DOI:
10.1039/C0NR00758G
(Paper)
Nanoscale, 2011,
3, 1558-1567
Curcumin-loaded biodegradable polymeric micelles for colon cancer therapy in vitro and in vivo
Received
12th October 2010
, Accepted 28th November 2010
First published on 31st January 2011
Abstract
Curcumin is an effective and safe anticancer agent, but its hydrophobicity inhibits its clinical application. Nanotechnology provides an effective method to improve the water solubility of hydrophobic drug. In this work, curcumin was encapsulated into monomethoxy poly(ethylene glycol)-poly(ε-caprolactone) (MPEG-PCL) micelles through a single-step nano-precipitation method, creating curcumin-loaded MPEG-PCL (Cur/MPEG-PCL) micelles. These Cur/MPEG-PCL micelles were monodisperse (PDI = 0.097 ± 0.011) with a mean particle size of 27.3 ± 1.3 nm, good re-solubility after freeze-drying, an encapsulation efficiency of 99.16 ± 1.02%, and drug loading of 12.95 ± 0.15%. Moreover, these micelles were prepared by a simple and reproducible procedure, making them potentially suitable for scale-up. Curcumin was molecularly dispersed in the PCL core of MPEG-PCL micelles, and could be slow-released in vitro. Encapsulation of curcumin in MPEG-PCL micelles improved the t1/2 and AUC of curcuminin vivo. As well as free curcumin, Cur/MPEG-PCL micelles efficiently inhibited the angiogenesis on transgenic zebrafish model. In an alginate-encapsulated cancer cell assay, intravenous application of Cur/MPEG-PCL micelles more efficiently inhibited the tumor cell-induced angiogenesisin vivo than that of free curcumin. MPEG-PCL micelle-encapsulated curcumin maintained the cytotoxicity of curcumin on C-26 colon carcinoma cellsin vitro. Intravenous application of Cur/MPEG-PCL micelle (25 mg kg−1curcumin) inhibited the growth of subcutaneous C-26 colon carcinoma in vivo (p < 0.01), and induced a stronger anticancer effect than that of free curcumin (p < 0.05). In conclusion, Cur/MPEG-PCL micelles are an excellent intravenously injectable aqueous formulation of curcumin; this formulation can inhibit the growth of colon carcinoma through inhibiting angiogenesis and directly killing cancer cells.
1. Introduction
Medicines derived from plants play an important role in the healthcare of many cultures, both ancient and modern.1–4Curcumin (diferuloylmethane, a polyphenol) is an active principle of the perennial herb Curcuma longa (commonly known as turmeric); its molecular structure is presented in Fig. 1a. Turmeric has been used as spice, coloring agent and folk medicine for many centuries, and recently, many therapeutic effects of curcumin have been confirmed by modern science.5–8 The potential anticancer effect of curcumin has attracted great attention, and extensive research has been carried out in this direction, gradually revealing the molecular targets involved in cancer treatment using curcumin.9–13 Previous excellent studies have suggested that curcumin is a universal anticancer agent, suppressing many types of cancers;13–24 while it also has potential in overcoming multidrug-resistant cancers,25 and shows a synergistic antitumor effect with other anticancer agents.26–29 More importantly, to date, no studies in either animals or humans have discovered any toxicity associated with the use of curcumin.30,31 Currently, curcumin (as an anticancer drug) is the subject of clinical trials.32
While the pharmacodynamics and pharmacology of curcumin attract great attention, the formulation of curcumin needs also to be addressed, because curcumin itself is water-insoluble. Traditionally, curcumin is orally administered, but its oral bioavailability is very low, and a high dosage of curcumin must be applied.31,33–36 Therefore, it is of interest to develop an intravenously injectable aqueous formulation for curcumin.37 Some attempts have already been made to develop aqueous curcumin formulations, such as a PAMAM dendrimer curcumin conjugate,38 a PEG–curcumin conjugate,39 a cyclodextrin–curcumin complex,40 liposomal curcumin,19,41 and phospholipid-based curcumin-encapsulated microemulsions.42 Although those curcumin formulations are novel and interesting, an ideal aqueous formulation of curcumin is still very desirable.
Nanotechnology provides a novel method to overcome the poor water solubility of lipophilic drugs.43 Encapsulation of hydrophobic drugs into nanoparticles can render the drug completely dispersible in water, making the drug intravenously injectable. Biodegradable polymeric nanoparticles are viewed as excellent candidates for anticancer drug delivery systems,44–48 and some biodegradable polymer nanoparticle-delivered anticancer drugs are already marketed.49PCL/PEG copolymers are biodegradable, amphiphilic and easy to produce, showing promising applications in drug delivery systems.50,51
MPEG-PCL is a diblock PCL/PEG copolymer; its molecular structure is shown in Fig. 1b. Previously, we prepared self-assembled MPEG-PCL micelles and used them to deliver honokiol, creating a novel honokiol formulation.52 In attempt to develop an aqueous formulation for curcumin, in this work, we prepared and characterized MPEG-PCL micelles containing curcumin. We then evaluated the potential of these Cur/MPEG-PCL micelles in inhibiting angiogenesis and suppressing the growth of colon carcinoma in vitro and in vivo. These results suggested that Cur/MPEG-PCL micelles are an excellent intravenously injectable aqueous formulation of curcumin, with potential application in antiangiogenesis and colon carcinoma therapy.
2. Materials and methods
2.1 Materials
Curcumin, alginate, FITC-dextran, Dulbecco's modified Eagle's medium (DMEM) and 3-(4,5-dimethylthiazol-2-yl)-2,5-diphenyl tetrazolium bromide (MTT) were purchased from Sigma (USA). Methanol and acetic acid (HPLC grade) were purchased from Fisher Scientific (UK). Dimethyl sulfoxide (DMSO) and acetone were purchased from KeLong Chemicals (China). MPEG-PCL diblock copolymer with a designed molecular weight of 4000 was synthesized according to our previous reports.52 The Mn of MPEG-PCL copolymer was ∼4050 (determined by 1H-NMR, data not shown).
Tg(flk:EGFP) transgenic zebrafish were provided by Dr Shuo Lin (UCLA, USA). Female BALB/c mice (6–8 weeks old) and Sprague-Dawley (SD) rats (weight of 180 ± 20 g) were purchased from the Laboratory Animal Center of Sichuan University (Chengdu, China). All studies involving mice were approved by the institute's animal care and use committee.
2.2 Preparation of Cur/MPEG-PCL micelles
Cur/MPEG-PCL micelles were prepared by a single-step nano-precipitation method. Curcumin and MPEG-PCL diblock copolymer (500 mg) were co-dissolved in 5 mL of acetone; this solution was added into 5 mL of water while stirring constantly. In this process, MPEG-PCL self-assembled into core-shell structured micelles with core-encapsulated curcumin. Then, the acetone was evaporated in rotary evaporator. The Cur/MPEG-PCL micelle solution was filtered using a syringe filter (pore size: 220 nm) (Millex-LG, Millipore Co., USA) to remove the impurity and bacterium. Finally, the prepared Cur/MPEG-PCL micelles were lyophilized and storage at 4 °C.
2.3 Characterization of Cur/MPEG-PCL micelles
The particle size of Cur/MPEG-PCL micelles was determined by dynamic light scattering (Malvern Nano-ZS 90). The temperature was kept at 25 °C during the measuring process. All results were the mean of 3 test runs.
The morphology of Cur/MPEG-PCL micelles was observed under a transmission electron microscope (TEM) (H-6009IV, Hitachi, Japan): micelles were diluted with distilled water and placed on a copper grid covered with nitrocellulose. Samples were negatively stained with phosphotungstic acid and dried at room temperature.
X-ray diffraction (XRD) spectra of free curcumin, blank MPEG-PCL micelles, and Cur/MPEG-PCL micelles were determined using an X-ray diffractometer (X'Pert Pro, Philips, Netherlands) using Cu Kα radiation.
