DOI:
10.1039/C9RA10257D
(Paper)
RSC Adv., 2020,
10, 1658-1665
Preparation, structure and up-conversion luminescence properties of novel cryolite K3YF6:Er3+, Yb3+
Received
6th December 2019
, Accepted 31st December 2019
First published on 9th January 2020
Abstract
Cryolite is a suitable host for up-conversion luminescent materials due to its low phonon energy and good optical transparency. In this work, a novel up-conversion material K3YF6:Yb3+, Er3+ with a cryolite structure was prepared successfully by a solid state method. The crystal structure, morphology, composition and up-conversion luminescence properties of the as-prepared sample were characterized by X-ray diffractometry (XRD), field emission scanning electron microscopy (SEM) and fluorescence spectrometer in detail. K3YF6:Er3+, Yb3+ has a cryolite structure. Under 980 nm excitation, the as-prepared sample can generate slight green emission at 524 and 546 nm (attributed to 2H11/2 → 4I15/2 transition, 4S3/2→4I15/2 transition of Er3+) and strong red emission at 661 and 672 nm (corresponding to 4F9/2 → 4I15/2 transition, 4I9/2 → 4I15/2 transition of Er3+). All the green and red up-conversion emission of K3YF6:Er3+, Yb3+ transfer and electronic transition process of the red and green light the sample emitted, the possible luminescence mechanism is discussed in this paper.
1. Introduction
Under the excitation of ultraviolet light, visible light or infrared light source, a material with certain luminescent properties is called photoluminescent material. Photoluminescent materials are classified into down-conversion luminescent materials and up-conversion luminescent materials, which are widely used in illumination sources, plasma displays, luminescent coatings, etc.1–3 The material that absorbs short-wave radiation and then emits long-wave radiation is called a down-converting luminescent material, while the material that first absorbs long-wave radiation and then emits short-wave radiation is called an up-converting luminescent material. The up-converting luminescent material can be excited with low energy near infrared (NIR) radiation, and emits the light of higher energy in a visible range, due to the multi-photon absorption followed by an anti-Stokes emission.4–8 So far, rare earth doped up-conversion materials have aroused widespread attention due to their important applications9–14 in the fields of the national economy and national defense construction, such as in infrared quantum counters, night vision systems, light-emitting diodes, other laser materials, etc.15–18
The urgent problem to be solved by up-converting luminescent materials is to find a suitable host. Although the host does not constitute an excitation level, it provides a suitable crystal field environment for the sensitizer ions and activator ions to cause matching energy level splitting to produce energy transfer and up-conversion luminescence.19,20 At present, there are four main types of up-conversion host materials: oxide systems, sulfide-containing systems, halide systems (excluding fluorine), and fluoride systems. Compared to the other systems, the fluoride system has the advantages of low phonon energy, wide light transmission range, and easy to form waveguides and so on. Recently, fluoride has attracted the attention of researchers as the most popular up-conversion host.21,22
Cryolite, an important fluoride, has general formula of A3BF6 (A = Li, Na, K, NH4, etc.; B = Al, Sc, V, Cr, Fe, Y, Ga, etc.), which is one of the most promising up-conversion host in the field of luminescent materials due to its low phonon energy, stable chemical composition and good optical transparency. Recently, compounds with cryolite structure have been widely used as host for luminescent materials, such as: K3GaF6:Mn4+,23 Na3GaF6:Mn4+,24 K3LuF6:Er3+,25 K3LuF6:Ce3+.26 Na3AlF6:Mn4+ red phosphor was prepared via the coprecipitation and hydrothermal methods, and the phosphor was a promising candidate for application in warm WLEDs.27 By controlling the concentration ratio of Eu3+/Tb3+, K3LuF6:Tb3+, Eu3+ phosphors could be adjusted from green to yellowish pink.28 K3YF6 is an a cryolite compound. Marek29 reported the crystal structure and vibrational properties of K3YF6 solid solution and considered it suitable as luminescent materials. These works mainly focus on the down-conversion of cryolite structure. So far, there is little research on K3YF6 to be used as an up-conversion host. In addition, Er3+/Yb3+ doped monoclinic K3YF6 have not been reported.
