DOI:
10.1039/C6QO00311G
(Research Article)
Org. Chem. Front., 2016,
3, 1149-1153
Rh-catalysed asymmetric conjugate addition of boronic acids to nitroalkenes employing a P-chiral P,π-hybrid ligand†
Received
28th June 2016
, Accepted 23rd July 2016
First published on 26th July 2016
Abstract
A Rh-catalysed asymmetric conjugate addition of aryl boronic acids to β-substituted nitroalkenes was developed employing a P-chiral P-alkene hybrid bidentate ligand with enantioselectivities of up to 94
:
6 er. DFT modelling of the transition state for the addition reaction was consistent with our previous model for stereocontrol employing this family of chiral ligands. Application of the β-chiral nitroalkanes was demonstrated in the intramolecular Buchwald–Hartwig amination and aminocarbonylation to provide 5- and 6-membered chiral heterocycles.
Introduction
The metal catalysed asymmetric conjugate addition of carbon-based nucleophiles to nitroalkenes is an important reaction for the asymmetric synthesis of β-chiral amines and α-chiral carbonyl compounds.1 In particular, the asymmetric conjugate addition of aryl boronic acids to nitrostyrenes2 allows access to β-chiral benzhydryl amines (3) that can be applied to the synthesis of important biologically relevant compounds3 (Scheme 1). After the pioneering work of Hayashi and Miyaura4 who demonstrated the Rh-catalysed conjugate addition of aryl boronic acids to α,β-unsaturated carbonyl electrophiles enabled by Rh-boron exchange,5 significant research efforts by many groups in this area have expanded the scope of this reaction to include imines6 and α-ketoesters7 as competent electrophiles. Notably, Hayashi8 and Carreira9 first demonstrated that bidentate alkene-based ligands lead to more reactive catalysts for the Rh-catalysed conjugate addition of aryl boronic acids. Since that time, olefin-containing bidentate ligands including dienes, sulphur–alkene, and phosphorous–alkene bidentate ligands have been developed and applied to this class of transformation.10
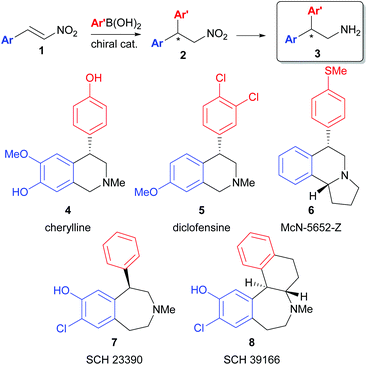 |
| Scheme 1 Synthetic utility of nitroalkene conjugate addition reactions. | |
Recently, we have expanded the scope of the P-chiral dihydrobenzooxaphosphole-based phosphine ligand series developed within Boehringer Ingelheim (Scheme 2) for a wide array of catalytic asymmetric transformations11 to include a new series of P-chiral P-alkene hybrid ligands (JoshPhos)12 that are applicable to the asymmetric Rh-catalysed conjugate addition of aryl boronic acid nucleophiles to enones and imines with high enantioselectivities. Therefore, we reasoned that this system may also be useful in the conjugate addition of arylboronic acids to nitroalkene electrophiles. Herein, we disclose the application of our JoshPhos-based ligands to the asymmetric Rh-catalysed conjugate addition of aryl boronic acids to nitroalkenes.
