DOI:
10.1039/D0RA04636A
(Paper)
RSC Adv., 2020,
10, 29257-29262
Transition-metal and oxidant-free approach for the synthesis of diverse N-heterocycles by TMSCl activation of isocyanides†
Received
26th May 2020
, Accepted 7th July 2020
First published on 7th August 2020
Abstract
A highly efficient TMSCl-mediated addition of N-nucleophiles to isocyanides has been achieved. This transition-metal and oxidant-free strategy has been applied to the construction of various N-heterocyles such as quinazolinone, benzimidazole and benzothiazole derivatives by the use of distinct amino-based binucleophiles. The notable feature of this protocol includes its mild reaction condition, broad functional group tolerance and excellent yield.
In the past decades, isocyanides have proved themselves to be irreplaceable structural scaffolds in organic synthesis.1 The chemistry of isocyanides is characterized by the great diversity of transformations that includes multicomponent reactions (MCRs, such as Passerini and Ugi reaction),2 transition metal-catalyzed insertions (also called imidoylative reaction),3 as well as isocyanide-mediated radical cascade reactions.4 Generally, the isocyanide group can act as a mild nucleophile by electrophilic activation in the presence of carbonyl, imine or transition-metal catalysts, which allow further transformations after the incorporation of isocyanide core into starting material (Scheme 1a). In contrast, the reactions of isocyanides with external nucleophiles are particularly challenging because of the poor electrophilicity of isocyanides, and most of these reactions require highly reactive organometallic nucleophiles (Scheme 1b).5 Only a few reports achieved the direct additions of weak nucleophiles to isocyanides by Lewis acid complexation6 or NHC catalyst (Scheme 1b).7 Therefore, the development of new catalyst system for the activation of isocyanide as electrophilic reagent would be highly desirable.
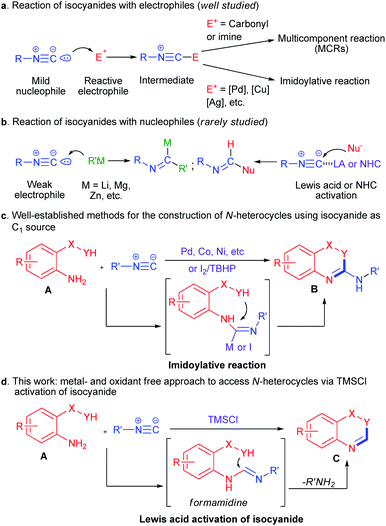 |
| Scheme 1 Strategies for isocyanide activation. | |
On the other hand, nitrogen-containing heterocycles are invaluable building blocks in organic chemistry and are considered to be “privileged” structure in medicinal chemistry.8 In this context, the construction of N-heterocycles has been a major research topic in synthetic chemistry.9 Among these reports, isocyanides have emerged as C1 synthons for the synthesis of various N-heterocycles via isocyanide insertion reactions10 (similar to carbon monoxide11). For example, bisnucleophile agents A could be applied to the synthesis of N-heterocycles B through isocyanide insertion-cyclizations by the use of transition metals (such as Pd, Co, Ni, etc.)12 or I2/TBHP catalytic system13 (Scheme 1c). However, these reports suffer from the use of expensive transition metals or peroxide reagents. Meanwhile, in light of the success of Lewis acid promoted nucleophilic additions to isocyanides. We envisaged that the use of Lewis acid might catalyse the nucleophilic addition of A to isocynide,14 and subsequent cyclization of the formamidine intermediate could deliver the corresponding N-heterocycles C (Scheme 1d). Thus, an unprecedented transition-metal and oxidant-free approach to access various N-heterocycles using isocynide as C1 source could be achieved.