Owing to the large absorption section and wide absorption region of Yb3+, the up-conversion luminescence intensities of Er3+ are largely enhanced in (Er3+, Yb3+) co-doped materials. Some works of Er3+/Yb3+ co-doped up-conversion luminescent material have been done, such as: K2GdF5:Yb3+/Er3+,30 Y2O3:Er3+/Yb3+,31 Ba2LaF7:Er3+/Yb3+,32 Na5Lu9F32:Er3+/Yb3+.33
Herein, a novel cryolite up-conversion luminescent material K3YF6:Yb3+, Er3+ was successfully prepared by a solid state method. The crystal structure, elemental composition and up-conversion luminescence properties of the as-prepared samples were investigated in detail. Besides, the possible luminescence mechanism and electron transition process proposed were also discussed in this paper.
2. Experimental details
2.1 Synthesis
K3YF6:Er3+, Yb3+ was prepared by a solid state method. The raw materials of K2CO3 (A.R.), NH4HF2 (A.R.), Y2O3 (99.99%), Er2O3 (99.99%) and Yb2O3 (99.99%) were purchased from Aladdin. The starting materials were weighted with electronic balance according to stoichiometric ratio. To prevent the fluorine from volatilizing during heating, NH4HF2 is treated with an excess of 30%. The weighed raw materials were ground in an agate mortar until well mixed. Then, they were transferred to a muffle furnace and calcined at 750 °C for 3 h. After cooling to room temperature, the samples were taken out and grinded into powder again for a series of test followed.
2.2 Measurement
The structure of the prepared samples was characterized by X-ray diffraction (XRD) measurements using an X-ray powder diffractometer (D8 Advance, Bruker, Germany; Cu Kα radiation, λ = 0.15418 nm) at 40 kV and 100 mA, and the step width is 0.02° (2θ) with 2θ ranging from 10 to 70°. The morphology and energy dispersive X-ray (EDX) spectra of the as-prepared samples were obtained by a field emission scanning electron microscope (SEM, JSM-6701F, Hitachi, Japan) with 15 kV acceleration voltage. Under room temperature, the fluorescence emission spectra of samples were measured on a fluorescence spectrophotometer (Hitachi F4600) with an external tunable 980 nm infrared laser as excitation source.
3. Results and discussions
As we know, cryolite has two crystal forms, that is, mono-clinic and cubic. Fig. 1 shows the crystal structure for mono-clinic K3YF6 (space group P21/n). As shown in Fig. 1, Y3+ can occupy strongly distorted octahedral sites with centrosymmetric Ci local symmetry with six-fold coordinated by fluorine ions.34 Since the electricity valences and ionic radii of Yb3+/Er3+ and Y3+ are close to each other, it is considered that Yb3+/Er3+ replaces the site for Y3+ during the doping process.
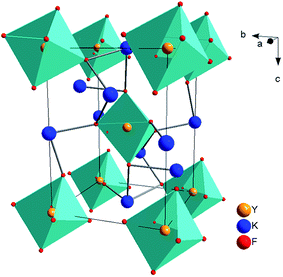 |
| Fig. 1 Crystal structure of mono-clinic K3YF6. | |
The luminous efficiency of the up-converting luminescent material is not only related to the host structure, but also to the phase purity of the prepared material. Therefore, the structure of the prepared sample was analyzed by an X-ray powder diffractometer. Fig. 2 shows the XRD patterns of K3YF6:xEr3+ (x = 0.005, 0.01, 0.03, 0.05, 0.07, and 0.10), and the data for K3YF6 (JCPDF no. 27-467) is shown a reference. As illustrated in Fig. 2, the diffraction peak positions of the as-prepared samples match well with these of standard K3YF6 (JCPDF no. 27-467) without any impurity peak, indicating that all the as-prepared samples were cryolite compound. The increasing doping ratios of Er3+ did not cause K3YF6 structural changes.35–37
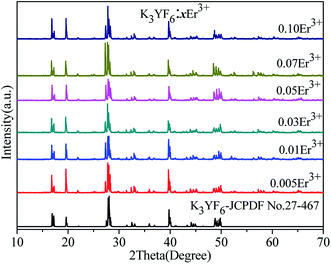 |
| Fig. 2 XRD patterns of K3YF6:xEr3+ (x = 0.005, 0.01, 0.03, 0.05, 0.07, and 0.10), and the data for K3YF6 (JCPDF no. 27-467) is shown a reference. | |
The emission spectra of as-prepared K3YF6:xEr3+ (x = 0.005, 0.01, 0.03, 0.05, 0.07 and 0.10) samples are shown in Fig. 3. The centers of the six emission peaks are 526 (2H11/2 → 4F15/2), 535 (2H11/2 → 4F15/2), 548 (4S3/2 → 4F15/2), 556 (4S3/2 → 4F15/2), 661 (4F9/2 → 4F15/2) and 672 nm (4I9/2 → 4F15/2) in the green and red regions, respectively. Remarkably, for K3YF6:0.03Er3+, the emission peak at 672 nm is the highest and the inset shows the dependence of luminescence intensity on the Er3+ doping ratio at 672 nm. With the increasing for Er3+ doping ratio from 0.005 to 0.10, the intensity of the emission peak of the samples firstly increased and then decreased and finally stabilized. When the Er3+ doping ratio was 0.03, the intensity of the emission peak at 672 nm was the highest. Fig. 4 shows the visual illumination of K3YF6:xEr3+ (x = 0.005, 0.01, 0.03, 0.05, 0.07, and 0.10) under 980 nm laser excite. As shown in Fig. 4, the emitted light changes from green to red, and the luminous intensity increases firstly and then decreases. When the Er3+ doping ratio is 0.03, the sample is the brightest and present red. This lays the foundation for the preparation of the up-conversion luminescent material with excellent performance.