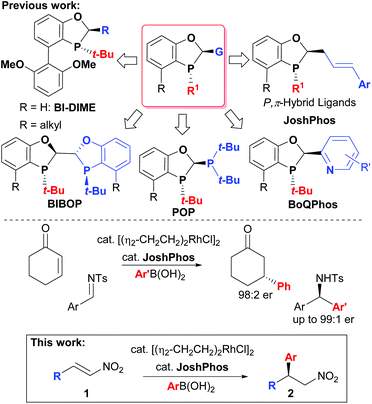 |
| Scheme 2
P-Chiral dihydrobenzooxaphosphole-based ligands. | |
Results and discussion
Initial reaction optimization studies were performed using nitrostyrene (1a) and p-methoxyphenyl boronic acid (Table 1). Use of aqueous KOH to prepare the Rh–OH catalyst in situ at variable reaction temperatures gave poor to modest yields despite the fact that complete consumption of 1a was observed in all cases (entries 1–3). The only impurity that could be isolated and identified was tentatively assigned as 11 that presumably formed from the conjugate addition of the product 10a onto 1a. Although HRMS and 1HNMR spectroscopic analysis was consistent with the proposed assignment, 11 was isolated as an inseparable mixture of what appeared to be four diastereomers, and therefore, conclusive structural assignment was not possible. We next examined KHF2 as an additive given its demonstrated utility in the Rh-catalysed addition of boronic acids to nitroalkenes (entries 4–11).2b,e Use of KHF2 eliminated the formation of 11 resulting in an improved yield, but incomplete conversion was observed (entry 4). Incomplete conversion was partly due to the fact that 60 °C was required for good reaction rates, and protodeboronation of the boronic acid became an issue (i.e. no boronic acid remained after ∼3 h by HPLC analysis). Tuning of the electronics of the JoshPhos ligand did not lead to improved conversions (entries 5 and 6). A solvent screen (entries 7–11) identified THF as the optimal solvent leading to full conversion and good yield with minimal formation of 11. Cyclopentyl methyl ether (CPME) was also identified as a good solvent. Finally, use of the ArBF3K salt in place of boronic acid 9a could also be employed; however, the reaction was significantly slower under these conditions (entries 12 and 13).
Table 1 Optimization of the Rh-catalysed conjugate addition employing 1aa
The substrate scope was next analysed (Scheme 3). In general, electron-rich boronic acids gave the best yields in the conjugate addition reaction (10a–10c, 10h–10j, 10l). Notably, a thioether (10c) and a free phenol (10i) were tolerated well in the chemistry to afford the requisite enantioenriched chiral precursors to cherylline (4)3a and McN-5652-Z (6).3b Halogen containing aryl boronic acids were somewhat problematic due to competitive protodeboronation of the aryl boronic acid (10e, ent-10j). However, this limitation can be circumvented by reversing the polarity of the coupling by incorporating the halogen substitution on the nitroalkene with use of an electron-rich boronic acid as nucleophile (10j). However, in this case, improved yields were realized when CPME was employed, as use of THF led to the formation of significant amount of the impurity analogous to 11. This solvent switch inhibited impurity formation and enabled an excellent yield of 10j which maps onto the core of the anti-depressant diclofensine (5). ortho-Substitution was not tolerated on the arylboronic acid nucleophile, however, ortho-substitution was tolerated on the nitroalkene partner (10g, 10h). Heterocycles could also be employed on the nitroalkene fragment (10k, 10l), as could alkyl substitution (10m, 10n). Lastly, an α,β-disubstituted nitroalkene was tested (1-nitrocyclohexene)2a but was found to be unreactive using the current system.
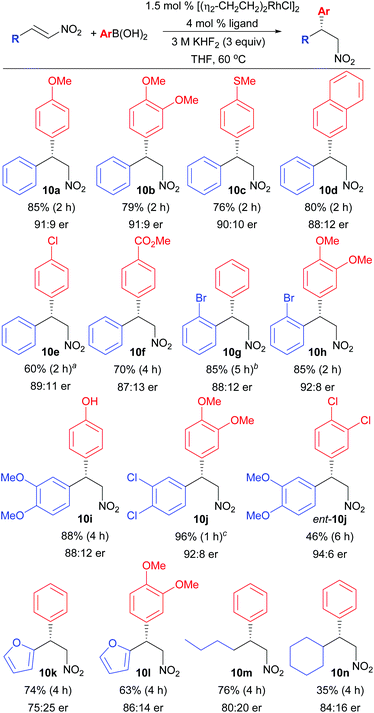 |
| Scheme 3 Rh-catalysed conjugate addition of aryl boronic acids to nitroalkenes. Reaction conditions: 0.5 mmol nitroalkene, boronic acid (1.5 mmol), [(η2-CH2CH2)2RhCl]2 (2.9 mg, 0.0075 mmol), L1 (6.2 mg, 0.020 mmol), 0.5 mL 3 M KHF2, 1.9 mL THF. a Reaction performed using 5 equiv. ArB(OH)2. b Reaction performed on 1.0 g scale. c Reaction performed using CPME as solvent. | |
The proposed mechanism for the present conjugate addition is given in Scheme 4. Based on our previous work employing aryl boronic acids as nucleophiles with our (JoshPhos) RhOH catalyst12 and the work of others,2 the active catalyst is likely the (L1) Rh–OH complex 12. Transmetalation likely occurs through either the boronic acid 9 or a mixed species such as 13 rather than from 14 because when 14 is used directly in place of boronic acid 9 the rate of the reaction was significantly reduced (Table 1, entries 12 & 13 vs. 4 & 10).