Our study commenced with the reaction between 4-methylaniline (1a) and tert-butyl isocyanide (2a) in acetonitrile at 70 °C. A survey of reaction parameters was summarized in Table 1. First, no desired product was observed in the absence of Lewis acid catalyst (Table 1, entries 1). Then, 1.0 equivalent of CuCl was selected as the Lewis acid based on the literature report,14 formamidine product 3a could be obtained in 50% yield after stirring for 24 h (entry 2). Then, a series of transition metal-based Lewis acids such as AgCl, FeCl3 and ZnCl2 were also evaluated in the same reaction condition, and the results were still unsatisfactory (entries 3–5). Next, we chose Brønsted acids15 such as CF3COOH, and TfOH as the activation reagents for this reaction (entries 5–7). Only a trace mount of formamidine 3a was detected along with unreacted starting material. Fortunately, in the presence of BF3·Et2O, the reaction could afford the corresponding product 3a in 55% yield (entry 8). Surprisingly, further optimization of the reaction conditions revealed silicon-based Lewis acid TMSCl could catalyse the reaction with 85% yield (entry 9).16 To the best of our knowledge, the nucleophilic activation of isocyanides using silicon-based Lewis acid has not yet been reported.17 Meanwhile, catalyst loading had obvious effects on the reaction yields. A slightly increased yield was observed with 1.5 equiv. of TMSCl, while decreasing the amount of TMSCl to 0.5 equiv. resulted in a lower yield of 3a (entries 10 and 11). A survey of other reaction media revealed that the overall results could not be improved (entries 12–14). In addition, a lower yield was obtained when the reaction was performed at room temperature (entry 15). Finally, formamidine 3b could also be obtained in high yield using 4-chloroaniline 1b as nucleophile (entry 16).
Table 1 Optimization of the reaction conditionsb
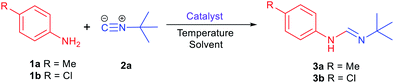
|
Entry |
Catalyst (equiv.) |
Temperature (°C) |
Solvent |
Product |
Yieldb (%) |
Reaction conditions: 1 (0.2 mmol), 2a (0.3 mmol), catalyst (0.5–1.5 equiv.), solvent (2 mL), 24 h. Isolated yields. |
1 |
— |
70 |
CH3CN |
3a |
0 |
2 |
CuCl (1.0) |
70 |
CH3CN |
3a |
50 |
3 |
AgCl (1.0) |
70 |
CH3CN |
3a |
Trace |
4 |
FeCl3 (1.0) |
70 |
CH3CN |
3a |
Trace |
5 |
ZnCl2 (1.0) |
70 |
CH3CN |
3a |
55 |
6 |
CF3COOH (1.0) |
70 |
CH3CN |
3a |
0 |
7 |
TfOH (1.0) |
70 |
CH3CN |
3a |
10 |
8 |
BF3·Et2O (1.0) |
70 |
CH3CN |
3a |
55 |
9 |
TMSCl (1.0) |
70 |
CH3CN |
3a |
85 |
10 |
TMSCl (1.5) |
70 |
CH3CN |
3a |
90 |
11 |
TMSCl (0.5) |
70 |
CH3CN |
3a |
71 |
12 |
TMSCl (1.5) |
70 |
DCE |
3a |
79 |
13 |
TMSCl (1.5) |
70 |
THF |
3a |
59 |
14 |
TMSCl (1.5) |
70 |
Toluene |
3a |
80 |
15 |
TMSCl (1.5) |
rt |
CH3CN |
3a |
52 |
16 |
TMSCl (1.5) |
70 |
CH3CN |
3b |
92 |
With the optimal conditions in hand, we applied this strategy to the synthesis of various quinazolinones18 by employing 2-aminobenzamides 4 as bisnucleophile agents (Table 2). In general, the reaction works well when R1 was an aromatic group. Substituents at para-positions bearing either electron-donating or electron-withdrawing groups can afford the desired products in good to excellent yields (5a–5f). The cyclization products with substituents at meta-positions were also obtained in good yields (5g, 5h), while lower yield was observed with substituent at ortho-position (5i). Then, substrates with aliphatic groups, such as methyl, n-propyl, benzyl, propargyl, etc., were also employed in this reaction to give the corresponding products in 84–90% yields (5j–5o). Next, 2-aminobenzamides with various R2 groups were evaluated in the standard condition, and functionalized quinazolinones were generated in 88–93% yields (5p–5u). It is worth noting that 2-aminobenzene sulfonamide could also be tolerated in this reaction, affording the cyclization products 5v in 87% yield.