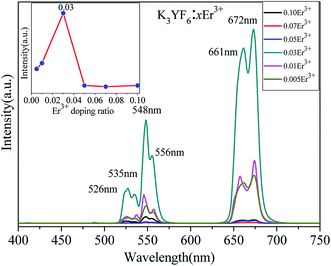 |
| Fig. 3 The emission spectra of K3YF6:xEr3+ (0.005, 0.01, 0.03, 0.05, 0.07 and 0.10) excited by 980 nm infrared laser, with the doping ratio dependences of emission intensity at 672 nm shown in the inset. | |
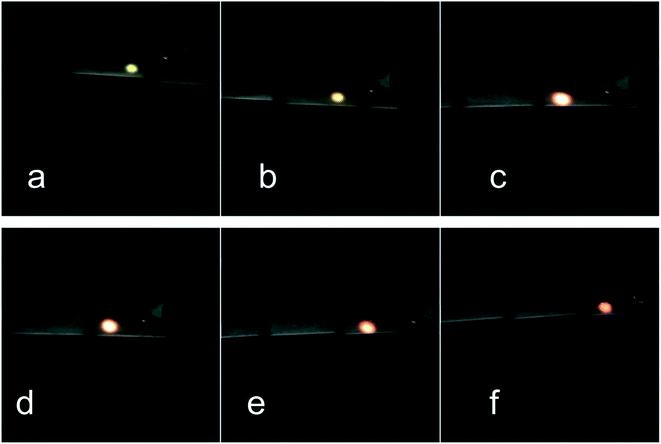 |
| Fig. 4 The luminescence of K3YF6:xEr3+ (x = 0.005 (a), 0.01 (b), 0.03 (c), 0.05 (d), 0.07 (e) and 0.10 (f)) under 980 nm laser irradiation. | |
A series of Er3+/Yb3+ co-doped K3YF6 up-conversion luminescent materials have been prepared. The microscopic, structures of the K3YF6:0.03Er3+/yYb3+ (y = 0.01, 0.03, 0.07, 0.09, 0.12, 0.15, and 0.18) were determined. Fig. 5 shows the XRD patterns of K3YF6:0.03Er3+/yYb3+ (y = 0.01, 0.03, 0.07, 0.09, 0.12, 0.15 and 0.18), and the data for K3YF6 (JCPDF no. 27-467) is shown a reference. It can be found that the diffraction peaks of the as-prepared samples match well with K3YF6 (JCPDS 27-467), indicating that Er3+/Yb3+ co-doping does not change the crystal structure significantly.
