-G. Hu, Chin. Chem. Lett., 2017, 28, 467–470 CrossRef CAS.
- D. W. Kim, H.-J. Jeong, S. T. Lim and M.-H. Sohn, Angew. Chem., Int. Ed., 2008, 47, 8404–8406 CrossRef CAS.
- L. Zhang, Y. Geng and Z. Jin, J. Org. Chem., 2016, 81, 3542–3552 CrossRef CAS.
Footnotes |
† Electronic supplementary information (ESI) available: Additional experimental details and complete NMR spectral data for all synthesized compounds. See DOI: 10.1039/d1ra04013h |
‡ These authors contribute equally. |
|
This journal is © The Royal Society of Chemistry 2021 |
Click here to see how this site uses Cookies. View our privacy policy here.