DOI:
10.1039/D0RA00560F
(Paper)
RSC Adv., 2020,
10, 11608-11614
Structure and luminescence properties of a novel broadband green-emitting oxyapatite-type phosphor
Received
18th January 2020
, Accepted 28th February 2020
First published on 23rd March 2020
Abstract
In recent years, synthetic apatite-doped rare-earth luminescent materials and their optical properties have attracted extensive worldwide attention. In this study, a series of novel green phosphors Sr2Y3(SiO4)2(PO4)O:Eu2+ with apatite structure was fabricated via a high temperature solid-state reaction. X-ray diffraction, structure refinement, photoluminescence excitation, emission spectra, and temperature-dependent emission intensity were employed to describe the phase and property of the samples. Under the excitation of 365 nm, the phosphors emit strong green emission in the broad band range from 400 nm to 700 nm, which almost covers the visible light spectrum. The quenching concentration of Eu2+ in Sr2Y3(SiO4)2(PO4)O was about 0.05, which was attributed to the dipole–dipole interactions. The evidence that the as-prepared phosphor can be successfully excited by near ultraviolet light indicates that it can be potentially used as a near UV-convertible phosphor for white light-emitting diodes.
1. Introduction
It is well known that energy efficiency is one of the key parameters in illumination sources and other pervasive products. White light-emitting diodes (wLEDs) have bright future in traffic lights, displays, general lighting, and decorative lighting owing to their high efficiency and environment friendly nature.1–3 Currently, phosphor-converted wLEDs combining tricolor phosphors and near ultraviolet (n-UV) or blue chips have been extensively applied as solid-state lighting sources owing to their excellent performance and properties, e.g., long life, conversion efficiency, high color-rending index, and lower power consumption.4–6
In general, a phosphor is composed of hosts and activators (sometimes sensitizers are also included).7 Rare-earth (RE) ions (Ce3+, Eu2+, and Tb3+) can be used as activators for white light-emitting diodes (wLEDs). The emission color changes when the RE ions are doped into different hosts derived from their 4f–4f or 4f–5d transitions.8,9 Eu2+ with a wide emission color range is a popular activator with intensive absorption in the UV and visible regions.10–14 Transitions between the ground state (4f energy level) and the excited 5d-state of Eu2+ can be influenced by the crystal lattice pattern, e.g., crystal-field strength, atom coordination, and covalence.15–17 Moreover, the careful selection of the host material is also critical since the host structure will affect the crystal field environment of the RE ions, which will change the energy transitions of the RE ions.18 A deeper understanding of the crystal structure of the targeted compound is necessary for choosing the host material because there are at least two or three suitable non-equivalent crystallographic sites for the Eu2+ occupation that can regulate the crystal field environment of Eu2+.19 Accordingly, efforts have been devoted for the development of novel host materials, containing abundant cationic crystallographic sites doped with Eu2+, which can be typically excited by n-UV light.20–23
The apatite-type structured compounds have two nonequivalent cationic sites for the Eu2+ occupation, which enables them to be one of the most potential phosphor host materials.24,25 In general, compounds with the apatite structure are characterized by a general chemical formula of M10[XO4]6Y2, where M is defined as a divalent cation (Mg2+, Ca2+, Ba2+, etc.), whereas rare-earth ions (Y3+, La3+, Eu2+ etc.), alkali metal ions (Na+, K+, Ag+, etc.) can also enter the M-site, thus forming a coupling isomorphic substitution. [XO4] is defined as a complex anionic group ([PO4], [VO4], [AsO4], etc.), and Y commonly represents the anions such as F−, Br−, or OH−.26 In the past few decades, Eu2+-doped apatite phosphors have attracted considerable attention worldwide, e.g., Ca5(PO4)3F,27 Sr5(PO4)3Cl,28 Ca8Gd2(PO4)6O2,29 and Ca2Ba3(PO4)3Cl.30 However, no report is available on the Eu2+-doped Sr2Y3(SiO4)2(PO4)O2 phosphor with the apatite structure. On the other hand, developing novel apatite phosphors doped with Eu2+ that can be excited by n-UV light (350–420 nm) has a significant practical value.
