Open Access Article
DOI:
10.1039/C1SC00468A
(Edge Article)
Chem. Sci., 2011,
2, 2314-2320
[6]Saddlequat: a [6]helquat captured on its racemization pathway†
Received
14th July 2011
, Accepted 8th August 2011
First published on 8th September 2011
Abstract
A dicationic [6]helicene congener captured on the racemization pathway in its saddle-shaped geometry is introduced. Synthesis, structure, resolution, and dynamic properties of this chiral [6]saddlequat in-between and its highly stereocontrolled transformation into enantiopure [6]helquat are discussed and demonstrated. The dynamic aspects established by experiment and supported by detailed DFT-D calculations are presented visually in the form of a movie (electronic table-of-contents and electronic supplementary information). The title [6]saddlequat was found to be an isolable chiral species on the entirely chiral enantiomerization pathway of a [6]helquat that is discussed as an example of Mislow's “rubber glove” molecule.
Introduction
Helicenes and helicene congeners continue to attract attention due to their fascinating helical shape, inherent chirality, and electronics.1–4 Because of their unique structure, their self-assembly,5,6catalytic,7 chiroptical,8 and acid–base9 properties have been intensively studied. Furthermore, the recently reported ability of helicene congeners to inhibit the activity of telomerase,10 topoisomerase I,11 and interact with DNA duplexes12 and G quadruplexes13 promises interesting future applications.
A distinctive feature of helicenes is the existence of a thermal racemization pathway equilibrating the right-handed helix with the left-handed one (P ⇌ M, Scheme 1 and Scheme 2). This unique phenomenon of thermally triggered racemization via helix inversion has been extensively investigated.14–17 In a typical helicene, e.g.[6]helicene, helix inversion proceeds via a Cs-symmetric saddle-shaped transition state that is achiral (Scheme 1a).16 In some rare cases, helicene congeners having saddle-shaped energy minima on their racemization pathways have been reported. Two examples, where the presence of such saddle-shaped energy minima is predicted, are [9]helicene (Scheme 1b)16b and a recently disclosed [11]helicene derivative.17,18 We supposed, if the saddle-shaped energy minima existed in a sufficiently deep energy valley, then, the species corresponding to these minima could, in principle, be isolated and characterized as stable intermediates. They would represent a helical system captured during its racemization pathway.
![(a) Racemization of [6]helicenevia achiral saddle-shaped transition state, (b) racemization of [9]helicenevia saddle-shaped intermediate, and the corresponding energy profiles. r.c. = reaction coordinate.](/image/article/2011/SC/c1sc00468a/c1sc00468a-s1.gif) |
| Scheme 1 (a) Racemization of [6]helicenevia achiral saddle-shaped transition state, (b) racemization of [9]helicenevia saddle-shaped intermediate, and the corresponding energy profiles. r.c. = reaction coordinate. | |
![Racemization of [6]helicene congener via isolable saddle-shaped intermediate studied in this work.](/image/article/2011/SC/c1sc00468a/c1sc00468a-s2.gif) |
| Scheme 2 Racemization of [6]helicene congener via isolable saddle-shaped intermediate studied in this work. | |
Here we describe the synthesis, structure, and dynamic properties of a [6]helquat, a dicationic [6]helicene congener captured on the racemization pathway in its saddle-shaped geometry (Scheme 2). Resolution of this chiral saddle-shaped species and its highly stereocontrolled transformation into enantiopure [6]helquat is demonstrated.
Results and discussion
In our recent studies, we introduced helquats,19–22 helical dications that represent a missing structural link between helicenes and viologens. By taking advantage of a highly flexible three-step synthetic route,21 we accessed isomeric triynes1 and 3 (Scheme 3 and ESI†). We found that, as usual, triyne1 is transformed solely to the corresponding [6]helquat 2viarhodium-catalyzed [2 + 2 + 2] cycloaddition.23,24 However, the isomeric triyne3 leads under the same reaction conditions to formation of an isolable saddle-shaped species 4 along with the formation of [6]helquat 5, featuring the typical helical shape. Both structures 4 and 5 were confirmed by a variety of spectroscopic methods.13C, 1H, HSQC, HMBC, COSY and ROESY NMRspectra permitted full assignment of all 1H and 13C resonances and established connectivity and spatial arrangement. Importantly, the three-dimensional identity of the individual dicationic frameworks has been unambiguously proved by single-crystal X-ray diffraction analyses (vide infra).23
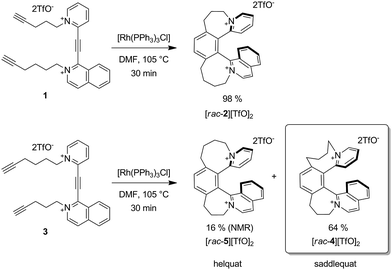 |
| Scheme 3
Triyne
1 leads solely to helical product 2 while isomeric triyne3 gives the saddle-shaped species 4 as the major product. | |
The saddle-shaped product 4, which we suggest to denote as [6]saddlequat 4, arises from a kinetically controlled [Rh(PPh3)3Cl]-catalyzed [2 + 2 + 2] cycloisomerization that furnishes a mixture of [6]saddlequat 4 and the corresponding [6]helquat 5 in a 4
:
1 ratio.25 The solubilities of the diastereoisomeric salts [4][TfO]2 and [5][TfO]2 in tetrahydrofuran are considerably different and allow for the straightforward non-chromatographic separation of the two stereoisomers. Notably, and of particular importance for this study is that the saddle-shaped species [4] is sufficiently long-lived to be comfortably studied experimentally.
