DOI:
10.1039/C6QO00252H
(Research Article)
Org. Chem. Front., 2016,
3, 1154-1158
Equilibrium acidities of BINOL type chiral phenolic hydrogen bonding donors in DMSO†
Received
7th June 2016
, Accepted 23rd July 2016
First published on 26th July 2016
Abstract
The pKa values of fifteen BINOL type chiral phenolic catalysts were determined by the overlapping indicator method in DMSO via UV spectrophotometric titrations. The acidities cover the range from 9.30 to 16.43. The pKa gap between BINOL and 2-naphthol was explained by IR spectrum data, crystal structure and 1H NMR. The relationship between the pKa values of 3,3′-modified BINOLs and Hammett ortho substituent constants (σo−) was investigated and a good correlation (R2 = 0.984) was obtained. The results may be helpful for the rational design and development of novel BINOL type phenolic catalysts, sensors and ligands.
Introduction
1,1′-Binaphthyl-2,2′-diol (BINOL) is one of the most representative axially chiral C2 symmetric molecules in organic chemistry.1 The steric hindrances between 2,2′-hydroxyls and between 8,8′-hydrogens restrict the free rotation of the two 2-naphthol units around the 1,1′-bond, which orient the chirality of BINOL. The two optically active enantiomers of BINOL can readily be resolved and both possess a thermally stable chiral configuration even at high temperature under neutral conditions.2 Although BINOL was first reported in 1873,3 its potential as a chirality source was not recognized until the early 1970s when Cram initiated his pioneering work of high chiral recognition by utilizing optically active BINOL based macrocycle hosts.4 Soon afterwards in 1979, Noyori first reported that chiral BINOL could serve as a superb ligand in the enantioselective reduction of ketones and aldehydes.5 Inspired by these monumental contributions, great efforts have been devoted to develop structurally diverse BINOL derivatives, and a tremendous amount of achievements has been reported in research fields such as molecular recognition,1e,i metal-mediated asymmetric catalysis1b–d,g and chiral material building.1a,6 Recently, with the advent and dramatic blossoming of the area of organocatalysis,7 BINOL has also been recognized as a unique scaffold for designing hydrogen bonding donor organocatalysts1f,h,7e and a broad array of asymmetric transformations, such as alkenyl(alkynyl)boration,8 allyl(propargyl)boration9 and MBH reaction10 have been realized. Today, the versatile BINOL backbone has been identified as one of the most privileged chirality inducers.11
It's worth mentioning that the hydrogen bonding interaction between BINOL and other molecules plays a key role in different fields, especially in molecule recognition1e,i and organocatalysis (Scheme 1).1f,h,8–10 Thus, the hydrogen bonding strengths, generally represented by donors’ equilibrium acidities,12 must drastically influence the capability of recognition and activation. Although, as an essential physical organic parameter, the pKa value of BINOL has been frequently cited in reports, we were astonished to find that the strictness of citation has been largely ignored.1b,7e,13 To the best of our knowledge, there are only a few reports on the dissociation constant of BINOL in aqueous or mixed protic solvents without a systematic discussion of the substitution effect.14 Therefore, accurate pKa measurements of BINOL and its derivatives are significantly desirable.
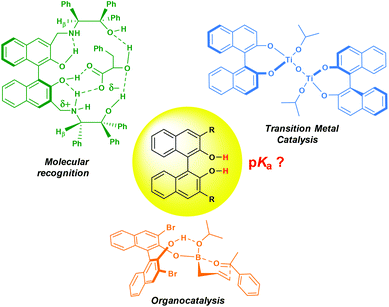 |
| Scheme 1 Representative applications promoted by BINOLs. | |
In the past decade, great attention has been attracted by the field of equilibrium acidities of Brønsted acid type organocatalysts in DMSO,15 owing to the development of numerous classes of novel catalysts. Our group has also reported the pKa values of some widely used hydrogen bonding organocatalysts including thioureas,15a squaramides,15h proline derivatives15j and cinchona alkaloids.15k Herein, we report the determination of the pKa values of BINOL type phenolic catalysts.
