DOI:
10.1039/C7RA01507K
(Paper)
RSC Adv., 2017,
7, 19497-19501
Optical and electrical switching of cholesteric liquid crystals containing azo dye
Received
6th February 2017
, Accepted 28th March 2017
First published on 3rd April 2017
Abstract
We propose an optically and electrically switchable cholesteric liquid crystal (ChLC) cell doped with a push–pull azo dye. When the proposed ChLC cell is exposed to UV light, it is switched from the focal-conic to isotropic state by a cholesteric-isotropic phase transition through a trans–cis photo-isomerization of push–pull azo dye molecules. With removal of UV light, the ChLC cell will rapidly relax back to its initial state without exposure to a second light source that has a longer wavelength. We confirmed that the proposed optically and electrically switchable ChLC cell can be used as a smart window that can be switched on by applying sunlight or an electric field.
1. Introduction
Recently, photochemically-induced phase transitions have become technologically attractive and important because of their smart optical properties.1–3 Photochromic compounds, such as azo dyes, spiropyran, and diarylethene, have been intensively studied as candidates for the direct photo-induced phase transition. It is well known that the conformational change between the trans and cis isomers of an azo dye can be applied to control the macroscopic molecular order.4–11 Liquid crystals (LCs) mixed with azo dyes undergo a significant change in the order parameter as a result of trans–cis photo-isomerization,12–15 which lowers the clearing point of the LC mixture over the course of continuous UV exposure. Thus, LC devices can be switched optically by a nematic-isotropic phase transition.
Meanwhile, cholesteric LCs (ChLCs) are typically characterized by a helical structure, resulting in selective reflection of the incident light in the planar state and scattering of the light in the focal conic state. Especially, switching between the focal conic and homeotropic states can be applied as a switchable reflector for reflective displays and light shutters.16–18 Optically controllable ChLC devices can be fabricated simply by doping azo dye to LCs.13 However, although the trans–cis isomerization is rapidly induced, the cis–trans isomerization typically takes tens of hours. To return to the initial state rapidly, a second light source with a longer wavelength is required that can induce the cis–trans photo-isomerization. Slow relaxation and the requirement of two light sources can make it difficult practical display or smart window application.
In this study, we propose an optically and electrically switchable ChLC cell doped with push–pull azo dye, which is known to speed up thermal relaxation. When the proposed ChLC cell is exposed to UV light, it is switched from the focal conic to the isotropic state by cholesteric-isotropic phase transition through trans–cis photo-isomerization of push–pull azo dye molecules. With the UV light is removed, the ChLC cell rapidly relaxes back to its initial state. We have shown that the proposed ChLC cell can be optically switchable between the focal conic and isotropic states by sunlight, and electrically switchable between the focal conic and homeotropic states by applying a vertical electric field. An optically and electrically switchable ChLC cell, which enables transition between the translucent and transparent states depending upon the ambient conditions, will be a promising approach for a smart window.
2. Experimental
2.1. Materials
We mixed LCs to set the switching temperature range, which depends on the clearing point of the LCs. Fig. 1 shows the chemical structure, clearing point, and percentage of LCs in the mixture used in this study. The LC mixture consists of four compounds: 4′-n-pentyl-4-cyanobiphenyl (5CB), 4′-n-heptyl-4-cyanobiphenyl (7CB), 4′-n-octyloxy-4-cyanobiphenyl (8OCB), and 4′-n-pentyl-4-cyano-p-triphenyl (5CT). The clearing point of the mixture was 48.1 °C, which can be decreased by doping chiral material. To overcome the slow cis–trans isomerization, we can use push–pull azo dye HABA (2-(4-hydroxyphenylazo)benzoic) acid which has been used to speed up thermal relaxation (Fig. 2).20,21
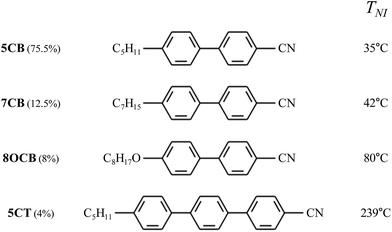 |
| Fig. 1 Structures and wt% of the components present in the nematic mixture (5CB, 7CB, 8OCB, and 5CT) used in our experiment. | |
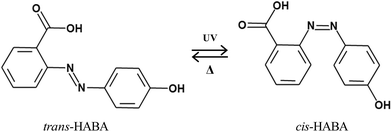 |
| Fig. 2 Trans–cis isomerization of push–pull azo dye HABA. | |
2.2. Cell fabrication
The LC mixture was doped with chiral dopant S-811 and HABA by stirring continuously for 24 h at room temperature. In order to apply a vertical electric field for electrical switching, the top and bottom substrates contain the transparent indium–tin-oxide electrodes. Indium–tin-oxide-coated glass substrates were subjected to several cleaning steps before the spin-coating process. To obtain the ChLC cells with vertical anchoring at surfaces, we used polyimide SE-5662 (Nissan, Japan), which was spin-coated on each substrate. Spin coating was made at 3000 rpm, for 30 s. The coated substrates were pre-baked at 100 °C for 10 min on a hot plate and annealed at 180 °C for 1 h. The mixture was filled to a 20 μm-thick cell via capillary action at room temperature.
