Effective removal of Pb(II) from synthetic wastewater using Ti3C2Tx MXene†
Received
19th July 2019
, Accepted 20th November 2019
First published on 20th November 2019
Abstract
MXene (Ti3C2Tx) was used to remove heavy metals from aqueous solutions; we focused principally on Pb(II) adsorption by MXene using several performance tests and various forms of characterization. Powder activated carbon (PAC) served as the control material of MXene. We used scanning electron microscopy, porosimetry, and a zeta potential analyzer to evaluate the intrinsic properties of MXene and PAC. Although the surface area of MXene was ∼50-fold less than that of PAC, MXene exhibited better adsorption because of its higher negative surface charge. We systematically evaluated MXene-mediated Pb(II) adsorption both kinetically and isothermally, and found that adsorption was well-explained by a pseudo-second-order kinetic and the Freundlich isotherm models, respectively. Notably, attainment of equilibrium within 30 min is valuable when MXene serves as an adsorbent. The principal Pb(II) adsorption mechanism in play was electrostatic attraction, as revealed by experimental results using solutions of different pH levels, ionic strengths, and humic acid concentrations. MXene exhibited good adsorption of four different heavy metals [Pb(II), Cu(II), Zn(II), and Cd(II)] from single-electrolyte solutions. The Pb(II) adsorption mechanisms in play were ion exchange and inner-sphere complex formation, as revealed by Fourier-transform infrared spectroscopy and X-ray photoelectron spectroscopy. Additionally, MXene exhibited good re-usability over four cycles of adsorption/desorption. Therefore, MXene may be very valuable when heavy metals must be removed from wastewater, judging from good adsorption properties and re-usability.
Water impact
MXene, as a new nanomaterial, has attracted much attention due to its potential environmental applications. The novelty of our work is that we comprehensively explored whether an MXene can efficient adsorb Pb(II) from water. The results confirmed that MXene is practical in water and wastewater treatment.
|
1. Introduction
Wastewater treatment, including heavy metal removal, is recognized as an important environmental issue. Even low concentrations of heavy metals [Pb(II), Cu(II), Zn(II), and Cd(II)] can cause serious health problems (e.g., dyspnea, nervous system damage, and kidney issues) and these heavy metals are not amenable to biological degradation.1,2 The US Environmental Protection Agency and the World Health Organization (WHO) permit only very low concentrations of heavy metals in drinking water.3 Hence, efficient removal of heavy metals, particularly Pb(II), from wastewater has attracted significant interest.4 The several available physical, chemical, and biological treatments include flocculation,5 coagulation via ferrate peroxidation,6 precipitation using the 1,3-benzenediamidoethanethiol dianion,7 adsorption,2 biosorption onto pine cone powder,8 ion exchange using Fe(III)-modified zeolite,9 extraction into nitrobenzene,10 filtration through acetate membranes,11 and bioreactor removal.12 Adsorptive processes have been widely used to remove heavy metals from water; these technologies are economical, simple, regenerative, environmentally friendly, and inexpensive.13,14 Adsorption of Pb(II) generally features electrostatic interactions,2,15 so useful adsorbents must exhibit highly negative surfaces.
The MXenes are a new family of two-dimensional materials similar to graphene.3 They are fabricated via chemical etching, thus exfoliation of IVA- or IIIA-element-based layers from transition metal carbides.13 MXenes exhibit high-level hydrophilicity, chemical stability, tunable chemistry, and negative surface charges.16 Very recently, the unique intrinsic properties of MXene-based materials have been exploited to adsorb heavy metals (and other materials) from wastewater.1,3,13,15,16 MXene-based nanomaterials efficiently adsorbed Pb(II) under several experimental conditions (various pH values and different temperatures), as revealed by kinetic and isothermal data.15 However, to the best of our knowledge, no study has explored Pb(II) adsorption by MXene under the following experimental feed solution conditions: (i) in the presence of various background ions (e.g., NaCl, KCl, CaCl2, and MgCl2) at different concentrations (e.g., 50, 250, and 1000 μS cm−1); (ii) in the presence of humic acids (HAs) (dissolved organic matter); or (iii) in the presence of one or more other heavy metals [e.g., Cu(II), Zn(II), and Cd(II)]. Such experimental conditions are important from both engineering and practical perspectives.
The novelty of our work is that we comprehensively explored whether an MXene can efficient adsorb Pb(II) from water. We focused on the adsorption mechanism in play and performed meaningful experiments: we varied the MXene dose, the initial Pb(II) concentration, the solution temperature and pH, the background ion/HA levels in the feed solution, and the levels of other heavy metals [i.e., Cu(II), Zn(II), and Cd(II)]. We performed kinetic experiments, and then used Fourier-transform infrared spectroscopy (FTIR) to define the chemical bonds in play and X-ray photoelectron spectroscopy (XPS) to clarify how MXene adsorbed Pb(II). Finally, we confirmed the re-usability of MXene by performing four cycles of adsorption/desorption. The results confirmed that MXene is both practical and economical.