Moreover, both [5] and [4] are chiral entities formed as racemates during the [2 + 2 + 2] cycloaddition depicted in Scheme 3. This is evidenced by capillary electrophoresis (CE) with sulfated γ-cyclodextrin chiral selector, which shows two peaks of equal intensity corresponding to the two enantiomers of compound 4 (Scheme 4). A similar situation is observed in the case of compound 5.26,27
![Saddlequat [4] and helquat [5] are both chiral entities. Both, [4] and [5], are produced from the triyne3 in racemic form as evidenced by capillary electrophoresis with sulfated γ-cyclodextrin chiral selector.27 See ESI for details.](/image/article/2011/SC/c1sc00468a/c1sc00468a-s4.gif) |
| Scheme 4 Saddlequat [4] and helquat [5] are both chiral entities. Both, [4] and [5], are produced from the triyne3 in racemic form as evidenced by capillary electrophoresis with sulfated γ-cyclodextrin chiral selector.27 See ESI for details.† | |
The calculated Gibbs free energy of the saddlequat [4] is 18 kJ mol−1 higher relative to helquat [5] in DMSO as determined by DFT-D calculations28 (TURBOMOLE:29 B3LYP/def2-TZVP//ri-PBE/def2-SV(P) + disp30 + DMSO,31 see ESI for details†32). In line with this predicted energy difference, upon heating in DMSO-d6 at 100 °C saddlequat [4][TfO]2 gradually and completely converted to helquat [5][TfO]2 (Scheme 5). This process can be followed by NMRvia disappearance of peaks corresponding to [4] and appearance of peaks corresponding to [5] (Scheme 5). Data analysis from this experiment led to the activation free energy value ΔG≠ of 120 kJ mol−1 for the transformation [4] → [5].
![Transformation [rac-4] → [rac-5] at 100 °C in DMSO-d6 followed by 1H NMR.](/image/article/2011/SC/c1sc00468a/c1sc00468a-s5.gif) |
| Scheme 5 Transformation [rac-4] → [rac-5] at 100 °C in DMSO-d6 followed by 1H NMR. | |
In order to gain a thorough understanding of the conversion of [4] to [5] we turned to detailed DFT calculations. To this end, TURBOMOLE calculations at the DFT-D level28–31 shed light on the energy profile of and structures involved in the entire racemization pathway of helquat [5] (Scheme 6). In line with our experimental results, calculation predicts that the saddlequat [4] exists in this calculated energy profile as a local energy minimum that is in a sufficiently deep energy valley that it can be isolated and studied.
![Calculated racemization pathway of helquat [5] passing through saddlequat [4]. Pathways a and b denote the two distinct routes from saddlequat [4] to the two enantiomeric helquats [P-5] and [M-5], respectively. See also the movie in the electronic table-of-contents and ESI for details.](/image/article/2011/SC/c1sc00468a/c1sc00468a-s6.gif) |
| Scheme 6 Calculated racemization pathway of helquat [5] passing through saddlequat [4]. Pathways a and b denote the two distinct routes from saddlequat [4] to the two enantiomeric helquats [P-5] and [M-5], respectively. See also the movie in the electronic table-of-contents and ESI for details.† | |
Inspection of the calculated energy profile around saddlequat [4] indicates that there are two possible, energetically distinct, pathways leading to two helquat [5] structures of opposite helicity (pathways a and b in Scheme 6). The lower, pathway a, corresponds to a ΔG≠ value of 119 kJ mol−1, which fits very well with the experimentally determined value of 120 kJ mol−1 for transformation [4] → [5] (see NMR study, Scheme 5). Following the energetically less favorable pathway b would also transform the same saddlequat to helquat, but now to the helquat of the opposite helicity.
We thus speculated that the existence of these two energetically distinct pathways would allow for the stereocontrolled transformation of enantiopure saddlequat [4] into enantiopure helquat [5] (via pathway a in Scheme 6). To test this hypothesis, access to non-racemic [4] was crucial. Therefore, we turned to resolution of racemic [4]. By employing the same resolution protocol recently reported for a [5]helquat derivative,20,33 we were able to achieve straightforward resolution of [rac-4][TfO]2 (Scheme 7). Specifically, the exchange of triflate anions in [rac-4][TfO]2 for enantiopure (R,R)-dibenzoyltartrate anions led to the formation of two diastereomeric salts, one of which crystallized from methanol in diastereomerically pure form, as evidenced by a single peak in the chiral CE trace.26,27 Single crystals suitable for X-ray diffraction analysis were obtained and enabled absolute configuration assignment of the dicationic scaffold of [Sa,Ra-4] (Fig. 1).23 Subsequent exchange of the (R,R)-dibenzoyltartrate anions in this diastereomerically pure salt for triflate anions via ion exchange resin completed the resolution giving (+)-[Sa,Ra-4] ditriflate in its enantiomerically pure form (CE in Scheme 7).
![Resolution procedure for [rac-4][TfO]2 and the corresponding CE traces showing two peaks for [rac-4] ditriflate and a single peak for the isolated (+)-[Sa,Ra-4] ditriflate after resolution. See ESI for further details.](/image/article/2011/SC/c1sc00468a/c1sc00468a-s7.gif) |
| Scheme 7 Resolution procedure for [rac-4][TfO]2 and the corresponding CE traces showing two peaks for [rac-4] ditriflate and a single peak for the isolated (+)-[Sa,Ra-4] ditriflate after resolution. See ESI for further details.† | |
![X-Ray single crystal structure assigning absolute configuration of the dicationic framework of (+)-[Sa,Ra-4][(R,R)-dibenzoyltartrate]2. (R,R)-Dibenzoyltartrate anions and crystal water molecules are omitted for clarity.23](/image/article/2011/SC/c1sc00468a/c1sc00468a-f1.gif) |
| Fig. 1 X-Ray single crystal structure assigning absolute configuration of the dicationic framework of (+)-[Sa,Ra-4][(R,R)-dibenzoyltartrate]2. (R,R)-Dibenzoyltartrate anions and crystal water molecules are omitted for clarity.23 | |
By heating this enantiopure sample of saddlequat (+)-[Sa,Ra-4] ditriflate at 100 °C in DMSO-d6, we were able to confirm our hypothesis that the saddle-shaped species can indeed be transformed into enantiopure helquat [5] with no loss of chirality (Scheme 8). The experimental proof for this phenomenon was made possible by a powerful CE method using sulfated γ-cyclodextrin as a chiral selector.26,27 This provided us with an ideal experimental tool for monitoring the enantiocomposition of both [4] and [5] during the stereocontrolled transformation [4] → [5]. CE electropherograms show a single peak corresponding to the starting (+)-[Sa,Ra-4] ditriflate giving rise to a single peak corresponding to (−)-[5], providing definitive evidence for the complete stereocontrol governing this process (Scheme 8). The course of this stereocontrolled transformation (+)-[Sa,Ra-4][TfO]2 → (−)-[5][TfO]2 was also followed by ECD spectroscopy. During the transformation, the characteristic peaks of (+)-[Sa,Ra-4] ditriflate gradually give way to peaks of (−)-[5] ditriflate positioned at longer wavelengths (Scheme 9).