Herein, a novel apatite-type phosphor Sr2Y3(SiO4)2(PO4)O:Eu2+, emitting green fluorescence, was successfully prepared via a high-temperature solid-state reaction,. The crystal structure of the as-prepared sample was identified by the Rietveld refinement method in detail, which was used to study how the crystal structure and composition affected the luminescence property of the sample. In addition, the thermal luminescence property of the phosphor has also been revealed in this study. Under 365 nm excitation, the phosphor exhibits a strong green emission at 505 nm with an obvious band ranging from 400 nm to 700 nm, which almost covers the visible light spectrum. The results indicate that the Sr2Y3(SiO4)2(PO4)O:Eu2+ phosphor can act as a potential green phosphor for n-UV phosphor-converted wLEDs.
2. Experimental procedure
2.1. Materials and synthesis
The Sr2Y3(SiO4)2(PO4)O:Eu2+ phosphor was synthesized using the traditional high-temperature solid-phase synthesis method with starting materials, including SrCO3 (Aldrich, 99.9%), Y2O3 (Aldrich, 99.99%), NH4H2PO4 (Aldrich, 99.9%), SiO2 (Aldrich, 99.99%), and Eu2O3 (Aldrich, 99.999%). First, they were weighed in an electronic balance according to the stoichiometric ratio. Then, they were mixed and ground thoroughly in an agate mortar. Further, the raw materials were preheated at 500 °C for 3 h in a furnace and moved into alumina crucibles after being cooled down to room temperature. In addition, the preliminary products were annealed at 1400 °C for 5 h under a reducing atmosphere (10% H2 + 90% N2). When the furnace was cooled down to room temperature again, the final product was smashed and re-ground for subsequent tests.
2.2. Characterization
The phase structure of each sample was evaluated using an X-ray powder diffractometer (RigakuDmax 12 kW, Japan) with Cu Kα radiation (λ = 0.15418 nm) from 10° to 70° (2θ). The Rietveld refinement of Sr3.95Y6(SiO4)4(PO4)2O2:0.05Eu2+ was carried out by the TOPAS software. Photoluminescence and excitation spectra of the sample were recorded using a fluorescence spectrofluorometer (FL-4600, HITACHI, Japan) fitted with a 700 V and 150 W Xe lamp source. Lifetime of the samples was tested using a spectrometer (HORIBA JOBIN YVON FL3-21) with excitation by a pulsed radiation laser at 370 nm.
3. Results and discussions
Fig. 1 presents the XRD patterns of Sr(2−x)Y3(SiO4)2(PO4)O:xEu2+ (x = 0.01 and 0.05) and the referred standard data for Ca8Nd2(PO4)6O2 (PDF-32-175). The diffraction peaks of the Sr(2−x)Y3(SiO4)2(PO4)O:xEu2+ phosphors are consistent with those of the Ca8Nd2(PO4)6O2 (Fig. 1), where a minor deviation of the dominant diffraction peak at higher 2θ can be found due to the replacement of Sr by Eu, indicating that a small amount of Eu2+ doping does not lead to a variation in the structure.
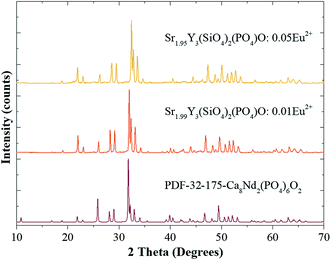 |
| Fig. 1 The XRD patterns of Sr(2−x)Y3(SiO4)2(PO4)O:xEu2+ (x = 0.01 and 0.05), and the standard card (PDF-32-175-Ca8Nd2(PO4)6O2) is shown as a reference. | |
To further investigate the phase purity and occupation of Eu2+, TOPAS 4.2 (ref. 31) was used to analyze the Rietveld refinement. The structure of Ca8Nd2(PO4)6O2, possessing a hexagonal structure with the space group P63/m, was employed as the initial model.32 Silicon oxygen tetrahedron and phosphorus oxygen tetrahedron is independent with each other Sr1 and Sr2 occupy two independent sites for Sr2+ in the crystal lattice. Sr1 is in a 9-fold coordination state, and Sr2 is in a 7-fold coordination state. Similar to Sr2+, the radii of Eu2+ in the 7-fold coordination and 9-fold coordination sites are 0.120 and 0.130 nm, respectively. Eu2+ can occupy the two positions of Sr2+. The Rietveld analysis patterns for the X-ray power diffraction data of Sr3.95Y6(SiO4)4(PO4)2O2:0.05Eu2+ is shown in Fig. 2. The occupation of the cations was set to a fixed value according the chemical formula. The fractional atomic coordinates, isotropic displacement parameters, and the cell parameters were refined. The atomic coordinates, occupancies and displacement parameters (Å2) are listed in Table 1, and the cell parameters and reliability factors are listed in Table 2. The cell parameters are a = b = 9.477(10) Å, c = 6.954(80) Å, α = 90°, β = 90°, γ = 120°, and V = 540.53(16) Å. The refinement was stable and the fit is convincing with low R-factors, which verify the phase purity of the as-prepared sample.