2. Materials and methods
2.1. Materials and chemicals
The adsorbents MXene (Ti3C2Tx) and PAC were purchased from Advanced Ceramic Esoterica (Hangzhou, Zhejiang, China) and Evoqua Water Technologies (Pittsburgh, PA, USA), respectively. NaCl, KCl, CaCl2, MgCl2, Pb(NO3)2, CuSO4, Zn(NO3)2, CdSO4, HA, HCl, and NaOH were obtained from Sigma-Aldrich (St. Louis, MO, USA). Deionized water (DI) was used to prepare all solutions.
2.2. Experimental conditions for adsorption and regeneration tests
We initially measured heavy metal concentrations via inductively coupled plasma-mass spectrometry (ICP-MS; model 7900; Agilent, CA, USA), as in other studies.2,17 The standard experimental conditions featured 40 mL of solution in a Falcon tube with 50 mg L−1 of adsorbents and 2 mg L−1 of a heavy metal at pH 6 for 2 h. We varied the experimental factors as follows: (i) adsorbent dosage (5, 10, 25, and 50 mg L−1); (ii) Pb(II) concentration (1, 1.5, 2, 2.5, 5, 6.5, and 8 mg L−1); (iii) exposure time (0, 10, 20, 30, 60, 120, 240, 480, and 1440 min); (iv) solution temperature (293, 303, and 313 K); (v) solution pH (2, 3, 4, 5, and 6); (vi) background ions (NaCl, KCl, CaCl2, and MgCl2 at different concentrations, thus 50, 250, and 1000 μS cm−1); (vii) HA concentration (2.5 and 10 mg L−1); and (viii) the presence of other heavy metals [Cu(II), Zn(II), and Cd(II)] at 2 mg L−1 both alone and mixed. All preparations were filtered through 0.2 μm-pore-sized syringe filters prior to ICP-MS.18
MXene regeneration tests were performed; 1 N HCl served as the regenerating solution.1 Used MXene was soaked in 1 N HCl for 4 h (the MXene and acid were physically separated by a 0.2 μm-pore sized membrane filter), followed by rinsing by DI water. The MXene was then re-tested under the same experimental conditions, and performed four adsorption/desorption cycles.
2.3. Data analysis for isotherms, kinetics, thermodynamics, and selectivity studies
Eqn (1) to (3) were used to analyze heavy metal adsorption; they reflect the adsorption capacity at a fixed time (qt, mg g−1), the adsorption capacity at equilibrium (qe, mg g−1), and the adsorption efficiency (E, %), respectively: | 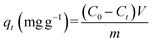 | (1) |
| 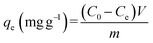 | (2) |
| 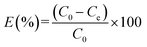 | (3) |
where C0, Ct, and Ce (mg L−1) are the heavy metal concentrations at the initial, fixed, and equilibrium times, respectively; V is the solution volume (L), and m is the weight (g) of adsorbents in solution.
We used the Langmuir and Freundlich isotherm models for the isothermal studies, and used Elovich, intra-particle, pseudo-first-order, and pseudo-second-order kinetic models for the kinetic studies.2
We obtained the adsorption selectivity (αAB) from a previous study,19 and used this parameter to compare the adsorptions of two different ions [eqn (4)]. The selectivities of single or mixed electrolyte solutions were evaluated (thus, we assayed the ideal and mixture selectivities, respectively):
|  | (4) |
where
EA is the adsorption efficiency of the A ion and
EB is the adsorption efficiency of B ion.
Pb(II) adsorption by MXene was evaluated at different solution temperatures (293, 303, and 313 K), and thermodynamic factors were calculated using the Gibbs equation, as in a previous study.2
2.4. Characterization of adsorbents
The surface microstructures of MXene and PAC were examined by scanning electron microscopy (SEM; S-4200; Hitachi, Tokyo, Japan) as described previously.19,20 The nitrogen adsorption/desorption isotherms of both adsorbents were analyzed porosimetrically (Quadrasorb SI; Quantachrome, FL, USA); this yielded adsorbent surface areas using Brunauer–Emmett–Teller (BET) analysis.21 Surface charges in 100 mg L−1 MXene and PAC were assessed using a zeta potential analyzer (ZetaPals; Brookhaven Instruments Corporation, NY, USA) over the pH range of 2–6 (adjusted using 1 N HCl), referred from previous studies.22,23 Powder X-ray diffraction (XRD) was utilized as a confirmation of crystallinity of MXene, referred to a previous study.24 To explore how Pb(II) became adsorbed onto MXene, we used FTIR to obtain infrared MXene spectra (Thermo Nicolet; Madison, WI, USA).25 We also derived MXene elemental data using XPS (PHI Quantera SXM; ULVAC-PHI, Inc., Osaka, Japan).26
3. Results and discussion
3.1. Characterization of the adsorbents
The surface microstructures of MXene and PAC as revealed by SEM are shown in Fig. S1(a.1) and (b.1).† MXene features a unique, multi-nanolamellar stacking structure, because of the fabrication method employed [Fig. S1(a.1)†]; MXene is produced by etching of an aluminum layer from a precursor phase.27 PAC contains powder particles of various sizes [Fig. S1(b.1)†]. The porosimetric nitrogen adsorption/desorption isotherms yielded the adsorbent surface areas [Fig. S1(a.2) and (b.2)†]. The PAC surface area was ∼470 m2 g−1, thus much greater than the 10 m2 g−1 of MXene;28 PAC would thus afford a higher qe if the van der Waals force was the principal adsorption mechanism.29 Section 3.4 explores the mechanism of Pb(II) absorption by MXene. The surface charges of 100 mg L−1 MXene and PAC are shown in Fig. S1(a.3) and (b.3).† The isoelectric point of MXene was much lower than that of PAC, and MXene had much more negative surface charges than PAC at the same pH values. MXene would thus afford a higher qe if adsorbates were positively charged. The crystallinity structure of MXene is shown in Fig. S2;† the pattern was similar to Ti3C2Tx MXene reported previously.30
