DOI:
10.1039/D0RA01052A
(Paper)
RSC Adv., 2020,
10, 11615-11623
Microwave-assisted synthesis, biological evaluation and molecular docking studies of new coumarin-based 1,2,3-triazoles†
Received
4th February 2020
, Accepted 24th February 2020
First published on 23rd March 2020
Abstract
Coumarin-based 1,4-disubstituted 1,2,3-triazole derivatives were synthesized using a highly efficient, eco-friendly protocol via a copper(I)-catalyzed click reaction between various substituted arylazides and terminal alkynes. The synthetic route was easy to access and gave excellent yields under microwave irradiation conditions compared to the conventional heating route. The structures of all the compounds were characterized by IR, 1H NMR, 13C NMR spectroscopy and mass spectrometry. All the synthesized compounds were screened for their in vitro antimicrobial, antioxidant and anti-inflammatory activities; among all compounds, 8a, 8j, 8k and 8l exhibited better results with respect to standard drugs. Furthermore, molecular docking studies have been carried out with PDB IDs 2VCX (anti-inflammatory), 3VXI (antioxidant), 4GEE (antimicrobial) and 2XFH (antifungal) using the Glide module of the Schrödinger suite. The final compounds 8d, 8e, 8h, and 8k showed the highest hydrogen bond interactions with His-88 and Val-191 proteins and with water in all the proteins.
1. Introduction
In spite of the extensive use of antibiotic and vaccination programs, infectious diseases continue to be a primary cause of morbidity and mortality worldwide. The continued emergence of antibiotic resistance is one of the utmost global health threats.1 Multidrug resistant bacterial infections are increasing in frequency and require the use of more aggressive antibiotic therapies.2 While the continued development of novel antibiotics is crucial, alternative strategies are also needed, such as the development of adjuvants that target bacterial pathways responsible for antibiotic tolerance or resistance.3 Inflammation is a general condition that occurs during infections in many number of diseases from hay fever, periodontitis, atherosclerosis, rheumatoid arthritis to cancer. The generally used non-steroidal anti-inflammatory drugs (NSAIDs) provide analgesic and antipyretic effects in addition to anti-inflammatory effects in higher doses. The therapeutics are prevailing, but they seriously increase vascular and gastrointestinal risks.4 Although several drugs exist in the market, there is a serious need to required for the development of new antimicrobial drugs and NSAID (Nonsteroidal Anti-Inflammatory Drugs).
The chemistry of heterocyclic compounds has been an interesting field of study for a long period. Coumarin belongs to the class of flavonoids and are found in many natural as well as synthetic products. Coumarin, a well-known structural motif, shows many pharmacological activities such as anticancer,5 antioxidant,6 anti-inflammatory,7 antimicrobial,8 and anticoagulant9 effects; it is also proved to be a promising anti-acetylcholinesterase10 agent. Some of the marketed drugs like Warfarin,9 Phenprocoumon,10 Psoralen11 and Angelicin12 contain the coumarin skeleton in their structure and are found to exhibit various pharmacological activities (Fig. 1). Coumarins can be synthesized by using the Perkin,13,14 Pechmann15,16 and Knoevenagel17 reactions. Among the coumarin compounds, the 3-aryl substituted coumarins have gained attention due to their biological activities such as antimicrobial, antioxidant and anti-inflammatory activities. The 3-aryl coumarin derivatives can be synthesized by using reagents such as DCC, DDQ, NaOH, POCl3 and Mukaiyama reagent (2-chloro-1-methylpyridiniumiodide).18,19 Many of these methods suffer limitations such as the formation of a complex mixture of products, usage of excess reagents and longer reaction times with poor yields. For this reason, a relatively more viable reagent such as 1,1-carbonyldiimidazole20,21 (CDI) is used for the synthesis of 3-aryl coumarin derivatives, which provides excellent yields by avoiding all the above-mentioned issues. On the other hand 1,2,3-triazole, an important heterocyclic motif, has gained much importance in medicinal chemistry and attracted much attention from organic chemists due to its exceptional pharmacological activities such as antimicrobial,22–24 anticancer,25–29 anti-HIV30 and antitubercular activities.31 Some of the important drugs like TSAO and Tazobactam contain 1,2,3-triazole in their structure (Fig. 1).
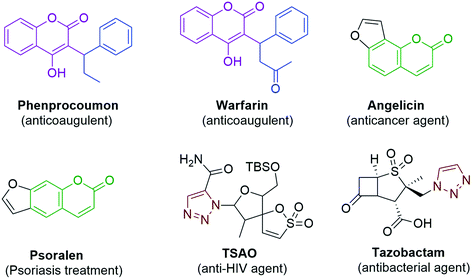 |
| Fig. 1 Marketed drugs containing coumarin and 1,2,3-triazole. | |
In addition, some of the coumarin-based 1,2,3-triazole (Fig. 2) compounds were reported with considerable anti-Alzheimer's,32 anticancer,33 and antibacterial activities34 and as acetylcholinesterase inhibitors.35 The coumarin-based triazole compound 2H-chromen-2-one36 showed antimicrobial activity, 3-[1-(4,5-dicarbomethoxy-1,2,3-triazoloacetyl)]coumarin37 showed antifungal activity and 2-chromene-3-carboxylate derivative38 showed good antioxidant activities.