The thermal properties of free curcumin, blank MPEG-PCL micelles, and Cur/MPEG-PCL micelles were studied using a differential scanning calorimeter (DSC) (NETSCZ 204, Germany). Samples were heated from 10 °C to 200 °C under nitrogen atmosphere at a heating rate of 10 °C min−1. The DSC curves were recorded.
The stability of Cur/MPEG-PCL micelles was evaluated qualitatively. Cur/MPEG-PCL micelle aqueous solution was kept at 4 °C for 24 h; then, aggregates of Cur/MPEG-PCL micelles were examined by naked eye. The presence of precipitation indicates instability of the Cur/MPEG-PCL micelles, while a uniformly transparent solution indicates stability.
The concentration of curcumin was determined by High Performance Liquid Chromatography (HPLC, Waters Alliance 2695). Solvent delivery system was equipped with a column heater and a plus autosampler. Detection was carried out on a Waters 2996 detector. Chromatographic separations were performed on a reversed phase C18 column (4.6 × 150 mm–5 μm, Sunfire Analysis column), with the column temperature kept at 28 °C. Methanol–0.3% acetic acid (80/20, v/v) was used as eluent at a flow rate of 1 mL min−1.
Drug loading (DL) and encapsulation efficiency (EE) of Cur/MPEG-PCL micelles were determined as follows. Briefly, 10 mg of lyophilized Cur/MPEG-PCL micelles were dissolved in 0.1 mL dichloromethane (DCM) and was diluted with methanol. The amount of curcumin in the solution was determined by HPLC. Finally, the drug loading (DL) and encapsulation efficiency (EE) of Cur/MPEG-PCL micelles were calculated according to eqn (1) and (2):
| 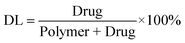 | (1) |
|  | (2) |
2.4 Computer simulation
The interaction between curcumin and PEG or PCL was simulated. The initial structures of PCL (n = 18) and PEG (n = 45) were constructed using HyperChem53 software, and optimized at the molecular mechanics level with the OPLS54 method. Then the optimized structures were subjected to a series of molecular dynamics simulations: heating from 0 K to 600 K, simulating at 600 K, cooling from 600 K to 300 K, and running at 300 K. Simulated structures were run for 100 ps at each stage. In order to explore the recognition of polymers PCL for curcumin, a docking study was conducted using AutoDock Vina,55 and the suggested conformation of the PCL–curcumin complex was obtained.
2.5
In vitro release study
To determine the release kinetics of curcumin from Cur/MPEG-PCL micelles, 0.5 mL of Cur/MPEG-PCL micelle solution was placed in a dialysis bag (molecular weight cutoff, 3.5 kDa). Dialysis bags were incubated in 30 mL of phosphate buffer solution (pH 7.4) containing Tween-80 (0.5% w/w) at 37 °C with gentle shaking. At predetermined time points, the incubation medium was replaced with fresh incubation medium. The amount of released drug in the incubation medium was quantified by HPLC. This study was repeated three times, and the result was expressed as mean value ± sd.
2.6 Pharmacokinetics study
Twenty four hours prior to assays, rats were subjected to jugular venous cannulation. Under chloral hydrate anaesthesia, 3 cm of the cannula was introduced into the jugular vein toward the heart. The free end of the cannula was subcutaneously conducted to the dorsal base of the neck, and the exterior end was closed with a plug. The inside of implanted cannulas remained permanently filled with heparinized normal saline. These rats were fasted overnight prior to drug administration, and were divided into two groups (3 rats in each group): a curcumin treatment group and a Cur/MPEG-PCL micelle treatment group. Free curcumin was dissolved in Cremophor EL (polyoxyl 35 castor oil) and dehydrated alcohol (1
:
1, v/v); and Cur/MPEG-PCL micelles were dissolved in normal saline. Following the intravenous administration of 100 mg kg−1 of free curcumin or micelle-encapsulated curcumin (Cur/MPEG-PCL micelles), the blood was collected from the jugular venous cannula at different time intervals. Plasma was separated and extracted with ethyl acetate; and supernatant fluid was collected and evaporated to dryness. The dry residues were dissolved in methanol for HPLC analysis. Tmax and Cmax values were recorded directly from the measured data. Then t1/2, AUC0→t and AUC0→∞ values were calculated. The Drug and Statistics (DAS) software (version 2.1.1, edited and published by the Mathematical Pharmacology Professional Committee of China), was used for pharmacokinetic analysis.
2.7 Transgenic zebrafish model
The transgenic Tg(flk: EGFP) zebrafish line was raised by standard methods as previously described.56 After transplantation procedures, embryos were arrayed into 24-well plates (4–5 embryos per well) in 1 mL of Holtfreter's solution, and maintained at 28 °C. Forty-eight hours later, embryos were treated with Holtfreter's solution (control), free curcumin (5 μg mL−1) or Cur/MPEG-PCL micelles (5 μg mL−1curcumin) for 72 h. Zebrafish blood vessels were examined using a Zeiss M2Bio fluorescence microscope (Carl Zeiss Microimaging Inc.).
2.8
Alginate-encapsulated tumor cell assay
An alginate-encapsulated tumor cell assay was performed as described previously.57C-26 colon carcinoma cells were re-suspended in a solution of alginate (1.8%) and dropped into a solution of calcium chloride (250 mM), forming alginate beads containing 1 × 105 tumor cells per bead. The bead was then implanted subcutaneously into an incision made on the dorsal side of mouse on day 0. Twelve bead-bearing mice were divided into four groups (3 mice per group), and treated with Cur/MPEG-PCL micelles (curcumin: 25 mg kg−1), free curcumin (25 mg kg−1), MPEG-PCL micelles (168 mg kg−1), or normal saline once a day for 5 days, respectively. On day 12, mice were injected intravenously with 0.1 mL of a 100 mg kg−1 FITC-dextran solution. Alginate beads were photographed and rapidly removed 20 min after FITC-dextran injection. The uptake of FITC-dextran was measured as described.57
2.9 Analysis of cytotoxicity
The cytotoxicity of free curcumin or MPEG-PCL micelle-encapsulated curcumin to C-26 cell line was evaluated by MTT method. C-26 cells were plated at a density of 5 × 103cells per well in 100 μL of DMEM containing 10% FBS in 96-well plates and grown for 24 h. Cells were then exposed to a series of free curcumin or micelle-encapsulated curcumin at different concentrations for 48 h. The viability of cells was measured using the MTT method. Results were the mean of six test runs.
2.10
In vivo tumor model
Female BALB/c mice (6–8 weeks old) were subcutaneously inoculated with 1.5 × 106C-26 cells on day 0. On day 4, the tumors were detectable. Then, the mice were randomized into four groups (5 mice per group). The four groups were intravenously injected with normal saline (control), empty MPEG-PCL micelles (168 mg kg−1), 25 mg kg−1 of free curcumin and 25 mg kg−1 of MPEG-PCL micelle-encapsulated curcumin daily for ten days, respectively. The tumor size was measured externally daily using calipers during the experimental period. The tumor volume was approximated by using the equation volume = ab2/2, where a is the length of the major axis, and b is the length of the minor axis. On day 18, the mice were killed by cervical vertebra dislocation, and their tumors were immediately harvested and analyzed.
2.11 Statistical analysis
Data were expressed as the mean value ± sd. Statistical analysis was performed with one-way analysis of variance (ANOVA) using SPSS software. P values less than 0.05 were considered to be statistically significant.
3. Results
Biodegradable polymeric nanoparticles (as a drug delivery system) have promising applications in solving the issue of poor water solubility of hydrophobic drugs. The focus of this work was to use MPEG-PCL micelles to encapsulate hydrophobic curcumin, with a goal to develop an intravenously injectable aqueous formulation for curcumin.