Table 2 Substrate scope for the synthesis of various quinazolinonesa
Reaction conditions: 4 (0.2 mmol), 2a (0.3 mmol), TMSCl (1.5 equiv.), CH3CN (2 mL), 70 °C, 24 h. Isolated yields. |
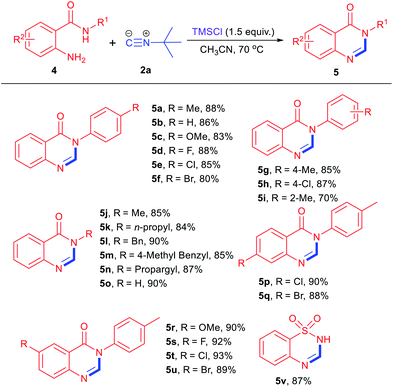 |
The scope of this methodology has been also extended to the synthesis of other N-heterocycles by simply changing the amino-based binucleophiles (Table 3). First, diverse o-phenylene-diamines were subjected to the same reaction conditions. To our delight, the reaction proceed smoothly in all cases regardless of the electronic and steric properties of the substituents, giving the corresponding 1H-benzo[d]imidazole derivatives19 in moderate to good yields (7a–7m). Furthermore, N-methyl and N-phenyl-o-phenylenediamine were also tolerated in this reaction, delivering 2-aminobenzimidazole 7n and 7o in 84% and 80% yields respectively. It is worth noting 2-amino-benzenethiol could undergo the same transformation to furnish benzo[d]thiazole product 7p in 92% yield. However, the reaction failed to generate benzo[d]oxazole 7q with o-aminophenol under identical condition. Finally, diversified facile synthesis of benzimidazo[1,2-c]quinazolines 7r and 7s could be achieved in reasonable yields.
Table 3 Substrate scope for the synthesis of other N-heterocyclesa
Reaction conditions: 6 (0.2 mmol), 2a (0.3 mmol), TMSCl (1.5 equiv.), CH3CN (2 mL), 70 °C, 24 h. Isolated yield. 2.0 equiv. of TMSBr in 2 mL C2H5OH was used. |
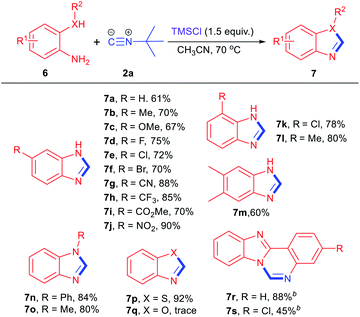 |
To gain an insight into the reaction mechanism, several control experiments were performed as presented in Scheme 2. Initial radical inhibition studies using TEMPO and BHT indicated that the reaction does not proceed through a radical pathway (Scheme 2a). The reaction of 2-aminobenzamides 4a with 2a by the standard condition under N2 provided 5a in 86% yield, revealing that oxygen is not participated in this reaction (Scheme 2b). In the meantime, the generation of tBuNH2 as byproduct was confirmed by GC-MS.20
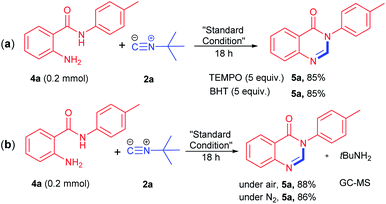 |
| Scheme 2 Control experiments. (a) Radical inhibiton studies. (b) Standard conditon under N2 conditions. | |
The following reaction mechanism is proposed based on our experimental observations and previous literature reports.20 First, nucleophilic addition of bisnucleophile agents A to tert-butyl isocyanide 2a via TMSCl activation could generate formamidine intermediate D. Then intramolecular nucleophilic addition of formamidine D could deliver the cylization intermediate E. Finally, β-elimination of intermediate E could afford the desired product C along with byproduct tBuNH2 (Scheme 3).