Results and discussion
As shown in Scheme 2, thirteen (S)-BINOL derivatives and two similar diol catalysts were selected as target compounds. The pKa values were measured by means of the indicator overlapping method via UV/vis spectrophotometric titration in DMSO.16 As illustrated by the example of catalyst 1 (Fig. 1), a solution of 2-Br-9-PhS-FH was titrated into the K-dimsyl solution until the base was completely consumed, during which the weight of the cell and the UV spectrum were both recorded (Fig. 1a). Then, a solution of catalyst 1 was added and the weight of the cell and the corresponding spectrum were also recorded after each titration. The equilibrium acidity of catalyst 1 could be obtained from the data of the changed absorbance (Fig. 1b). The indicators employed in this study are shown in Scheme 3. The results of the pKa values are summarized in Table 1.
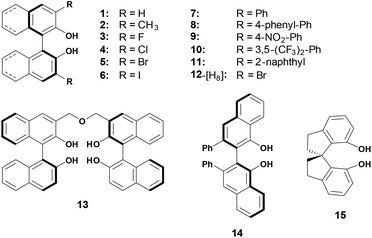 |
| Scheme 2 Studied BINOL type phenolic catalysts in this work. | |
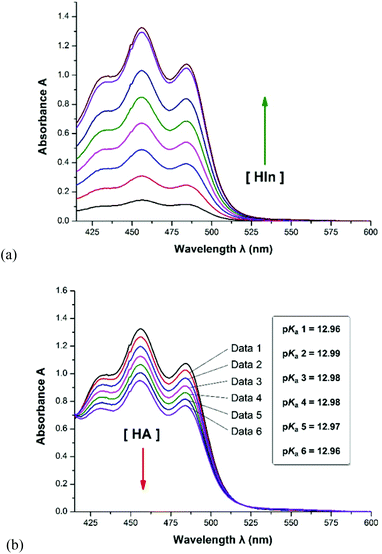 |
| Fig. 1 (a) Absorption spectra of the anion derived from 2-Br-9-PhS-FH for various added amounts of 2-Br-9-PhS-FH during the titration. (b) Absorption spectra of the anion derived from 2-Br-9-PhS-FH for various added amounts of catalyst 1 during the titration. | |
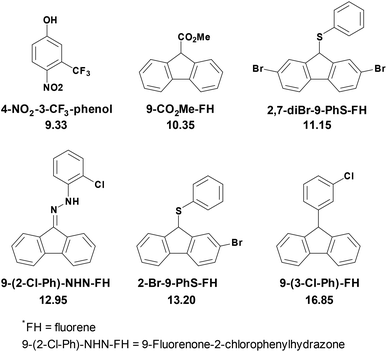 |
| Scheme 3 Indicators’ structures and their pKa values in DMSO.16,17 | |
Table 1 pKa values of BINOL type phenolic catalysts in DMSO
Catalyst |
pKa value |
Indicatora |
FH = fluorene; 9-(2-Cl-Ph)-NHN-FH = 9-fluorenone-2-chlorophenylhydrazone.
See ref. 18.
|
1
|
12.98 ± 0.05b |
2-Br-9-PhS-FH
|
2
|
11.95 ± 0.03 |
2,7-diBr-9-PhS-FH
|
3
|
10.39 ± 0.03 |
9-CO2Me-FH
|
4
|
9.78 ± 0.02 |
4-NO2-3-CF3-phenol
|
5
|
9.44 ± 0.02 |
4-NO2-3-CF3-phenol
|
6
|
9.30 ± 0.03 |
4-NO2-3-CF3-phenol
|
7
|
10.93 ± 0.03 |
2,7-diBr-9-PhS-FH
|
8
|
10.89 ± 0.01 |
2,7-diBr-9-PhS-FH
|
9
|
9.99 ± 0.01 |
9-CO2Me-FH
|
10
|
9.68 ± 0.03 |
9-CO2Me-FH
|
11
|
10.73 ± 0.01 |
2,7-diBr-9-PhS-FH
|
12
|
12.35 ± 0.02 |
9-(2-Cl-Ph)-NHN-FH
|
13
|
11.92 ± 0.04 |
2,7-diBr-9-PhS-FH
|
14
|
10.45 ± 0.02 |
2,7-diBr-9-PhS-FH
|
15
|
16.43 ± 0.02 |
9-(3-Cl-Ph)-FH
|
As shown in Table 1, the pKa values of studied hydrogen bonding donor catalysts cover the range of 9.30–16.43, which fall in the acidity range of thioureas/squaramides. Compared with 2-naphthol (pKa = 17.14 in DMSO),19 the acidity of BINOL (catalyst 1) increased by about 4 pK units, which agrees well with the previous calculation results (13.22 in DMSO).15i Evidenced by IR spectrum data,20 almost only the intermolecular hydrogen bond between BINOL and solvent molecules was observed in DMSO, indicating that BINOL's acidity can't be enhanced by “OH⋯OH” intramolecular hydrogen bonding. However, a strong intramolecular hydrogen bonding was clearly observed in mono deprotonated BINOL and derivatives,21 which may primarily account for this great acidification phenomenon (Scheme 4).