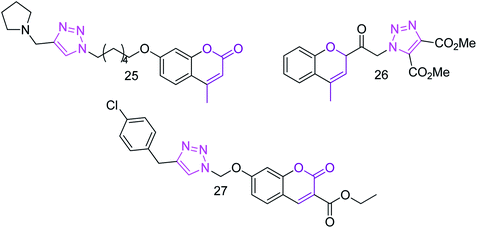 |
| Fig. 2 Synthetic coumarin-based 1,2,3-triazoles reported as antifungal and antioxidant agents. | |
Microwave-assisted synthesis plays a significant role in synthetic chemistry as it reduces the reaction time with enhanced yields when compared to the conventional method and thus is known to be environmentally friendly.39,40 In view of the environmental effects, the microwave-assisted synthesis is considered as an alternative for the conventional heating method to approach green synthesis. This technique is also used in medicinal chemistry to develop drug molecules as it offers higher yields in a short reaction time and reduces the waste products when compared to the conventional heating method. Moreover, these reactions are simple, clean, eco-friendly and easy to carry out. The rate of reaction is enhanced due to thermal/kinetic effects, which are significance of high temperatures that can be quickly attained when the reaction mixture is exposed to a microwave field.
Inspired by the diverse pharmacological activities of the coumarin and 1,2,3-triazole derivatives, we synthesized a series of coumarin-based 1,2,3-triazole compounds 8(a–l) using conventional and microwave irradiation methods; they were then evaluated for their in vitro antioxidant, anti-inflammatory, and antimicrobial activities followed by molecular docking studies.
Computational biology and bioinformatics play a major role in designing drug molecules and have the potential to speed up the drug discovery process. Molecular docking of the drug molecules with the receptor (target) gives significant information about drug–receptor interactions and is frequently used to find out the binding orientation of drug candidates to their protein targets in order to calculate the affinity and activity.39 In view of the pharmaceutical activities of two or more pharmacophore moieties, i.e., coumarin and 1,2,3-triazole, in order to find the combined effects of the newly synthesized scaffolds on biological potency, we herein report a protocol for the design and synthesis of some coumarin-based 1,2,3-triazole compounds.
2. Results and discussion
2.1 Chemistry
The protocol for the synthesis of coumarin-based 1,2,3-triazoles is depicted in Scheme 1. The first step of the synthetic route involves the activation of substituted phenyl acetic acid 1 by using 1,1-carbonyldiimidazole 2 (CDI) using potassium carbonate as the base and acetone as the solvent at room temperature for 1 h to obtain the intermediate 3. In the second step, the alkyne-substituted hydroxy acetophenone 5 is obtained by the selective propargylation of 2,4-dihydroxyacetophenone 4 using K2CO3 in DMF and it is further treated with the intermediate 3 under reflux conditions for 4 h using potassium carbonate and acetone to afford 3-aryl-substituted coumarin compounds 6(a–d). Finally, the alkyne coumarin intermediates 6(a–d) undergo copper(I)-catalyzed Huisgen cycloaddition41 (click chemistry42–44) when treated with different aryl azides 7(a–c) using copper iodide in the presence of DMF/H2O (1
:
1) to obtain coumarin-containing 1,2,3-triazole title compounds 8(a–l).
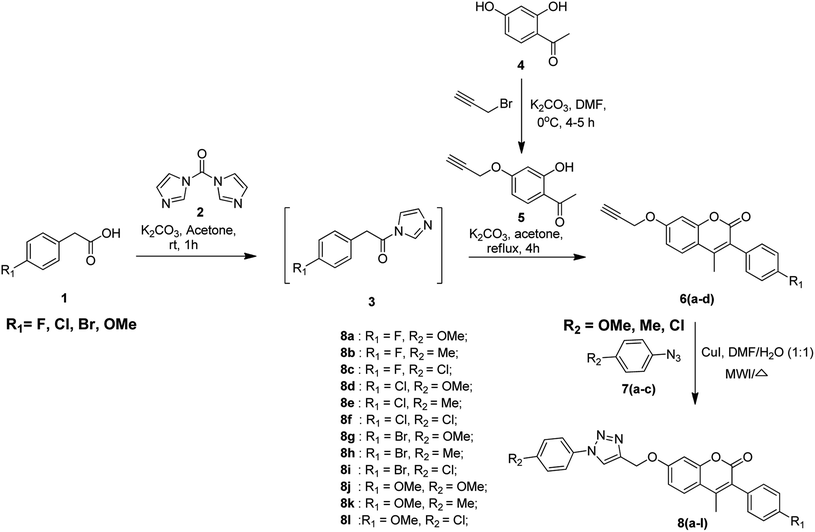 |
| Scheme 1 Synthetic route for coumarin-based 1,2,3-triazole compounds 8(a–l). | |
Initially, the activation of phenyl acetic acids was attempted using DABCO as the coupling agent by using a procedure reported in literature,45 but it could not give promising results. Activation was then performed by treating an acid (1 eq.) with DBU (1 eq.) as a base using two different solvents, namely, dichloromethane and toluene by following a literature protocol.46 Finally, the reaction conditions were successfully optimized with acid (1 eq.), CDI (1.2 eq.) using K2CO3 as the base and dry acetone as the solvent under inert atmospheric conditions.
The syntheses of alkyne-substituted 3-aryl coumarin intermediates 6(a–d) and coumarin-based 1,2,3-triazole final compounds 8(a–l) were carried out using both conventional and microwave (MW) irradiation methods. Among the two methods, the microwave irradiation method gave good yields (80–90%) when compared to the conventional method (68–79%). The reaction time and yields obtained for the intermediates 6(a–d) and final compounds 8(a–l) via both the methods are summarized in Table 1.