3.2. Optimum dosage, isotherm, and kinetic studies for adsorption of Pb(II)
3.2.1. Dosage of adsorbents.
The adsorbent dose affects adsorption.2 The effects of MXene and PAC levels on Pb(II) adsorption are shown in Fig. S3(a) and (b),† respectively. MXene removed Pb(II) more extensively than did PAC, although the MXene surface area was ∼50-fold less than that of PAC [Fig. S1(a.2) and (b.2)†]. Thus, Pb(II) adsorption was not a physical process, but was rather chemical in nature, given the difference in the surface charges of MXene and PAC [Fig. S1(a.3) and (b.3)†]. All subsequent experiments used MXene at 50 mg L−1; this afforded the highest Pb(II) adsorption efficiency [Fig. S3(a)†].
3.2.2. Isotherms study.
To evaluate the effects of initial Pb(II) concentration on MXene adsorption, we performed an isothermal study using different initial Pb(II) levels (1 to 8 mg L−1). Fig. S4(a)† shows that the Pb(II) adsorption efficiency was inversely proportional to qe, which is explained by the relative ratio of adsorbate to adsorbent; in other words, this ratio affects the diffusion rate of adsorbate onto the adsorbent.31 A high ratio of adsorbate to adsorbent decreases the diffusion rate; the qe value and the MXene Pb(II) adsorption efficiency thus vary inversely. The Freundlich isotherm model was more appropriate than the Langmuir model [Fig. S4(b) and (c)†]; the results were in good agreement with previous data.28,30,32
3.2.3. Kinetics study.
The Pb(II) adsorption efficiency by MXene, and the qt, were evaluated with increasing contact time [Fig. 1(a)]; the adsorption efficiency became saturated within 30 min. Thus, MXene affords very fast adsorption; equilibrium was achieved within 30 min. Fig. 1(b) to (e) show that the kinetics of Pb(II) adsorption by MXene were well-matched using a pseudo-second-order kinetic model, consistent with earlier data.3,28,32 Table S1† lists the kinetic parameters of Pb(II) adsorption by MXene afforded by four different kinetic models (the Elovich, intra-particle, pseudo-first-order, and pseudo-second-order kinetic models) using the data in Fig. 1(b) to (e). The experiments described below featured a 2 h contact time; again, equilibrium was attained within 30 min [Fig. 1(a)].
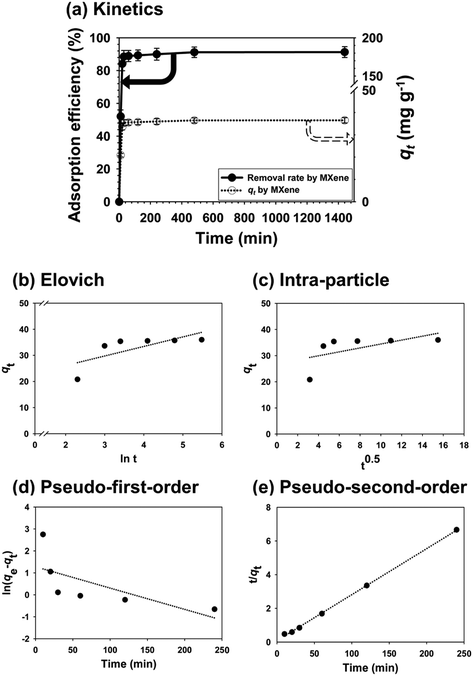 |
| Fig. 1 (a) Kinetic study of Pb(II) adsorption with increasing contact time. Four different kinetic models to explain the adsorption of Pb(II) by MXene: (b) Elovich, (c) intra-particle, (d) pseudo-first-order, and (e) pseudo-second-order diffusion kinetic model (conditions: MXene dose = 50 mg L−1; Pb2+ concentration = 2 mg L−1; temperature = 293 K; pH = 6). | |