3.1 Preparation and characterization of Cur/MPEG-PCL micelles
Cur/MPEG-PCL micelles were prepared through a single-step nano-precipitation method, as schematically presented in Fig. 2. Adding MPEG-PCL and curcumin co-dissolved acetone solution into water resulted in the precipitation of MPEG-PCL and curcumin; in this process, MPEG-PCL self-assembled into core-shell structured micelles with core-encapsulated curcumin. To optimize the process parameters, we studied the effect of curcumin/MPEG-PCL mass ratio in the feed on the properties of the resultant micelles, and results are presented in Table 1. In our experimental condition, the encapsulation efficiency was very high (>97%). The drug loading, particle size and PDI increased with increase in curcumin/MPEG-PCL mass ratio in the feed. When the curcumin/MPEG-PCL mass ratio in the feed was ≥20/100, the obtained Cur/MPEG-PCL micelles were not stable in vitro (micelles tended to aggregate, forming aggregates); thus, we chose 15/100 as the curcumin/MPEG-PCL mass ratio in feed in our following protocol.
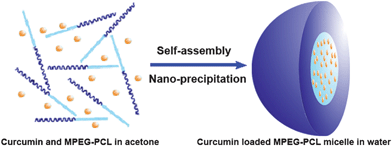 |
| Fig. 2 Preparation scheme of Cur/MPEG-PCL micelles using a nano-precipitation method. Adding curcumin and MPEG-PCL co-dissolved acetone solution into water resulted in the precipitation of MPEG-PCL and curcumin; in this process, MPEG-PCL self-assembled into core-shell structured micelles with core-encapsulated curcumin. | |
Table 1 Properties of curcumin-loaded MPEG-PCL micelles
Sample no. |
Curcumin/MPEG-PCL mass ratio in feed |
Encapsulation efficiency (%) |
Drug loading (%) |
Mean size (nm) |
Polydispersity index (PDI) |
Stability |
C-0
|
0/100 |
0 |
0 |
24.5 ± 0.9 |
0.069 ± 0.004 |
✓ |
C-1
|
5/100 |
99.04 ± 1.24 |
4.72 ± 0.06 |
24.4 ± 1.1 |
0.089 ± 0.009 |
✓ |
C-2 |
10/100 |
99.14 ± 1.12 |
9.02 ± 0.11 |
26.1 ± 1.6 |
0.091 ± 0.008 |
✓ |
C-3
|
15/100 |
99.16 ± 1.02 |
12.95 ± 0.15 |
27.3 ± 1.3 |
0.097 ± 0.011 |
✓ |
C-4
|
20/100 |
98.20 ± 1.07 |
16.42 ± 0.21 |
31.2 ± 1.4 |
0.153 ± 0.021 |
✗ |
C-5
|
25/100 |
97.40 ± 1.17 |
19.58 ± 0.29 |
33.9 ± 2.5 |
0.188 ± 0.029 |
✗ |
Sample C-3 was characterized in detail, and used for future applications. The encapsulation efficiency and drug loading were 99.16 ± 1.02% and 12.95 ± 0.15%, respectively. Moreover, these Cur/MPEG-PCL micelles were monodisperse (PDI = 0.097 ± 0.011) with mean particle size of 27.3 ± 1.3 nm (determined by DLS). In Fig. 3a, the particle size distribution spectrum of freshly prepared Cur/MPEG-PCL micelles were presented; this indicated that Cur/MPEG-PCL micelles had a very narrow particle size distribution. According to the TEM image of Cur/MPEG-PCL micelles (Fig. 3b), these micelles were spherical and monodisperse with a mean diameter of ∼23 nm. After Cur/MPEG-PCL micelles were freeze-dried, the re-solubility of these lyophilized Cur/MPEG-PCL micelles was examined. As shown in Fig. 3c, these re-dissolved micelles were monodisperse (PDI = 0.098 ± 0.013) with mean particle size of 28.1 ± 1.1 nm. In Fig. 3d, the TEM image of re-dissolved micelles indicated that these micelles were still spherical and monodisperse with a mean diameter of ∼23 nm. Thus, the freeze-dried Cur/MPEG-PCL micelle powder has good re-solubility.
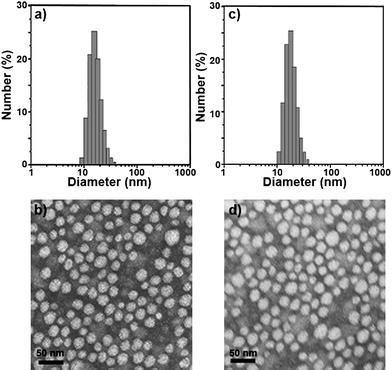 |
| Fig. 3 Particle size distribution and morphology of Cur/MPEG-PCL micelles. (a) and (b) are the particle size distribution spectrum and TEM image of freshly prepared Cur/MPEG-PCL micelles, respectively; (c) and (d) are the particle size distribution spectrum and TEM image of re-dissolved Cur/MPEG-PCL micelles after freeze-drying, respectively. | |
To examine the crystallinity of micelle-encapsulated curcumin, XRD analysis and DSC analysis were performed on the Cur/MPEG-PCL micelles. The XRD spectra are presented in Fig. 4a. In these spectra, the characteristic X-ray diffraction peaks of curcumin dispersed in the XRD spectrum of Cur/MPEG-PCL micelles; this implies that there are no curcumin crystals in the Cur/MPEG-PCL micelles. In Fig. 4b, the DSC curves are shown. No melting transition peaks of curcumin are present, also confirming that there are no curcumin crystals. Thus, Fig. 4 suggests that curcumin was molecularly incorporated in MPEG-PCL micelles.
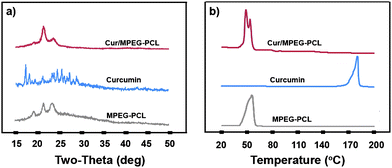 |
| Fig. 4 Confirmation of absence of curcumin crystals in the Cur/MPEG-PCL micelles. (a) XRD analysis of Cur/MPEG-PCL micelles, curcumin, and MPEG-PCL micelles; (b) DSC analysis of Cur/MPEG-PCL micelles, curcumin, and MPEG-PCL micelles. | |
In an attempt to pinpoint the location of curcumin in the MPEG-PCL micelles, we simulated the conformation of MPEG and PCL, and evaluated the bind affinity between curcumin and PEG or PCL. The simulated structures of MPEG and PCL are shown in Fig. 5a and Fig. 5b. According to the docking study, the binding affinity for PCL and curcumin was −17.99 kJ mol−1, while the value for PEG and curcumin was −12.97 kJ mol−1; this indicates that the bind affinity between PCL and curcumin is stronger than that between PEG and curcumin. This suggests that the curcumin is mainly located in the PCL core of the MPEG-PCL micelles. Moreover, the suggested conformation of complex composed of PCL and curcumin was obtained using AutoDock Vina, and result is presented in Fig. 5c. We found that curcumin could not be solubilized in PEG aqueous solution (data not shown), also implying that curcumin is mainly located in the PCL core of MPEG-PCL micelles, rather than the PEG shell.
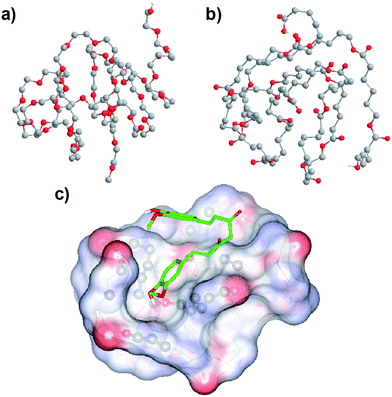 |
| Fig. 5 Simulated conformation of PEG (a), PCL (b) and the PCL–curcumin complex (c); see Section 2.4 for details. The conformation of the PCL–curcumin complex was obtained by a docking study using AutoDock Vina. Atom coloring: grey, carbon; red, oxygen; white, hydrogen. PCL is represented by lines, while curcumin is represented by sticks, and atoms of PCL interacting with curcumin are shown using balls. Non-polar hydrogen atoms are hidden for the sake of clarity. | |
One of the major purposes of the encapsulation of curcumin in MPEG-PCL micelles is making curcumin completely dispersible in aqueous media. The appearance of Cur/MPEG-PCL micelles aqueous solution is shown in Fig. 6. Curcumin cannot be dissolved in pure water (Fig. 6a) or 5% PEG solution (Fig. 6b), as confirmed by the observation of a turbid yellow slurry. In contrast, Cur/MPEG-PCL micelle solution with an equivalent quantity of curcumin was transparent (Fig. 6c), indicating full dispersiblity in water. Freeze-dried Cur/MPEG-PCL micelles (Fig. 6d) were also fully dispersible in water (Fig. 6e). Thus, it is clear that encapsulation of curcumin in MPEG-PCL micelles renders curcumin completely dispersible in aqueous media, making curcumin intravenously injectable.