Table 1 Comparison of the time and yields of the synthesized compounds 6(a–d) and 8(a–l) using conventional and microwave irradiation methods
Product |
Conventional method |
Microwave irradiation method |
Time (h) |
% yield |
Time (min) |
% yield |
6a |
5 |
75 |
5 |
86 |
6b |
5.5 |
78 |
6 |
89 |
6c |
4 |
79 |
7 |
90 |
6d |
4.5 |
75 |
6 |
86 |
8a |
5 |
72 |
7 |
85 |
8b |
4.5 |
69 |
7 |
82 |
8c |
5 |
75 |
6 |
84 |
8d |
4.5 |
76 |
8 |
85 |
8e |
5.5 |
75 |
7 |
85 |
8f |
5 |
76 |
6 |
88 |
8g |
5 |
70 |
8 |
82 |
8h |
5.5 |
69 |
8 |
80 |
8i |
4.5 |
72 |
7 |
85 |
8j |
5 |
68 |
5.5 |
80 |
8k |
6 |
72 |
6 |
85 |
8l |
5.5 |
68 |
7 |
80 |
The optimized reaction conditions for the synthesis of the final compounds 8(a–l) by the click reaction of different aryl azides 7(a–c) with alkyne intermediates 6(a–d) using copper(I) iodide as a catalyst under different solvent conditions in both conventional and MW irradiation methods are tabulated in Table 2. Among the reaction conditions performed, the best results were achieved when DMF/H2O (1
:
1) was used as the solvent system in both the methods.
Table 2 Optimization of compound 8a in various methods
Entry |
Solvent |
Conventional method |
Microwave irradiation method |
(% yield) |
(% yield) |
1 |
THF |
35 |
40 |
2 |
CH3CN |
20 |
27 |
3 |
1,4-Dioxane |
17 |
24 |
4 |
DMF |
58 |
62 |
5 |
DMF/H2O (7 : 3) |
60 |
65 |
6 |
DMF/H2O (3 : 2) |
68 |
75 |
7 |
DMF/H2O (1 : 1) |
72 |
85 |
2.2 Biological activity
All the synthesized compounds 6(a–d) and 8(a–l) were screened for antioxidant, anti-inflammatory and antimicrobial scavenging activities. Among all the screened compounds 8a, 8j, 8k and 8l showed better results compared with standard drugs.
2.3 SAR studies
2.3.1 Antioxidant activity. The in vitro antioxidant47 activities of intermediates 6(a–d) and final targets 8(a–l) were determined by using two methods, namely, the DPPH47 radical scavenging assay and H2O2
48 scavenging assay method31 with Ascorbic acid taken as the standard drug. All the synthesized compounds, i.e., 6(a–d) and 8(a–l) were screened for in vitro scavenging activity. The IC50 values ranged from 338.48 μM mL−1 to −0.064 μM mL−1 compared with that of standard Ascorbic acid (IC50 value 1.46 μM mL−1). Compounds 8k (IC50 value 0.06146 μM mL−1), 8j (IC50 value 1.11 μM mL−1) and 8d (IC50 value 1.29 μM mL−1) showed excellent activity because the presence of methoxy and other electron releasing groups like methyl maintained the stability of the compounds and also contributed to the improved activity for compound 8k. The presence of two methoxy groups in compound 8d slightly decreased the activity. Further substitution of electron-withdrawing groups like chlorine and methoxy in compound 8l decreased the activity. In addition, 8a (3.50 μM mL−1), 8l (3.52 μM mL−1) and 8f (3.72 μM mL−1) exhibited good activity than the standard drug in the DPPH method. In contrast, in the H2O2 method, compound 8k (IC50 value 0.06146 μM mL−1) exhibited significant results and 8j (IC50 value 1.29 μM mL−1), 8c (IC50 value 1.45 μM mL−1), 8f (IC50 value 1.72 μM mL−1) and 8a (IC50 value 1.76 μM mL−1) revealed good activity than standard Ascorbic acid (IC50 value 1.16 μM mL−1). The antioxidant activity values are shown in Table 3.
Table 3 Antioxidant activity of compounds 6(a–d) and 8(a–l)
Compound |
Antioxidant activity |
DPPH method IC50 (μM mL−1) |
H2O2 method IC50 (μM mL−1) |
6a |
2.24 ± 0.93 |
3.524 ± 0.47 |
6b |
20.00 ± 0.34 |
23.57 ± 0.68 |
6c |
293.18 ± 0.25 |
7.07 ± 0.02 |
6d |
4.5 ± 0.47 |
1.35 ± 0.45 |
8a |
3.5 ± 0.25 |
1.76 ± 0.06 |
8b |
338.48 ± 0.47 |
124.48 ± 0.53 |
8c |
42.50 ± 0.58 |
1.45 ± 0.45 |
8d |
1.11 ± 0.43 |
71.21 ± 0.47 |
8e |
343.33 ± 0.58 |
343.33 ± 0.57 |
8f |
3.72 ± 0.69 |
1.72 ± 0.47 |
8g |
18.57 ± 0.12 |
18.57 ± 0.69 |
8h |
2.51 ± 0.36 |
42.51 ± 0.47 |
8i |
4.8 ± 0.24 |
4.8 ± 0.56 |
8j |
1.29 ± 0.35 |
1.269 ± 0.14 |
8k |
0.061 ± 0.38 |
0.061 ± 0.41 |
8l |
3.524 ± 0.65 |
3.06 ± 0.32 |
Ascorbic acid |
1.46 ± 0.52 |
1.16 ± 0.89 |
2.3.2 Anti-inflammatory activity. The in vitro anti-inflammatory49,50 activities of all the synthesized compounds 6(a–d) and 8(a–l) were determined by using two methods, namely, the egg-albumin method and heat-induced hemolytic method by taking Diclofenac as the standard drug. In the egg-albumin method, 8a (IC50 value 15.78 μM mL−1) and 6d (IC50 value 16.50 μM mL−1) exhibited excellent activity than Diclofenac (IC50 value 17.52 μM mL−1). In the heat-induced hemolytic method, compounds 6a (IC50 value 12.06 μM mL−1), 8g (IC50 value 13.16 μM mL−1), 6d (IC50 value 15.00 μM mL−1), 8i (IC50 value 15.35 μM mL−1), 8j (IC50 value 15.90 μM mL−1) and 8f (IC50 value 17.11 μM mL−1) exhibited excellent activity; also, 8a (IC50 value 17.78 μM mL−1) and 8l (IC50 value 18.90 μM mL−1) demonstrated good activity compared to the standard Diclofenac drug (IC50 value 17.52 μM mL−1). The anti-inflammatory activity values are shown in Table 4.