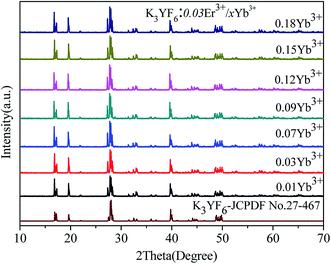 |
| Fig. 5 XRD patterns of K3YF6:0.03Er3+/yYb3+ (y = 0.01, 0.03, 0.07, 0.09, 0.12, 0.15 and 0.18), and the data for K3YF6 (JCPDF no. 27-467) is shown a reference. | |
Fig. 6 shows the SEM image and elemental mapping image of K3YF6:0.03Er3+, 0.12Yb3+ sample (a) and the EDX spectrum of K3YF6:0.03Er3+, 0.12Yb3+ (b). As shown in Fig. 6(a), the prepared sample appeared to be irregular particles with a grain size of about 10 μm. And the sample appear to partly agglomerate, which can be explained by the inhibition of grain growth due to the accumulation of vacancies on the grain boundaries when the Er3+/Yb3+ with higher valency occupies the K+ site. The element mapping results shown in Fig. 6(a) indicate that K, F, Y, Er, and Yb elements can be observed in K3YF6:0.03Er3+, 0.12Yb3+, and all the elements in the sample are uniformly distributed. The K (31.5%), F (36.5%), Y (29.5%), Er (0.4%), and Yb (2.0%) peaks were observed in the EDX spectrum of Fig. 6(b), further indicating that the measured lanthanide atomic ratios of K3YF6:0.03Er3+, 0.12Yb3+ are close to the calculated values.
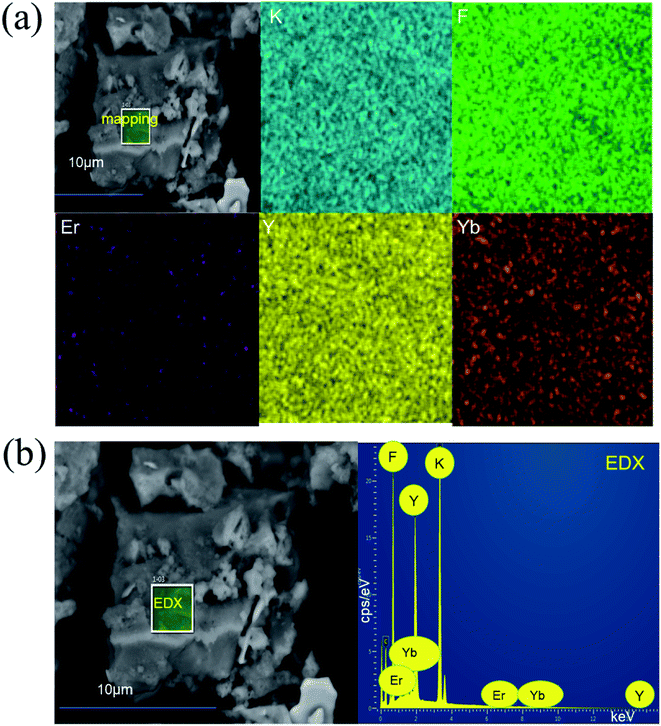 |
| Fig. 6 (a) The SEM image and elemental mapping image of K3YF6:0.03Er3+, 0.12Yb3+ sample; (b) the EDX spectrum of K3YF6:0.03Er3+, 0.12Yb3+. | |
The up-conversion emission spectra of K3YF6:0.03Er3+, yYb3+ (y = 0.01, 0.03, 0.07, 0.09, 0.12, 0.15 and 0.18) are shown in Fig. 7. Four emission peaks at 524, 546, 661 and 672 nm in the green and red regions can be founded, which can be ascribed to the transitions from 2H11/2, 4S3/2, 4F9/2, and 4I9/2 states to 4I15/2 state of Er3+, respectively.38,39 The inset shows the effect of Yb3+ doping ratio on the luminescence intensity of the K3YF6:0.03Er3+, yYb3+ (y = 0.01, 0.03, 0.07, 0.09, 0.12, 0.15 and 0.18). With the increasing for Yb3+ from 0.01 to 0.18, the emission intensity of the samples firstly increased and then decreased. When the Yb3+ doping ratio was 0.12, the intensity of the emission peak at 672 nm was the highest. Fig. 8 is the visual illumination of K3YF6:0.03Er3+, yYb3+ (y = 0, 0.01, 0.03, 0.07, 0.09, 0.12, 0.15 and 0.18) under 980 nm laser excite. As is shown in Fig. 8, the emitted light changes from light red to deep red, and the luminous intensity increases first and then decreases. When the Yb3+ doping ratio is 0.12, the sample is the brightest and present deep red.