![Chiral CE monitoring confirms complete stereocontrol in the transformation of saddlequat (+)-[Sa,Ra-4][TfO]2 to helquat (−)-[5][TfO]2 at 100 °C, as evidenced by the exclusive production of a single enantiomer of [5]. Bottom CE trace: reference mixture of [rac-4] and [rac-5]. See ESI for details.](/image/article/2011/SC/c1sc00468a/c1sc00468a-s8.gif) |
| Scheme 8 Chiral CE monitoring confirms complete stereocontrol in the transformation of saddlequat (+)-[Sa,Ra-4][TfO]2 to helquat (−)-[5][TfO]2 at 100 °C, as evidenced by the exclusive production of a single enantiomer of [5]. Bottom CE trace: reference mixture of [rac-4] and [rac-5]. See ESI for details.† | |
![ECD spectra recorded in the course of the stereocontrolled transformation (+)-[Sa,Ra-4][TfO]2 → (−)-[5][TfO]2 in DMSO at 100 °C. See ESI for further details.](/image/article/2011/SC/c1sc00468a/c1sc00468a-s9.gif) |
| Scheme 9
ECD spectra recorded in the course of the stereocontrolled transformation (+)-[Sa,Ra-4][TfO]2 → (−)-[5][TfO]2 in DMSO at 100 °C. See ESI for further details.† | |
To confirm that the saddlequat (+)-[Sa,Ra-4][TfO]2 was transformed as predicted by calculation we sought the absolute configuration of the helquat (−)-[5][TfO]2 formed during the process. Calculation predicted that in following the energetically more favorable pathway a, saddlequat (+)-[Sa,Ra-4] would give rise to helquat [5] of P helicity (Scheme 6). Single-crystal X-ray diffraction analyses confirmed that this was indeed the case (Fig. 2).23
![X-Ray crystal structure assigning absolute configuration of helquat (−)-[P-5][TfO]2 as obtained by stereocontrolled transformation from saddlequat (+)-[Sa,Ra-4][TfO]2 at 100 °C. X-Ray quality crystals were grown from the perchlorate salt of [P-5]. Perchlorate anions and crystal water molecules are omitted for clarity.23](/image/article/2011/SC/c1sc00468a/c1sc00468a-f2.gif) |
| Fig. 2 X-Ray crystal structure assigning absolute configuration of helquat (−)-[P-5][TfO]2 as obtained by stereocontrolled transformation from saddlequat (+)-[Sa,Ra-4][TfO]2 at 100 °C. X-Ray quality crystals were grown from the perchlorate salt of [P-5]. Perchlorate anions and crystal water molecules are omitted for clarity.23 | |
To evaluate the racemization barrier of helquat [5] it was essential to have a non-racemic sample of the compound. In our hands, stereocontrolled transformation of enantiopure (+)-[Sa,Ra-4] proved to be the only feasible method to access enantiopure samples of helquat [5] as independent attempts at resolution of racemic [5] via diastereomeric salts failed.
With a route to enantiopure helix 5 established, a solution of (−)-[P-5][TfO]2 in DMSO was heated at 180 °C. CE enantiocomposition analysis of samples taken over the course of heating determined the activation free energy value to be ΔG≠ = 153.3 kJ mol−1 and the racemization half-life (T1/2) to be 4.8 h at 180 °C. This experimentally determined racemization barrier fits well with that predicted by DFT-D calculations, ΔG≠ of 157.7 kJ mol−1 (Scheme 10).
![Thermal racemization of helquat [5][TfO]2 proceeds at 180 °C in DMSO with T1/2 of 4.8 h (experimental ΔG≠ = 153.3 kJmol−1, calculated ΔG≠ = 157.7 kJ mol−1). See ESI for details.](/image/article/2011/SC/c1sc00468a/c1sc00468a-s10.gif) |
| Scheme 10 Thermal racemization of helquat [5][TfO]2 proceeds at 180 °C in DMSO with T1/2 of 4.8 h (experimental ΔG≠ = 153.3 kJmol−1, calculated ΔG≠ = 157.7 kJ mol−1). See ESI for details.† | |
The consistent agreement between the experimental data and DFT-D calculations forms a body of evidence that can be best summarized in the dynamic visual form of the movie we present in the electronic table-of-contents.
We note, that [6]helquat 5 represents an interesting example of a “rubber glove” molecule34,35 as its enantiomerization takes place exclusively by a chiral pathway via chiral [6]saddlequat 4. As introduced by Mislow in the 1950s,34 enantiomerization in “rubber glove” molecules can only proceed via chiral pathways as no achiral conformations can be attained for such structures. This molecular phenomenon is paralleled in the macroscopic world by turning a right-handed rubber glove inside-out leading to an object superimposable with a left handed-glove, never attaining an achiral (symmetric) conformation on its enantiomerization pathway.