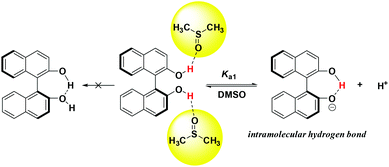 |
| Scheme 4 Proposed dissociation equilibrium of BINOL in DMSO. | |
For 2–11, each of which bears identical substituents at the 3,3′-positions, their acidities are all stronger than the unmodified BINOL. Unexpectedly, even substituted by the methyl group, catalyst 2's pKa value is also lower than BINOL by about 1 pK unit. This peculiar increase of acidity may be attributed to the steric interaction in the ortho position, which also exists in ortho-substituted phenol and protonated aniline.22 While for 3–6, which are substituted by halogen atoms, the acidities increase in an order which is opposite to that of the substituents’ electro-negativities. In order to investigate the substituent effect, the relationship between pKa values of catalysts 1–7 and corresponding Hammett σo− constants23 was then investigated and a good correlation (R2 = 0.986) was obtained in the regression analysis (Fig. 2). However, to our surprise, unmodified BINOL deviated badly from linearity,18 indicating that the ortho effect significantly influences the chemical environment around the OH groups of 3,3′-modified BINOL derivatives. For 12, which is partially hydrogenated from 5, the pKa value increases by about 3 units compared with 5. This pKa gap may be attributed to the combination of both electronic and steric effects aroused by the reduction on the naphthol ring.
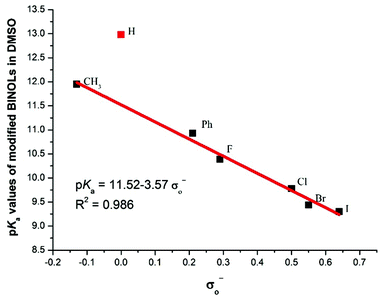 |
| Fig. 2 Correlation between the pKa values of 3,3′-modified BINOLs and the Hammett substituent constants (σo−). | |
For 7–11, which are substituted by aromatic groups at the 3,3′-position, their acidities increased by 2–3 pK units compared with BINOL. With regard to 7, 8 and 11, extending the conjugated system of the substituent may weakly enhance the acidities. For 9 and 10, the acidities were strengthened by about 1 pK unit when strong electron-withdrawing groups (NO2, CF3) were introduced into the Ph substituent. It is well accepted that the Lewis acidities of BINOL–metal complexes which may directly affect their catalytic activities can easily be tuned by varying the electronic effect of the substituents. Thus, the pKa values discussed above must be valuable for quantitatively estimating the influences caused by modifications at 3,3′-positions.
Finally, three similar hydrogen bonding donor catalysts were also investigated. Linked by two BINOL units at their 3-positions, catalyst 13 with four OH groups exhibited a lower pKa value than the single BINOL by 1 pK unit. For 14 (VANOL), the acidity is stronger than its isomer 7 by 0.44 pK units, which may be interpreted by the pKa gap between the two positional isomers of naphthol.18 While, for 15 (SPINOL) containing a spirocyclic framework, the acidic strength is much weaker than that of BINOL by 3 pK units. Due to the higher rigid scaffold of SPINOL, the intramolecular hydrogen bond is almost forbidden in its mono deprotonated form, which may primarily account for the magnitude of pKa increment. In addition, a strong homoconjugation phenomenon was observed exclusively during the measurement of the acidity of SPINOL, which also powerfully supports the hypothesis we proposed above.