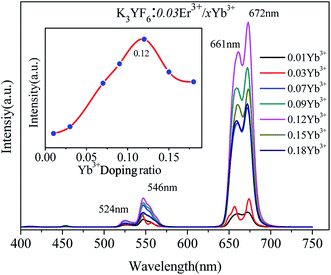 |
| Fig. 7 Up-conversion emission spectrum of K3YF6:0.03Er3+/yYb3+ (y = 0.01, 0.03, 0.07, 0.09, 0.12, 0.15 and 0.18) samples excited by 980 nm laser, with the doping ratio dependences of emission intensity at 672 nm shown in the inset. | |
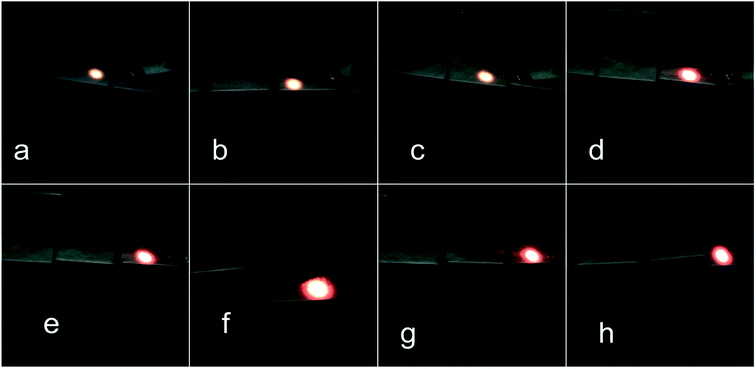 |
| Fig. 8 The luminescence of K3YF6:0.03Er3+, yYb3+ (y = 0 (a), 0.01 (b), 0.03 (c), 0.07 (d), 0.09 (e), 0.12 (f), 0.15 (g) and 0.18 (h)) under 980 nm laser irradiation. | |
The pump power dependent UC emission spectra of K3YF6:0.05Er3+, 0.12Yb3+ are shown in Fig. 9. With the increase of pump power from 257 to 300 mW, the up-conversion emission intensity shows an upward trend. The relationship between up-conversion intensity and excitation pump power can be applied as follow:
In which
I and
P are the intensity of the up-conversion emission and the power of the excitation pump, respectively.
n represents the number of phonons that need to populate the upper excited state energy level.
30 In order to obtain the value of
n, the double logarithmic figure of the emission intensity of green and red up-conversion emission with pump power for K
3YF
6:0.05Er
3+, 0.15Yb
3+ were depicted in inset of
Fig. 9 The green and red dots represent up-conversion emission peaks at 546 and 672 nm, respectively. The experimental data are fitted in a straight line. The
n values of green and red up-conversion lines are calculated to be 2.53 and 2.04, respectively. The number of
n is near to 2, indicating that the green and red up-conversion emission of K
3YF
6:0.03Er
3+, 0.12Yb
3+ all belong to two-photon process.
40
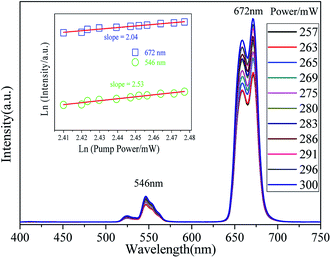 |
| Fig. 9 Pump power dependence UC emission spectra of K3YF6:0.03Er3+, 0.12Yb3+; and the inset shows lnarithmic plots of green and red UC emission intensity versus pump power for K3YF6:0.03Er3+, 0.12Yb3+. | |
The possible up-conversion luminescence mechanism and electron transition process for the sample of K3YF6:0.01Er3+, 0.12Yb3+ to emit red and green light are shown in Fig. 10. Under 980 nm laser excitation, the electron of the sensitizer Yb3+ absorbs a 980 nm photon from the energy level 2F7/2 to the 2F5/2 level. When the excited state is unstable, the electrons of Yb3+ will return to the 2F7/2 level through the non-radiative transition, and the energy released during the transition will be transferred to the activator Er3+. The electrons in the ground state of Er3+ absorb the energy transmitted by Yb3+ and transited to the 4I11/2 level. Since the Er3+ and Yb3+ levels match very well, the electrons of the activator Er3+ can also directly absorb a photon of 980 nm from the ground state level 4I15/2 to the 4I11/2 excited state level. The specific process is as follows:
2F7/2 (Yb3+) + hν (980 nm) → 2F5/2 (Yb3+) |
2F5/2 (Yb3+) + 4I15/2 (Er3+) → 2F7/2 (Yb3+) + 4I11/2 (Er3+) |
4I15/2 (Er3+) + hν (980 nm) → 4I11/2 (Er3+) |
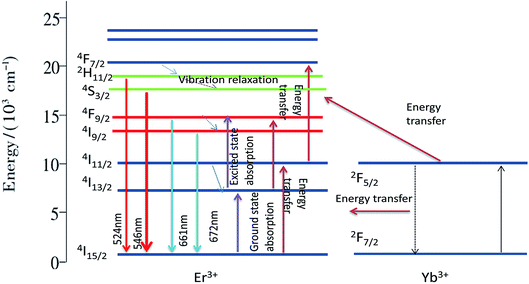 |
| Fig. 10 K3YF6:0.03Er3+, 0.12Yb3+ up-conversion luminescence mechanism. | |
Some electrons at the 4I11/2 level of Er3+ directly accept the energy released from the electronic transition in the excited state of Yb3+ back to the ground state, and jump up to the 4F7/2 level. Since the energy gap between 4F7/2 and 2H11/2, 2H11/2 and 4S3/2 is small, electrons at the 4F7/2 level will quickly relax to the 2H11/2/4S3/2 level without radiation. When the electron absorption energy at the 4I13/2 level shifts to the 4F9/2 level, the electrons of the 4F9/2 level will undergo a non-radiative transition to 4I9/2, and the electron will go back to the ground state of the 4I9/2 and 4F9/2 levels.