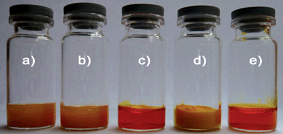 |
| Fig. 6 The encapsulation of curcumin in MPEG-PCL micelles renders curcumin completely dispersible in aqueous media. a): curcumin in water, b): curcumin in 5% PEG solution, c): Cur/MPEG-PCL micelles in water, d) freeze-dried powder of Cur/MPEG-PCL micelles, and e) re-dissolved Cur/MPEG-PCL micelles after freeze-drying. | |
3.2 Release profile in vitro and pharmacokinetics analysis in vivo
To confirm whether MPEG-PCL micelle-encapsulated curcumin can be released, the release profile of curcumin from Cur/MPEG-PCL micelles was studied using a dialysis method in vitro. Results indicate that Cur/MPEG-PCL micelles can slow-release curcuminin vitro (Fig. 7a, about 54.6% of total curcumin was released in 9 days).
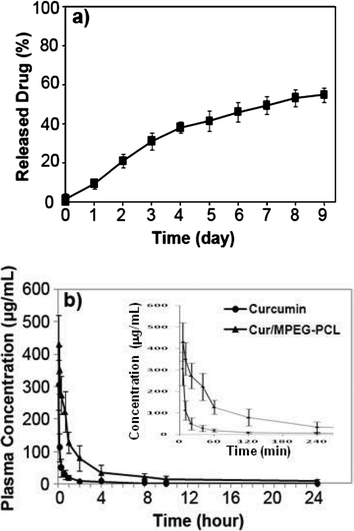 |
| Fig. 7
In vitro release study (a) and in vivo pharmacokinetics assays (b) of Cur/MPEG-PCL micelles. The in vitro release profile of Cur/MPEG-PCL micelles was examined using a dialysis method; see Sections 2.5 and 2.6 for details. | |
Another reason for preparing Cur/MPEG-PCL micelles was to improve the pharmacokinetics of curcuminin vivo. For this, rats were intravenously administered with curcumin or Cur/MPEG-PCL micelles (curcumin: 100 mg kg−1). Blood was collected at different time intervals. For the MPEG-PCL micelle-encapsulated curcumin, the tmax, t1/2, AUC(0→t), AUC(0→∞) and Cmax was 5 min, 34.2 min, 47642.1 μg L−1 min−1, 47864.6 μg L−1 min−1 and 430.5 μg mL−1, respectively. For the free curcumin, the tmax, t1/2, AUC(0→t), AUC(0→∞) and Cmax were 5 min, 19.6 min, 7933.2 μg L−1 min−1, 7944.6 μg L−1 min−1 and 305.7 μg mL−1, respectively. Thus, it was suggested that encapsulation of curcumin in MPEG-PCL micelles improved the t1/2, AUC(0→t), AUC(0→∞) and Cmax of curcuminin vivo.
3.3
Inhibition of angiogenesis
Angiogenesis is necessary for tumor growth, making inhibition of vessel formation an excellent target for cancer therapy. The antiangiogenesis effect of Cur/MPEG-PCL micelles was evaluated using a transgenic zebrafish model and our alginate-encapsulated cancer cells. For the zebrafish model, transgenic Tg(flk: EGFP) zebrafish embryos were treated with free curcumin (5 μg mL−1) or Cur/MPEG-PCL micelles (5 μg mL−1) for 72 h. To assess the functional integrity of the vasculature of each embryo, microangiography analysis was performed on embryos. In Fig. 8, either free curcumin (Fig. 8b) or Cur/MPEG-PCL micelles (Fig. 8c) treated embryos showed defective vascular formation in varying extents of severity; these intersegmental vessels either sprouted abnormally or failed to form (arrows) compared with that of control embryo (Fig. 8a). This suggests that both free curcumin and Cur/MPEG-PCL micelles can inhibit the angiogenesis on zebrafish model.
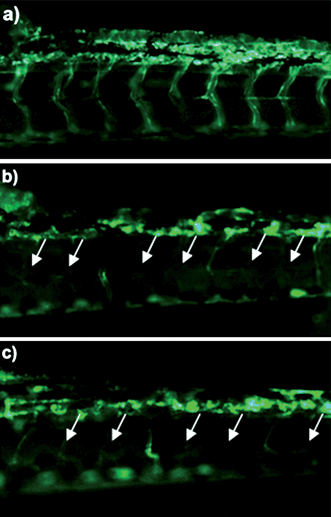 |
| Fig. 8 Antiangiogenesis effect of Cur/MPEG-PCL micelles on a zebrafish model. Zebrafish embryos were treated with Holtfreter's solution (control), free curcumin (5 μg mL−1) or Cur/MPEG-PCL micelles (5 μg mL−1) for 72 h. Blood vessels were examined using a fluorescence microscope. The control embryo (a) shows a regular spacing of intersegmental vessels. Embryos treated with curcumin (b) or Cur/MPEG-PCL micelles (c) showed defective vascular formation in varying extents of severity: either they sprouted abnormally or failed to form (arrows). | |
Moreover, the inhibition of angiogenesis caused by Cur/MPEG-PCL micelles was confirmed in alginate-encapsulated tumor cells assay. In Fig. 9a–d, alginate implant angiogenesis can be directly observed. The vascularization of bead with Cur/MPEG-PCL micelles treatment was clearly suppressed compared with that of other treatments. The alginate implant angiogenesis also was quantified by measuring the uptake of FITC-dextran into beads. FITC-dextran uptake was significantly lower in mice treated with Cur/MPEG-PCL micelles compared with control groups (Fig. 9e). Meanwhile, MPEG-PCL micelle-encapsulated curcumin more efficiently induced the inhibition of angiogenesis than free curcumin; this result may be caused by the improved pharmacokinetics of Cur/MPEG-PCL micelles.