Conclusions
In summary, we have determined the pKa values of 15 representative BINOL type hydrogen bonding donor catalysts in DMSO by adopting the classical overlapping indicator method. The pKa values are in the range of 9.30–16.43. The relationship between the pKa values of several 3,3′-modified BINOL derivatives and the Hammett ortho substituent constants was investigated and a good correlation was demonstrated. We believe that these data should be helpful in providing a clearer insight into the mechanism of recognition and catalysis. Furthermore, the results we reported here may benefit the advances in rational design and development of novel BINOL and other diol type catalysts, sensors and ligands.
Experimental
General experimental methods
The UV spectrum measurements were performed on a Hitachi U-3000 UV/vis spectrometer. Commercial reagents were used as received, unless otherwise stated. Catalyst 1 was used after recrystallization from ethyl acetate. Catalysts 2–13 were synthesized according to the literature procedures.24 Catalysts 14 and 15 were purchased from Daicel Chiral Technologies (China) Co, Ltd.
pKa measurement
The treatment of DMSO and base (K-dimsyl solution) strictly followed the description in the literature.16a The determinations of the pKa values were carried out under an atmosphere of argon. A solution of the indicator with known pKa value and concentration (1.3–2.0 × 10−2 M) was added dropwise to a solution of K-dimsyl (1.0–1.5 mL, 0.5–1.0 × 10−3 M), then a work calibration curve was obtained from the spectrum and the weight of cell was recorded after each addition of the titrant. After the base was completely consumed by adding excess indicator solution, which is monitored by a UV/vis spectrophotometer, a solution of the target compound with known concentration (1.3–2.0 × 10−2 M) was added. The corresponding pKa value was obtained from the change of absorbance and the amount of target compound recorded after each addition of the titrant. The equilibrium acidity of the target compound was calculated according to eqn (1) and (2). Each target compound was measured at least two times against the corresponding indicator demonstrated in Scheme 3 by the method described above. |  | (1) |
|  | (2) |
The interferences of anion absorption between catalysts 9, 10 and the corresponding indicator were serious during pKa determination and the problem was solved by a “double wavelength calculation method” developed by our group which is unpublished.
Acknowledgements
We thank the 973 Program (2012CB821600) and NSFC (21390400 and 21421062) for financial support.
Notes and references
- For selected reviews, see:
(a) L. Pu, Chem. Rev., 1998, 98, 2405 CrossRef CAS PubMed;
(b) Y. Chen, S. Yekta and A. K. Yudin, Chem. Rev., 2003, 103, 3155 CrossRef CAS PubMed;
(c) S. G. Telfer and R. Kuroda, Coord. Chem. Rev., 2003, 242, 33 CrossRef CAS;
(d) J. M. Brunel, Chem. Rev., 2007, 107, PR1 CrossRef CAS;
(e) L. Pu, Acc. Chem. Res., 2012, 45, 150 CrossRef CAS PubMed;
(f) P. S. Bhadury, Y.-Y. Yao and Y.-G. He, Curr. Org. Chem., 2012, 16, 1730 CrossRef CAS;
(g) L. Pu, Acc. Chem. Res., 2014, 47, 1523 CrossRef CAS PubMed;
(h) G.-Z. Li, F.-J. Liu and M.-S. Wu, ARKIVOC, 2015, 6, 140 Search PubMed;
(i) S.-S. Yu and L. Pu, Tetrahedron, 2015, 71, 745 CrossRef CAS.
-
(a) E. P. Kyba, G. W. Gokel, F. de Jong, K. Koga, L. R. Sousa, M. G. Siegel, L. Kaplan, G. D. Y. Sogah and D. J. Cram, J. Org. Chem., 1977, 42, 4173 CrossRef CAS;
(b) L. Meca, D. Reha and Z. Havlas, J. Org. Chem., 2003, 68, 5677 CrossRef CAS PubMed.
- V. von Richter, Chem. Ber., 1873, 6, 1252 Search PubMed.