4. Conclusions
A series of up-conversion luminescent materials K3YF6:Er3+, Yb3+ with cryolite structure were prepared by a solid state method for the first time. XRD results indicated that all prepared K3YF6:Er3+, Yb3+ samples were pure phase, belonging to monoclinic system, space group P21/n. Under the 980 nm light exhibition, the K3YF6:Er3+, Yb3+ shows typical transition of Er3+, which composed of two parts in the visible region that green and red. When the doping ratio of Er3+is 0.03 and the doping ratio of Yb3+is 0.12, the luminous intensity reaches the maximum. According to the relation between the up-conversion intensity and the excitation pump power at 980 nm laser excitation, the two-photon process dominated in the red and green up-conversion processes of Er3+ ions. All the results indicated K3YF6:0.03Er3+, 0.12Yb3+ is an excellent up-conversion luminescent material.
Conflicts of interest
There are no conflicts to declare.
Acknowledgements
The current work has been supported by the National Natural Science Foundation of China project (Grant No. 41672044).
References
- J. Y. Sun, H. Y. Du and W. X. Hu, Solid luminescent materials, Chemical Industry Press, 2003 Search PubMed.
- J. Y. Li, Rare earth luminescent materials and their applications, Chemical Industry Press, 2003 Search PubMed.
- Y. Y. Liu and X. Wang, Controllable synthesis and up-conversion luminescence properties of Yb3+/Er3+ codoped (MLaFn)x (M = 0, K; n = 3, 4; x = 1, 1.5) of nanomaterials, Chin. J. Lumin., 2019, 40(2), 1000–7032 Search PubMed.
- G. Y. Zhang, Q. P. Qiang, S. S. Du and Y. H. Wang, An up-conversion luminescence and temperature sensor based on Yb3+/Er3+co-doped GdSr2AlO5, RSC Adv., 2018, 8, 9512–9518 RSC.
- N. Z. Zhang, M. S. Molokeev, Q. L. Liu and Z. G. Xia, Pure red up-conversion luminescence and optical thermometry of Er3+ doped sensitizer-rich SrYbInO4 phosphors, J. Mater. Chem. C, 2018, 6, 7361–7366 RSC.
- W. Xu, X. Chen and H. W. Song, Manipulation of local electromagnetic field in up-conversion luminescence of rare earth ions, Chin. J. Lumin., 2018, 39(1), 1–26 Search PubMed.
- Y. Fu, J. Leng, M. M. Xing, Y. Tian and X. X. Luo, Synthesis and luminescence properties of Ca3Y2Si3O12:Tm3+, Yb3+ up-conversion phosphors, Chin. J. Lumin., 2017, 38(5), 561–566 CrossRef.
- X. W. Yao, G. F. Yao, Y. Li and J. S. Zhang, Synthesis and up-conversion luminescence of NaLuF4: Yb3+, Er3+ microcrystals, Chin. J. Lumin., 2013, 34(10), 1319–1323 CrossRef CAS.
- Y. M. Yang, F. Y. Jiao, H. X. Su and Z. Q. Li, Preparation and up-conversion efficiencies of Yb3+, Er3+ co-doped BaGd2ZnO5, Chin. J. Lumin., 2012, 33(12), 1319–1323 CrossRef CAS.