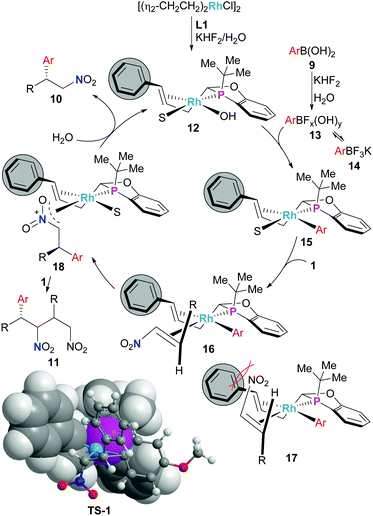 |
| Scheme 4 Proposed catalytic cycle and stereochemical model for the Rh-catalysed conjugate addition of boronic acids to nitroalkenes employing ligand L1. S = solvent. | |
Binding of the nitroalkene electrophile is proposed to prefer coordination through 16 rather than 17 to avoid a steric interaction between the nitro-group and the phenyl group on the alkene of the P,olefin-ligand backbone. Subsequent insertion from complex 16 generates 18 that upon protonation releases the product with the observed absolute stereochemistry and regenerates 12. DFT modelling13,14 of the reaction identified transition structure TS-1 that was fully consistent with this proposal. The formation of impurity 11 is possible from 18 through coordination of additional nitroalkene followed by insertion and protonation to regenerate catalyst 12. If protonation of 18 is difficult, then significant amounts of impurity 11 would be expected. This scenario would be likely under more neutral to basic reaction conditions. Thus, the role of the KHF2 in minimizing the formation of 11 may be due to the increased acidity of the reaction medium with this additive. In support of this conjecture is the fact that the pH of 3 M KHF2 was measured to be ∼2–3, and the aqueous layer present at the end of the nitroalkene conjugate addition reaction had a pH of ∼6.
The synthetic utility of the conjugate addition products is illustrated in Scheme 5. Nitrocompound 10g was chemoselectively reduced to amine 19 using heterogeneous catalysed hydrogenation. Amine 19 was then converted to useful pharmacophores15 using Pd-catalysed transformations. Intramolecular aminocarbonylation16 led to the synthesis of 6-membered ring lactam 20, and Cu-catalysed intramolecular Buchwald–Hartwig17 amination gave access to the chiral 5-membered ring indoline 21.
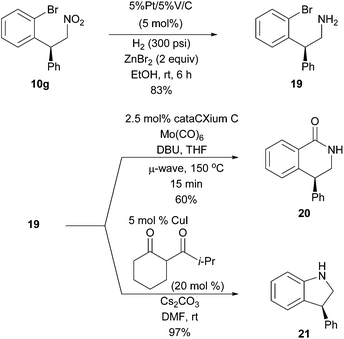 |
| Scheme 5 Application of the Rh-catalysed conjugate addition products in Pd-catalysed ring-formation reactions to access 5- and 6-membered chiral heterocycles. | |
Conclusions
In summary, a Rh-catalysed asymmetric conjugate addition of aryl boronic acids to nitroalkenes was developed employing our P-chiral P,π-hybrid JoshPhos ligands based off of the dihydrobenzooxaphosphole core. The reaction proceeds in moderate to excellent yields with moderate to good enantioselectivities. A mechanistic rational was presented that accounts for the observed stereochemical outcome in the reaction and describes the beneficial effect of KHF2 at minimizing double addition to the nitroalkene electrophile by providing a more acidic reaction mixture to facilitate product protonation from the catalyst. Finally, the synthetic utility of the conjugate addition reaction was demonstrated in the Pd-catalysed synthesis of 5- and 6-membered chiral heterocycles.