- For selective examples, see:
(a) E. B. Kyba, K. Koga, L. R. Sousa, M. G. Siegel and D. J. Cram, J. Am. Chem. Soc., 1973, 95, 2692 CrossRef CAS;
(b) D. J. Cram and J. M. Cram, Science, 1974, 183, 803 CAS;
(c) R. C. Helgeson, J. M. Timko, P. Moreau, S. C. Peacock, J. M. Mayer and D. J. Cram, J. Am. Chem. Soc., 1974, 96, 6762 CrossRef CAS;
(d) D. J. Cram and J. M. Cram, Acc. Chem. Res., 1978, 11, 8 CrossRef CAS;
(e) M. Newcomb, J. L. Toner, R. C. Helgeson and D. J. Cram, J. Am. Chem. Soc., 1979, 101, 4941 CrossRef CAS;
(f) S. S. Peacock, D. M. Walba, F. C. A. Gaeta, R. C. Helgeson and D. J. Cram, J. Am. Chem. Soc., 1980, 102, 2043 CrossRef CAS;
(g) D. J. Cram, Science, 1988, 240, 760 CAS.
- R. Noyori, I. Tomino and Y. Tanimoto, J. Am. Chem. Soc., 1979, 101, 3129 CrossRef CAS.
-
L. Pu, 1,1′-Binaphthyl-Based Chiral Materials: Our Journey, World Scientific Inc., Imperial College Press, London, 2010 Search PubMed.
- For selected reviews, see:
(a) P. I. Dalko and L. Moisan, Angew. Chem., Int. Ed., 2001, 40, 3726 CrossRef CAS;
(b) P. R. Schreiner, Chem. Soc. Rev., 2003, 32, 289 RSC;
(c) P. M. Pihko, Angew. Chem., Int. Ed., 2004, 43, 2062 CrossRef CAS PubMed;
(d) M. S. Taylor and E. N. Jacobsen, Angew. Chem., Int. Ed., 2006, 45, 1520 CrossRef CAS PubMed;
(e) A. G. Doyle and E. N. Jacobsen, Chem. Rev., 2007, 107, 5713 CrossRef CAS PubMed;
(f) D. W. C. MacMillan, Nature, 2008, 455, 304 CrossRef CAS PubMed.
- For selective examples, see:
(a) T. R. Wu and J. M. Chong, J. Am. Chem. Soc., 2005, 127, 3244 CrossRef CAS PubMed;
(b) S. C. Pellegrinet and J. M. Goodman, J. Am. Chem. Soc., 2006, 128, 3116 CrossRef CAS PubMed;
(c) T. R. Wu and J. M. Chong, J. Am. Chem. Soc., 2007, 129, 4908 CrossRef CAS PubMed;
(d) S. Lou and S. E. Schaus, J. Am. Chem. Soc., 2008, 130, 6922 CrossRef CAS PubMed;
(e) R. S. Paton, J. M. Goodman and S. C. Pellegrinet, J. Org. Chem., 2008, 73, 5078 CrossRef CAS PubMed;
(f) B. J. Lundy, S. Jansone-Popova and J. A. May, Org. Lett., 2011, 13, 4958 CrossRef CAS PubMed;
(g) P. Q. Le, T. S. Nguyen and J. A. May, Org. Lett., 2012, 14, 6104 CrossRef CAS PubMed.
- For selective examples, see:
(a) T. R. Wu, L.-X. Shen and J. M. Chong, Org. Lett., 2004, 6, 2701 CrossRef CAS PubMed;
(b) S.-H. Yu, M. J. Ferguson, R. McDonald and D. G. Hall, J. Am. Chem. Soc., 2005, 127, 12808 CrossRef CAS PubMed;
(c) T. R. Wu and J. M. Chong, J. Am. Chem. Soc., 2006, 128, 9646 CrossRef CAS PubMed;
(d) S. Lou, P. N. Moquist and S. E. Schaus, J. Am. Chem. Soc., 2006, 128, 12660 CrossRef CAS PubMed;
(e) S. Lou, P. N. Moquist and S. E. Schaus, J. Am. Chem. Soc., 2007, 129, 15398 CrossRef CAS PubMed;
(f) D. S. Barnett, P. N. Moquist and S. E. Schaus, Angew. Chem., Int. Ed., 2009, 48, 8679 CrossRef CAS PubMed;
(g) D. S. Barnett and S. E. Schaus, Org. Lett., 2011, 13, 4020 CrossRef CAS PubMed.