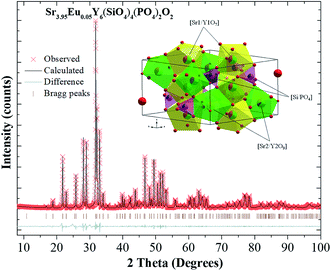 |
| Fig. 2 XRD refinement result of the Sr3.95Eu0.05Y6(SiO4)4(PO4)2O2 sample: experimental results (×), calculated results (|), and their difference (bottom). The brown solid lines represent the Bragg reflection positions, and the inset shows a crystal structure diagram of the host. | |
Table 1 Crystal structural data and lattice parameters for Sr3.95Y6(SiO4)4(PO4)2O2:0.05Eu2+
Formula |
Sr3.95Y6(SiO4)4(PO4)2O2:0.05Eu2+ |
Space group |
P63/m – hexagonal |
Cell parameters |
a = b = 9.477(10) Å, c = 6.954(80) Å |
α = 90°, β = 90°, γ = 120° |
V = 540.53(16) Å, Z = 2 |
Reliability factors |
Rwp = 8.89% |
Rp = 6.66% |
χ2 = 3.64 |
Table 2 Fractional atomic coordinates and isotropic displacement parameters (Å2) of Sr3.95Y6(SiO4)4(PO4)2O2:0.05Eu2+
Atom |
x |
y |
z |
Occupancy |
Uiso/Å2 |
Sr1(Eu1)/Y1 |
1/3 |
2/3 |
0 |
0.35(0.05)/0.6 |
0.0292(7) |
Sr2(Eu2)/Y2 |
0.24632(0) |
0.23413(5) |
1/4 |
0.35(0.05)/0.6 |
0.0250(2) |
P1/Si1 |
0.03324(1) |
0.40456(0) |
1/4 |
0.33/0.67 |
0.0239(3) |
O1 |
0.48621(8) |
0.15352(7) |
1/4 |
1 |
0.0431(0) |
O2 |
0.12523(5) |
0.59325(1) |
1/4 |
1 |
0.0341(2) |
O3 |
0.11200(4) |
0.34721(7) |
0.07382(0) |
1 |
0.0181(6) |
O4 |
0 |
0 |
1/4 |
1 |
0.0197(3) |
The photoluminescence excitation and emission spectra of the Sr3.95Y6(SiO4)4(PO4)2O2:0.05Eu2+ are given in Fig. 3(a). It is clear that the PLE spectrum has an absorption band at 274 nm, which resulted from the 4f–5d transitions in Eu2+. The PL spectrum exhibits a green emission peak at 505 nm, which can be decomposed into two sub-bands with Gauss imitating decomposition at 498 nm and 555 nm, respectively (Fig. 3(b)). Therefore, Eu2+ can occupy two cationic lattices, which is consistent with the refined results. The lattice site centers of Eu2+ can be determined using the van Uitert empirical equation:33
|
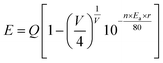 | (1) |
where
E is the position of the low-energy of the 5f excited-state (cm
−1) or the emission peak of Eu
2+,
Q is 34
![[thin space (1/6-em)]](https://www.rsc.org/images/entities/char_2009.gif)
000 cm
−1,
V is 2,
n is the anion number around Eu
2+,
Ea is a constant,
r is the effective radius of Sr
2+ (nm). Therefore,
E is negatively correlated with
n. Two different luminescent centers, having nine and seven coordinations, contribute to the fluorescence emission peaks at 498 and 555 nm, respectively.
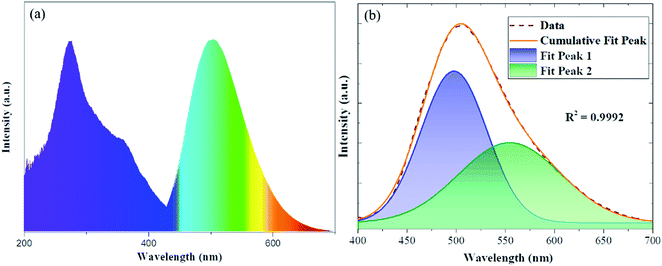 |
| Fig. 3 (a) Excitation and emission spectra of the Sr3.95Y6(SiO4)4(PO4)2O2:0.05Eu2+ sample, (b) PL spectrum of Sr3.95Y6(SiO4)4(PO4)2O2:0.05Eu2+ excited at 365 nm and Gaussian fitting result. Two Gaussian components are peaked at 498 nm and 555 nm. | |
Fig. 4(a) shows PL spectra of Sr(4−x)Y6(SiO4)4(PO4)2O2:xEu2+ (x = 0.01, 0.03, 0.05, 0.07, 0.09, and 0.12). It can be seen that the luminescence intensity increases first and then decreases. The luminescence properties of phosphors mainly depend on the rare-earth ion content, e.g., the luminous intensity is the highest when Eu2+ is 0.05.