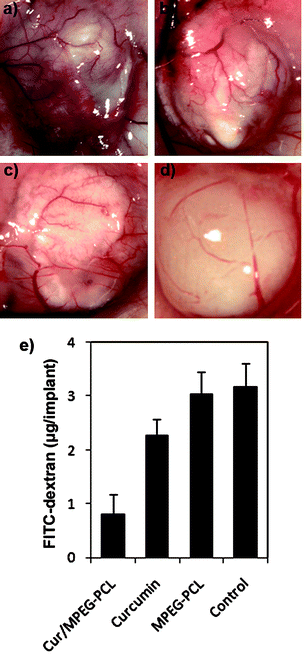 |
| Fig. 9 Vasculation of alginate implants. Alginate beads containing colon carcinoma cells were implanted subcutaneously into the mice on day 0. Then, these mice were intravenously administered with normal saline, MPEG-PCL micelles, free curcumin, or Cur/MPEG-PCL micelles once a day for 5 days. On day 12, beads were surgically removed, and FITC-dextran was quantified. Photographs of alginate implants (a: control, b: MPEG-PCL, c: free curcumin, and d: Cur/MPEG-PCL) and FITC-dextran uptake (e) showed the reduction of vascularization in implants of mice treated with Cur/MPEG-PCL micelles. | |
3.4 Anticancer activity in vitro and in vivo
The anticancer activity of Cur/MPEG-PCL micelles was determined in vitro and in vivo. The cytotoxicity of Cur/MPEG-PCL micelles to C-26 colon carcinoma cells was studied in vitro; results are presented in Fig. 10a. Both curcumin and Cur/MPEG-PCL micelles efficiently killed C-26 colon carcinoma cells. The IC50 for curcumin was 3.95 μg mL−1, and that for Cur/MPEG-PCL micelles was 5.78 μg mL−1. Compared to free curcumin, Cur/MPEG-PCL micelles had slightly lower cytotoxicity, which may be due to the slow release of curcumin.58
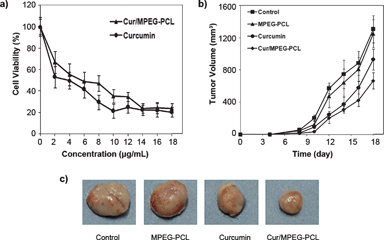 |
| Fig. 10 Anticancer effect of Cur/MPEG-PCL micelles in vitro and in vivo. (a): Cytotoxicity of curcumin or Cur/MPEG-PCL micelles on C-26 cellsin vitro after exposure for 48 h. (b): Tumor development curve. Female mice were inoculated with C-26 cells on day 0. On day 4, the mice were randomized into four groups, and were injected intravenously with saline (control), empty MPEG-PCL micelles, free curcumin or Cur/MPEG-PCL micelles daily for ten days. (c) Representative photos of tumors in each treatment group on day 18. | |
The ability of Cur/MPEG-PCL micelles to inhibit the growth of C-26 colon carcinoma in vivo was evaluated on a mouse model. Four groups of mice bearing C-26 colon carcinoma were intravenously administered with normal saline, MPEG-PCL micelles (168 mg kg−1), free curcumin (25 mg kg−1) and Cur/MPEG-PCL micelles (curcumin: 25 mg kg−1), respectively. The tumor growth curves of each group are presented in Fig. 10b. Results indicated that Cur/MPEG-PCL micelles treatment resulted in smaller tumor volume compared to others treatment (p < 0.01, vs. control; p < 0.01, vs. MPEG-PCL; p < 0.05, vs.curcumin). A representative tumor in each group is presented in Fig. 10c. We can clearly observe that the tumor in the group treated with Cur/MPEG-PCL micelles is smaller than that of the other groups. This in vivo study indicates that: 1) systemic application of Cur/MPEG-PCL micelles (25 mg kg−1) can inhibit the growth of subcutaneous C-26 colon carcinoma in vivo; and 2) encapsulation of curcumin in MPEG-PCL micelles enhances the anticancer activity of curcuminin vivo.
4. Discussion
Curcumin is recognized as an efficient and safe anticancer agent,13–32 but it is water-insoluble, and this limits its application in oral administration. Oral administration of curcumin results in very low bioavailability of curcuminin vivo; thus developing intravenously injectable aqueous formulation for curcumin is interesting. Biodegradable nano drug carriers have promising application in solving the issue of poor water solubility of hydrophobic drugs. In this paper, we used MPEG-PCL micelles to deliver curcumin, developing an intravenously injectable aqueous formulation for curcumin; moreover, we characterized this formulation in detail and evaluated its antiangiogenesis and anticancer effect in vitro and in vivo.
MPEG-PCL micelles have potential application in drug delivery systems, and are already used to deliver some drugs.49,52 In this protocol, curcumin was encapsulated into MPEG-PCL micelles by a single-step nano-precipitation method, creating Cur/MPEG-PCL micelles. In this nano-precipitation process, MPEG-PCL copolymer self-assembled into core-shell structured MPEG-PCL micelles with encapsulated curcumin (Fig. 2). The XRD and DSC analyses indicated that the encapsulated curcumin was amorphous (Fig. 4); meanwhile, both computer simulation and experiments implied that the curcumin was mainly located in the PCL core of MPEG-PCL micelles (Fig. 5). This procedure (nano-precipitation method) of preparing Cur/MPEG-PCL was simple, and easy to scale up. The obtained Cur/MPEG-PCL micelles were monodisperse (PDI = 0.097 ± 0.011) with a mean particle size of 27.3 ± 1.3 nm (Fig. 3); and had encapsulation efficiency of 99.16 ± 1.02% and a drug loading of 12.95 ± 0.15% (Table 1). Confirmed by the appearance of Cur/MPEG-PCL micelles aqueous solution, encapsulation of curcumin in MPEG-PCL micelles rendered curcumin completely dispersible in water (Fig. 6).
In previous reports, some polymeric nano-vectors were used to deliver curcumin. Bisht et al. synthesized a polymeric nanoparticle encapsulated formulation of curcumin utilizing micellar aggregates of cross-linked and random copolymers of N-isopropylacrylamide (NIPAAM), with N-vinyl-2-pyrrolidone (VP) and poly(ethyleneglycol) monoacrylate (PEG-A). These nanoparticles had a narrow size distribution in the 50 nm range.59 Sou et al. used lipid-based nanoparticles to deliver curcumin; nanoparticles with a mean particle size of ∼187 nm improved intravenous delivery of curcumin to tissue macrophages, specifically bone marrow and splenic macrophages.60 Shaikh et al. prepared curcumin-loaded PLGA nanoparticles with a particle size of 264 nm (polydispersity index 0.31), an encapsulation efficiency of 76.9% and drug loading of 10.2%. These nanoparticles improved oral bioavailability of curcumin at least 9-fold when compared to curcumin administered with piperine as absorption enhancer.61 Aneand et al. used PLGA-PEG nanoparticles to deliver curcumin. These curcumin-loaded nanoparticles (∼80.9 nm) had encapsulation efficiency of 97.5%, but had low drug loading (4 μg curcumin per mg of nanoparticles). They found that encapsulation of curcumin in PLGA-PEG nanoparticles enhanced cellular uptake, increased bioactivity in vitro and improved bioavailability in vivo.62 Recently, Letchford et al. suggested that drug solubilization in MPEG-PCL micelles is directly related to the compatibility between the solubilizate and PCL; and found that MPEG-PCL micelles had potential applications in delivering curcumin.63 Subsequently, Mohanty et al. prepared curcumin encapsulated within MPEG-PCL micelles and evaluated their application in cancer therapy in vitro. These micelles had a mean particle size of ∼110 nm, an encapsulation efficiency of ∼57%, and a drug loading of ∼5.5%.64
Recently, we developed a direct dissolution method to encapsulate honokiol into PCL/PEG micelles, without using an organic solvent.52 When the direct dissolution method was used to prepare Cur/MPEG-PCL micelles, the obtained micelles have low drug loading (∼3.5%) and large particle size (∼47 nm). Therefore, the direct dissolution method may be not suitable for preparing Cur/MPEG-PCL micelles. Alternatively, Chin et al. used superparamagnetic silica reservoirs to encapsulate curcumin. The obtained nanoparticles, with high magnetic mobility have drug loading of ∼30% and a particle size of 200–1000 nm.65 Compared to previously reported nanoparticle-encapsulated curcumin, our Cur/MPEG-PCL micelles still hadve some advantages, such as small size (27.3 ± 1.3 nm), narrow size distribution (PDI = 0.097 ± 0.011), high encapsulation efficiency (99.16 ± 1.02%), high drug loading (12.95 ± 0.15%), simple preparation method, and good re-solubility. Thus, Cur/MPEG-PCL micelles may have potential as an intravenous injectable aqueous formulation of curcumin.
An in vitro release study indicated that Cur/MPEG-PCL micelles could release curcumin slowly (Fig. 7a). Moreover, an in vivo study indicated that encapsulation of curcumin in MPEG-PCL micelles improved the t1/2 and AUC of curcumin (Fig. 7b). The small size and hydrophilic PEG shell may allow Cur/MPEG-PCL micelles to circulate for a long time in vivo after systemic application. The long circulation time of MPEG-PCL micelles and the slow release of curcuminin vivo may contribute to the improved t1/2 and AUC of curcumin.