3.3. Effect of solution factors on adsorption of Pb(II)
3.3.1. Solution temperature.
Pb(II) adsorption by MXene was studied at different solution temperatures. Fig. 2(a) shows that adsorption increased with increasing solution temperature. We used the method of a previous study to derive the thermodynamic parameters of Pb(II) adsorption by MXene,2 based on the adsorption data in Fig. 2(a). Table S2† lists these thermodynamic parameters; adsorption was spontaneous, endothermic, and associated with an increase in randomness, judging from negative ΔG, positive ΔH, and ΔS, respectively. This reflects an increased diffusion rate of Pb(II) onto MXene and a reduced boundary layer thickness of MXene as the solution temperature increased.33
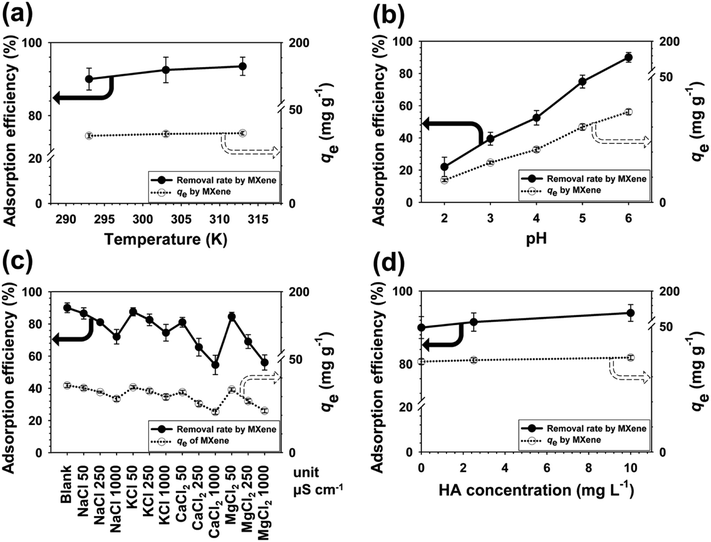 |
| Fig. 2 Influence of environmental factors on the qe and adsorption efficiency of Pb(II): (a) temperature, (b) pH, (c) background ions, and (d) HA (conditions: MXene dose = 50 mg L−1; Pb2+ concentration = 2 mg L−1; contact time = 2 h). | |
3.3.2. Solution pH.
Pb(II) adsorption by MXene was studied at different solution pH values, because these affect the charge characteristics of both the adsorbent and adsorbate, changing adsorption performance.32 Solution pH may critically affect Pb(II) adsorption by MXene. Fig. 2(b) shows adsorption by solution pH in the range pH 2 to 6; divalent cations [e.g., Pb(II)] become monovalent [i.e., PbOH(I)] or neutral [i.e., Pb(OH)2] at pH values greater than 7.15 Pb(II) adsorption increased continuously as the solution pH rose [Fig. 2(b)], reflecting the effects of pH on surface charge [Fig. S1(a.3)†] and supporting the suggestion that Pb(II) adsorption by MXene is electrostatic in nature (section 3.2.1).
3.3.3. Background ions.
A previous study found that salts in feed solutions inhibited electrostatic interactions because of a screening effect.34 It was thus important to investigate the effects of background ions on Pb(II) adsorption. Fig. 2(c) shows the effects of various background ions [Na(I), K(I), Ca(II), and Mg(II)] on adsorption of Pb(II) by MXene in terms of the Pb(II) adsorption efficiency and qe. Adsorption decreased monotonically with increasing ionic strength of any of the cations, reflecting not only mutual screening of MXene and Pb(II), but also competition between the cations and Pb(II).2 Notably, Fig. 2(c) shows that the cations reduced Pb(II) adsorption in the following order: Ca(II) > Mg(II) > Na(I) > K(I), reflecting the hydrated cation radii; those of Pb(II), Na(I), K(I), Ca(II), and Mg(II) are 4.01, 3.58, 3.31, 4.12, and 4.28 nm, respectively.35 Thus, the extent of competition between Pb(II) and other cations reflected how similar the hydrated radii of the cations were to that of hydrated Pb(II). The radius of hydrated Ca(II) is closest to that of hydrated Pb(II); Ca(II) thus maximally reduced Pb(II) adsorption. Therefore, background ions in feed solution significantly affect Pb(II) adsorption by MXene [Fig. 2(c)].
3.3.4. Humic acids (HA).
HA is a representative form of dissolved organic matter. It features both aromatic and carboxylic substituents36 and behaves as a multivalent anion after deprotonation of the latter substituents.37Fig. 2(d) shows the effects of HA on adsorption of Pb(II) by MXene; the Pb(II) adsorption efficiency and qe increased with increasing HA concentration in the feed solution. HA affected the electrostatic interaction between Pb(II) and MXene. HA exists as divalent anions at pH 6, as revealed by its five different pKa values.38 One study found that adsorption of perfluorooctanoic acid (PFOA) by MOF increased when Ca(II) was present in the feed solution.39 Monovalent PFOA anions bind to divalent cationic Ca(II), thus serving to bridge the anions. Similarly, divalent Pb(II) may bind the divalent HA anion, creating a bridge effect that enhances Pb(II) absorption by MXene. The data also support the idea that electrostatic interaction plays a major role in Pb(II) adsorption by MXene.