2.3. Measurements
The measurement of the clearing point was carried out using a Mettler FP82 hot-stage and a Mettler FP90 controller. The specular transmittance was measured using a spectrometer (MCPD 3000, Photal) covering from 380 to 780 nm. The fabricated ChLC cell was exposed to unpolarized UV light (365 nm) using a mercury arc lamp (Osram HBO 103 W/2). The electro-optical performance of the fabricated ChLC cell was measured using a haze meter (HM-65W, Murakami Color Research Laboratory). The specular [diffuse] transmittance Ts [Td] refers to the ratio of the power of the beam that emerges from a sample cell, which is parallel (within a small range of angles of 2.5°) [not parallel] to a beam entering the cell, and to the power carried by the beam entering the sample. The total transmittance Tt is the sum of the specular transmittance Ts and the diffuse transmittance Td. The haze H can be calculated as the ratio H = Td/Tt.
3. Results and discussion
3.1. Optically and electrical switching of a ChLC cell
To confirm the use of the proposed ChLC cell as a switchable reflector, we fabricated the ChLC cell with the initial focal conic state. The initial focal conic state can be obtained with sufficiently strong homeotropic anchoring.22–24 The minimal helical pitch value for the homeotropic state can be expressed aswhere d is the cell gap, and K22 and K33 are elastic constants for twist and bend deformations, respectively. If the pitch is larger than the threshold pitch Pth, the chiral torque is too weak and the system is unable to twist, resulting in homeotropic alignment of LCs. When the pitch is smaller than Pth, the chiral torque is sufficiently strong, and the initial focal conic state can be observed.
Fig. 3 depicts the specular transmittances, haze values, and photographs of ChLC cells with vertical anchoring at surfaces as a function of the number of pitches. The number of pitches was varied by changing the chiral dopant concentration for a fixed cell gap. As we expected from eqn (1), the homeotropic state can be obtained when the number of pitches is smaller than 2 because the calculated minimal helical pitch value for the homeotropic state is approximately 1 μm. As the number of pitches is increased, the specular transmittance decreases and the haze gradually increases. The measured haze value was saturated and we can hide the printed texts when the number of pitches reached 16, which was chosen as the initial focal conic state of the proposed ChLC cell.
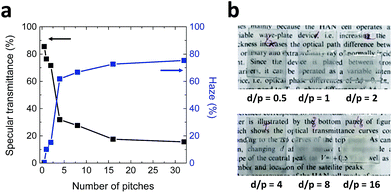 |
| Fig. 3 (a) Specular transmittances and haze values and (b) photographs of ChLC cells with vertical anchoring at surfaces as functions of the number of pitches. | |
We measured the clearing point of the LC mixture without chiral dopant as a function of the HABA concentration, as shown in Fig. 4(a). Before UV irradiation, the clearing point does not change with the increase of HABA concentration. Once the mixture is irradiated by UV light and the trans–cis photo-isomerization takes place, the bent shape of the cis-azo dye introduces molecular disorder in the mixture.21 Thus, the clearing point is lowered. When exposed to UV light, the clearing point of the mixture (HABA 5 wt%) changed from 47 °C to 38 °C. It should be noted that the switching temperature of the proposed ChLC cell depends on the LC material.