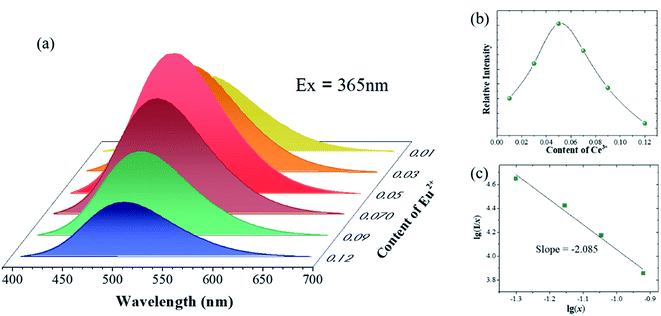 |
| Fig. 4 (a) PL spectra of Sr(4−x)Y6(SiO4)4(PO4)2O2:xEu2+ (x = 0.01, 0.03, 0.05, 0.07, 0.09, and 0.12) under the excitation of 365 nm, (b) intensities of Eu2+ as a function of Eu2+ doping concentration, and (c) linear fitting of log(x) versus log(I/x) in the Sr3.95Y6(SiO4)4(PO4)2O2:0.05Eu2+ samples. | |
As shown in Fig. 4(b), the luminescence intensity of Eu2+ first increases with the increase in the Eu2+ concentration, and reaches the maximum when x = 0.05; further, the emission intensity decreases caused by the concentration quenching effect. The concentration quenching is caused by the energy transfer of Eu2+. In order to establish the concentration quenching mechanism, the critical energy transfer distance RC can be calculated first:34
|
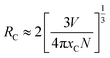 | (2) |
For the host with xC = 0.05, V = 540.53 Å3, N = 10, the obtained critical distance RC is 12.74 Å. According to Dexter theory, when the RC value is >5, a non-radiative transition occurs between the Eu2+ ions; therefore, the type of energy transfer can be described as follows:35,36
|
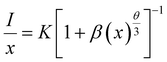 | (3) |
where
I is the emission intensity and
x is the activator concentration of Eu
2+,
θ = 6, 8, and 10 for dipole–dipole (d–d), dipole–quadrupole (d–q), and quadrupole–quadrupole (q–q), respectively.
37,38 Fig. 4(c) shows the relational curve between log(
I/
x) and log(
x) in the Sr
3.95Y
6(SiO
4)
4(PO
4)
2O
2:0.05Eu
2+ phosphors under the 365 nm light irradiation with line slope of −2.085, and the calculated
θ value based on
eqn (3) is 6.255, which means that the concentration quenching mechanism of Eu
2+ in the sample is d–d.
The PL decay curve of the Eu2+ phosphor was detected at 365 nm, which can be used to determine the fluorescence lifetime. Fig. 5 describes the decay curves of the phosphor, which can be fitted by a double-exponential equation:39,40
|
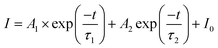 | (4) |
where
I0 is the initial emission intensity,
t represents time,
A1 and
A2 are invariant constants after fitting, and
τ1 and
τ2 are two different decay lives. The calculated Eu
2+ lifetime is 0.613 μs.