Notes and references
-
(a) A. G. M. Barrett and G. G. Graboski, Chem. Rev., 1986, 86, 751–762 CrossRef CAS;
(b) N. Sewald and V. Wendisch, Tetrahedron: Asymmetry, 1998, 9, 1341–1344 CrossRef CAS;
(c) J. P. G. Versleijen, A. M. Van Leusen and B. L. Feringa, Tetrahedron Lett., 1999, 40, 5803–5806 CrossRef CAS;
(d) A. Alexakis and C. Benhaim, Org. Lett., 2000, 2, 2579–2581 CrossRef CAS PubMed;
(e) S. Ongeri, U. Piarulli, R. F. W. Jackson and C. Gennari, Eur. J. Org. Chem., 2001, 803–807 CrossRef CAS;
(f) A. Duursma, A. J. Minaard and B. L. Feringa, Tetrahedron, 2002, 58, 5773–5778 CrossRef CAS;
(g) C. A. Luchaco-Cullis and A. H. Hoveyda, J. Am. Chem. Soc., 2002, 124, 8192–8193 CrossRef CAS PubMed;
(h) A. Duursma, A. J. Minnaard and B. L. Feringa, J. Am. Chem. Soc., 2003, 125, 3700–3701 CrossRef CAS PubMed;
(i) J. Wu, D. M. Mampreian and A. H. Hoveyda, J. Am. Chem. Soc., 2005, 127, 4584–4585 CrossRef CAS PubMed;
(j) X. Zeng, J. J. Gao, J. J. Song, S. Ma, J.-N. Desrosiers, J. A. Mulder, S. Rodriguez, M. A. Herbage, N. Haddad, B. Qu, K. R. Fandrick, N. Grinberg, H. Lee, X. Wei, N. K. Yee and C. H. Senanayake, Angew. Chem., Int. Ed., 2014, 53, 12153–12157 CrossRef CAS PubMed.
-
(a) T. Hayashi, T. Senda and M. Ogasawara, J. Am. Chem. Soc., 2000, 122, 10716–10717 CrossRef CAS;
(b) Z.-Q. Wang, C.-G. Feng, S.-S. Zhang, M.-H. Xu and G.-Q. Lin, Angew. Chem., Int. Ed., 2010, 49, 5780–5783 CrossRef CAS PubMed;
(c) F. Lang, G. Chen, L. Li, J. Xing, F. Han, L. Cun and J. Liao, Chem. – Eur. J., 2011, 17, 5242–5245 CrossRef CAS PubMed;
(d) J. Xing, G. Chen, P. Cao and J. Liao, Eur. J. Org. Chem., 2012, 1230–1236 CrossRef CAS;
(e) K.-C. Huang, B. Gopula, T.-S. Kuo, C.-W. Chiang, P.-Y. Wu, J. P. Henschke and H.-L. Wu, Org. Lett., 2013, 15, 5730–5733 CrossRef CAS PubMed;
(f) F. Xue, D. Wang, X. Li and B. Wan, J. Org. Chem., 2012, 77, 3071–3081 CrossRef CAS PubMed;
(g) V. R. Jumde and A. Iuliano, Adv. Synth. Catal., 2013, 355, 3475–3483 CrossRef CAS.