Conclusions
In conclusion, we have presented a detailed experimental and theoretical investigation of a stereochemically rich36helicene-type racemization pathway featuring a saddle-shaped local energy minimum sufficiently stable to be isolated, characterized, and studied in detail. This saddle-shaped species arises from a kinetically controlled [Rh(PPh3)3Cl]-catalyzed [2 + 2 + 2] cycloisomerization of the triyne precursor.37 Resolution of this chiral saddle species allows for production of enantiopure helix via a highly stereocontrolled chiral information transfer.38Capillary electrophoresis with sulfated γ-cyclodextrin chiral selector is shown as an advantageous and direct method for analysis of enantiocomposition of both saddle- as well as helical-shaped charged species. Finally, this study describes [6]helquat 5 as an example of a “rubber glove” molecule and introduces [6]saddlequat 4 as an isolable chiral species lying on the entirely chiral enantiomerization pathway of [6]helquat 5.
Acknowledgements
Financial support from the Czech Science Foundation (203/09/1614, P207/10/2391, 203/08/1428, 203/09/P485) and IOCB (Z40550506) is gratefully acknowledged. IC thanks MSM0021620857 and PN thanks Specific University Research (MSMT No. 21/2011) for support. We are grateful to Dr L. Rulíšek, Mr M. Ončák, and Dr P. Slavíček for their valuable help in calculations.
Notes and references
- For selected reviews on helicenes and heterohelicenes, see:
(a)
A. Rajca and M. Miyasaka, in Functional Organic Materials, ed. T. J. J. Müller and U. H. F. Bunz, Wiley-VCH, Weinheim, 2007, pp. 543–577 Search PubMed;
(b) A. Urbano, Angew. Chem., Int. Ed., 2003, 42, 3986 CrossRef CAS;
(c)
H. Hopf, Classics in Hydrocarbon Chemistry: Syntheses, Concepts, Perspectives, Wiley-VCH, Weinheim, 2000, pp. 323–330 Search PubMed;
(d) T. J. Katz, Angew. Chem., Int. Ed., 2000, 39, 1921 CrossRef CAS;
(e) C. Schmuck, Angew. Chem., Int. Ed., 2003, 42, 2448 CrossRef CAS;
(f) S. K. Collins and M. P. Vachon, Org. Biomol. Chem., 2006, 4, 2518 RSC;
(g) A. Rajca, S. Rajca, M. Pink and M. Miyasaka, Synlett, 2007, 1799 CrossRef CAS;
(h)
I. Starý and I. G. Stará, in Strained Hydrocarbons, ed. H. Dodziuk, Wiley-VCH, Weinheim, 2009, pp. 166–204 Search PubMed;
(i) for discussion on early helicenes, see: C. A. Johnson II and M. M. Haley, in Carbon-Rich Compounds: From Molecules to Materials, ed. M. M. Haley and R. R. Tykwinski, Wiley-VCH, Weinheim, Germany, 2006, pp. 1–25 Search PubMed.
- For early work, see:
(a) M. S. Newman, W. B. Lutz and D. Lednicer, J. Am. Chem. Soc., 1955, 77, 3420 CrossRef CAS;
(b) M. S. Newman and D. Lednicer, J. Am. Chem. Soc., 1956, 78, 4765 CrossRef CAS;
(c) M. Flammang-Barbieux, J. Nasielski and R. H. Martin, Tetrahedron Lett., 1967, 8, 743 Search PubMed;
(d) H. Wynberg and M. B. Groen, J. Am. Chem. Soc., 1968, 90, 5339 CrossRef CAS; and reviews:
(e) R. H. Martin, Angew. Chem., Int. Ed. Engl., 1974, 13, 649 CrossRef;
(f) W. H. Laarhoven and W. J. C. Prinsen, Top. Curr. Chem., 1984, 125, 63 CAS;
(g) H. Wynberg, Acc. Chem. Res., 1971, 4, 65 CrossRef CAS;
(h) K. P. Meurer and E. Vögtle, Top. Curr. Chem., 1985, 127, 1 CAS;
(i)
F. Vögtle, Fascinating Molecules in Organic Chemistry, Wiley, New York, 1992, p. 156 Search PubMed;
(j) G. Oremek, U. Seiffert and A. Janecka, Chem.-Ztg., 1987, 111, 69 Search PubMed;
(k) H. Osuga and H. Suzuki, J. Synth. Org. Chem. Jpn., 1994, 52, 1020 Search PubMed.
- For aza- and azoniahelicenes, see:
(a) J. Guin, C. Besnard, P. Pattison and J. Lacour, Chem. Sci., 2011, 2, 425 RSC;
(b) C. Herse, D. Bas, F. C. Krebs, T. Bürgi, J. Weber, T. Wesolowski, B. W. Laursen and J. Lacour, Angew. Chem., Int. Ed., 2003, 42, 3162 CrossRef;
(c) B. Laleu, P. Mobian, C. Herse, B. W. Laursen, G. Hopfgartner, G. Bernardinelli and J. Lacour, Angew. Chem., Int. Ed., 2005, 44, 1879 CrossRef CAS;
(d) M. Ostermeier, C. Limberg, B. Ziemer and V. Karunakaran, Angew. Chem., Int. Ed., 2007, 46, 5329 CrossRef CAS;
(e) S. Arai, T. Yafune, M. Ohkubo and M. Hida, Tetrahedron Lett., 1989, 30, 7217 CrossRef CAS; for reviews, see:
(f)
K. Sato, S. Arai, in Cyclophane Chemistry for the 21st Century, ed. H. Takemura, Research Signpost, Trivandrum, 2002, pp. 186–197 Search PubMed;
(g) F. Dumitrascu, D. G. Dumitrescu and I. Aron, Arkivoc, 2010, (i), 1 CAS.