Table 4 Anti-inflammatory activity of compounds 6(a–d) and 8(a–l)
Compound |
Anti-inflammatory |
Egg-albumin method IC50 (μM mL−1) |
Heat-induced hemolytic method IC50 (μM mL−1) |
6a |
42.06 ± 0.57 |
12.06 ± 0.57 |
6b |
22.91 ± 0.25 |
22.91 ± 0.25 |
6c |
63.72 ± 0.32 |
63.72 ± 0.32 |
6d |
16.50 ± 0.91 |
15.00 ± 0.91 |
8a |
15.78 ± 0.52 |
17.78 ± 0.42 |
8b |
59.809 ± 0.69 |
69.809 ± 0.69 |
8c |
76.49 ± 0.14 |
66.49 ± 0.14 |
8d |
238.13 ± 0.35 |
138.13 ± 0.35 |
8e |
59.80 ± 0.63 |
69.80 ± 0.63 |
8f |
31.21 ± 0.47 |
17.11 ± 0.47 |
8g |
103.26 ± 0.69 |
13.16 ± 0.69 |
8h |
53.77 ± 0.14 |
43.77 ± 0.14 |
8i |
45.35 ± 0.87 |
15.35 ± 0.87 |
8j |
18.90 ± 0.37 |
15.90 ± 0.37 |
8k |
42.53 ± 0.41 |
60.67 ± 0.41 |
8l |
28.90 ± 0.65 |
18.90 ± 0.65 |
Diclofenac |
17.52 ± 0.98 |
17.52 ± 0.98 |
2.3.3 Antibacterial activity. The newly synthesized compounds 6(a–d) and 8(a–l) were screened for their antibacterial activity against Gram-positive strains such as Staphylococcus aureus (MTCC 96) and Bacillus subtilis (MTCC 121) and Gram-negative strains such as Escherichia coli (MTCC 43) and Klebsiella pneumonia (MTCC 530) at various concentrations, i.e., 10 μg mL−1 and 20 μg mL−1 by using the agar disc diffusion method.51 The zone of inhibition was measured in mm and Gatifloxacin was used as the standard drug; the results are shown in Table 5. From the biological evaluation of the activity of the intermediates 6(a–d) to the final compounds 8(a–l), activity further increased due to the presence of 1,2,3-triazole moieties. Among all the prepared compounds, 8a, 8d, 8g and 8j were highly potent due to the presence of the methoxy group in the triazole ring and also, compounds 8b, 8c, 8e, 8f, 8h and 8i showed good activity against all bacterial strains due to the electron-withdrawing groups on the coumarin ring. Compound 8k and compound 8l both showed similar activity to that of the standard drug. The starting compounds 6(a–d) did not show activity. The results also demonstrated that the activity of these compounds 6(a–d) and 8(a–l) was influenced by their structures. In conclusion, 8a and 8j showed very high potential antibacterial activity against tested organisms.
Table 5 Antibacterial activity of compounds 6(a–d) and 8(a–l) at different concentrations
Compound |
Zone of inhibition (mm) |
Gram positive bacteria |
Gram negative bacteria |
Staphylococcus aureus |
Bacillus subtilis |
Escherichia coli |
Klebsiella pneumonia |
10 μg mL−1 |
20 μg mL−1 |
10 μg mL−1 |
20 μg mL−1 |
10 μg mL−1 |
20 μg mL−1 |
10 μg mL−1 |
20 μg mL−1 |
6a |
12 |
18 |
11 |
21 |
08 |
11 |
06 |
10 |
6b |
09 |
19 |
12 |
19 |
10 |
12 |
04 |
11 |
6c |
10 |
20 |
10 |
22 |
11 |
15 |
07 |
09 |
6d |
12 |
17 |
09 |
18 |
09 |
12 |
12 |
14 |
8a |
22 |
32 |
23 |
40 |
16 |
21 |
14 |
20 |
8b |
15 |
24 |
16 |
32 |
13 |
16 |
08 |
14 |
8c |
18 |
36 |
16 |
34 |
14 |
18 |
09 |
16 |
8d |
23 |
32 |
22 |
42 |
18 |
23 |
13 |
20 |
8e |
16 |
23 |
15 |
32 |
12 |
16 |
08 |
13 |
8f |
18 |
25 |
19 |
38 |
15 |
19 |
11 |
17 |
8g |
24 |
34 |
22 |
42 |
18 |
25 |
12 |
22 |
8h |
15 |
24 |
16 |
28 |
14 |
17 |
06 |
13 |
8i |
16 |
27 |
19 |
39 |
12 |
20 |
09 |
17 |
8j |
26 |
34 |
27 |
39 |
21 |
29 |
14 |
22 |
8k |
19 |
26 |
16 |
32 |
12 |
14 |
06 |
12 |
8l |
19 |
27 |
18 |
37 |
14 |
25 |
08 |
15 |
Gatifloxacin |
20 |
30 |
20 |
40 |
15 |
20 |
10 |
18 |
2.3.4 Antifungal activity. All the synthesized compounds 6(a–d) and 8(a–l) were screened for their in vitro antifungal activity against three fungal organisms, namely, Aspergillus niger, Aspergillus flavus and Fusariumoxy sporum at a concentration of 50 μg mL−1 by using the disc diffusion method51 and the results were compared with that of Clotrimazole, used as a standard drug. The study of antifungal activity is shown in Table 6; it was observed that among all the synthesized compounds, 8a, 8b, and 8c showed better activity against the three pathogenic fungi due to the presence of fluorine group on coumarin. Compounds 8j, 8k and 8l showed better activity due to the presence of the methoxy group in the triazole ring. The remaining compounds exhibited similar activity to that of the standard drug. Due to the methoxy group of the triazole compounds 8d and 8g, they showed poor activity. It was observed that the intermediate-to-product activity increased due to the presence of the 1,2,3-triazole moiety. Overall, the antifungal activities of the compounds were good against the tested fungal strains.