-
(a) A. Brossi, G. Grethe, S. Teitel, W. C. Wildman and D. T. Bailey, J. Org. Chem., 1970, 35, 1100–1104 CrossRef CAS;
(b) B. E. Maryanoff, D. F. McComsey, J. F. Gardocki, R. P. Shank, M. J. Costanzo, S. O. Nortey, C. R. Schneider and P. E. Setler, J. Med. Chem., 1987, 30, 1433–1454 CrossRef CAS PubMed;
(c) B. E. Maryanoff, D. F. McComsey and J. C. Craig, Chirality, 1998, 10, 169–172 CrossRef CAS;
(d) N. Kapadia and W. W. Harding, Bioorg. Med. Chem. Lett., 2015, 25, 3451–3454 CrossRef CAS PubMed;
(e) J. D. Scott and R. M. Williams, Chem. Rev., 2002, 102, 1669–1730 CrossRef CAS PubMed;
(f) W.-L. Wu, D. A. Burnett, R. Spring, W. J. Greenlee, M. Smith, L. Favreau, A. Fawzi, H. Zhang and J. E. Lachowicz, J. Med. Chem., 2005, 48, 680 CrossRef CAS PubMed.
-
(a) M. Sakai, H. Hayashi and N. Miyaura, Organometallics, 1997, 16, 4229–4231 CrossRef CAS;
(b) Y. Takaya, M. Ogasawara and T. Hayashi, J. Am. Chem. Soc., 1998, 120, 5579–5580 CrossRef CAS.
- Reviews:
(a) T. Hayashi and K. Yamasaki, Chem. Rev., 2003, 103, 2829 CrossRef CAS PubMed;
(b) K. Fagnou and M. Lautens, Chem. Rev., 2003, 103, 169 CrossRef CAS PubMed;
(c) J. Christoffers, G. Koripelly, A. Rosiak and M. Rossle, Synthesis, 2007, 1279 CrossRef CAS.
- Selected examples:
(a) M. Kuriyama, T. Soeta, X. Hao, Q. Chen and K. Tomioka, J. Am. Chem. Soc., 2004, 126, 8128–8129 CrossRef CAS PubMed;
(b) N. Tokunaga, Y. Otomaru, K. Okamoto, K. Ueyama, R. Shintani and T. Hayashi, J. Am. Chem. Soc., 2004, 126, 13584–13585 CrossRef CAS PubMed;
(c) Y. Otomaru, N. Tokunaga, R. Shintani and T. Hayashi, Org. Lett., 2005, 7, 307–310 CrossRef CAS PubMed;
(d) Z.-Q. Wang, C.-G. Feng, M.-H. Xu and G.-Q. Lin, J. Am. Chem. Soc., 2007, 129, 5336–5337 CrossRef CAS PubMed;
(e) R. B. C. Jagt, P. Y. Toulle, D. Geerdink, J. G. de Vries, B. L. Feringa and A. J. Minnaard, Angew. Chem., Int. Ed., 2006, 45, 2789–2791 CrossRef CAS PubMed;
(f) Z. Cui, H.-J. Yu, R.-F. Yang, W.-Y. Gao, C.-G. Feng and G.-Q. Lin, J. Am. Chem. Soc., 2011, 133, 12394–12397 CrossRef CAS PubMed; Z. Cui, Y.-J. Chen, W.-Y. Gao, C.-G. Feng and G.-Q. Lin, Org. Lett., 2014, 16, 1016–1019 Search PubMed;
(g) D. J. Weix, Y. Shi and J. A. Ellman, J. Am. Chem. Soc., 2005, 127, 1092–1093 CrossRef CAS PubMed.
-
(a) H.-F. Duan, J.-H. Xie, X.-C. Qiao, L.-X. Wang and Q.-L. Zhou, Angew. Chem., Int. Ed., 2008, 47, 4351 CrossRef CAS PubMed;
(b) T.-S. Zhu, S.-S. Jin and M.-H. Xu, Angew. Chem., Int. Ed., 2012, 51, 780 CrossRef CAS PubMed.
- T. Hayashi, K. Ueyama, N. Tokunaga and K. Yoshida, J. Am. Chem. Soc., 2003, 125, 11508 CrossRef CAS PubMed.
- C. Defieber, J.-F. Paquin, S. Serna and E. M. Carreira, Org. Lett., 2004, 6, 3873–3876 CrossRef CAS PubMed.
- Reviews:
(a) X. Feng and H. Du, Asian J. Org. Chem., 2012, 1, 204–213 CrossRef CAS;
(b) C.-G. Feng, M.-H. Xu and G.-Q. Lin, Synlett, 2011, 1345–1356 CAS.