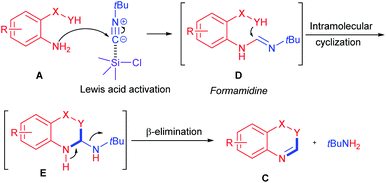 |
| Scheme 3 Plausible reaction mechanism. | |
The present activating strategy was also applied to the synthesis of a biologically active molecule Erlotinib (FDA-approved tyrosine kinase inhibitor).21 The reaction of starting material 8 with isocyanide 2a was performed under the standard condition, affording the key intermediate 9 in 92% yield. Subsequent chlorination and amination reactions could afford Erlotinib in 74% yield over two steps (Scheme 4).
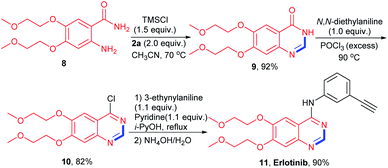 |
| Scheme 4 Synthesis of biologically active compounds. | |
Conclusions
In conclusion, we have developed an efficient silicon-based Lewis acid system for the activation of isocyanides. Based on this strategy, a new robust transition-metal and oxidant free method for the construction of various N-heterocycles could be realized using isocyanide as methine source. Quinazolinone, benzoimidazole, and benzothiazole derivatives could be obtained in good to excellent yields under mild conditions. The present strategy opens a powerful pathway for the activation of isocyanides, and further studies on the application of this methodology are currently underway.
Conflicts of interest
There are no conflicts to declare.
Acknowledgements
The authors are grateful for the financial support from the Fundamental Research Funds for the Central Universities (Grant YJ201853 and YJ201805), National Natural Science Foundation (Grant 21907072).
Notes and references
-
(a) Isocyanide chemistry applications in synthesis and materials science, ed. V. Nenajdenko, Wiley-VCH, Weinheim, 2012 Search PubMed;
(b) A. V. Lygin and A. de Meijere, Isocyanides in the synthesis of nitrogen heterocycles, Angew. Chem., Int. Ed., 2010, 49, 9094–9124 CrossRef CAS PubMed;
(c) M. Giustiniano, A. Basso, V. Mercalli, A. Massarotti, E. Novellino, G. C. Tron and J. Zhu, To each his own: isonitriles for all flavors. Functionalized isocyanides as valuable tools in organic synthesis, Chem. Soc. Rev., 2017, 46, 1295–1357 RSC.
- For selected recent reviews, see:
(a) A. Dömling, Recent developments in isocyanide based multicomponent reactions in applied chemistry, Chem. Rev., 2006, 106, 17–89 CrossRef PubMed;
(b) A. Dömling, W. Wang and K. Wang, Chemistry and biology of multicomponent reactions, Chem. Rev., 2012, 112, 3083–3135 CrossRef PubMed;
(c) C. de Graaff, E. Ruijter and R. V. A. Orru, Recent developments in asymmetric multicomponent reactions, Chem. Soc. Rev., 2012, 41, 3969–4009 RSC.
- For selected recent reviews, see:
(a) V. P. Boyarskiy, N. A. Bokach, K. V. Luzyanin and V. Y. Kukushikin, Metal-mediated and metal-catalyzed reactions of isocyanides, Chem. Rev., 2015, 115, 2698–2779 CrossRef CAS PubMed;
(b) G. Qiu, Q. Ding and J. Wu, Recent advances in isocyanide insertion chemistry, Chem. Soc. Rev., 2013, 42, 5257–5269 RSC;
(c) T. Vlaar, E. Ruijter, B. U. W. Maes and R. V. A. Orru, Palladium-catalyzed migratory insertion of isocyanides: an emerging platform in cross-coupling chemistry, Angew. Chem., Int. Ed., 2013, 52, 7084–7097 CrossRef CAS PubMed;
(d) J. W. Collet, T. R. Roose, E. Ruijter, B. U. W. Maes and R. V. A. Orru, Base metal catalyzed isocyanide insertions, Angew. Chem., Int. Ed., 2020, 59, 540–558 CrossRef CAS PubMed.