- For recent reports, see: D. Conreaux, N. Mehanna, C. Herse and J. Lacour, J. Org. Chem., 2011, 76, 2716 Search PubMed; J. D. Chen, H. Y. Lu and C. F. Chen, Chem.–Eur. J., 2010, 16, 11843 CrossRef CAS; L. Norel, M. Rudolph, N. Vanthuyne, J. A. G. Williams, C. Lescop, C. Roussel, J. Autschbach, J. Crassous and R. Réau, Angew. Chem., Int. Ed., 2010, 49, 99 CrossRef CAS; S. Graule, M. Rudolph, W. Shen, J. A. G. Williams, C. Lescop, J. Autschbach, J. Crassous and R. Réau, Chem.–Eur. J., 2010, 16, 5976 CAS; Z. Wang, J. Shi, J. Wang, C. Li, X. Tian, Y. Cheng and H. Wang, Org. Lett., 2010, 12, 456 CAS; O. Songis, J. Míšek, M. B. Schmidt, A. Kollárovič, I. G. Stará, D. Šaman, I. Císařová and I. Starý, J. Org. Chem., 2010, 75, 6889 Search PubMed; J. Štorch, J. Čermák, J. Karban, I. Císařová and J. Sýkora, J. Org. Chem., 2010, 75, 3137 Search PubMed; I. Alkorta, F. Blanco, J. Elguero and D. Schröder, Tetrahedron: Asymmetry, 2010, 21, 962 Search PubMed; Latorre, A. Urbano and M. C. Carreño, Chem. Commun., 2009, 6652 Search PubMed; M. Miyasaka, M. Pink, S. Rajca and A. Rajca, Angew. Chem., Int. Ed., 2009, 48, 5954 RSC; C. L. Li, J. W. Shi, L. Xu, Y. G. Wang, Y. X. Chen and H. Wang, J. Org. Chem., 2009, 74, 408 CrossRef CAS; J. Štorch, J. Sýkora, J. Čermák, J. Karban, I. Císařová and A. Růžička, J. Org. Chem., 2009, 74, 3090 CrossRef CAS; M. S. M. Pearson and D. R. Carbery, J. Org. Chem., 2009, 74, 5320 CrossRef CAS; J. Cote and C. K. Collins, Synthesis, 2009, 1499 CrossRef CAS; S. Graule, M. Rudolph, N. Vanthuyne, J. Autschbach, C. Roussel, J. Crassous and R. Réau, J. Am. Chem. Soc., 2009, 131, 3183 CAS; S. Goretta, C. Tasciotti, S. Mathieu, M. Smet, W. Maes, Y. M. Chabre, W. Dehaen, R. Giasson, J. M. Raimundo, C. R. Henry, C. Barth and M. Gingras, Org. Lett., 2009, 11, 3846 CrossRef CAS; G. Pieters, A. Gaucher, D. Prim and J. Marrot, Chem. Commun., 2009, 4827 CrossRef CAS; C. Kim, T. J. Marks, A. Facchetti, M. Schiavo, A. Bossi, S. Maiorana, E. Licandro, F. Todescato, S. Toffanin, M. Muccini, C. Graiff and A. Tiripicchio, Org. Electron., 2009, 10, 1511 RSC; F. Aloui, R. El Abed, A. Marinetti and B. Ben Hassine, C. R. Chim., 2009, 12, 284 CrossRef CAS; F. Aloui and B. Ben Hassine, Tetrahedron Lett., 2009, 50, 4321 CrossRef CAS; A. Grandbois and S. K. Collins, Chem.–Eur. J., 2008, 14, 9323 CrossRef CAS; J. Ichikawa, M. Yokota, T. Kudo and S. Umezaki, Angew. Chem., Int. Ed., 2008, 47, 4870 CrossRef; H. Sugiura, R. Amemiya and M. Yamaguchi, Chem.–Asian J., 2008, 3, 244 CrossRef CAS; M. Shimizu, I. Nagao, Y. Tomioka and T. Hiyama, Angew. Chem., Int. Ed., 2008, 47, 8096 CrossRef CAS; G. Lamanna, C. Faggi, F. Gasparrini, A. Ciogli, C. Villani., P. J. Stephens, F. J. Devlin and S. Menichetti, Chem.–Eur. J., 2008, 14, 5747 CrossRef CAS; L. Rulíšek, O. Exner, L. Cwiklik, P. Jungwirth, I. Starý, L. Pospíšil and Z. Havlas, J. Phys. Chem. C, 2007, 111, 14948 CrossRef CAS; X. Xue and L. T. Scott, Org. Lett., 2007, 9, 3937 CrossRef CAS.
- T. Kaseyama, S. Furumi, X. Zhang, K. Tanaka and M. Takeuchi, Angew. Chem., Int. Ed., 2011, 50, 3684 CrossRef CAS; P. Rahe, M. Nimmrich, A. Greuling, J. Schütte, I. G. Stará, J. Rybáček, G. Huerta-Angeles, I. Starý, M. Rohlfing and A. Kühnle, J. Phys. Chem. C, 2010, 114, 1547 CrossRef CAS; M. A. Shcherbina, X. B. Zeng, T. Tadjiev, G. Ungar, S. H. Eichhorn, K. E. S. Phillips and T. J. Katz, Angew. Chem., Int. Ed., 2009, 48, 7837 CrossRef CAS; R. Fasel, M. Parschau and K.-H. Ernst, Nature, 2006, 439, 449 CrossRef CAS.