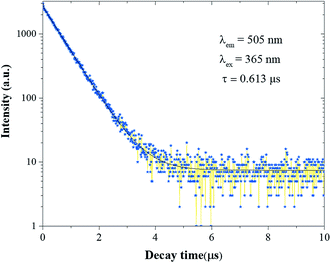 |
| Fig. 5 Decay curves of Eu2+ in the Sr3.95Y6(SiO4)4(PO4)2O2:0.05Eu2+ phosphor. | |
The variable temperature fluorescence test was carried out to show the thermal stability of the sample. Fig. 6(a) presents the relationship between the emitted light color and the ambient temperature in the wavelength range from 400 to 700 nm. As shown in Fig. 6(b), the emission intensity of the sample is 63% of that at room temperature with the temperature rising to 150 °C, indicating an excellent thermal stability. In addition, the strongest emission peak moves from 502 to 490 nm. The emission band has slight blue shift with an increase in temperature. The activation energy (E) can be obtained by the Arrhenius equation:41,42
|
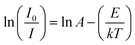 | (5) |
where
I(0) is the initial emission intensity of the phosphor at 25 °C and
I(
T) is the emission intensity at different temperatures,
c is constant,
E is the activation energy for thermal quenching, and
k is the Boltzmann constant (8.617 × 10
−5 eV). The plot of ln[(
I0/
I)
−1]
vs. 1/
kT in
Fig. 6(c) follows a line trend, obtaining 0.210 eV as the activation energy
E.
Fig. 6(d) shows that the calculated CIE chromaticity coordinates of Sr
3.95Y
6(SiO
4)
4(PO
4)
2O
2:0.05Eu
2+ is (0.233, 0.446), and the green emission can be observed clearly from the image of Sr
3.95Y
6(SiO
4)
4(PO
4)
2O
2:0.05Eu
2+ at ultraviolet excitation.
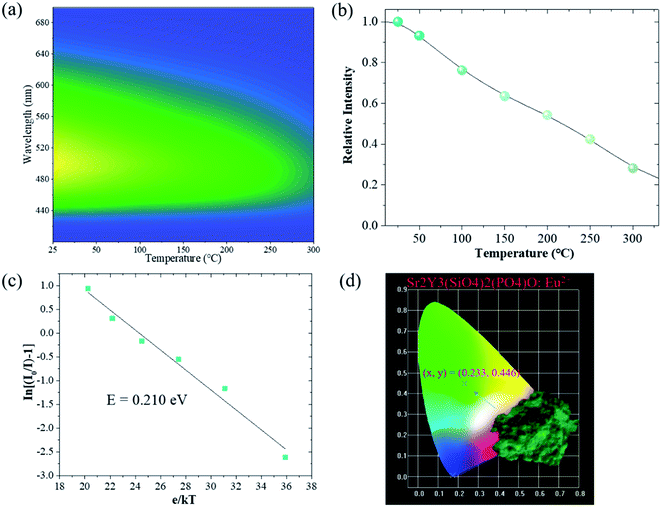 |
| Fig. 6 (a) Temperature-dependent emission intensity of the Sr3.95Y6(SiO4)4(PO4)2O2:0.05Eu2+ sample in the wavelength range of 400–700 nm, (b) temperature dependence of the emission intensity, (c) the plot of ln[(I0/IT) − 1] varied as a temperature function, (d) the CIE chromaticity coordinate diagram for Sr3.95Y6(SiO4)4(PO4)2O2:0.05Eu2+ and the inset shows a digital photograph of the green-emitting phosphor. | |
4. Conclusions
A series of green phosphors Sr2Y3(SiO4)2(PO4)O2:Eu2+ with apatite structure were successfully synthesized via a traditional high-temperature solid-phase reaction and their luminescent properties were investigated. The phosphors generated a strong green emission band at 505 nm with an obvious broad band ranging from 400 to 700 nm. The determined optimal Eu2+ concentration of Sr2Y3(SiO4)2(PO4)O:Eu2+ is 0.05. The experimental results and theoretical calculations suggest that the concentration quenching mechanism of Eu2+ in the Sr3.95Y6(SiO4)4(PO4)2O2:0.05Eu2+ phosphor is d–d and the decay time of it was around 0.613 μs. All the investigations show that the as-prepared phosphor can be a green phosphor having potential research value.
Conflicts of interest
There are no conflicts to declare.
Acknowledgements
The current work has been supported by the National Natural Science Foundation of China project (Grant No. 41672044 and 41802040), the Fundamental Research Funds for the Central Universities (Grant No. 2652017091), and China Postdoctoral International Exchange Program.
References
- R. P. Cao, Y. L. Cao, T. Fu, S. H. Jiang, W. S. Li, Z. Y. Luo and J. W. Fu, Synthesis and luminescence properties of novel red-emitting R3P4O13:Bi2+ (R = Sr and Ba) phosphors, J. Alloys Compd., 2016, 661, 77–81 CrossRef CAS.
- L. Li, X. H. Tang, Z. Q. Jiang, X. J. Zhou, S. Jiang, X. B. Luo, G. T. Xiang and K. N. Zhou, NaBaLa2(PO4)3: A novel host lattice for Sm3+-doped phosphor materials emitting reddish-orange light, J. Alloys Compd., 2017, 701, 515–523 CrossRef CAS.