- For recent reviews, see:
(a) B. Zhang and A. Studer, Recent advances in the synthesis of nitrogen heterocycles via radical cascade reactions using isonitriles as radical acceptors, Chem. Soc. Rev., 2015, 44, 3505–3521 RSC;
(b) J. Lei, J. Huang and Q. Zhu, Recent progress in imidoyl radical-involved reactions, Org. Biomol. Chem., 2016, 14, 2593–2598 RSC.
- For selected examples, see:
(a) H. M. Walborsky and G. E. Niznik, Radical cations in the chlorine fluoride-antimony pentafluoride systems, J. Am. Chem. Soc., 1969, 91, 7778–7780 CrossRef CAS;
(b) G. E. Niznik, W. H. Morrison and H. M. Walborsky, Metallo aldimines, masked acyl carbanion, J. Org. Chem., 1974, 39, 600–604 CrossRef CAS;
(c) M. Murakami, H. Ito and Y. Ito, Preparation of [1-(arylimino)alkyl]zinc by the alpha-addition of organozinc to isocyanide, J. Org. Chem., 1988, 53, 4156–4158 CrossRef.
- For examples of Lewis acid-promoted reactions of carbon nucleophiles to isocyanides, see:
(a) M. Tobisu, S. Yamaguchi and N. Chatani, Lewis acid-promoted imine synthesis by the insertion of isocyanides into C–H bonds of electron-rich aromatic compounds, Org. Lett., 2007, 9, 3351–3353 CrossRef CAS PubMed;
(b) P. R. Krishna and E. R. Sekhar, p-Toluenesulfonylmethyl isocyanide (TosMIC) and indium manifold strategy to access β-keto-(E)-enamino esters from 1,3-dicarbonyl compounds, Adv. Synth. Catal., 2008, 350, 2871–2876 CrossRef CAS.
- For examples of NHC activation of isocyanides, see:
(a) J. Kim and S. H. Hong, Organocatalytic activation of isocyanides: N-heterocyclic carbene-catalyzed enaminone synthesis from ketones, Chem. Sci., 2017, 8, 2401–2406 RSC;
(b) J. Kim and S. H. Hong, Dual activation of nucleophiles and electrophiles by N-heterocyclic carbene organocatalysis: chemoselective N-imination of indoles with isocyanides, Org. Lett., 2017, 19, 3259–3262 CrossRef CAS PubMed.
-
(a) M. Somei and F. Yamada, Simple indole alkaloids and those with a nonrearranged monoterpenoid unit, Nat. Prod. Rep., 2004, 21, 278–311 RSC;
(b) M. Somei and F. Yamada, Simple indole
alkaloids and those with a non-rearranged monoterpenoid unit, Nat. Prod. Rep., 2005, 22, 73–103 RSC;
(c) M. Z. Zhang, Q. Chen and G.-F. Yang, A review on recent developments of indole-containing antiviral agents, Eur. J. Med. Chem., 2015, 89, 421–441 CrossRef CAS PubMed;
(d) D. J. Foley, A. Nelson and S. P. Marsden, Evaluating new chemistry to drive molecular discovery: fit for purpose?, Angew. Chem., Int. Ed., 2016, 55, 13650–13657 CrossRef CAS PubMed;
(e) M. D. Eastgate, M. A. Schmidt and K. R. Fandrick, On the design of complex drug candidate syntheses in the pharmaceutical industry, Nat. Rev. Chem., 2017, 1, 0016–0031 CrossRef CAS;
(f) D. C. Blakemore, L. Castro, I. Churcher, D. C. Rees, A. W. Thomas, D. M. Wilson and A. Wood, Organic synthesis provides opportunities to transform drug discovery, Nat. Chem., 2018, 10, 383–394 CrossRef CAS PubMed.
- For selected recent reviews, see:
(a) C.-V. T. Vo and J. W. Bode, Synthesis of saturated N-heterocycles, J. Org. Chem., 2014, 79, 2809–2815 CrossRef CAS PubMed;
(b) C. Allais, J.-M. Grassot, J. Rodriguez and T. Constantieux, Metal-free multi- component syntheses of pyridines, Chem. Rev., 2014, 114, 10829–10868 CrossRef CAS PubMed;
(c) Y. Yamamoto, Synthesis of heterocycles via transition-metal-catalyzed hydroarylation of alkynes, Chem. Soc. Rev., 2014, 43, 1575–1600 RSC.