Table 6 Antifungal activities of the compounds 6(a–d) and 8(a–l) at a concentration 50 μg mL−1
Compound |
Zone of inhibition (mm) at 50 μg mL−1 concentration |
Aspergillus niger |
Aspergillus flavus |
Fusariumoxy sporum |
6a |
07.2 |
06.4 |
07.3 |
6b |
08.8 |
07.2 |
06.6 |
6c |
07.2 |
08.6 |
09.2 |
6d |
08.2 |
07.9 |
06.5 |
8a |
18.8 |
17.6 |
18.6 |
8b |
17.9 |
16.8 |
17.8 |
8c |
18.2 |
16.3 |
18.9 |
8d |
10.6 |
11.8 |
11.6 |
8e |
13.6 |
14.2 |
14.8 |
8f |
15.2 |
14.8 |
16.2 |
8g |
10.3 |
12.5 |
12.6 |
8h |
14.6 |
13.8 |
14.6 |
8i |
15.9 |
14.5 |
15.8 |
8j |
18.6 |
16.7 |
19.0 |
8k |
16.5 |
15.8 |
16.2 |
8l |
15.9 |
14.8 |
14.3 |
Clotrimazole |
17.3 |
16.4 |
18.2 |
2.4 Molecular docking studies
To gain more insights into the interactions of coumarin-based 1,2,3-triazole derivatives 8(a–l), molecular docking studies were performed. Molecular docking was carried out using the Glide module of the Schrödinger suite using PDB IDs 2VCX (anti-inflammatory),52 3VXI (antioxidant),53 4GEE (antimicrobial)54 and 2XFH (antifungal)55 to correlate the binding mode of the synthesized compounds with the proteins. The above-mentioned proteins were selected on the basis of the reference compound that existed as a co-crystal ligand on the target protein. In the current work, we have considered 2VCX for its anti-inflammatory activity since it is a prostaglandin D2 synthase56 protein involved in the cyclooxygenase pathway.57 Similarly, 3VXI was used for its anti-oxidant activity due to its dye decolourising peroxidase (DyP) complex with Ascorbic acid as the crystal ligand.58 The protein 4GEE was considered because of its DNA–gyrase B and topoisomerase IV-mediated broad spectrum activity.59 For the identification of antifungal activity, we thereby considered 2XFH Cytochrome P450 EryK cocrystallized with the inhibitor Clotrimazole.60 The potential binding free energies (ΔG) were evaluated and the rationality of the compounds was further evaluated through QikProp for their drug likeness.
In conclusion, comparative docking studies from all the proteins revealed that compound 8l showed a high docking score of −7.792 and a binding energy of −117.95 in the protein 2VCX; also, their predicted activities were found to be slightly higher than that of the standard (provided in the ESI Table†). This clearly indicated that compound 8l showed an explicit effect as a potential anti-inflammatory, antimicrobial and antioxidant molecule. The intermediate compounds 6b and 6d showed best H-bond interactions with water in all the proteins (2XFH, 4GEE and 3VXI) as the standard Ascorbic acid and Gatifloxacin predominantly (as illustrated in Fig. 1 and 3 of the ESI†), stating that these compounds could be further exploited to newer chemical agents possessing antioxidant and antimicrobial activities, as depicted in Fig. 4. The standard antifungal agent Clotrimazole shows hydrogen bond interactions with Arg-293 (depicted in Fig. 2 provided in the ESI†). The final compounds 8d, 8e, 8h and 8k showed hydrogen bond interactions with His-88 (Fig. 4a) and Val-191 (4b) amino acids and with water (4c) in all the proteins 2VCX, 3VXI, 4GEE and 2XFH. This signifies that the synthesized compounds possess antibacterial and antifungal activities in addition to antioxidant and anti-inflammatory activities. Docking scores along with the binding free energies for all the synthesized compounds have been quantified and tabulated in Table 7. The average predicted activities of different chemical methods have been calculated for the synthesized compounds (provided in the ESI from Tables 3d to 6d†). The pharmacokinetic properties (ADME) of the compounds exhibited excellent 100% human oral absorption and were found to be in the acceptable range, as shown in Table 2d (provided in the ESI†).