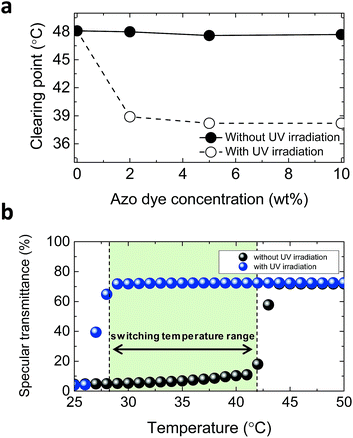 |
| Fig. 4 (a) Clearing point of the LC mixture as a function of HABA concentration. (b) The specular transmittance of the fabricated ChLC cell with and without UV irradiation as a function of temperature. | |
It is well known that LCs with a high molecular weight of chiral dopant can decrease the clearing point of the mixture.19 To investigate the switching temperature of the proposed ChLC cell, we fabricated a ChLC cell and measured the specular transmittance with and without UV irradiation as a function of temperature. The fabricated ChLC cell was exposed to unpolarized UV light (365 nm) with the intensity of 5 mW cm−2. Concentrations of azo dye and chiral dopant were 5 and 7.53 wt%, respectively. Fig. 4(b) shows a plot of the variation in transmittance of the proposed ChLC cell with temperature. Without UV irradiation, the transmittance abruptly increased at approximately 44 °C during the heating process. This temperature is close to the clearing point. The clearing point was decreased from 47 °C to 44 °C by doping a chiral dopant. With UV irradiation, the transmittance abruptly increased at approximately 29 °C during the heating process. In other words, the phase transition of the LC mixture between the cholesteric and isotropic states can be controlled by UV light for temperatures ranging from 29 °C to 44 °C. We should note that the switching temperature range was also increased from 9 °C to 15 °C by doping chiral dopant. Although the switching temperature range of the proposed ChLC cell is currently rather narrow, we expect that the temperature range can be widened further by developing new azo dye materials.
To confirm optical switching, we fabricated the proposed ChLC cell by exposing to UV light with the intensity of 5 mW cm−2. Fig. 5(a) shows changes in the specular transmittance of a ChLC cell fabricated by UV irradiation at 30 °C. Before UV irradiation, the transmittance was kept at about 4%. The transmittance was increased by UV irradiation and thermally relaxed with removal of UV light. The transition took place in around 30 s. The transition from the isotropic to the focal conic state can be achieved without any external power or light because the proposed ChLC cell contains the push–pull azo dye which has the fast thermal relaxation property.
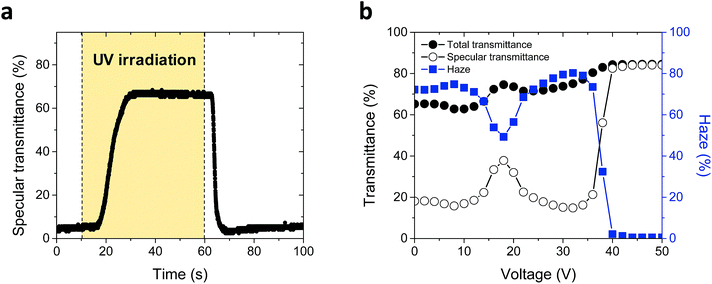 |
| Fig. 5 (a) Changes in specular transmittance of fabricated ChLC cell by UV irradiation at 30 °C. (b) Total transmittance, specular transmittance, and haze of fabricated ChLC cell as functions of the applied voltage. | |
To confirm electrical switching, the total transmittance, specular transmittance, and haze of the fabricated ChLC cell as functions of the applied voltage are shown in Fig. 5(b). In the initial focal conic state (opaque state), the measured total transmittance, specular transmittance, and haze of the fabricated ChLC cell were 65.2%, 18.1%, and 72.2%, respectively. Haze decreased gradually as the applied voltage increased to 18 V, but the haze increased at higher applied voltages. This increase in haze is caused by ion impurities that generate dynamic scattering.25–28 When the applied voltage was higher than 40 V, LCs were aligned homeotropically along the direction of the applied field. In the homeotropic state, light scattering was minimized. The measured total transmittance, specular transmittance, and haze in the homeotropic state were 83.6%, 81.5%, and 2.5%, respectively.
3.2. ChLC cell for smart window applications
Although the proposed ChLC cell exhibits fast thermal relaxation, optical switching of the proposed ChLC cell is still much slower than electrical switching. Therefore, its main application can be a smart window rather than optical components requiring a fast response time. Its optical switching time is similar to other smart window devices, such as electrochromic, thermochromic, and photochromic devices.29–31 A simple illustration of the proposed ChLC cell as a smart window is shown in Fig. 6. In the initial translucent state, the incident light is scattered by LCs in the focal conic state as a result of the randomly oriented ChLCs. The optical switching mechanism is based on the change of the LC order parameter by the photo-isomerization effect of push–pull azo dye. Under sunlight irradiation, the cell was switched from the focal conic to isotropic state by cholesteric-isotropic phase transition of the LCs triggered by trans–cis photo-isomerization of push–pull azo dye molecules. When the intensity of sunlight was low because of cloud cover or when there was little sunlight at sunset, the cell rapidly relaxed from the isotropic state back to its initial focal conic state. It can be switched from the translucent to transparent state by applying an electric field, too. Under an applied vertical electric field, the focal conic state is switched to the homeotropic state and the cell becomes transparent.