- For molecular recognition with helicenoids, see: M. T. Reetz and S. Sostmann, Tetrahedron, 2001, 57, 2515 Search PubMed; K. Yamamoto, T. Ikeda, T. Kitsuki, Y. Okamoto, H. Chikamatsu and M. Nakazaki, J. Chem. Soc., Perkin Trans. 1, 1990, 271 CrossRef CAS; D. J. Weix, S. D. Dreher and T. J. Katz, J. Am. Chem. Soc., 2000, 122, 10027 RSC; E. Murguly, R. McDonald and N. R. Branda, Org. Lett., 2000, 2, 3169 CrossRef CAS; L. Owens, C. Thilgen, F. Diederich and C. B. Knobler, Helv. Chim. Acta, 1993, 76, 2757 CrossRef CAS.
-
(a) J. Chen, B. Captain and N. Takenaka, Org. Lett., 2011, 13, 1654 Search PubMed;
(b) M. R. Crittall, H. S. Rzepa and D. R. Carbery, Org. Lett., 2011, 13, 1250 CrossRef CAS;
(c) M. Šámal, J. Míšek, I. G. Stará and I. Starý, Collect. Czech. Chem. Commun., 2009, 74, 1151 CrossRef CAS;
(d) N. Takenaka, J. Chen, B. Captain, R. S. Sarangthem and A. Chandrakumar, J. Am. Chem. Soc., 2010, 132, 4536 Search PubMed;
(e) N. Takenaka, R. S. Sarangthem and B. Captain, Angew. Chem., Int. Ed., 2008, 47, 9708 CrossRef CAS;
(f) J. S. Chen and N. Takenaka, Chem.–Eur. J., 2009, 15, 7268 CrossRef CAS;
(g) M. T. Reetz, E. W. Beuttenmüller and R. Goddard, Tetrahedron Lett., 1997, 38, 3211 CrossRef CAS;
(h) A. Terfort, H. Görls and H. Brunner, Synthesis, 1997, 79 CrossRef CAS;
(i) M. T. Reetz and S. Sostmann, J. Organomet. Chem., 2000, 603, 105 CrossRef CAS;
(j) A. Aloui, R. El Abed, A. Marinetti and B. Ben Hassine, Tetrahedron Lett., 2007, 48, 2017 Search PubMed;
(k) I. Sato, R. Yamashima, K. Kadowaki, J. Yamamoto, T. Shibata and K. Soai, Angew. Chem., Int. Ed., 2001, 40, 1096 CrossRef CAS;
(l) H. Okubo, M. Yamaguchi and C. Kabuto, J. Org. Chem., 1998, 63, 9500 CrossRef CAS;
(m) S. D. Dreher, T. J. Katz, K.-C. Lam and A. L. Rheingold, J. Org. Chem., 2000, 65, 815 CrossRef CAS.
- T. Verbiest, S. Van Elshocht, M. Kauranen, L. Hellemans, J. Snauwaert, C. Nuckolls, T. J. Katz and A. Persoons, Science, 1998, 282, 913 CrossRef CAS; C. Nuckolls, T. J. Katz, G. Katz, P. J. Collings and L. Castellanos, J. Am. Chem. Soc., 1999, 121, 79 CrossRef CAS; C. Nuckolls, T. J. Katz and L. Castellanos, J. Am. Chem. Soc., 1996, 118, 3767 CrossRef CAS; T. J. Wigglesworth, D. Sud, T. B. Norsten, V. S. Lekhi and N. R. Branda, J. Am. Chem. Soc., 2005, 127, 7272 CrossRef CAS; E. Botek, B. Champagne, M. Turki and J.-M. André, J. Chem. Phys., 2004, 120, 2042 CrossRef CAS; C. Nuckolls, T. J. Katz, T. Verbiest, S. Van Elshocht, H.-G. Kuball, S. Kiesewalter, A. J. Lovinger and A. Persoons, J. Am. Chem. Soc., 1998, 120, 8656 CrossRef CAS.
- S. Ehala, J. Míšek, I. G. Stará, I. Starý and V. Kašička, J. Sep. Sci., 2686, 2008, 31 Search PubMed; J. Míšek, M. Tichý, I. G. Stará, I. Starý, J. Roithová and D. Schröder, Croat. Chem. Acta, 2009, 82, 79 Search PubMed.
- K. Shinohara, Y. Sannohe, S. Kaieda, K. Tanaka, H. Osuga, H. Tahara, Y. Xu, T. Kawase, T. Bando and H. Sugiyama, J. Am. Chem. Soc., 2010, 132, 3778 CrossRef CAS.
- S. Basili, G. Basso, A. Faccio, A. Granzhan, H. Ihmels, S. Moro and G. Viola, ChemMedChem, 2008, 3, 1671 Search PubMed.
- Y. Xu, Y. X. Zhang, H. Sugiyama, T. Umano, H. Osuga and K. Tanaka, J. Am. Chem. Soc., 2004, 126, 6566 CrossRef CAS; S. Honzawa, H. Okubo, S. Anzai, M. Yamaguchi, K. Tsumoto and I. Kumagai, Bioorg. Med. Chem., 2002, 10, 3213 CrossRef CAS.
- A. Granzhan, H. Ihmels and K. Jäger, Chem. Commun., 2009, 1249 RSC.
- For an excellent overview, see: C. Wolf, Dynamic Stereochemistry of Chiral Compounds, RSC Publishing, Cambridge, UK, 2008, pp. 53–54 Search PubMed; for early studies: K. Yamada, H. Nakagawa and H. Kawazura, Bull. Chem. Soc. Jpn., 1986, 59, 2429 Search PubMed; R. H. Martin and M. J. Marchant, Tetrahedron, 1974, 30, 347 Search PubMed; R. H. Martin and M. J. Marchant, Tetrahedron Lett., 1972, 13, 3707 CAS; C. Goedicke and H. Stegemeyer, Tetrahedron Lett., 1970, 11, 937 CrossRef CAS; H. Wynberg and M. B. Groen, J. Chem. Soc. D, 1969, 964 Search PubMed; D. M. Hall, J. Chem. Soc., 1956, 3674 CrossRef; A. Mannschreck, E. Gmahl, T. Burgemeister, F. Kastner and V. Sinnwell, Angew. Chem., Int. Ed. Engl., 1988, 27, 270 RSC; R. H. Martin and V. Libert, J. Chem. Res. (S), 1980, 30, 130 RSC ; ref. 2a, 2b, 2e, 2f.