-
(a) W. Tang, A. G. Capacci, X. Wei, W. Li, A. White, N. D. Patel, J. Savoie, J. J. Gao, S. Rodriguez and B. Qu,
et al.
, Angew. Chem., Int. Ed., 2010, 49, 5879–5883 (
Angew. Chem.
, 2010
, 122
, 6015–6019
) CrossRef CAS PubMed;
(b) S. Rodriguez, B. Qu, N. Haddad, D. C. Reeves, W. Tang, H. Lee, D. Krishnamurthy and C. H. Senanayake, Adv. Synth. Catal., 2011, 353, 533–537 CrossRef CAS;
(c) W. Tang, N. D. Patel, G. Xu, X. Xu, J. Savoie, S. Ma, M.-H. Hao, S. Keshipeddy, A. G. Capacci and X. Wei,
et al.
, Org. Lett., 2012, 14, 2258–2261 CrossRef CAS PubMed;
(d) G. Xu, W. Fu, G. Liu, C. H. Senanayake and W. Tang, J. Am. Chem. Soc., 2014, 136, 570–573 CrossRef CAS PubMed;
(e) W. Tang, B. Qu, A. G. Capacci, S. Rodriguez, X. Wei, N. Haddad, B. Narayanan, S. Ma, N. Grinberg and K. N. Yee,
et al.
, Org. Lett., 2010, 12, 176–179 CrossRef CAS PubMed;
(f) W. Tang, A. G. Capacci, A. White, S. Ma, S. Rodriguez, B. Qu, J. Savoie, N. D. Patel, X. Wei and N. Haddad,
et al.
, Org. Lett., 2010, 12, 1104–1107 CrossRef CAS PubMed;
(g) S. Rodriguez, B. Qu, K. R. Fandrick, F. Buono, N. Haddad, Y. Xu, M. A. Herbage, X. Zeng, S. Ma and N. Grinberg,
et al.
, Adv. Synth. Catal., 2014, 356, 301–307 CrossRef CAS;
(h) D. R. Fandrick, K. R. Fandrick, J. T. Reeves, Z. Yan, W. Tang, A. G. Capacci, S. Rodriguez, J. J. Song, H. Lee and N. K. Lee,
et al.
, J. Am. Chem. Soc., 2010, 132, 7600–7601 CrossRef CAS PubMed;
(i) B. Qu, L. P. Samankumara, J. Savoie, D. R. Fandrick, N. Haddad, X. Wei, S. Ma, H. Lee, S. Rodriguez and C. A. Busacca,
et al.
, J. Org. Chem., 2014, 79, 993–1000 CrossRef CAS PubMed;
(j) B. Qu, L. P. Samankumara, S. Ma, D. R. Fandrick, J.-N. Desrosiers, S. Rodriguez, Z. Li, N. Haddad, Z. S. Han, K. McKellop, S. Pennino, N. Grinberg, N. C. Gonnella, J. J. Song and C. H. Senanayake, Angew. Chem., Int. Ed., 2014, 53, 14428 CrossRef CAS PubMed;
(k) J. D. Sieber, B. Qu, S. Rodriguez, N. Haddad, N. Grinberg, H. Lee, J. J. Song, N. K. Yee and C. H. Senanayake, J. Org. Chem., 2016, 81, 729–736 CrossRef CAS PubMed.
- J. D. Sieber, D. Chennamadhavuni, K. R. Fandrick, B. Qu, Z. S. Han, J. Savoie, S. Ma, L. P. Samankumara, N. Grinberg, H. Lee, J. J. Song and C. H. Senanayake, Org. Lett., 2014, 16, 5494–5497 CrossRef CAS PubMed.