3.4. Proposed mechanism of Pb(II) adsorption in this study
We used XPS and FTIR to explore adsorption of Pb(II) onto MXene. Fig. 3(a) shows wide-scan XPS spectra for MXene and Pb(II) adsorbed onto MXene; Pb(II) was clearly well-adsorbed. MXene consists of Na, O, C, F, and Ti elements as shown in Fig. 3(a). We used the Na 1s, Pb 4f, O 1s, and Ti 2p peaks to study the adsorption mechanism in detail. Fig. 3(b) shows that after Pb(II) adsorption to MXene, the Na 1s peak shifted to a higher binding energy, with loss of peak area, reflecting ionic exchange between Pb(II) and Na(I).40 Also, the Pb 4f peak shifted to a higher binding energy after Pb(II) adsorption onto MXene, reflecting the strong binding affinity between Pb(II) and MXene [Fig. 3(c)], similar to what was found in a previous study exploring Hg(II) uptake by a titanium carbide magnetic nanocomposite.1Fig. 3(d) and (e) support our interpretation of the Pb 4f data [Fig. 3(c)]: O 1s and Ti 2p peak shifts were also evident after Pb(II) adsorption onto MXene. Notably, the Ti–OH peak area [Fig. 3(d)] decreased after Pb(II) adsorption onto MXene, reflecting inner surface complex formation between MXene and Pb(II).41 An FTIR Ti–O peak shift after adsorption of Pb(II) onto MXene was apparent (Fig. S5†), consistent with the XPS data. Fig. S6† includes a schematic of the mechanism by which Pb(II) adsorbs to MXene.
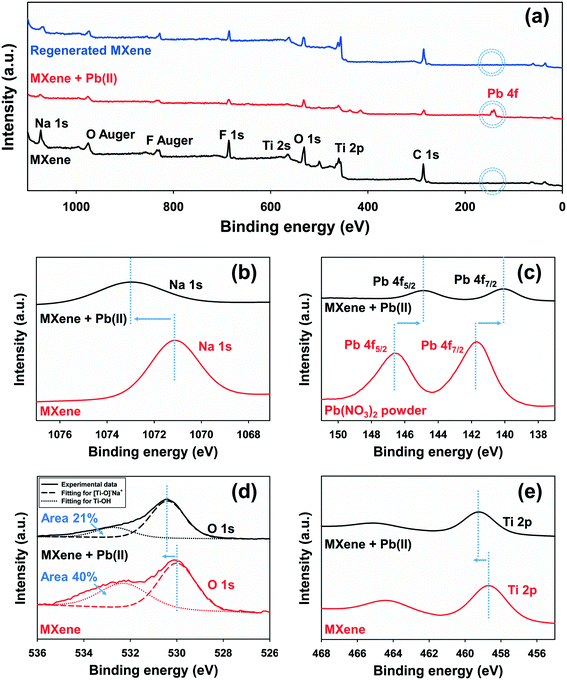 |
| Fig. 3 XPS spectra related to Pb(II) adsorption by MXene: (a) wide scan, (b) Na 1s, (c) Pb 4f, (d) O 1s, and (e) Ti 2p. | |
3.5. Selectivity of heavy metals
It was appropriate (in practical terms) to explore whether MXene could adsorb other heavy metals; we evaluated adsorption of Cu(II), Zn(II), and Cd(II) (alone or mixed in the feed solution) by MXene. The adsorption efficiency and qe values of the four heavy metals are shown in Fig. 4; all were efficiently (over 80%) removed by MXene from single-electrolyte solutions. MXene can thus adsorb a variety of heavy metals from wastewater. We then compared the adsorptions of Pb(II) and other heavy metals using mixed electrolyte solutions (Fig. 4). Compared to the single-electrolyte solutions, MXene preferentially adsorbed heavy metals in the order Pb(II) > Cu(II) > Zn(II) > Cd(II). This reflects the gradation in metallic electronegativity; MXene adsorbs Pb(II) electrostatically. The metallic electronegativities of Pb, Cu, Zn, and Cd are 1.80, 1.90, 1.65, and 1.69, respectively. Thus, the adsorption efficiency of Pb and Cu are much higher than those of Zn and Cd. Furthermore, the adsorption efficiency also reflect the properties of the metal hydroxides; the heavy metal hydroxide constants (log
K values) in play when cations change from divalent to monovalent affect the adsorption efficiency of the four different heavy metals.42 Log
K values of Pb, Cu, Zn, and Cd are 7.8, 7.0, 4.4, and 4.2, respectively.2 In other words, weakening of the affinity between MXene and a heavy metal, as reflected by the log
K value, affects adsorption performance when the electrolytes are in mixed solution. Table 1 summarizes the selectivities toward the four different heavy metals when MXene was used to adsorb the metals from single- or mixed-electrolyte solutions; the results are consistent with the trends in both metal electronegativity and the log
K value.