The zebrafish is an accepted model for studies of vertebrate developmental biology. The vascular system has been well described and shown to be highly conserved in the zebrafish. Many zebrafish blood vessels form by angiogenic sprouting, and appear to require the same proteins that are necessary for blood vessel growth in mammals. Currently, zebrafish (as an animal model) is widely used for screening small molecules that affect blood vessel formation.66 The antiangiogenesis effect of curcumin has already been estimated.16,19,27,41,66,67 In our experiments, MPEG-PCL micelle-encapsulated curcumin (as well as free curcumin) inhibited angiogenesis in an zebrafish model (Fig. 8), whereas empty MPEG-PCL micelles had no ability to inhibit angiogenesis (this also was proved for a zebrafish model in our experiments; data not shown). thus, the antiangiogenesis effect of Cur/MPEG-PCL micelles is caused by the encapsulated curcumin. To examine the effect of Cur/MPEG-PCL micelles on tumor angiogenesisin vivo, we used alginate implants encapsulating tumor cells (a remarkable approach to a specific tumor angiogenesis model in vivo); results indicated that i.v. administration of Cur/MPEG-PCL micelles more efficiently inhibited the tumor angiogenesis than that of free curcumin; this may due to the improved t1/2 and AUC of MPEG-PCL micelle-encapsulated curcuminin vivo. Thus, i.v. application of Cur/MPEG-PCL micelles has potential in inhibiting tumor angiogenesis.
An in vitro cytotoxicity study indicated that both free curcumin and Cur/MPEG-PCL micelles showed clear cytotoxicity to C-26 colon carcinoma cells. The IC50 of free curcumin and Cur/MPEG-PCL micelles was 3.95 μg mL−1 and 5.78 μg mL−1, respectively. An in vivo study indicated that systemic application of Cur/MPEG-PCL micelles inhibited the growth of subcutaneous C-26 colon carcinoma (p < 0.01). Moreover, Cur/MPEG-PCL micelles induced a stronger anticancer effect than free curcumin (p < 0.05); this implies that encapsulation of curcumin in MPEG-PCL micelles enhanced the anticancer activity of curcuminin vivo. Curcumin has cytotoxicity to cancer cells, thus it can directly kill cancer cellsin vivo; meanwhile, curcumin can inhibit angiogenesis, also resulting in suppression of tumor growth. Therefore, the anticancer effect of Cur/MPEG-PCL micelles may be induced by inhibiting angiogenesis and directly killing cancer cells.13–24,66,67
Alternatively, angiogenic blood vessels in tumor tissues have gaps between adjacent endothelial cells. This defective vascular architecture coupled with poor lymphatic drainage induces enhanced permeability and retention (EPR) effect, which allows nanoparticles to extravasate through these gaps into extravascular spaces and accumulate inside tumor tissues.49 For such a passive targeting mechanism (EPR effect) to work, these nanoparticles must circulate for a long time in vivo. Monodisperse Cur/MPEG-PCL micelles were small enough (<30 nm) for this, while our Cur/MPEG-PCL micelles have a core-shell structure, in which hydrophilic PEG acts as a brush-like protective coating. Encapsulation of curcumin in MPEG-PCL micelles improved the t1/2 and AUC of curcuminin vivo, which may contribute to enhancing the accumulation of curcumin in tumor site due to the EPR effect, thus improving the anticancer activities of curcumin. To our knowledge, this work is the first report concerning the application of polymeric micelle-encapsulated curcumin in colon cancer therapy in vivo. These results suggest that i.v. application of Cur/MPEG-PCL micelles may have potential application in treating colon carcinoma.
5. Conclusions
Curcumin-loaded MPEG-PCL (Cur/MPEG-PCL) micelles were prepared by a nano-precipitation method. Encapsulation of curcumin in MPEG-PCL micelles rendered curcumin completely dispersible in water, thus overcoming the poor water solubility of curcumin. Compared to previously reported nano-curcumin formulations, our Cur/MPEG-PCL micelles have advantages such as small size, narrow size distribution, high encapsulation efficiency, high drug loading, simple preparation method, and good re-solubility. Cur/MPEG-PCL micelles inhibited the growth of C-26 colon carcinoma through inhibiting angiogenesis and directly killing cancer cells. Encapsulation of curcumin in MPEG-PCL micelles improved the pharmacokinetics, antiangiogenesis effect and anticancer effect of curcuminin vivo. Therefore, Cur/MPEG-PCL micelles are an excellent intravenously injectable aqueous formulation of curcumin – one that has potential clinical application in colon cancer therapy, and warrants further evaluation of efficacy and safety in early-phase clinical trials.
Acknowledgements
This work was financially supported by the New Century Excellent Talents in University (NCET-08-0371), the Specialized Research Fund for the Doctoral Program of Higher Education (SRFDP 200806100065), the National Natural Science Foundation (NSFC20704027, NSFC30801294), the Chinese Key Basic Research Program (2010CB529906), and Huoyingdong Youth Fund from the Ministry of Education, P.R. China (No.122030). We would like to show our great thanks to Dr Shuo Lin (UCLA, USA) for providing the Tg (flk:EGFP) transgenic zebrafish.
References
- M. S. Butler, The role of natural product chemistry in drug discovery, J. Nat. Prod., 2004, 67, 2141–53 CrossRef CAS.
- D. J. Newman and G. M. Cragg, Natural products as sources of new drugs over the last 25 years, J. Nat. Prod., 2007, 70, 461–77 CrossRef CAS.
- M. J. Balunas and A. D. Kinghorn, Drug discovery from medicinal plants, Life Sci., 2005, 78, 431–41 CrossRef CAS.
- A. Gurib-Fakim, Medicinal plants: traditions of yesterday and drugs of tomorrow, Mol. Aspects Med., 2006, 27, 1–93 CrossRef CAS.
- M. E. Egan, M. Pearson, S. A. Weiner, V. Rajendran, D. Rubin and J. Glöckner-Pagel,
et al. Curcumin, a major constituent of turmeric, corrects cystic fibrosis defects, Science, 2004, 304, 600–2 CrossRef CAS.
- A. Goel, A. B. Kunnumakkara and B. B. Aggarwal, Curcumin as “Curecumin”: from kitchen to clinic, Biochem. Pharmacol., 2008, 75, 787–809 CrossRef CAS.
- G. C. Jagetia and B. B. Aggarwal, “Spicing up” of the immune system by curcumin, J. Clin. Immunol., 2007, 27, 19–35 CrossRef CAS.
- R. K. Maheshwari, A. K. Singh, J. Gaddipati and R. C. Srimal, Multiple biological activities of curcumin: A short review, Life Sci., 2006, 78, 2081–7 CrossRef CAS.
- B. B. Aggarwal, A. Kumar and A. C. Bharti, Anticancer potential of curcumin: preclinical and clinical studies, Anticancer. Res., 2003, 23, 363–98 CAS.
- C. L. Lin and J. K. Lin, Curcumin: a potential cancer chemopreventive agent through suppressing NF-κB signaling, J. Cancer Mol., 2008, 4, 11–6 Search PubMed.
- W. P. Steward and A. J. Gescher, Curcumin in cancer management: recent results of analogue design and clinical studies and desirable future research, Mol. Nutr. Food Res., 2008, 52, 1005–9 CrossRef CAS.
- I. Villegas, S. Sanchez-Fidalgo and C. Alarcón de la Lastra, New mechanisms and therapeutic potential of curcumin for colorectal cancer, Mol. Nutr. Food Res., 2008, 52, 1040–61 CrossRef CAS.
- J. K. Lin and S. Y. Lin-Shiau, Mechanisms of cancer chemoprevention by curcumin, Proc. Natl. Sci. Counc. ROC (B), 2001, 25, 59–66 Search PubMed.