- L. Zhao, D. Meng, Y. Li, Y. Zhang and H. Wang, Tunable emitting phosphors K3Gd(PO4)2:Tm3+/Dy3+ for light-emitting diodes and field emission displays, J. Alloys Compd., 2017, 728, 564–570 CrossRef CAS.
- I. E. Kolesnikov, A. A. Kalinichev, M. A. Kurochkin, D. V. Mamonova, E. Yu. Kolesnikov and E. Lähderanta, Ratiometric optical thermometry based on emission and excitation spectra of YVO4:Eu3+ nanophosphors, J. Phys. Chem. C, 2019, 123, 5136–5143 CrossRef CAS.
- J. K. Zaręba, M. Nyk, J. Janczak and M. Samoć, Three-photon absorption of coordination polymer transforms UV-to-VIS thermometry into NIR-to-VIS thermometry, ACS Appl. Mater. Interfaces, 2019, 11, 10435–10441 CrossRef PubMed.
- Z. L. Wu, H. M. Wu and Z. Yao, Up-conversion luminescence and temperature characteristics of GdNbO4:Er3+/Yb3+ Phosphors, Chin. J. Lumin., 2017, 38(9), 1129–1135 CrossRef.
- G. F. Liu and Z. L. Fu, Synthesis and temperature sensing of CaF2:Er3+, Yb3+ nanoparticles with up-conversion fluorescence, Chin. J. Lumin., 2017, 38(2), 133–138 CrossRef.
- G. Tripathi, V. K. Rai and S. B. Rai, Up-conversion and temperature sensing behavior of Er3+, doped Bi2O3–Li2O–BaO–PbO tertiary glass, Opt. Mater., 2007, 30(2), 201–206 CrossRef CAS.
- X. Wang, M. Lan, Y. Z. Yang, L. L. Wang, Z. H. Sun and W. W. Wang, Synthesis and enhanced up-conversion optical properties of Cu2+ Ion doped β-NaYF4:Yb3+, Er3+ crystals, Chin. J. Lumin., 2018, 39(8), 1082–1086 CrossRef.
- J. Wang, R. Deng, M. A. MacDonald, B. Chen, J. Yuan, F. Wang, D. Chi, T. S. Hor, P. Zhang, G. Liu, Y. Han and X. Liu, Enhancing multiphoton up-conversion through energy clustering at sublattice level, Nat. Mater., 2014, 13, 157–162 CrossRef CAS PubMed.
- W. Zou, C. Visser, J. A. Maduro, M. S. Pshenichnikov and J. C. Hummelen, Broadband dye-sensitized up-conversion of near-infrared light, Nat. Photonics, 2012, 6, 560–564 CrossRef CAS.
- H. Li and L. Wang, NaYF4:Yb3+/Er3+ nanoparticle-based up-conversion luminescence resonance energy transfer sensor for mercury (II) quantification, Analyst, 2013, 138(5), 1589–1595 RSC.
- J. Zhang, C. Mi and H. Wu, Synthesis of NaYF4:Yb/Er/Gd up-conversion luminescent nanoparticles and luminescence resonance energy transfer-based protein detection, Anal. Biochem., 2012, 421(2), 673–679 CrossRef CAS PubMed.
- Y. Yu, D. Qi and H. Zhao, Enhanced green up-conversion luminescence in Ho3+/Yb3+ codoped Y2O3, ceramics with Gd3+, J. Lumin., 2013, 143(6), 388–392 CrossRef CAS.
- P. Bhoyar and S. J. Dhoble, Electron-vibrational interaction in 5d state of Eu2+ in LiMgBF6, Li2NaBF6, and Li3BF6:Eu2+ phosphors, J. Lumin., 2013, 139, 22–27 CrossRef CAS.
- T. T. Deng, E. H. Song and Y. Y. Zhou, Stable narrowband red phosphor K3GaF6:Mn4+ derived from hydrous K2GaF5 (H2O) and K2MnF6, J. Mater. Chem. C, 2017, 5, 9588–9596 RSC.
- T. T. Deng, E. H. Song, J. Sun, L. Y. Wang, Y. Deng, S. Ye, J. Wang and Q. Y. Zhang, Design and preparation of thermally stable Mn4+ ions activated narrowband red emitting fluoride Na3GaF6:Mn4+ for warm WLED application, J. Mater. Chem. C, 2017, 11, 2910–2918 RSC.
- J. k. Cao, Research on temperature sensing characteristics based on K3LuF6:Er3+ glass-ceramic glass green up-conversion, J. Chin. Rare Earth Soc., 2017, 124 Search PubMed.