- DFT calculations: B3LYP and LANL2DZ basis set, see:
(a)
T. H. Dunning Jr. and P. J. Hay, in Modern Theoretical Chemistry, ed. H. F. Schaefer III, Plenum, New York, 1976, vol. 3, pp. 1–28 Search PubMed;
(b) P. J. Hay and W. R. Wadt, J. Chem. Phys., 1985, 82, 270–283 CrossRef CAS;
(c) P. J. Hay and W. R. Wadt, J. Chem. Phys., 1985, 82, 299–310 CrossRef CAS;
(d) W. R. Wadt and P. J. Hay, J. Chem. Phys., 1985, 82, 284–298 CrossRef CAS.
-
M. J. Frisch, W. G. Trucks, H. B. Schlegel, G. E. Scuseria, M. A. Robb, J. R. Cheeseman, G. Scalmani, V. Barone, B. Mennucci, G. A. Petersson, H. Nakatsuji, M. Caricato, X. Li, H. P. Hratchian, A. F. Izmaylov, J. Bloino, G. Zheng, J. L. Sonnenberg, M. Hada, M. Ehara, K. Toyota, R. Fukuda, J. Hasegawa, M. Ishida, T. Nakajima, Y. Honda, O. Kitao, H. Nakai, T. Vreven, J. A. Montgomery Jr., J. E. Peralta, F. Ogliaro, M. Bearpark, J. J. Heyd, E. Brothers, K. N. Kudin, V. N. Staroverov, T. Keith, R. Kobayashi, J. Normand, K. Raghavachari, A. Rendell, J. C. Burant, S. S. Iyengar, J. Tomasi, M. Cossi, N. Rega, J. M. Millam, M. Klene, J. E. Knox, J. B. Cross, V. Bakken, C. Adamo, J. Jaramillo, R. Gomperts, R. E. Stratmann, O. Yazyev, A. J. Austin, R. Cammi, C. Pomelli, J. W. Ochterski, R. L. Martin, K. Morokuma, V. G. Zakrzewski, G. A. Voth, P. Salvador, J. J. Dannenberg, S. Dapprich, A. D. Daniels, O. Farkas, J. B. Foresman, J. V. Ortiz, J. Cioslowski and D. J. Fox, Software for DFT: Gaussian 09, Revision C.01, Gaussian, Inc., Wallingford, CT, 2010 Search PubMed.
-
(a) J. Bermudez, S. Dabbs, K. A. Joiner and F. D. King, J. Med. Chem., 1990, 33, 1929–1935 CrossRef CAS PubMed;
(b) F. Zhao, J. Li, Y. Chen, Y. Tian, C. Wu, Y. Xie, Y. Zhou, J. Wang, X. Xie and H. Liu, J. Med. Chem., 2016, 59, 3826–3839 CrossRef CAS PubMed;
(c) L. Chang, J. D. Podoll, W. Wang, S. Walls, C. P. O'Rourke and X. Wang, J. Med. Chem., 2014, 57, 3803–3817 CrossRef CAS PubMed;
(d) L. A. T. Cleghorn, S. Albrecht, L. Stojanovski, F. R. J. Simeons, S. Norval, R. Kime, I. T. Collie, M. De Rycker, L. Campbell, I. Hallyburton, J. A. Frearson, P. G. Wyatt, K. D. Read and I. H. Gilbert, J. Med. Chem., 2015, 58, 7695–7706 CrossRef CAS PubMed;
(e) R. Ortega, E. Ravina, C. F. Masaguer, F. Areias, J. Brea, M. I. Loza, L. Lopex, J. Selent, M. Pastor and F. Sanz, Bioorg. Med. Chem. Lett., 2009, 19, 1773–1778 CrossRef CAS PubMed.
-
(a) J. Wannbert and M. Larhed, J. Org. Chem., 2003, 68, 5750–5753 CrossRef PubMed;
(b) J. R. Martinelli, D. A. Watson, D. M. M. Freckmann, T. E. Barder and S. L. Buchwald, J. Org. Chem., 2008, 73, 7102–7107 CrossRef CAS PubMed.
- A. Shafir and S. L. Buchwald, J. Am. Chem. Soc., 2006, 128, 8742–8743 CrossRef CAS PubMed.
Footnote |
† Electronic supplementary information (ESI) available. See DOI: 10.1039/c6qo00311g |
|
This journal is © the Partner Organisations 2016 |