- For selective examples, see:
(a) N. T. McDougal and S. E. Schaus, J. Am. Chem. Soc., 2003, 125, 12094 CrossRef CAS PubMed;
(b) N. T. McDougal, W. L. Trevellini, S. A. Rodgen, L. T. Kliman and S. E. Schaus, Adv. Synth. Catal., 2004, 346, 1231 CrossRef CAS.
-
Q.-L. Zhou, Privileged Chiral Ligands and Catalysts, Wiley-VCH, 2011 Search PubMed.
- P. Gilli, L. Pretto, V. Bertolasi and G. Gilli, Acc. Chem. Res., 2009, 42, 33 CrossRef CAS PubMed.
- For selective examples, see:
(a) L. J. P. Martyn, S. Pandiaraju and A. K. Yudin, J. Organomet. Chem., 2000, 603, 98 CrossRef CAS;
(b) S. Pandiaraju, G. Chen, A. Lough and A. K. Yudin, J. Am. Chem. Soc., 2001, 123, 3850 CrossRef CAS PubMed;
(c) P. N. H. Huynh, R. R. Walvoord and M. C. Kozlowski, J. Am. Chem. Soc., 2012, 134, 15621 CrossRef CAS PubMed;
(d) R. R. Walvoord, P. N. H. Huynh and M. C. Kozlowski, J. Am. Chem. Soc., 2014, 136, 16055 CrossRef CAS PubMed;
(e) T. Akiyama and K. Mori, Chem. Rev., 2015, 115, 9277 CrossRef CAS PubMed;
(f) M. Samet and S. R. Kass, J. Org. Chem., 2015, 80, 7727 CrossRef CAS PubMed.
-
(a) H. Musso and H. Matthies, Chem. Ber., 1961, 94, 356 CrossRef CAS;
(b) E. Billiot, J. Macossay, S. Thibodeaux, S. A. Shamsi and I. M. Warner, Anal. Chem., 1998, 70, 1375 CrossRef CAS PubMed;
(c) A. K. Yudin, L. J. P. Martyn, S. Pandiaraju, J. Zheng and A. Lough, Org. Lett., 2000, 2, 41 CrossRef CAS PubMed;
(d) C. Niezborala and F. Hache, J. Am. Chem. Soc., 2008, 130, 12783 CrossRef CAS PubMed;
(e) N. Mofaddela, H. Krajian, D. Villeminb and P. L. Desbenea, Talanta, 2009, 78, 631 CrossRef PubMed;
(f) D. Seebach, A. K. Beck, H. Bichsel, A. Pichota, C. Sparr, R. Wünsch and W. B. Schweizer, Helv. Chim. Acta, 2012, 95, 1273 CrossRef.
- For selected examples, see:
(a) X. Li, H. Deng, B. Zhang, J. Y. Li, L. Zhang, S. Z. Luo and J.-P. Cheng, Chem. – Eur. J., 2010, 16, 450 CrossRef CAS PubMed;
(b) X.-Y. Huang, H.-J. Wang and J. Shi, J. Phys. Chem. A, 2010, 114, 1068 CrossRef CAS PubMed;
(c) P. Christ, A. G. Lindsay, S. S. Vormittag, J.-M. Neudörfl, A. Berkessel and A. C. O'Donoghue, Chem. – Eur. J., 2011, 17, 8524 CrossRef CAS PubMed;
(d) G. Jakab, C. Tancon, Z. G. Zhang, K. M. Lippert and P. R. Schreiner, Org. Lett., 2012, 14, 1724 CrossRef CAS PubMed;
(e) R. S. Massey, C. J. Collett, A. G. Lindsay, A. D. Smith and A. C. O'Donoghue, J. Am. Chem. Soc., 2012, 134, 20421 CrossRef CAS PubMed;
(f) K. Kaupmees, N. Tolstoluzhsky, S. Raja, M. Rueping and I. Leito, Angew. Chem., Int. Ed., 2013, 52, 11569 CrossRef CAS PubMed;
(g) C. Yang, X.-S. Xue, J.-L. Jin, X. Li and J.-P. Cheng, J. Org. Chem., 2013, 78, 7076 CrossRef CAS PubMed;
(h) X. Ni, X. Li, Z. Wang and J.-P. Cheng, Org. Lett., 2014, 16, 1786 CrossRef CAS PubMed;
(i) C. Yang, X.-S. Xue, X. Li and J.-P. Cheng, J. Org. Chem., 2014, 79, 4340 CrossRef CAS PubMed;
(j) Z. Li, X. Li, X. Ni and J.-P. Cheng, Org. Lett., 2015, 17, 1196 CrossRef CAS PubMed;
(k) X. Ni, X. Li and J.-P. Cheng, Org. Chem. Front., 2016, 3, 170 RSC.