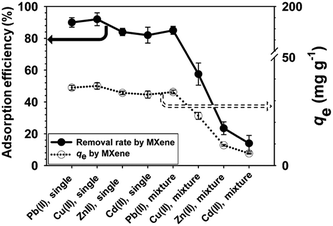 |
| Fig. 4 Adsorption performances of various heavy metals by MXene in single or mixture solutions (conditions: MXene dose = 50 mg L−1; heavy metal concentration of each single or mixture electrolyte solution = 2 mg L−1; temperature = 293 K; pH = 6; contact time = 2 h). | |
Table 1 Results for ideal or mixture selectivity of various heavy metals by MXene
α
AB (A/B) |
Ideal selectivity |
Mixture selectivity |
Cd(II)/Pb(II) |
1.8 ± 0.10 |
5.7 ± 0.32 |
Cd(II)/Cu(II) |
2.3 ± 0.14 |
2.0 ± 0.17 |
Zn(II)/Cd(II) |
1.1 ± 0.06 |
1.1 ± 0.07 |
Zn(II)/Pb(II) |
1.6 ± 0.06 |
5.1 ± 0.24 |
Zn(II)/Cu(II) |
2.0 ± 0.09 |
1.8 ± 0.15 |
Cu(II)/Pb(II) |
0.8 ± 0.04 |
2.8 ± 0.20 |
3.6. Re-usability testing
Adsorbent re-usability is of major practical importance;43 we evaluated re-usability in terms of Pb(II) adsorption over four adsorption/desorption cycles (Fig. S7†). We used 1 N HCl as the regeneration solution.1 As shown in Fig. 3(a), MXene was clearly regenerated, based on the disappearance of the Pb 4f peak. MXene also exhibited good re-usability (Fig. S7†): the Pb(II) adsorption efficiency was better than that from PAC after four adsorption/desorption cycles. Thus, MXene can be efficiently and economically used to treat wastewater containing heavy metals [e.g., Pb(II)].
4. Conclusions
We used SEM, BET, and zeta potential analyses to study the physicochemical properties of PAC and MXene. We focused on how efficiently MXene adsorbed heavy metals [e.g., Pb(II), Cu(II), Zn(II), and Cd(II)] and studied the adsorption mechanism in play using several approaches and techniques (i.e., FTIR and XPS). MXene exhibited better Pb(II) adsorption than did PAC; MXene had a more negative surface charge at the same pH. Also, the MXene adsorption rate was very rapid; equilibrium was attained within 30 min. Isothermal studies revealed that the Freundlich model was more appropriate than the Langmuir model. Thermodynamically, Pb(II) adsorption by MXene was under the spontaneous process, as revealed by the increase in qe as solution temperature increased. The solution pH, ionic strength, and HA concentration critically affected adsorption performance; adsorption of Pb(II) by MXene was electrostatic in nature. Both ionic exchange and inner-sphere complex formation were important in this context, as revealed by XPS and FTIR. MXene efficiently adsorbed four different heavy metals [Pb(II), Cu(II), Zn(II), and Cd(II)] when they were present alone in model wastewater. MXene exhibited good re-usability over at least four cycles of adsorption/desorption. Thus, MXene, a highly efficient adsorbent, can be used to remove heavy metals from wastewater.
Conflicts of interest
There are no conflicts to declare.
Acknowledgements
This research was funded by the Korea Ministry of Environment (The SEM projects; 2018002470005, South Korea).
References
- A. Shahzad, K. Rasool, W. Miran, M. Nawaz, J. Jang, K. A. Mahmoud and D. S. Lee, Mercuric ion capturing by recoverable titanium carbide magnetic nanocomposite, J. Hazard. Mater., 2018, 344, 811–818 CrossRef CAS PubMed.
- B.-M. Jun, S. Kim, Y. Kim, N. Her, J. Heo, J. Han, M. Jang, C. M. Park and Y. Yoon, Comprehensive evaluation on removal of lead by graphene oxide and metal organic framework, Chemosphere, 2019, 231, 82–92 CrossRef CAS PubMed.
- A. Shahzad, K. Rasool, W. Miran, M. Nawaz, J. Jang, K. A. Mahmoud and D. S. Lee, Two-Dimensional Ti3C2Tx MXene Nanosheets for Efficient Copper Removal from Water, ACS Sustainable Chem. Eng., 2017, 5, 11481–11488 CrossRef CAS.
- L. Joseph, B.-M. Jun, J. R. V. Flora, C. M. Park and Y. Yoon, Removal of heavy metals from water sources in the developing world using low-cost materials: A review, Chemosphere, 2019, 229, 142–159 CrossRef CAS PubMed.
- A. R. Karbassi and S. Nadjafpour, Flocculation of dissolved Pb, Cu, Zn and Mn during estuarine mixing of river water with the Caspian Sea, Environ. Pollut., 1996, 93, 257–260 CrossRef CAS.
- J. Ma, W. Liu, Y. Zhang and C. Li, Enhanced removal of cadmium and lead from water by ferrate preoxidation in the process of coagulation, ACS Symp. Ser., 2008, 985, 456–465 CrossRef CAS.
- M. M. Matlock, B. S. Howerton and D. A. Atwood, Chemical precipitation of lead from lead battery recycling plant wastewater, Ind. Eng. Chem. Res., 2002, 41, 1579–1582 CrossRef CAS.
- A. E. Ofomaja, E. B. Naidoo and S. J. Modise, Biosorption of copper(II) and lead(II) onto potassium hydroxide treated pine cone powder, J. Environ. Manage., 2010, 91, 1674–1685 CrossRef CAS PubMed.
- M. Kragović, A. Daković, M. Marković, J. Krstić, G. D. Gatta and N. Rotiroti, Characterization of lead sorption by the natural and Fe(III)-modified zeolite, Appl. Surf. Sci., 2013, 283, 764–774 CrossRef.
- E. Makrlík and P. Vaňura, Solvent extraction of lead using a nitrobenzene solution of strontium dicarbollylcobaltate in the presence of polyethylene glycol PEG 400, J. Radioanal. Nucl. Chem., 2005, 267, 233–235 CrossRef.