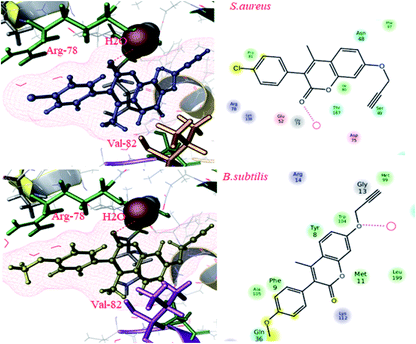 |
| Fig. 3 Docking pose images of intermediate compounds 6b and 6d showing H-bond interaction with water in Gram positive bacteria by the protein 4GEE. | |
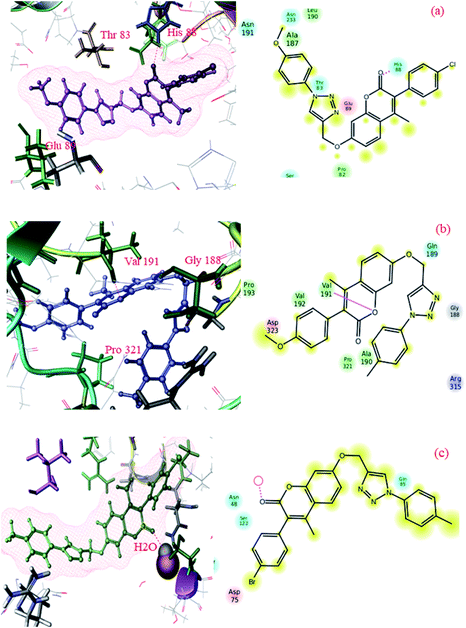 |
| Fig. 4 Docking pose images of final compounds 8d, 8h and 8k showing H-bond interaction with His-88 in 2VCX protein (a), Val-191 in 3VXI (b) and water in 2XFH and 4GEE proteins (c), respectively. | |
Table 7 Quantified active site binding free energies along with the docking scores of the synthesized compounds and standard molecules
Compounds |
Docking scores |
Binding free energies |
Anti-inflammatory activity (2VCX) |
Anti-oxidant activity (3VXI) |
Anti-microbial |
Anti-inflammatory activity (2CVX) |
Anti-oxidant activity (3VXI) |
Anti-microbial |
Anti-bacterial (4GEE) |
Anti-fungal (2XFH) |
Anti-bacterial (4GEE) |
Anti-fungal (2XFH) |
6a |
−5.984 |
−4.882 |
−5.760 |
−6.657 |
−83.72 |
−54.41 |
−69.13 |
−77.31 |
6b |
−6.315 |
−4.826 |
−6.748 |
−5.148 |
−86.94 |
−55.04 |
−70.79 |
−61.08 |
6c |
−6.177 |
−4.559 |
−6.585 |
−4.857 |
−86.36 |
−55.68 |
−71.29 |
−61.61 |
6d |
−6.232 |
−3.801 |
−6.580 |
−4.791 |
−83.57 |
−46.00 |
−78.56 |
−57.66 |
8a |
−6.928 |
−3.355 |
−5.756 |
−3.180 |
−94.07 |
−52.26 |
−68.97 |
−52.0 |
8b |
−6.587 |
−3.636 |
−4.994 |
−4.096 |
−88.37 |
−53.29 |
−57.01 |
−52.6 |
8c |
−6.165 |
−2.949 |
−5.122 |
−5.676 |
−88.66 |
−59.42 |
−53.52 |
−69.37 |
8d |
−6.962 |
−2.438 |
−5.970 |
−2.927 |
−92.74 |
−54.29 |
−71.94 |
−45.55 |
8e |
−6.927 |
−3.740 |
−5.796 |
−2.368 |
−92.26 |
−60.44 |
−71.17 |
−38.23 |
8f |
−6.051 |
−3.528 |
−4.818 |
−4.339 |
−92.16 |
−47.36 |
−62.39 |
−67.33 |
8g |
−6.972 |
−3.720 |
−5.554 |
−2.792 |
−106.36 |
−57.00 |
−67.63 |
−44.93 |
8h |
−6.725 |
−3.778 |
−5.654 |
−3.594 |
−92.263 |
−47.41 |
−72.09 |
−51.41 |
8i |
−6.575 |
−2.823 |
−5.659 |
−4.112 |
−94.63 |
−53.35 |
−71.45 |
−68.46 |
8k |
−6.587 |
−3.240 |
−5.006 |
−2.587 |
−94.638 |
−54.15 |
−62.72 |
−37.15 |
8l |
−7.792 |
−2.868 |
−4.869 |
−3.984 |
−117.95 |
−50.68 |
−61.15 |
−47.79 |
Diclofenac |
−5.553 |
— |
— |
— |
−91.55 |
— |
— |
— |
Ascorbic acid |
— |
−6.184 |
— |
— |
— |
−85.23 |
— |
— |
Gatifloxacin |
— |
— |
−5.553 |
— |
— |
— |
−56.38 |
— |
Clotrimazole |
— |
— |
— |
−6.288 |
— |
— |
— |
−89.15 |
3. Conclusion
We herein, reported the new scaffolds 6(a–d) and 8(a–l) were synthesized by using a microwave irradiation method to obtained better yield as compared to conventional heating method. The use of a DMF
:
water (1
:
1) solvent system helped in increasing the product yield through traditional, conventional and microwave irradiation routes. However, we observed greater yields via the microwave irradiation route in lesser duration. Biological evaluations and molecular docking studies revealed an increase in the activity of the target compounds from their intermediates. Compounds 8d, 8e, 8h and 8k showed excellent hydrogen bonding interactions with His-88, Val-191 and water.
Conflicts of interest
There are no conflicts to declare.
Acknowledgements
Author Ravinder Dharavath (File No. 09/132(0865)/2017-EMR-I) is grateful to CSIR, New Delhi, for financial assistance in the form of SRF. One of the authors D. Ashok is thankful to UGC-New Delhi, for the award of BSR Faculty Fellowship. Authors NN and MRR are thankful to UGC-New Delhi. The authors thankful to The Head, Department of Chemistry, Osmania University, Hyderabad, for providing laboratory facilities. We thank CFRD, Osmania University analytical team for providing spectral analysis facilities.
References
- D. J. Payne, Science, 2008, 321(5896), 1644–1645 CrossRef CAS PubMed.
- H. C. Neu, Science, 1992, 257(5073), 1064–1073 CrossRef CAS PubMed.