- For recent studies: ref. 3b, J. Guin, C. Besnard and J. Lacour, Org. Lett., 2010, 12, 1748 Search PubMed; G. Pieters, A. Gaucher, S. Marque, F. Maurel, P. Lesot and D. Prim, J. Org. Chem., 2010, 75, 2096 CrossRef CAS; M. Llunell, P. Alemany and J. M. Bofill, ChemPhysChem, 2008, 9, 1117 CrossRef CAS; M. C. Carreño, A. Enríquez, S. García-Cerrada, M. J. Sanz-Cuesta, A. Urbano, F. Maseras and A. Nonell-Canals, Chem.–Eur. J., 2008, 14, 603 Search PubMed; K. Shiraishi, A. Rajca, M. Pink and S. Rajca, J. Am. Chem. Soc., 2005, 127, 9312 CrossRef CAS; Z. Y. Wang, E. K. Todd, X. S. Meng and J. P. Gao, J. Am. Chem. Soc., 2005, 127, 11552 CrossRef CAS; A. Rajca, M. Miyasaka, M. Pink, H. Wang and S. Rajca, J. Am. Chem. Soc., 2004, 126, 15211 CrossRef CAS; F. Lebon, G. Longhi, F. Gangemi, S. Abbate, J. Priess, M. Juza., C. Bazzini, T. Caronna and A. Mele, J. Phys. Chem. A, 2004, 108, 11752 CrossRef CAS.
- For theoretical studies, see:
(a) S. Grimme and S. D. Peyerimhoff, Chem. Phys., 1996, 204, 411 CrossRef CAS;
(b) R. H. Janke, G. Haufe, E. U. Würthwein and J. H. Borkent, J. Am. Chem. Soc., 1996, 118, 6031 CrossRef CAS;
(c) H. J. Lindner, Tetrahedron, 1975, 31, 281 Search PubMed;
(d) J. H. Borkent and W. H. Laarhoven, Tetrahedron, 1978, 34, 2565 CrossRef CAS.
- For a predicted [11]anthrahelicene saddle-shaped intermediate, see: P. Sehnal, I. G. Stará, D. Šaman, M. Tichý, J. Míšek, J. Cvačka, L. Rulíšek, J. Chocholoušová, J. Vacek, G. Goryl, M. Szymonski, I. Císařová and I. Starý, Proc. Natl. Acad. Sci. U. S. A., 2009, 106, 13169 Search PubMed.
- An interesting strategy to fix the saddle-shaped helicene species has been recently proposed in a theoretical paper by Patzschke and Johansson: M. P. Johansson and M. Patzschke, Chem.–Eur. J., 2009, 15, 13210 Search PubMed.
- L. Adriaenssens, L. Severa, T. Šálová, I. Císařová, R. Pohl, D. Šaman, S. V. Rocha, N. S. Finney, L. Pospíšil, P. Slavíček and F. Teplý, Chem.–Eur. J., 2009, 15, 1072 CAS.
- L. Severa, D. Koval, P. Novotná, M. Ončák, P. Sázelová, D. Šaman, P. Slavíček, M. Urbanová, V. Kašička and F. Teplý, New J. Chem., 2010, 34, 1063 RSC.
- L. Severa, L. Adriaenssens, J. Vávra, D. Šaman, I. Císařová, P. Fiedler and F. Teplý, Tetrahedron, 2010, 66, 3537 CrossRef CAS.
- L. Pospíšil, F. Teplý, M. Gál, L. Adriaenssens, M. Horáček and L. Severa, Phys. Chem. Chem. Phys., 2010, 12, 1550 RSC.
- [rac-2][PF6]2 (CCDC 829266), [rac-5][TfO]2 (CCDC 829267), [Sa,Ra-4][(R, R)-dibenzoyl tartrate]2 (CCDC 828959), and [P-5][ClO4]2 (CCDC 829265)†.
- Intramolecular [2 + 2 + 2] cycloaddition as an entry to non-ionic helical scaffolds was pioneered in the group of Stará and Starý, for initial reports, see:
(a) I. G. Stará, I. Starý, A. Kollárovič, F. Teplý, D. Šaman and M. Tichý, J. Org. Chem., 1998, 63, 4046 CrossRef CAS;
(b) I. G. Stará, I. Starý, A. Kollárovič, F. Teplý, Š. Vyskočil and D. Šaman, Tetrahedron Lett., 1999, 40, 1993 CrossRef CAS;
(c) F. Teplý, I. G. Stará, I. Starý, A. Kollárovič, D. Šaman, L. Rulíšek and P. Fiedler, J. Am. Chem. Soc., 2002, 124, 9175 CrossRef CAS; for further reports see also:
(d) K. Tanaka, N. Fukawa, T. Suda and K. Noguchi, Angew. Chem., Int. Ed., 2009, 48, 5470 CrossRef CAS;
(e) K. Tanaka, A. Kamisawa, T. Suda, K. Noguchi and M. Hirano, J. Am. Chem. Soc., 2007, 129, 12078 CrossRef CAS;
(f) S. Han, A. D. Bond, R. L. Disch, D. Holmes, J. M. Schulman, S. J. Teat, K. P. C. Vollhardt and G. D. Whitener, Angew. Chem., Int. Ed., 2002, 41, 3223 CrossRef CAS; see also overview in:
(g) A. Rajca and S. Rajca, Angew. Chem., Int. Ed., 2010, 49, 672 CAS, and ref. 7b, 17.