- M. Soylak, Y. E. Unsal, N. Kizil and A. Aydin, Utilization of membrane filtration for preconcentration and determination of Cu(II) and Pb(II) in food, water and geological samples by atomic absorption spectrometry, Food Chem. Toxicol., 2010, 48, 517–521 CrossRef CAS PubMed.
- A. Oehmen, D. Vergel, J. Fradinho, M. A. M. Reis, J. G. Crespo and S. Velizarov, Mercury removal from water streams through the ion exchange membrane bioreactor concept, J. Hazard. Mater., 2014, 264, 65–70 CrossRef CAS PubMed.
- X. Dong, Y. Wang, M. Jia, Z. Niu, J. Cai, X. Yu, X. Ke, J. Yao and X. Zhang, Sustainable and scalable in-situ synthesis of hydrochar-wrapped Ti3AlC2-derived nanofibers as adsorbents to remove heavy metals, Bioresour. Technol., 2019, 282, 222–227 CrossRef CAS PubMed.
- L. Joseph, B.-M. Jun, M. Jang, C. M. Park, J. C. Muñoz-Senmache, A. J. Hernández-Maldonado, A. Heyden, M. Yu and Y. Yoon, Removal of contaminants of emerging concern by metal-organic framework nanoadsorbents: A review, Chem. Eng. J., 2019, 369, 928–946 CrossRef CAS.
- P. Gu, J. Xing, T. Wen, R. Zhang, J. Wang, G. Zhao, T. Hayat, Y. Ai, Z. Lin and X. Wang, Experimental and theoretical calculation investigation on efficient Pb(II) adsorption on etched Ti3AlC2 nanofibers and nanosheets, Environ. Sci.: Nano, 2018, 5, 946–955 RSC.
- B.-M. Jun, S. Kim, J. Heo, C. M. Park, N. Her, M. Jang, Y. Huang, J. Han and Y. Yoon, Review of MXenes as new nanomaterials for energy storage/delivery and selected environmental applications, Nano Res., 2019, 12, 471–487 CrossRef CAS.
- S.-W. Han, W. Kim, Y. Lee, B.-M. Jun and Y.-N. Kwon, Investigation of Hydrate-induced Ice Desalination (HIID) and its application to a pretreatment of reverse osmosis (RO) process, Desalination, 2016, 395, 8–16 CrossRef CAS.
- B.-M. Jun, S. Kim, J. Heo, N. Her, M. Jang, C. M. Park and Y. Yoon, Enhanced sonocatalytic degradation of carbamazepine and salicylic acid using a metal-organic framework, Ultrason. Sonochem., 2019, 56, 174–182 CrossRef CAS PubMed.
- B.-M. Jun, H. K. Lee and Y.-N. Kwon, Acid-catalyzed hydrolysis of semi-aromatic polyamide NF membrane and its application to water softening and antibiotics enrichment, Chem. Eng. J., 2018, 332, 419–430 CrossRef CAS.
- B.-M. Jun, H. K. Lee, Y.-I. Park and Y.-N. Kwon, Degradation of full aromatic polyamide NF membrane by sulfuric acid and hydrogen halides: Change of the surface/permeability properties, Polym. Degrad. Stab., 2019, 162, 1–11 CrossRef CAS.
- J. Han, B.-M. Jun, J. Heo, S. Kim, Y. Yoon and C. M. Park, Heterogeneous sonocatalytic degradation of an anionic dye in aqueous solution using a magnetic lanthanum dioxide carbonate-doped zinc ferrite-reduced graphene oxide nanostructure, Ecotoxicol. Environ. Saf., 2019, 182, 109396–109404 CrossRef CAS PubMed.
- B.-M. Jun, S. H. Kim, S. K. Kwak and Y.-N. Kwon, Effect of acidic aqueous solution on chemical and physical properties of polyamide NF membranes, Appl. Surf. Sci., 2018, 444, 387–398 CrossRef CAS.
- B.-M. Jun, T. P. N. Nguyen, S.-H. Ahn, I.-C. Kim and Y.-N. Kwon, The application of polyethyleneimine draw solution in a combined forward osmosis/nanofiltration system, J. Appl. Polym. Sci., 2015, 132, 42198–42206 CrossRef.
- B.-M. Jun, J. Heo, N. Taheri-Qazvini, C. M. Park and Y. Yoon, Adsorption of selected dyes on Ti3C2Tx MXene and Al-based metal-organic framework, Ceram. Int., 2019 DOI:10.1016/j.ceramint.2019.09.293 , in press.
- B.-M. Jun, T. P. N. Nguyen, Y.-K. Kim, H. K. Lee and Y.-N. Kwon, Surface modification of TFC FO membrane using N-isopropylacrylamide (NIPAM) to enhance fouling resistance and cleaning efficiency, Desalin. Water Treat., 2017, 65, 11–21 CrossRef CAS.
- T. P. N. Nguyen, B.-M. Jun and Y.-N. Kwon, The chlorination mechanism of integrally asymmetric cellulose triacetate (CTA)-based and thin film composite polyamide-based forward osmosis membrane, J. Membr. Sci., 2017, 523, 111–121 CrossRef CAS.