- For selected recent reviews, see:
(a) A. V. Lyginand and A. deMeijere, Isocyanides in the synthesis of nitrogen heterocycles, Angew. Chem., Int. Ed., 2010, 49, 9094–9124 CrossRef PubMed;
(b) S. Lang, Unravelling the labyrinth of palladium-catalysed reactions involving isocyanides, Chem. Soc. Rev., 2013, 42, 4867–4880 RSC;
(c) S. Chakrabarty, S. Choudhary, A. Doshi, F.-Q. Liu, R. Mohan, M. P. Ravindra, D. Shah, X. Yang and F. F. Fleming, Catalytic isonitrile insertions and condensations initiated by RNC–X complexation, Adv. Synth. Catal., 2014, 356, 2135–2196 CrossRef CAS PubMed.
- For a recent review, see: J.-B. Peng, F.-P. Wu and X.-F. Wu, First-row transition-metal-catalyzed carbonylative trans- formations of carbon electrophiles, Chem. Rev., 2019, 119, 2090–2127 CrossRef CAS PubMed.
- For selected examples, see:
(a) T. Vlaar, R. C. Cioc, P. Mampuys, B. U. W. Maes, R. V. A. Orru and E. Ruijter, Sustainable synthesis of diverse privileged heterocycles by palladium-catalyzed aerobic oxidative isocyanide insertion, Angew. Chem., Int. Ed., 2012, 51, 13058–13061 CrossRef CAS PubMed;
(b) T. Vlaar, R. V. A. Orru, B. U. W. Maes and E. Ruijter, Palladium-catalyzed synthesis of 2-aminobenzoxazinones by aerobic oxidative coupling of anthranilic acids and isocyanides, J. Org. Chem., 2013, 78, 10469–10475 CrossRef CAS PubMed;
(c) J. Liu and J. M. Hoover, Cobalt-catalyzed aerobic oxidative cyclization of 2-aminophenols with isonitriles: 2-aminophenol enabled O2 activation by cobalt(II), Org. Lett., 2019, 21, 4510–4514 CrossRef CAS PubMed;
(d) T. Vlaar, L. Bensch, J. Kraakman, C. M. L. Vande Velde, B. U. W. Maes, R. V. A. Orru and E. Ruijter, Synthesis of diverse azolo[c]quinazolines by palladium(II)-catalyzed aerobic oxidative insertion of isocyanides, Adv. Synth. Catal., 2014, 356, 1205–1209 CrossRef CAS;
(e) F. Ahmadi and A. Bazgir, Synthesis of benzoimidazoquinazolines by cobalt-catalyzed isocyanide insertion-cyclization, RSC Adv., 2016, 6, 61955–61958 RSC;
(f) T.-H. Zhu, S.-Y. Wang, G.-N. Wang and S.-J. Ji, Cobalt-catalyzed oxidative isocyanide insertion to amine-based bisnucleophiles: diverse synthesis of substituted 2-aminobenzimidazoles, 2-aminobenzothiazoles, and 2-amino benzoxazoles, Chem. - Eur. J., 2013, 19, 5850–5853 CrossRef CAS PubMed;
(g) A. H. Shinde, S. Arepally, M. D. Baravkar and D. S. Sharada, Nickel-catalyzed aerobic oxidative isocyanide insertion: access to benzimidazoquinazoline derivatives via a sequential double annulation cascade (SDAC) strategy, J. Org. Chem., 2017, 82, 331–342 CrossRef CAS PubMed.