- R. P. Cao, C. L. Liao, F. Xiao, G. T. Zheng, W. Hu, Y. M. Guo and Y. X. Ye, Emission enhancement of LiLaMo2O8:Eu3+ phosphor by co-doping with Bi3+ and Sm3+ ions, Dyes Pigm., 2018, 149, 574–580 CrossRef CAS.
- M. Runowski, S. Goderski, J. Paczesny, M. Księżopolska-Gocalska, A. Ekner-Grzyb, T. Grzyb, J. D. Rybka and M. Giersig, Stefan Lis Preparation of Biocompatible, Luminescent-Plasmonic Core/Shell Nanomaterials Based on Lanthanide and Gold Nanoparticles Exhibiting SERS Effects, J. Phys. Chem. C, 2016, 120, 23788–23798 CrossRef CAS.
- W. Wang, H. Yang, Y. X. Liu, X. H. Yun, Y. Wei and G. G. Liu, (Ln3+ = Ce3+, Eu3+)-doped oxyapatite-type phosphors, CrystEngComm, 2020, 22, 311–319 RSC.
- B. Han, Y. Z. Dai, J. Zhang, B. B. Liu and H. Z. Shi, Development of near-ultraviolet-excitable single-phase white-light-emitting phosphor KBaY(BO3)2:Ce3+,Dy3+ for phosphor-converted white light-emitting-diodes, Ceram. Int., 2010, 71, 1–34 Search PubMed.
- M. Runowski and S. Lis, Preparation and photophysical properties of luminescent nanoparticles based on lanthanide doped fluorides (LaF3:Ce3+, Gd3+, Eu3+), obtained in the presence of different surfactants, J. Alloys Compd., 2014, 597, 63–71 CrossRef CAS.
- P. Du, W. G. Ran, W. P. Li, L. H. Luo and X. Y. Huang, Morphology evolution of Eu3+-activated NaTbF4nanorods: a highly-efficient near-ultraviolet light-triggered red-emitting platform towards application in white light-emitting diodes, RSC Adv., 2019, 7, 10802–10809 CAS.
- Q. Y. Bai, Z. J. Wang, P. L. Li, S. C. Xu, T. Li and Z. P. Yang, Zn2−aGeO4:aRE and Zn2Ge1−aO4:aRE (RE = Ce3+, Eu3+, Tb3+, Dy3+): 4f–4f and 5d–4f transition luminescence of rare earth ions under different substitution, RSC Adv., 2016, 6, 102183–102192 RSC.
- W. J. Tang, Z. X. Huang and J. X. Liang, Controllable luminescence in Eu2+-doped Li2Sr2Al(PO4)3 phosphor via tuning the Eu2+ concentration and codoping Ce3+, J. Alloys Compd., 2019, 778, 612–617 CrossRef.
- H. R. Li, Y. J. Liang, Y. L. Zhu, S. Q. Liu, J. H. Chen, H. Zhang and Y. J. Chen, Investigation of local structure distortion and electron cloud interaction on emission-band broadening induced by the concentration perturbation effect of cation substitution in BaY2Si3O10:Eu phosphors, CrystEngComm, 2019, 21, 4771–4785 RSC.
- Q. Zhang, X. Ding and Y. H. Wang, Novel highly efficient blue-emitting SrHfSi2O7:Eu2+ phosphor: a potential color converter for WLEDs and FEDs, Dyes Pigm., 2019, 163, 168–175 CrossRef CAS.
- S. S. Lin, H. Lin, Q. M. Huang, Y. Cheng, J. Xu, J. M. Wang, X. Q. Xiang, C. Y. Wang, L. Q. Zhang and Y. S. Wang, Optical Storage: A Photostimulated BaSi2O5:Eu2+,Nd3+ Phosphor-in-Glass for Erasable-Rewritable Optical Storage Medium, Laser Photonics Rev., 2019, 13(4), 1863–8880 Search PubMed.
- M. Fei, Y. F. Tian, Z. Zhang, L. R. He, J. Chen, F. D. Bao, P. J. Zhang, Q. H. Zhang and L. Chen, The competitive mechanisms of nano-SiO2 and reaction temperature on phase transformation and Eu2+ site occupation in Sr2SiO4:Eu2+ phosphor, J. Alloys Compd., 2017, 728, 231–240 CrossRef CAS.