- A. A. Alqadami, M. Naushad, Z. A. Alothman and T. Ahamad, Adsorptive performance of MOF nanocomposite for methylene blue and malachite green dyes: Kinetics, isotherm and mechanism, J. Environ. Manage., 2018, 223, 29–36 CrossRef CAS PubMed.
- A. K. Fard, G. McKay, R. Chamoun, T. Rhadfi, H. Preud'Homme and M. A. Atieh, Barium removal from synthetic natural and produced water using MXene as two dimensional (2-D) nanosheet adsorbent, Chem. Eng. J., 2017, 317, 331–342 CrossRef CAS.
- Z. Hasan and S. H. Jhung, Removal of hazardous organics from water using metal-organic frameworks (MOFs): Plausible mechanisms for selective adsorptions, J. Hazard. Mater., 2015, 283, 329–339 CrossRef CAS PubMed.
- O. Mashtalir, K. M. Cook, V. N. Mochalin, M. Crowe, M. W. Barsoum and Y. Gogotsi, Dye adsorption and decomposition on two-dimensional titanium carbide in aqueous media, J. Mater. Chem. A, 2014, 2, 14334–14338 RSC.
- B.-M. Jun, J. Heo, C. M. Park and Y. Yoon, Comprehensive evaluation of the removal mechanism of carbamazepine and ibuprofen by metal organic framework, Chemosphere, 2019, 235, 527–537 CrossRef CAS PubMed.
- S. Li, L. Wang, J. Peng, M. Zhai and W. Shi, Efficient thorium(IV) removal by two-dimensional Ti2CTx MXene from aqueous solution, Chem. Eng. J., 2019, 366, 192–199 CrossRef CAS.
- S. M. Mirsoleimani-azizi, P. Setoodeh, S. Zeinali and M. R. Rahimpour, Tetracycline antibiotic removal from aqueous solutions by MOF-5: Adsorption isotherm, kinetic and thermodynamic studies, J. Environ. Chem. Eng., 2018, 6, 6118–6130 CrossRef CAS.
- Y.-H. Fan, S.-W. Zhang, S.-B. Qin, X.-S. Li and S.-H. Qi, An enhanced adsorption of organic dyes onto NH2 functionalization titanium-based metal-organic frameworks and the mechanism investigation, Microporous Mesoporous Mater., 2018, 263, 120–127 CrossRef CAS.
- E. R. Nightingale, Phenomenological theory of ion solvation. Effective radii of hydrated ions, J. Phys. Chem., 1959, 63, 1381–1387 CrossRef CAS.
- A. Bhatnagar and M. Sillanpää, Removal of natural organic matter (NOM) and its constituents from water by adsorption – A review, Chemosphere, 2017, 166, 497–510 CrossRef CAS PubMed.
- B.-M. Jun, H. S. Hwang, J. Heo, J. Han, M. Jang, J. Sohn, C. M. Park and Y. Yoon, Removal of selected endocrine-disrupting compounds using Al-based metal organic framework: Performance and mechanism of competitive adsorption, J. Ind. Eng. Chem., 2019, 79, 345–352 CrossRef CAS.
- C. P. Jordão, R. B. A. Fernandes, K. de Lima Ribeiro, B. de Souza Nascimento and P. M. de Barros, Zn(II) adsorption from synthetic solution and kaolin wastewater onto vermicompost, J. Hazard. Mater., 2009, 162, 804–811 CrossRef PubMed.
- L. Liu, D. Li, C. Li, R. Ji and X. Tian, Metal nanoparticles by doping carbon nanotubes improved the sorption of perfluorooctanoic acid, J. Hazard. Mater., 2018, 351, 206–214 CrossRef CAS PubMed.
- F. Meng, Z. Hong, J. Arndt, M. Li, M. Zhi, F. Yang and N. Wu, Visible light photocatalytic activity of nitrogen-doped La2Ti2O7 nanosheets originating from band gap narrowing, Nano Res., 2012, 5, 213–221 CrossRef CAS.
- S. Zhao, D. Chen, F. Wei, N. Chen, Z. Liang and Y. Luo, Removal of Congo red dye from aqueous solution with nickel-based metal-organic framework/graphene oxide composites prepared by ultrasonic wave-assisted ball milling, Ultrason. Sonochem., 2017, 39, 845–852 CrossRef CAS PubMed.
- R. Sitko, E. Turek, B. Zawisza, E. Malicka, E. Talik, J. Heimann, A. Gagor, B. Feist and R. Wrzalik, Adsorption of divalent metal ions from aqueous solutions using graphene oxide, Dalton Trans., 2013, 42, 5682–5689 RSC.
- J. Han, B.-M. Jun, J. Heo, G. Lee, Y. Yoon and C. M. Park, Highly efficient organic dye removal from waters by magnetically recoverable La2O2CO3/ZnFe2O4-reduced graphene oxide nanohybrid, Ceram. Int., 2019, 45, 19247–19256 CrossRef CAS.
Footnote |
† Electronic supplementary information (ESI) available. See DOI: 10.1039/c9ew00625g |
|
This journal is © The Royal Society of Chemistry 2020 |