- C. V. Rao, A. Rivenson, B. Simi and B. S. Reddy, Chemoprevention of colon carcinogenesis by dietary curcumin, a naturally occurring plant phenolic compound, Cancer Res., 1995, 55, 259–66 CAS.
- T. Kawamori, R. Lubet, V. E. Steele, G. J. Kelloff, R. B. Kaskey and C. V. Rao,
et al. Chemopreventive effect of curcumin, a naturally occurring anti-inflammatory agent, during the promotion/progression stages of colon cancer, Cancer Res., 1999, 59, 597–601 CAS.
- T. Dorai, Y. C. Cao, B. Dorai, R. Buttyan and A. E. Katz, Therapeutic potential of curcumin in human prostate cancer. III. Curcumin inhibits proliferation, induces apoptosis, and inhibits angiogenesis of LNCaP prostate cancer cells in vivo, Prostate, 2001, 47, 293–303 CrossRef CAS.
- A. Mukhopadhyay, C. Bueso-Ramos, D. Chatterjee, P. Pantazis and B. B. Aggarwal, Curcumin downregulates cell survival mechanisms in human prostate cancer cell lines, Oncogene, 2001, 20, 7597–609 CrossRef CAS.
- T. Choudhuri, S. Pal, M. L. Agwarwal, T. Das and G. Sa, Curcumin induces apoptosis in human breast cancer cells through p53-dependent Bax induction, FEBS Lett., 2002, 512, 334–40 CrossRef CAS.
- L. Li, F. S. Braiteh and R. Kurzrock, Liposome-encapsulated curcumin in vitro and in vivo effects on proliferation, apoptosis, signaling, and angiogenesis, Cancer, 2005, 104, 1322–31 CrossRef CAS.
- A. C. Bharti, N. Donato, S. Singh and B. B. Aggarwal, Curcumin (diferuloylmethane) down-regulates the constitutive activation of nuclear factor-κB and IκBα kinase in human multiple myeloma cells, leading to suppression of proliferation and induction of apoptosis, Blood, 2003, 101, 1053–62 CrossRef CAS.
- B. Tian, Z. Wang, Y. Zhao, D. Wang, Y. Li and L. Ma,
et al. Effects of curcumin on bladder cancer cells and development of urothelial tumors in a rat bladder carcinogenesis model, Cancer Lett., 2008, 264, 299–308 CrossRef CAS.
- M. Shi, Q. Cai, L. Yao, Y. Mao, Y. Ming and G. Ouyang, Antiproliferation and apoptosis induced by curcumin in human ovarian cancer cells, Cell Biol. Int., 2006, 30, 221–6 CrossRef CAS.
- P. C. Everett, J. A. Meyers, A. Makkinje, M. Rabbi and A. Lerner, Preclinical assessment of curcumin as a potential therapy for B-CLL, Am. J. Hematol., 2007, 82, 23–30 CrossRef CAS.
- M. López-Lázaro, E. Willmore, A. Jobson, K. L. Gilroy, H. Curtis and K. Padget,
et al. Curcumin induces high levels of topoisomerase I- and II-DNA complexes in K562 leukemia cells, J. Nat. Prod., 2007, 70, 1884–8 CrossRef CAS.
- W. Chearwae, S. Anuchapreeda, K. Nandigama, S. V. Ambudkar and P. Limtrakul, Biochemical mechanism of modulation of human P-glycoprotein (ABCB1) by curcumin I, II, and III purified from turmeric powder, Biochem. Pharmacol., 2004, 68, 2043–52 CrossRef CAS.
- S. Lev-Ari, L. Strier, D. Kazanov, L. Madar-Shapiro, H. Dvory-Sobol and I. Pinchuk,
et al. Celecoxib and curcumin synergistically inhibit the growth of colorectal cancer cells, Clin. Cancer Res., 2005, 11, 6738–44 CrossRef CAS.
- A. B. Kunnumakkara, S. Guha, S. Krishnan, P. Diagaradjane, J. Gelovani and B. B. Aggarwal, Curcumin potentiates antitumor activity of gemcitabine in an orthotopic model of pancreatic cancer through suppression of proliferation, angiogenesis, and inhibition of nuclear factor-κB-regulated gene products, Cancer Res., 2007, 67, 3853–61 CrossRef CAS.
- S. HemaIswarya and M. Doble, Potential Synergism of Natural Products in the Treatment of Cancer, Phytother. Res., 2006, 20, 239–49 CrossRef CAS.
- T. O. Khor, Y. S. Keum, W. Lin, J. H. Kim, R. Hu and G. Shen,
et al. Combined inhibitory effects of curcumin and phenethyl isothiocyanate on the growth of human PC-3 prostate xenografts in immunodeficient mice, Cancer Res., 2006, 66, 613–21 CrossRef CAS.
- C. D. Lao, I. V. M. T. Ruffin, D. Normolle, D. D. Heath, S. I. Murray and J. M. Bailey,
et al. Dose escalation of a curcuminoid formulation, BMC Complementary Altern. Med., 2006, 6, 10 Search PubMed.
- A. L. Cheng, C. H. Hsu, J. K. Lin, M. M. Hsu, Y. F. Ho and T. S. Shen,
et al. Phase I clinical trial of curcumin, a chemopreventive agent, in patients with high-risk or pre-malignant lesions, Anticancer Res., 2001, 21, 2895–900 CAS.
- N. Dhillon, B. B. Aggarwal, R. A. Newman, R. A. Wolff, A. B. Kunnumakkara and J. L. Abbruzzese,
et al. Phase II trial of curcumin in patients with advanced pancreatic cancer, Clin. Cancer Res., 2008, 14, 4491–9 CrossRef CAS.
- R. A. Sharma, S. A. Euden, S. L. Platton, D. N. Cooke, A. Shafayat and H. R. Hewitt,
et al. Phase I clinical trial of oral curcumin: biomarkers of systemic activity and compliance, Clin. Cancer Res., 2004, 10, 6847–54 CrossRef CAS.
- R. A. Sharma, H. R. McLelland, K. A. Hill, C. R. Ireson, S. A. Euden and M. M. Manson,
et al. Pharmacodynamic and pharmacokinetic study of oral curcuma extract in patients with colorectal cancer, Clin. Cancer Res., 2001, 7, 1894–900 CAS.
- R. A. Sharma, C. R. Ireson, R. D. Verschoyle, K. A. Hill, M. L. Williams and C. Leuratti,
et al. Effects of dietary curcumin on glutathione S-transferase and malondialdehyde-DNA adducts in rat liver and colon mucosa: Relationship with drug levels, Clin. Cancer Res., 2001, 7, 1452–8 CAS.
- G. Shoba, D. Joy, T. Joseph, M. Majeed, R. Rajendran and P. S. S. R. Srinivas, Influence of piperine on the pharmacokinetics of curcumin in animals and human volunteers, Planta Med., 1998, 64, 353–6 CrossRef CAS.
- P. Anand, A. B. Kunnumakkara, R. A. Newman and B. B. Aggarwal, Bioavailability of Curcumin: Problems and Promises, Mol. Pharmaceutics, 2007, 4, 807–8 CrossRef CAS.
- W. Shi, S. Dolai, S. Rizk, A. Hussain, H. Tariq and S. Averick,
et al. Synthesis of monofunctional curcumin derivatives, clicked curcumin dimer, and a PAMAM dendrimer curcumin conjugate for therapeutic applications, Org. Lett., 2007, 9, 5461–4 CrossRef CAS.
- A. Safavy, K. P. Raisch, S. Mantena, L. L. Sanford, S. W. Sham and N. R. Krishna,
et al. Design and development of water-soluble curcumin conjugates as potential anticancer agents, J. Med. Chem., 2007, 50, 6284–8 CrossRef CAS.