- D. Yang and H. k. Liu, Crystal structure and luminescence properties of a novel cryolite-type K3LuF6:Ce3+ phosphor, J. Solid State Chem., 2019, 277, 32–36 CrossRef CAS.
- F. Hong, H. P. Xu, G. X. Liu and C. Song, Mn4+ nonequivalent-doped Al3+-based cryolite high-performance warm WLED red phosphors, New J. Chem., 2019, 43, 14859–14871 RSC.
- D. Yang, L. B. Liao and Q. F. Guo, Luminescence properties and energy transfer of K3LuF6:Tb3+, Eu3+ multicolor phosphors with a cryolite structure, RSC Adv., 2019, 9, 4295–4302 RSC.
- A. G. Marek, G. Anna, T. G. Monika and R. R. Witold, Crystal structure and vibrational properties of new luminescent hosts K3YF6 and K3GdF6, J. Solid State Chem., 2006, 179, 3145–3150 CrossRef.
- F. F. Chi, F. F. Hu, X. T. Wei, Y. H. Chen and M. Yi, Synthesis and thermometric properties of Yb3+/Er3+ co-doped K2GdF5 up-conversion phosphors, J. Rare Earths, 2017, 35(5), 436–440 CrossRef CAS.
- C. Joshi, A. Dwivedi and S. B. Rai, Structural morphology, up-conversion luminescence and optical thermometric sensing behavior of Y2O3:Er3+/Yb3+ nano-crystalline phosphor, Spectrochim. Acta, Part A, 2014,(129), 451–456 CrossRef CAS PubMed.
- J. M. Hu, D. C. Zhou and J. B. Qiu, Up-conversion of Er3+/Yb3+ co-doped transparent glass-ceramics containing Ba2LaF7 nanocrystals, J. Rare Earths, 2013, 31(9), 843–848 CrossRef.
- X. Zhou, H. Wang and B. J. Chen, Efficient up-conversion Yb3+, Er3+co-doped Na5Lu9F32 single crystal for photovoltaic application under solar cell spectrum excitation [J], Chin. Opt. Lett., 2019, 17(09), 72–76 Search PubMed.
- M. A. Gusowski, A. Gagor, M. Trzebiatowska-Gusowska and W. Ryba-Romanowski, Crystal structure and vibrational properties of new luminescent hosts K3YF6 and K3GdF6, J. Solid State Chem., 2006, 179, 3145–3150 CrossRef CAS.
- M. Runowski, S. Goderski, J. Paczesny, M. Księżopolska-Gocalska, A. Ekner-Grzyb, T. Grzyb, J. D. Rybka, M. Giersig and S. Lis, Preparation of biocompatible, luminescent-plasmonic core/shell nanomaterials based on lanthanide and gold nanoparticles exhibiting SERS effects, J. Phys. Chem. C, 2016, 120(41), 23788–23798 CrossRef CAS.
- B. Li, X. Huang and J. Lin, Single-phased white-emitting Ca3Y(GaO)3(BO3)4:Ce3+, Tb3+, Sm3+ phosphors with high-efficiency: Photoluminescence, energy transfer and application in near-UV-pumped white LEDs, J. Lumin., 2018, 204, 410–418 CrossRef CAS.
- S. Q. Liu, Y. J. Liang, Y. L. Zhu, H. R. Li, Y. X. Cai and D. Tu, Full visible spectra emission introduced by crystal-site engineering in β-Ca3(PO4)2-type solid solution phosphors for high quality white light emitting diodes application, Chem. Eng. J., 2019, 375 CAS.
- P. Liu, F. Wang, X. Chen and B. Yang, Up-conversion/Down-conversion luminescence and preparation of NIR-to-UV-excited Gd2O2S:Er phosphor, J. Lumin., 2018, 200, 126–132 CrossRef CAS.
- M. Hirano and K. Ishikawa, Hydrothermal formation and up-conversion luminescence of Er3+ doped GdNbO4, J. Am. Ceram. Soc., 2017, 100, 2814–2821 CrossRef CAS.
- Y. L. Wei, X. M. Li and H. Guo, Enhanced up-conversion in novel KLu2F7:Er3+ transparent oxyfluoride glass-ceramics, Opt. Mater. Express, 2014, 4, 1367–1372 CrossRef CAS.
|
This journal is © The Royal Society of Chemistry 2020 |