- Ratio of [4]
:
[5] (4
:
1) determined by integration of peaks in NMR spectra after the cyclization experiment conducted at 105 °C/30 min. See ESI†.
- For enantiocomposition analysis of helquats by CE, see ref. 20 and: D. Koval, L. Severa, L. Adriaenssens, J. Vávra, F. Teplý and V. Kašička, Electrophoresis, 2011 DOI:10.1002/elps.201100173.
- Sulfated γ-cyclodextrin sodium salt was obtained from Beckman-Coulter as 20 wt% solution in water (cat. no. A50924).
- P. Sehnal, Z. Krausová, F. Teplý, I. G. Stará, I. Starý, L. Rulíšek, D. Šaman and I. Císařová, J. Org. Chem., 2008, 73, 2074 CrossRef CAS; A. Andronová, F. Szydlo, F. Teplý, M. Tobrmanová, A. Volot, I. G. Stará, I. Starý, L. Rulíšek, D. Šaman, J. Cvačka, P. Fiedler and P. Vojtíšek, Collect. Czech. Chem. Commun., 2009, 74, 189 Search PubMed.
-
TURBOMOLE V6.1 2009, University of Karlsruhe and Forschungszentrum Karlsruhe GmbH, 1989–2007 Search PubMed, TURBOMOLE GmbH, since 2007, available from http://www.turbomole.com.
-
(a) S. Grimme, J. Comput. Chem., 2004, 25, 1463 CrossRef CAS;
(b) S. Grimme, J. Comput. Chem., 2006, 27, 1787 CrossRef.
-
COSMO model:
(a) A. Klamt and G. Schuurmann, J. Chem. Soc., Perkin Trans. 2, 1993, 799 RSC;
(b) A. Schäfer, A. Klamt, D. Sattel, J. C. W. Lohrenz and F. Eckert, Phys. Chem. Chem. Phys., 2000, 2, 2187 RSC.
- The energy difference between species 4 and 5 is similar for the calculations performed with ditriflates in the gas phase.
- For a recent successful resolution of non-ionic 1-aza[6]helicenevia its dibenzoyltartrate diastereoisomeric salts, see: J. Míšek, F. Teplý, I. G. Stará, M. Tichý, D. Šaman, I. Císařová, P. Vojtíšek and I. Starý, Angew. Chem., Int. Ed., 2008, 47, 3188 Search PubMed.
-
(a) K. Mislow, Science, 1954, 120, 232 CrossRef CAS;
(b) K. Mislow and R. Bolstad, J. Am. Chem. Soc., 1955, 77, 6712 CrossRef CAS.
- Specifically [6]helquat 5 is an example of a “Euclidean rubber glove” molecule, see: J. C. Chambron, J. P. Sauvage, K. Mislow, A. De Cian and J. Fischer, Chem.–Eur. J., 2001, 7, 4085 Search PubMed.
- For further examples of aromatics with interesting conformation behaviour and shape, see: G. S. Kottas, L. I. Clarke, D. Horinek and J. Michl, Chem. Rev., 2005, 105, 1281 Search PubMed; A. R. Karim, A. Linden, K. K. Baldridge and J. S. Siegel, Chem. Sci., 2010, 1, 102 CrossRef CAS; T. A. V. Khuong, J. E. Nuñez, C. E. Godinez and M. A. Garcia-Garibay, Acc. Chem. Res., 2006, 39, 413 RSC; R. Eelkema, M. M. Pollard, J. Vicario, N. Katsonis, B. Serrano Ramon, C. W. M. Bastiaansen, D. J. Broer and B. L. Feringa, Nature, 2006, 440, 163 CrossRef CAS; T. R. Kelly, H. De Silva and R. A. Silva, Nature, 1999, 401, 150 CrossRef; R. S. Walters, C. M. Kraml, N. Byrne, D. M. Ho, Q. Qin, F. J. Coughlin, S. Bernhard and R. A. Pascal Jr, J. Am. Chem. Soc., 2008, 130, 16435 CrossRef CAS; R. A. Pascal Jr, Chem. Rev., 2006, 106, 4809 CrossRef CAS; C. L. Hilton, J. M. Crowfoot, P. Rempala and B. T. King, J. Am. Chem. Soc., 2008, 130, 13392 CrossRef; Y. Wang, A. D. Stretton, M. C. McConnell, P. A. Wood, S. Parsons, J. B. Henry, A. R. Mount and T. H. Galow, J. Am. Chem. Soc., 2007, 129, 13193 CrossRef CAS; M. S. Betson, J. Clayden, C. P. Worrall and S. Peace, Angew. Chem., Int. Ed., 2006, 45, 5803 CrossRef CAS; D. Zehm, W. Fudickar and T. Linker, Angew. Chem., Int. Ed., 2007, 46, 7689 CrossRef CAS; D. Ajami, O. Oeckler, A. Simon and R. Herges, Nature, 2003, 426, 819 CrossRef CAS.
- During preparation of this manuscript an interesting saddle-shaped system was isolated and introduced by Bertozzi et al. However, it has not been studied in the context of its transformation to the corresponding helix. Interestingly, synthesis of this saddle-shaped system relies on non-catalyzed intermolecular [2 + 2 + 2] cycloaddition, see: E. M. Sletten, H. Nakamura, J. C. Jewett and C. R. Bertozzi, J. Am. Chem. Soc., 2010, 132, 11799 Search PubMed.
- For a recent report on isomerization of bis(metallahelicene), see: E. Anger, M. Rudolph, C. Shen, N. Vanthuyne, L. Toupet, C. Roussel, J. Autschbach, J. Crassous and R. Réau, J. Am. Chem. Soc., 2011, 133, 3800 Search PubMed.
|
This journal is © The Royal Society of Chemistry 2011 |