- S. A. Khan, H. Zhong, W. W. Ji, N. Z. Khan, H. Abadikhah, L. Y. Hao, X. Xu, S. Agathopoulos and Q. L. Bao, Crystal-site engineering for developing tunable green light emitting Ba9Lu2Si6O24:Eu2+ phosphors for efficient white LEDs, J. Alloys Compd., 2018, 767, 374–381 CrossRef CAS.
- A. M. I. Ibrahim, Structural, electronic and optical properties of prominent M2Si5N8:Eu2+ phosphors (M = Mg, Ca, Sr, Ba) from the ground–state and excited–state first principles calculations, J. Alloys Compd., 2019, 775, 30–38 CrossRef.
- X. G. Zhang, F. W. Mo, Z. P. Zhu and Z. Y. Guo, Structure, site-occpancy and luminescent properties of a novel blue phosphor Na3ZrMgP3O12:Eu2+, Mater. Res. Bull., 2018, 106, 396–402 CrossRef CAS.
- P. Dorenbos, A Review on How Lanthanide Impurity Levels Change with Chemistry and Structure of Inorganic Compounds, ECS J. Solid State Sci. Technol., 2013, 2, R3001–R3011 CrossRef CAS.
- H. G. Guo, Y. H. Wang, G. Li, J. Y. Liu and Y. Y. Li, The persistent energy transfer of Eu2+ and Dy3+ and luminescence properties of a new cyan afterglow phosphor alpha-Ca3(PO4)2:Eu2+, Dy3+, RSC Adv., 2016, 6, 101731–101736 RSC.
- X. C. Wang, Z. Y. Zhao, Q. S. Wu, Y. Y. Li, C. Wang, A. J. Mao and Y. H. Wang, Synthesis, structure, and luminescence properties of SrSiAl2O3N2:Eu2+ phosphors for light-emitting devices and field emission displays, Dalton Trans., 2015, 44, 11057–11066 RSC.
- X. X. Ma, L. F. Mei, H. K. Liu, L. B. Liao, K. Nie, Y. Q. Liu and Z. H. Li, Synthesis and luminescence properties of Eu2+-activated phosphor Ba3LaK(PO4)3F for n-UV white-LEDs, Polyhedron, 2016, 119, 223–226 CrossRef CAS.
- G. Li, Y. H. Wang, D. W. Liu, H. J. Guo and J. Liu, Cyan longlasting phosphorescence in green emitting phosphors Ba2Gd2Si4O13:Eu2+, RE3+ (RE3+ = Dy3+, Ho3+, Tm3+, Nd3+ and Tb3+), RSC Adv., 2016, 6, 7024–7032 Search PubMed.
- S. M. Xu, K. Tang, T. Han, L. L. Luo and D. C. Zhu, Effect of Eu2+ on the luminescence properties of Ba1.52−xZn0.2Li0.4SiO4:0.05Ce3+, 0.03Mn2+, xEu2+ phosphor, Optik, 2016, 127, 2370–2373 CrossRef CAS.
- B. Deng, J. Chen, H. Liu and C. S. Zhou, Photoluminescence properties of dysprosium doped novel apatite-type Ba2Y3(SiO4)3F white-emitting phosphor, J. Mater. Sci.: Mater. Electron., 2019, 30, 7507–7513 CrossRef CAS.
- N. Guo, C. Z. J. Jia, Y. F. Zhao, R. Z. Ouyang and W. Lu, White-Emitting Tuning and Energy Transfer in Eu2+/Mn2+-Substituted Apatite-Type Fluorophosphate Phosphors, J. Am. Ceram. Soc., 2015, 98, 1162–1168 CrossRef CAS.
- J. R. Peet, A. Piovano, M. R. Johnson and I. Radosavljevic Evans, Location and orientation of lone pairs in apatite-type materials: a computational study, Dalton Trans., 2015, 98(4), 1162–1168 Search PubMed.
- W. J. Tang and F. Zhang, A Single-Phase Emission-Tunable Ca5(PO4)3F:Eu2+,Mn2+ Phosphor with Efficient Energy Transfer for White LEDs, Eur. J. Inorg. Chem., 2014, 21, 3387–3392 Search PubMed.
- S. J. Dhoble, Preparation and characterization of the Sr5(PO4)3Cl:Eu2+ phosphor, J. Phys. D: Appl. Phys., 2000, 33(2), 158–161 CrossRef CAS.