- For selected examples, see:
(a) T.-H. Zhu, S.-Y. Wang, Y.-Q. Tao and S.-J. Ji, Synthesis of carbodiimides by I2/CHP-mediated cross-coupling reaction of isocyanides with amines under metal-free conditions, Org. Lett., 2015, 17, 1974–1977 CrossRef CAS PubMed;
(b) H.-X. Wang, T.-Q. Wei, P. Xu, S.-Y. Wang and S.-J. Ji, I2/TBHP-mediated oxidative coupling of amino-based bisnucleophiles and isocyanides: access to 2-amino benzoxazinones, 2-aminobenzoxazines, and 2-amino quinazolines under metal-free conditions, J. Org. Chem., 2018, 83, 13491–13497 CrossRef CAS PubMed.
- For selected examples of transition-metal catalysed isocyanide insertion into N-H bonds, see:
(a) S. Tong, Q. Wang, M.-X. Wang and J. Zhu, Tuning the reactivity of isocyano group: synthesis of imidazoles and imidazoliums from propargylamines and isonitriles in the presence of multiple catalysts, Angew. Chem., Int. Ed., 2015, 54, 1293–1297 CrossRef CAS PubMed;
(b) A. Clemenceau, Q. Wang and J. Zhu, Silver nitrate-catalyzed isocyanide insertion/lactamization sequence to imidazolones and quinazolin-4-ones: development and application in natural product synthesis, Org. Lett., 2017, 19, 4872–4875 CrossRef CAS PubMed;
(c) T. Saegusa, Y. Ito, S. Kobayashi, K. Hirata and H. Yashioka, Synthetic reactions by complex catalysts. XIV. Reaction of isocyanide with amine catalyzed by group IB and IIB metal compounds, Bull. Chem. Soc. Jpn., 1969, 42, 3310–3313 CrossRef CAS;
(d) T. Saegusa, Y. Ito, S. Kobayashi, K. Hirota and H. Yoshioka, A synthetic reactions by complex catalyst. I. Copper catalyzed reactions of amine with isocyanide, Tetrahedron Lett., 1966, 49, 6121–6124 CrossRef.
-
(a) A. Shaabani, E. Soleimani and A. H. Rezayan, A novel approach for the synthesis of aryl amides, Tetrahedron Lett., 2007, 48, 6137–6141 CrossRef CAS;
(b) A. Ramazani, S. W. JOO and F. Z. Nasrabadi, Environmentally green synthesis of thio- fomamide derivatives, Turk. J. Chem., 2013, 37, 405–412 CAS;
(c) A. Shaabanni, A. H. Rezayan, A. Sarvary, S. Keshipour and H. R. Khavasi, An unexpected coupling reaction between isocyanides and carboxylic acids: a method for the synthesis of highly stable symmetrical and unsymmetrical alkylamidine and arylamidine carbocations, Tetrahedron Lett., 2010, 51, 4091–4094 CrossRef;
(d) X. Li, Y. Yuan, W. F. Berkowitz, L. J. Todaro and S. J. Danishefsky, On the two-component microwave-mediated reaction of isonitriles with carboxylic acids: regarding alleged formimidate carboxylate mixed anhydrides, J. Am. Chem. Soc., 2008, 130, 13222–13224 CrossRef CAS PubMed.
- For selected reviews and examples on silicon-based Lewis acid mediated reactions, see:
(a) A. D. Dilman and S. L. loffe, Carbon−carbon bond forming reactions mediated by silicon Lewis acids, Chem. Rev., 2003, 103, 733–772 CrossRef CAS PubMed;
(b) W.-H. Deng, F. Ye, X.-F. Bai, L. Li, T. Song, Y.-L. Wei and L.-W. Xu, Chlorotrimethylsilane (TMSCl): an efficient silicon-based Lewis acid mediator in allylic alkylation using a diethylzinc reagent, RSC Adv., 2014, 4, 479–483 RSC;
(c) H. M. Yang, L. Li, F. Li, K. Z. Jiang, J. Y. Shang, G. Q. Lai and L. W. Xu, Silicon-based Lewis acid assisted cinchona alkaloid catalysis: highly enantioselective Aza-Michael reaction under solvent-free conditions, Org. Lett., 2011, 13, 6508–6511 CrossRef CAS PubMed;
(d) L. W. Xu, W. Zhou, L. Yang and C. G. Xia, Chlorotrimethylsilane: a powerful Lewis acidic catalyst in Michael-type Friedel–Crafts reactions of indoles and enones, Synth. Commun., 2007, 37, 3095–3104 CrossRef CAS.