- C. A. Arias and B. E. Murray, N. Engl. J. Med., 2009, 360(5), 439–443 CrossRef CAS PubMed.
- N. Bhala, J. Emberson, A. Merhi and S. Abramson, Lancet, 2013, 382, 769–779 CrossRef CAS.
- G. Achar, C. R. Shahini, S. A. Patil, J. G. Małecki and S. Budagumpi, New J. Chem., 2019, 43(3), 1216–1229 RSC.
- R. Nagamallu, B. Srinivasan, M. B. Ningappa and A. K. Kariyappa, Bioorg. Med. Chem. Lett., 2016, 26(2), 690–694 CrossRef CAS PubMed.
- P. Chandel, A. Kumar, N. Singla, A. Kumar, G. Singh and R. K. Gill, MedChemComm, 2019, 10(3), 421–430 RSC.
- K. Bhagat, J. Bhagat, M. K. Gupta, J. V. Singh, H. K. Gulati, A. Singh and H. Singh, ACS Omega, 2019, 4(5), 8720–8730 CrossRef CAS PubMed.
- R. A. O'Reilly and P. M. Aggeler, Circulation, 1968, 38(1), 169–177 CrossRef PubMed.
- D. Viña, M. J. Matos, M. Yáñez, L. Santana and E. Uriarte, MedChemComm, 2012, 3(2), 213–218 RSC.
- M. L. Panno and F. Giordano, World J. Clin. Oncol., 2014, 5(3), 348 CrossRef PubMed.
- Y. Wang, Y. Chen, X. Chen, Y. Liang, D. Yang, J. Dong and Z. Liang, Exp. Ther. Med., 2019, 18(5), 3365–3374 CAS.
- M. Trkovnik and Z. Ivezic, J. Heterocycl. Chem., 2009, 37, 137–141 CrossRef.
- S. H. Mashraqui, D. Vashi and H. D. Mistry, Synth. Commun., 2004, 34(17), 3129–3134 CrossRef CAS.
- Y. Ming and D. W. Boykin, Heterocycles, 1987, 26, 3229–3231 CrossRef CAS.
- R. S. Mali and S. G. Tilve, Synth. Commun., 1990, 20(12), 1781–1791 CrossRef CAS.
- N. Hans, M. Singhi, V. Sharma and S. K. Grover, Indian J. Chem., Sect. B: Org. Chem. Incl. Med. Chem., 1996, 35, 1159–1162 Search PubMed.
- D. V. Kadnikov and R. C. Larock, J. Organomet. Chem., 2003, 687, 422–435 CrossRef.
- D. Olmedo, R. Sancho, L. M. Bedoya, J. L. López-Pérez, E. D. Olmo, E. Muñoz, J. Alcamí, M. P. Gupta and A. S. Feliciano, Molecules, 2012, 17(8), 9245–9257 CrossRef CAS PubMed.
- S. K. Verma, R. Ghorpade, A. Pratap and M. P. Kaushik, Tetrahedron Lett., 2012, 53(19), 2373–2376 CrossRef CAS.
- D. Ashok, E. V. L. Madhuri and M. Sarasija, Asian J. Chem., 2020, 32(1), 205–208 CAS.
- N. Kuntala, J. R. Telu, V. Banothu, S. B. Nallapati, J. S. Anireddy and S. Pal, MedChemComm, 2015, 6(9), 1612–1619 RSC.
- P. Neeraja, S. Srinivas, K. Mukkanti, P. K. Dubey and S. Pal, Bioorg. Med. Chem. Lett., 2016, 26(21), 5212–5217 CrossRef CAS PubMed.
- N. Kuntala, J. R. Telu, V. Banothu, S. Balasubramanian, J. S. Anireddy and S. Pal, Mini-Rev. Med. Chem., 2018, 18(9), 803–809 CrossRef CAS PubMed.
- A. M. Macan, A. Harej, I. Cazin, M. Klobučar, V. Stepanić, K. Pavelić and S. Raić-Malić, Eur. J. Med. Chem., 2019, 184, 111739 CrossRef CAS PubMed.
- J. Mareddy, S. B. Nallapati, J. Anireddy, Y. P. Devi, L. N. Mangamoori, R. Kapavarapu and S. Pal, Bioorg. Med. Chem. Lett., 2013, 23(24), 6721–6727 CrossRef CAS PubMed.
- K. S. S. Praveena, S. Durgadas, N. S. Babu, S. Akkenapally, C. G. Kumar, G. S. Deora and S. Pal, Bioorg. Chem., 2014, 53, 8–14 CrossRef CAS PubMed.
- K. S. S. Praveena, E. V. V. S. Ramarao, N. Y. S. Murthy, S. Akkenapally, C. G. Kumar, R. Kapavarapu and S. Pal, Bioorg. Med. Chem. Lett., 2015, 25(5), 1057–1063 CrossRef CAS PubMed.
- J. Mareddy, N. Suresh, C. G. Kumar, R. Kapavarapu, A. Jayasree and S. Pal, Bioorg. Med. Chem. Lett., 2017, 27(3), 518–523 CrossRef CAS PubMed.
- R. Alvarez, S. Velazquez, A. San-Felix, S. Aquaro, E. D. Clercq, C. F. Perno and M. J. Camarasa, J. Med. Chem., 1994, 37(24), 4185–4194 CrossRef CAS PubMed.
- M. H. Shaikh, D. D. Subhedar, L. Nawale, D. Sarkar, F. A. K. Khan, J. N. Sangshetti and B. B. Shingate, MedChemComm, 2015, 6(6), 1104–1116 RSC.
- Z. Najafi, M. Mahdavi, M. Saeedi, E. Karimpour-Razkenari, N. Edraki, M. Sharifzadeh and T. Akbarzadeh, Bioorg. Chem., 2019, 83, 303–316 CrossRef CAS PubMed.