- G. Seeta Rama Raju, J. Y. Park, G. P. Nagaraju, E. Pavitra, H. K. Yang, B. K. Moon, J. S. Yu, Y. S. Huh and J. H. Jeong, Evolution of CaGd2ZnO5:Eu3+ nanostructures for rapid visualization of latent fingerprints(Article), J. Mater. Chem. C, 2017, 5, 4246–4256 RSC.
- M. M. Shang, D. L. Geng, D. M. Yang, X. J. Kang, Y. Zhang and J. Lin, Luminescence and Energy Transfer Properties of Ca2Ba3(PO4)3Cl and Ca2Ba3(PO4)3Cl:A (A = Eu2+/Ce3+/Dy3+/Tb3+) under UV and Low-Voltage Electron Beam Excitation, Inorg. Chem., 2013, 52, 3102–3112 CrossRef CAS PubMed.
- Bruker AXS, TOPAS V4: General Profile and Structure Analysis Software for PowderDiffraction Data – User's Manual, Bruker AXS, Karlsruhe, Germany, 2008 Search PubMed.
- Y. Z. Fang, F. X. Liu, J. S. Hou, Y. Zhang, X. F. Zheng, N. Zhang, G. Y. Zhao, M. S. Liao, G. Z. Dai, M. Q. Long and Y. F. Liu, Photoluminescence properties of blue light excited Ca8La2(PO4)6O2:Eu3+ red phosphors, J. Lumin., 2016, 177, 280–2885 CrossRef CAS.
- L. G. van Uitert, An empirical relation fitting the position in energy of the lower d-band edge for Eu2+ OR Ce3+ in various compounds, J. Lumin., 1984, 29, 1–9 CrossRef CAS.
- G. Blasse, Energy transfer in oxidic phosphors, Phys. Lett. A, 1968, 28, 444–445 CrossRef CAS.
- J. Y. Sun, X. Y. Zhang, Z. G. Xia and H. Y. Du, Synthesis and luminescence properties of novel LiSrPO4:Dy3+, phosphor, Mater. Res. Bull., 2011, 46, 2179–2182 CrossRef CAS.
- L. M. Liang, L. F. Mei, H. K. Liu, C. C. Wang and L. B. Liao, Intense broad-band absorption and blue-emitting Ca9La(PO4)5(SiO4)Cl2:Eu2+ phosphor under near-ultraviolet excitation, J. Lumin., 2019, 206, 154–157 CrossRef CAS.
- Q. F. Guo, B. Ma, L. B. Liao, M. S. Molokeev, L. F. Mei and H. K. Liu, Crystal structure and luminescence properties of novel Sr10-x(SiO4)3(SO4)3O:xEu2+ phosphor with apatite structure, Ceram. Int., 2016, 42, 11687–11691 CrossRef CAS.
- H. K. Liu, Y. Y. Zhang, L. B. Liao, Q. F. Guo and L. F. Mei, Synthesis, broad-band absorption and luminescence properties of blue-emitting phosphor Sr8La2(PO4)6O2:Eu2+ for n-UV white-light-emitting diodes, Ceram. Int., 2014, 40, 13709–13713 CrossRef CAS.
- B. Ramesha, G. Devarajulua, B. Deva Prasad Rajub, G. BhaskarKumarc, G. R. Dillipd, A. N. Banerjeed and S. W. Jood, Determination of strain, site occupancy,photoluminescent, and thermoluminescent-trapping parameters of Sm3+-doped NaSrB5O9 microstructures, Ceram. Int., 2016, 42, 1234–1245 CrossRef.
- H. Ye, M. Y. He, T. S. Zhou, Q. F. Guo, J. L. Zhang, L. B. Liao, L. F. Mei, H. K. Liu and M. Runowski, A novel reddish-orange fluorapatite phosphor, La6-xBa4(SiO4)6F2:xSm3+ - Structure, luminescence and energy transfer properties, J. Alloys Compd., 2018, 757, 79–86 CrossRef CAS.
- S. Arrhenius, Über Die Reaktionsgeschwindigkeitbei der Inversion von RohrzuckerDurchSäuren, Z. Phys. Chem., 1889, 4, 226–248 Search PubMed.
- J. W. Qiao, L. X. Ning, M. S. Molokeev, Y. C. Chuang, Q. L. Liu and Z. Xia, Eu2+ site preferences in the mixed cationK2BaCa(PO4)2 and thermally stable luminescence, J. Am. Chem. Soc., 2018, 140, 9730–9736 CrossRef CAS PubMed.
|
This journal is © The Royal Society of Chemistry 2020 |
Click here to see how this site uses Cookies. View our privacy policy here.