- M. A. Tomren, M. Másson, T. Loftsson and H. Hjorth Tønnesen, Studies on curcumin and curcuminoids XXXI. Symmetric and asymmetric curcuminoids: stability, activity and complexation with cyclodextrin, Int. J. Pharm., 2007, 338, 27–34 CrossRef CAS.
- L. Li, B. Ahmed, K. Mehta and R. Kurzrock, Liposomal curcumin with and without oxaliplatin: effects on cell growth, apoptosis, and angiogenesis in colorectal cancer, Mol. Cancer Ther., 2007, 6, 1276–82 CrossRef CAS.
- C. C. Lin, H. Y. Lin, H. C. Chen, M. W. Yu and M. H. Lee, Stability and characterisation of phospholipid-based curcumin-encapsulated microemulsions, Food Chem., 2009, 116, 923–8 CrossRef CAS.
- L. Zhang, J. M. Chan, F. X. Gu, J. W. Rhee, A. Z. Wang and A. F. Radovic-Moreno,
et al. Self-Assembled Lipid – Polymer Hybrid Nanoparticles: A Robust Drug Delivery Platform, ACS Nano, 2008, 2, 1696–1702 CrossRef CAS.
- T. G. Shutava, S. S. Balkundi, P. Vangala, J. J. Steffan, R. L. Bigelow and J. A. Cardelli,
et al. Layer-by-Layer-Coated Gelatin Nanoparticles as a Vehicle for Delivery of Natural Polyphenols, ACS Nano, 2009, 3, 1877–1885 CrossRef CAS.
- Y. A. Shieh, S. J. Yang, M. F. Wei and M. J. Shieh, Aptamer-Based Tumor-Targeted Drug Delivery for Photodynamic Therapy, ACS Nano, 2010, 4, 1433–42 CrossRef CAS.
- M.L. Gou, K. Men, J. Zhang, Y. H. Li, J. Song and S. Luo,
et al. Biodegradable Heparin Conjugated Polyethylenimine Nano-gel Delivering VSVMP Gene for C-26 Colon Carcinoma Therapy, ACS Nano, 2010, 4, 5573–84 CrossRef CAS.
- T. Wang, L. Xu, S. Li and N. He, Low Molecular Weight Alginate-Oligochitosan Nanocapsules: Preparation, Characterization and Applications, Nanoscale, 2010, 2, 230–9 RSC.
- N. He, T. Wang, L. Jiang, D. Wang, Y. Hu and L. Zhang, Therapy for Cerebral Ischemic Injury with Erythropoietin-containing Nanopariticles, J. Nanosci. Nanotechnol., 2010, 10, 5320–3 CrossRef CAS.
- X. Wang, L. Yang, Z. Chen and D. M. Shin, Application of nanotechnology in cancer therapy and imaging, CA Cancer J. Clin., 2008, 58, 97–110 Search PubMed.
- X. W. Wei, C. Y. Gong, M. L. Gou, S. Z. Fu, Q. F. Guo and S. Shi,
et al. Biodegradable poly(ε-caprolactone)-poly(ethylene glycol) copolymers as drug delivery system, Int. J. Pharm., 2009, 381, 1–18 CrossRef CAS.
- M. L. Gou, X. W. Wei, K. Men, B. L. Wang, F. Luo and X. Zhao,
et al. PCL/PEG copolymeric nanoparticles: potential nanoplatforms for anticancer agent delivery, Curr. Drug Targets, 2010 Search PubMed , in press.
- M. L. Gou, X. L. Zheng, K. Men, J. Zhang, B. L. Wang and L. Lv,
et al. Self-assembled hydrophobic honokiol loaded MPEG-PCL diblock copolymer micelles, Pharm. Res., 2009, 26, 2164–73 CAS.
-
HyperChem Professional 8.0, Hypercube, Inc., 1115 NW 4th Street, Gainesville, Florida 32601, USA Search PubMed.
- W. L. Jorgensen, D. S. Maxwell and J. Tirado-Rives, Development and testing of the opls all-atom force field on conformational energetics and properties of organic liquids, J. Am. Chem. Soc., 1996, 118, 11225–36 CrossRef CAS.
- O. Trotto and A. J. Olson, AutoDock Vina: improving the speed and accuracy of docking with a new scoring function, efficient optimization, and multithreading, J. Comput. Chem., 2010, 31, 455–61 CAS.
-
M. Westerfield. The zebrafish book, University of Oregon Press, Eugene, 1995 Search PubMed.
- J. Hoffmann, M. Schirner, A. Menrad and M. R. Schneider, A highly sensitive model for quantification of in vivo tumor angiogenesis induced by alginate-encapsulated tumor cells, Cancer Res., 1997, 57, 3847–51 CAS.
- T. Hamaguchi, Y. Matsumura, M. Suzuki, K. Shimizu, R. Goda and I. Nakamura,
et al. NK105, a paclitaxel-incorporating micellar nanoparticle formulation, can extend in vivo antitumour activity and reduce the neurotoxicity of paclitaxel, Br. J. Cancer, 2005, 92, 1240–6 CrossRef CAS.
- S. Bisht, G. Feldmann, S. Soni, R. Ravi, C. Karikar and A. Maitra,
et al. Polymeric nanoparticle-encapsulated curcumin (“nanocurcumin”): a novel strategy for human cancer therapy, J. Nanobiotechnol., 2007, 5, 3 CrossRef.
- K. Sou, S. Inenaga, S. Takeoka and E. Tsuchida, Loading of curcumin into macrophages using lipid-based nanoparticles, Int. J. Pharm., 2008, 352, 287–93 CrossRef CAS.
- J. Shaikh, D. D. Ankola, V. Beniwal, D. Singh and M. N. V. Ravi Kumar, Nanoparticle encapsulation improves oral bioavailability of curcumin by at least 9-fold when compared to curcumin administered with piperine as absorption enhancer, Eur. J. Pharm. Sci., 2009, 37, 223–30 CrossRef CAS.
- P. Anand, H. B. Nair, B. Sung, A. B. Kunnumakkara, V. R. Yadav and R. R. Tekmal,
et al. Design of curcumin-loaded PLGA nanoparticles formulation with enhanced cellular uptake, and increased bioactivity in vitro and superior bioavailability in vivo, Biochem. Pharmacol., 2010, 79, 330–8 CrossRef CAS.
- K. Letchford, R. Liggins and H. Burt, Solubilization of hydrophobic drugs by methoxy poly(ethylene glycol)-block-polycaprolactone diblock copolymer micelles: theoretical and experimental data and correlations, J. Pharm. Sci., 2008, 97, 1179–90 CrossRef CAS.
- C. Mohanty, S. Acharya, A. K. Mohanty, F. Dilnawaz and S. K. Sahoo, Curcumin-encapsulated MePEG/PCL diblock copolymeric micelles: a novel controlled delivery vehicle for cancer therapy, Nanomedicine, 2010, 5, 433–49 CrossRef CAS.
- S. F. Chin, K. Swaminathan Iyer, M. Saunders, T. G. St. Pierre, C. Buckley and M. Paskevicius,
et al. Encapsulation and sustained release of curcumin using superparamagnetic silica reservoirs, Chem.–Eur. J., 2009, 15, 5661–5 CrossRef CAS.
- J. L. Arbiser, N. Klauber, R. Rohan, R. van Leeuwen, M. T. Huang and C. Fisher,
et al. Curcumin is an in vivo inhibitor of angiogenesis, Mol. Med., 1998, 4, 376–83 CAS.
- Y. G. Lin, A. B. Kunnumakkara, A. Nair, W. M. Merritt, L. Y. Han and G. N. Armaiz-Pena,
et al. Curcumin inhibits tumor growth and angiogenesis in ovarian carcinoma by targeting the nuclear factor-κB pathway, Clin. Cancer Res., 2007, 13, 3423–30 CrossRef CAS.
|
This journal is © The Royal Society of Chemistry 2011 |
Click here to see how this site uses Cookies. View our privacy policy here.