- For an example of isocyanide insertion into N-Si bonds, see: K. G. Kishore, O. Ghashighaei, C. Estarellas, M. M. Mestre, C. Monturiol, N. Kielland, J. M. Kelly, A. F. Francisco, S. Jayawardhana, D. Muñoz-Torrero, B. PéREZ, F. J. Luque, R. Gámez-Montaño and R. Lavilla, Insertion of isocyanides into N–Si bonds: multicomponent reactions
with azines leading to potent antiparasitic compounds, Angew. Chem., Int. Ed., 2016, 55, 8994–8998 CrossRef CAS PubMed.
- Selected examples for the synthesis of quinazolinones, see:
(a) O. Jacquet, C. D. N. Gomes, M. Ephritikhine and T. Cantat, Complete catalytic deoxygenation of CO2 into formamidine derivatives, ChemCatChem, 2013, 5, 117–120 CrossRef CAS;
(b) R. Giri, J. K. Lam and J.-Q. Yu, Synthetic applications of Pd(II)-catalyzed C–H carboxylation and mechanistic insights: expedient routes to anthranilic acids, oxazolinones, and quinazolinones, J. Am. Chem. Soc., 2010, 132, 686–693 CrossRef PubMed;
(c) F. Li, L. Lu and P. Liu, Acceptorless dehydrogenative coupling of o-aminobenzamides with the activation of methanol as a C1 Source for the construction of quinazolinones, Org. Lett., 2016, 18, 2580–2583 CrossRef PubMed;
(d) F. Zeng and H. Alper, Tandem palladium-catalyzed addition/cyclocarbonylation: an efficient synthesis of 2-hetero- quinazolin-4(3H)-ones, Org. Lett., 2010, 12, 1188–1191 CrossRef PubMed;
(e) L. Xu, Y. Jiang and D. Ma, Synthesis of 3-substituted and 2,3-disubstituted quinazolinones via Cu-catalyzed aryl amidation, Org. Lett., 2012, 14, 1150–1153 CrossRef PubMed.
- Selected examples for the synthesis of benzoimidazoles, see:
(a) Z.-H. Zhang, J.-J. Li, Y.-Z. Gao and Y.-H. Liu, Synthesis of 2-substituted benzimidazoles by iodine-mediated condensation of orthoesters with 1,2-phenylenediamines, J. Heterocycl. Chem., 2007, 44, 1509–1512 CrossRef;
(b) S. Sharma, D. Bhattacherjee and P. Das, Oxalic/malonic Acids as carbon building blocks for benzazole, quinazoline and quinazolinone synthesis, Org. Biomol. Chem., 2018, 16, 1337–1342 RSC;
(c) Z. Zhang, Q. Sun, C. Xia and W. Sun, CO2 as a C1 source: B(C6F5)3-catalyzed cyclization of o-phenylene-diamines to construct benzimidazoles in the presence of hydrosilane, Org. Lett., 2016, 18, 6316–6319 CrossRef CAS PubMed;
(d) L. Hao, Y. Zhao, B. Yu, H. Zhang, H. Xu and Z. Liu, Au catalyzed synthesis of benzimidazoles from 2-nitroanilines and CO2/H2, Green Chem., 2014, 16, 3039–3044 RSC.
- Y. Ito, I. Ito, T. Hirao and T. Saegusa, Synthesis of some mono-α-chloro-alkyl ketones, Synth. Commun., 1974, 2, 97–104 CrossRef.
- J. Dowell, J. D. Minna and P. Kirkpatrick, Erlotinib hydrochloride, Nat. Rev. Drug Discovery, 2005, 4, 13–14 CrossRef CAS.
Footnote |
† Electronic supplementary information (ESI) available: 1H and 13C NMR spectra. See DOI: 10.1039/d0ra04636a |
|
This journal is © The Royal Society of Chemistry 2020 |
Click here to see how this site uses Cookies. View our privacy policy here.