-
(a) W. S. Matthews, J. E. Bares, J. E. Bartmess, F. G. Bordwell, F. J. Cornforth, G. E. Drucker, Z. Margolin, R. J. McCallum, G. J. McCollum and N. R. Vanier, J. Am. Chem. Soc., 1975, 97, 7006 CrossRef CAS;
(b) F. G. Bordwell, Acc. Chem. Res., 1988, 21, 456 CrossRef CAS.
-
(a) M. K. Chantooni Jr. and I. M. Kolthoff, J. Phys. Chem., 1976, 80, 1306 CrossRef;
(b) F. G. Bordwell and A. H. Clemens, J. Org. Chem., 1982, 47, 2510 CrossRef CAS;
(c) J.-P. Cheng, B. Liu, Y.-Y. Zhao, Y.-K. Sun, X.-M. Zhang and Y. Lu, J. Org. Chem., 1999, 64, 604 CrossRef CAS;
(d) Internet Bond-Energy Databank Home Page: http://ibond.nankai.edu.cn (accessed Nov 14, 2015).
- A value of 13.06 was obtained from the measurement against 9-(2-Cl-Ph)-NHN-FH, which agrees well with the result listed in Table 1.
- F. G. Bordwell and J.-P. Cheng, J. Am. Chem. Soc., 1991, 113, 1736 CrossRef CAS.
-
(a) W. F. Baitinger Jr., P. R. von Schleyer and K. Mislow, J. Am. Chem. Soc., 1965, 87, 3168 CrossRef;
(b) K. Nakao, Y. Kiogoku and H. Sugeta, Faraday Discuss., 1994, 99, 77 RSC;
(c) N. Biliškov and G. Baranovic, J. Phys. Chem. B, 2008, 112, 10638 CrossRef PubMed.
- For selective examples, see:
(a) H. C. Aspinall, J. F. Bickley, J. L. M. Dwyer, N. Greeves and A. Steiner, Angew. Chem., Int. Ed., 2000, 39, 2858 CrossRef CAS;
(b) K. Ohmatsu, M. Ito, T. Kunieda and T. Ooi, Nat. Chem., 2012, 4, 473 CrossRef CAS PubMed;
(c) G.-Q. Zhang and Q. Li, Supramol. Chem., 2014, 26, 817 CrossRef CAS;
(d) J. R. Robinson, J. Gu, P. J. Carroll, E. J. Schelter and P. J. Walsh, J. Am. Chem. Soc., 2015, 137, 7135 CrossRef CAS PubMed.
-
(a) H. C. Brown and A. Cahn, J. Am. Chem. Soc., 1950, 72, 2939 CrossRef CAS;
(b) F. G. Bordwell and X.-M. Zhang, J. Phys. Org. Chem., 1995, 8, 529 CrossRef CAS;
(c)
M. S. Newman, Steric Effects in Organic Chemistry, John Wiley and Sons Inc., New York, 1957 Search PubMed.
- M. T. Tribble and J. G. Traynham, J. Am. Chem. Soc., 1969, 91, 379 CrossRef CAS.
-
(a) T. R. Wu, L.-X. Shen and J. M. Chong, Org. Lett., 2004, 6, 2701 CrossRef CAS PubMed;
(b) M. Turlington, A. M. DeBerardinis and L. Pu, Org. Lett., 2009, 11, 2441 CrossRef CAS PubMed;
(c) Y.-H. Liu, L.-H. Chen and M. Shi, Adv. Synth. Catal., 2006, 348, 973 CrossRef CAS.
Footnote |
† Electronic supplementary information (ESI) available: UV/Vis spectra of BINOL type phenolic catalysts in DMSO (Fig. S1–S13). See DOI: 10.1039/c6qo00252h |
|
This journal is © the Partner Organisations 2016 |
Click here to see how this site uses Cookies. View our privacy policy here.