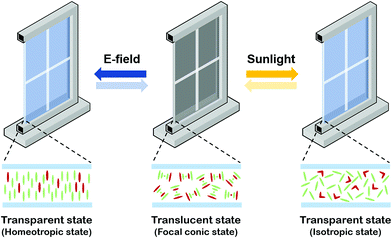 |
| Fig. 6 Schematic representation of the proposed smart window using a push–pull azo dye. | |
Photographs of the proposed smart window placed on printed texts in the focal conic, homeotropic, isotropic states are shown in Fig. 7(a). In the focal conic state, the proposed smart window can scatter the incident light by the refractive index difference between LC domains. In the homeotropic and isotropic states, we can identify characters behind a panel clearly. The measured specular transmittances were 9.40%, 67.1%, and 66.9% in the focal conic, homeotropic, isotropic states, respectively. The optically-induced isotropic state showed nearly the same specular transmittance as the electrically-induced homeotropic state.
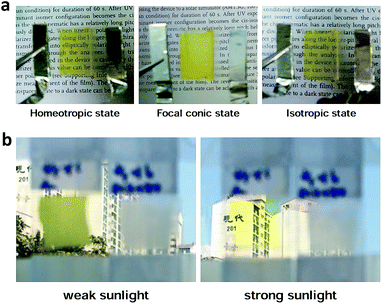 |
| Fig. 7 (a) Photographs of the proposed smart window in three states. (b) Photographs of the proposed smart window (left) and typical ChLC cell (right) under the outdoor environment. | |
Fig. 7(b) shows the results of an outdoor switching test performed under sunlight for an optically and electrically switchable smart window and a ChLC cell. The measured temperature of the fabricated cells was approximately 30 °C when irradiated with sunlight. In the initial state, both cells were translucent and blocked the view. When exposed to sunlight, the cell doped with HABA became transparent and the background scene was clearly visible, whereas the typical ChLC cell was still translucent. As soon as we blocked the sunlight, the cell doped with HABA returned to its initial translucent state within 30 s. We should note that the transition between these states was achieved without any external power or signal.
4. Conclusions
An optically and electrically switchable ChLC cell doped with push–pull azo dye was demonstrated. The proposed ChLC cell is switchable between the focal conic and homeotropic states by applying an electric field or between focal conic and isotropic states by UV irradiation. Its main application can be a smart window rather than optical components requiring a fast response time because optical switching of the proposed ChLC cell is much slower than electrical switching. Under sunlight irradiation, the proposed ChLC cell was switched from the translucent to the transparent state. When the intensity of sunlight was low because of cloud cover, or when there was little sunlight at sunset, the proposed ChLC cell rapidly relaxed without exposure to a second light source that had a longer wavelength. With such a proposed ChLC cell as a smart window, we could clearly see the outside through the window during the daytime, while blocking the view of the indoor area for privacy during the night without any external power or signal. Moreover, when the temperature is out of the sunlight-switchable temperature range, it can be switched between the translucent and transparent states by applying an electric field.
Acknowledgements
This work was supported by the IT R&D program of MOTIE/KEIT [10042412, More than 60 Transparent Flexible Display with UD Resolution, Transparency 40% for Transparent Flexible Display in Large Area] and the National Research Foundation of Korea (NRF) grant funded by the Korean government (MSIP) (No. 2017R1A2A1A05001067).
Notes and references
- S. Kobatake, H. Imagawa, H. Nakatani and S. Nakashima, New J. Chem., 2009, 33, 1362 RSC.
- R. Klajn, P. J. Wesson, K. J. M. Bishop and B. A. Grzybowski, Angew. Chem., Int. Ed., 2009, 48, 7035 CrossRef CAS PubMed.
- L. H. Urner, B. N. S. Thota, O. Nachtigall, S. Warnke, G. Helden, R. Haag and K. Pagel, Chem. Commun., 2015, 51, 8801 RSC.
- D. Y. Kim, S. K. Tripathy, L. Li and J. Kumar, Appl. Phys. Lett., 1995, 66, 1166 CrossRef CAS.