- M. Mohammadi-Khanaposhtani, K. Fahimi, E. Karimpour-Razkenari, M. Safavi, M. Mahdavi, M. Saeedi and T. Akbarzadeh, Lett. Drug Des. Discovery, 2019, 16(7), 818–824 CrossRef CAS.
- A. V. Lipeeva, D. O. Zakharov, L. G. Burova, T. S. Frolova, D. S. Baev, I. V. Shirokikh and E. E. Shults, Molecules, 2019, 24(11), 2126 CrossRef CAS PubMed.
- F. C. Torres, G. A. Gonçalves, K. L. Vanzolini, A. A. Merlo, B. Gauer, M. Holzschuh and G. L. V. Poser, J. Braz. Chem. Soc., 2016, 27(9), 1541–1550 CAS.
- K. Kushwaha, N. Kaushik and S. C. Jain, Bioorg. Med. Chem. Lett., 2014, 24, 1795–1801 CrossRef CAS PubMed.
- R. A. Kusanur and M. V. Kulkarni, Indian J. Chem., Sect. B: Org. Chem. Incl. Med. Chem., 2005, 44, 591–594 Search PubMed.
- M. H. Shaikh, D. D. Subhedar, F. A. K. Khan, J. N. Sangshetti and B. B. Shingate, Chin. Chem. Lett., 2016, 27(2), 295–301 CrossRef CAS.
- D. Ashok, K. Rangu, V. H. Rao, S. Gundu, B. Srilata and M. Vijjulatha, Med. Chem. Res., 2016, 25(3), 501–514 CrossRef CAS.
- D. Ashok, S. Gundu, V. K. Aamate, M. G. Devulapally, R. Bathini and V. Manga, J. Mol. Struct., 2018, 1157, 312–321 CrossRef CAS.
- V. V. Rostovtsev, L. G. Green, V. V. Fokin and K. B. Sharpless, Angew. Chem., Int. Ed., 2002, 41(14), 2596–2599 CrossRef CAS PubMed.
- H. C. Kolb, M. G. Finn and K. B. Sharpless, Angew. Chem., Int. Ed., 2001, 40, 2004–2021 CrossRef CAS PubMed.
- Y. Hua and A. H. Flood, Chem. Soc. Rev., 2010, 39(4), 1262–1271 RSC.
- P. Nouraie, S. Moradi Dehaghi and A. Foroumadi, Synth. Commun., 2019, 49(3), 386–394 CrossRef CAS.
- M. Roussaki, C. A. Kontogiorgis, D. Hadjipavlou-Litina, S. Hamilakis and A. Detsi, Bioorg. Med. Chem. Lett., 2010, 20(13), 3889–3892 CrossRef CAS PubMed.
- S. K. Verma, R. Ghorpade, A. Pratap and M. P. Kaushik, Tetrahedron Lett., 2012, 53(19), 2373–2376 CrossRef CAS.
- H. Barry, Drugs, 1991, 42(2), 569–605 Search PubMed.
- V. Jacob and A. Micheal, Nutrition, 1999, 49, 1–7 Search PubMed.
- S. Chandra, P. Chatterjee, P. Dey and S. Bhattacharya, Asian Pac. J. Trop. Biomed., 2012, 2(1), S178–S180 CrossRef.
- S. C. Chippada and M. Vangalapati, J. Chem., Biol. Phys. Sci., 2011, 1(2), 260–269 CAS.
- A. L. Barry, Manual of clinical microbiology, 1985, pp. 978–987 Search PubMed.
- M. Hohwy, L. Spadola, B. Lundquist, P. Hawtin, J. Dahmen, I. Groth-Clausen, E. Nilsson, S. Persdotter, K. Von Wachenfeldt, R. H. A. Folmer and K. Edman, J. Med. Chem., 2008, 51, 2178–2186 CrossRef CAS PubMed.
- Y. Sugano, T. Yoshida and H. Tsuge, wwPDB X-ray Structure Validation Report, 2018, pp. 1–12 Search PubMed.
- L. W. Tari, M. Trzoss, D. C. Bensen, X. Li, Z. Chen, T. Lam, J. Zhang, C. J. Creighton, M. L. Cunningham, B. Kwan, M. K. J. Shaw, F. C. Lightstone, S. E. Wong, T. B. Nguyen, J. Nix and J. Finn, Bioorg. Med. Chem. Lett., 2013, 23, 1529–1536 CrossRef CAS PubMed.
- L. C. Montemiglio, S. Gianni, B. Vallone and C. Savino, Biochemistry, 2010, 49, 9199 CrossRef CAS PubMed.
- R. G. Bellomo, S. M. Carmignano, T. Palermo, L. Cosenza, A. Saggini and R. Saggini, Nonsteroidal Anti-Inflammatory Drugs, 2017, vol. 67 Search PubMed.
- R. Emanuela and A. F. Garret, Arterioscler., Thromb., Vasc. Biol., 2011, 31(5), 986–1000 CrossRef PubMed.
- J. Fabri, W. Dabelstein, A. Reglitzky, A. Schütze and K. Reders, Ullmann's Encyclopedia of Industrial Chemistry, 2000 Search PubMed.
- F. Collin, S. Karkare and A. Maxwell, Appl. Microbiol. Biotechnol., 2011, 92(3), 479–497 CrossRef CAS PubMed.
- American Society of Health-System Pharmacists Clotrimazole Monograph for Professionals, 8 February 2016.
Footnote |
† Electronic supplementary information (ESI) available. See DOI: 10.1039/d0ra01052a |
|
This journal is © The Royal Society of Chemistry 2020 |
Click here to see how this site uses Cookies. View our privacy policy here.