- T. Ikeda and O. Tsutsumi, Science, 1995, 268, 1873 CAS.
- M. Yamada, M. Kondo, J. Mamiya, Y. Yu, M. Kinoshita, C. J. Barrett and T. Ikeda, Angew. Chem., Int. Ed., 2008, 47, 4986 CrossRef CAS PubMed.
- M. Yamada, M. Kondo, R. Miyasato, Y. Naka, J. Mamiya, M. Kinoshita, A. Shishido, Y. Yu, C. J. Barrett and T. Ikeda, J. Mater. Chem., 2009, 19, 60 RSC.
- M. Irie, Photochem. Photobiol. Sci., 2010, 9, 1535 CAS.
- J.-D. Lin, M.-H. Hsieh, G.-J. Wei, T.-S. Mo, S.-Y. Huang and C.-R. Lee, Opt. Express, 2013, 21, 15765 CAS.
- K. Masutani, M. Morikawa and N. Kimizuka, Chem. Commun., 2014, 50, 15803 RSC.
- J.-D. Lin, C.-P. Chen, L.-J. Chen, Y.-C. Chuang, S.-Y. Huang and C.-R. Lee, Opt. Express, 2016, 24, 3112 Search PubMed.
- J. P. Otruba III and R. G. Weiss, J. Org. Chem., 1983, 48, 3448 CrossRef.
- Y. Kawanishi, T. Tamaki and K. Ichimura, J. Phys. D: Appl. Phys., 1991, 24, 782 CrossRef CAS.
- J. Bin and W. S. Oates, Sci. Rep., 2015, 5, 14654 CrossRef PubMed.
- S.-W. Oh, J.-M. Baek and T.-H. Yoon, Opt. Express, 2016, 24, 26575 CrossRef PubMed.
- K.-H. Kim, H.-J. Jin, D. H. Song, B.-H. Cheong, H.-Y. Choi, S. T. Shin, J. C. Kim and T.-H. Yoon, Opt. Lett., 2010, 35, 3504 CrossRef CAS PubMed.
- K.-H. Kim, H.-J. Jin, K.-H. Park, J.-H. Lee, J. C. Kim and T.-H. Yoon, Opt. Express, 2010, 18, 16745 CrossRef CAS PubMed.
- K.-H. Kim, D. H. Song, Z.-G. Shen, B. W. Park, K.-H. Park, J.-H. Lee and T.-H. Yoon, Opt. Express, 2011, 19, 10174 CAS.
- U. A. Hrozhyk, S. V. Serak, N. V. Tabiryan and T. J. Bunning, Adv. Mater., 2007, 19, 3244 CrossRef CAS.
- P. El-Kallassi, R. Ferrini, L. Zuppiroli, N. L. Thomas, R. Houdré, A. Berrier, S. Anand and A. Talneau, J. Opt. Soc. Am. B, 2007, 24, 2165 CrossRef CAS.
- H. Yu and T. Kobayashi, Molecules, 2010, 15, 570 CrossRef CAS PubMed.
- P. E. Cladis and M. Kléman, Mol. Cryst. Liq. Cryst., 1972, 16, 1 CrossRef CAS.
- P. Oswald, J. Baudry and S. Pirkl, Phys. Rep., 2000, 337, 67 CrossRef CAS.
- I. Gvozdovskyy, O. Yaroshchuk, M. Serbina and R. Yamaguchi, Opt. Express, 2012, 20, 3499 CAS.
- D.-K. Yang and S.-T. Wu, Fundamentals of Liquid Crystal Devices, Wiley, 2006 Search PubMed.
- G. H. Heilmeier, L. A. Zanoni and L. A. Barton, Proc. IEEE, 1968, 56, 1162 CrossRef.
- G. H. Heilmeier, L. A. Zanoni and L. A. Barton, Appl. Phys. Lett., 1968, 13, 46 CrossRef CAS.
- G. H. Heilmeier, L. A. Zanoni and L. A. Barton, IEEE Trans. Electron Devices, 1970, 17, 22 CrossRef CAS.
- A. Seeboth and D. Lötzsch, Thermochromic and Thermotropic Materials, 2013 Search PubMed.
- R. J. Mortimer, D. R. Rosseinsky and P. M. Monk, Electrochromic Materials and Devices, 2015 Search PubMed.
- H. Tian and J. Zhang, Photochromic Materials: Preparation, Properties and Applications, 2016 Search PubMed.
|
This journal is © The Royal Society of Chemistry 2017 |
Click here to see how this site uses Cookies. View our privacy policy here.