DOI:
10.1039/C8SC02101E
(Edge Article)
Chem. Sci., 2018,
9, 6839-6843
Nickel(0)-catalyzed linear-selective hydroarylation of unactivated alkenes and styrenes with aryl boronic acids†
Received
11th May 2018
, Accepted 17th July 2018
First published on 18th July 2018
Abstract
Herein, we describe the first linear-selective hydroarylation reaction of unactivated alkenes and styrenes with aryl boronic acids, which was achieved by introducing a directing group on the alkenes. This efficient, scalable reaction serves as a method for modular assembly of structurally diverse alkyl arenes, including γ-aryl butyric acid derivatives, which are widely utilized as chemical building blocks for the synthesis of various drugs and other biologically active compounds.
Introduction
Transition-metal-catalyzed alkene hydroarylation involving metal hydrides (M–H)1 has become one of the most widely used strategies for the synthesis of alkyl arenes.2 To date, three types of aryl sources have been used for this purpose: (1) simple arenes, (2) aryl halides, and (3) aryl metals (i.e., aryl stannanes or boronic acids). The simple arenes are usually restricted to heteroarenes and arenes with a directing group because of the challenge of activating the inert C–H bonds of simple arenes.3 Aryl halides and aryl metals as the aryl sources require the use of O2 or an excess of a reductant (such as a silane or an alkyl halide), which limits the practicality of alkene hydroarylations with these two sources (Scheme 1a).4,5 A significant breakthrough was made by Zhou et al., who reported a highly selective Ni-catalyzed hydroarylation of styrenes and 1,3-dienes with aryl boron compounds under redox-neutral conditions (Scheme 1b).6 However, this method still suffers from some limitations: (1) the reaction is efficient only with styrene and 1,3-diene substrates, and (2) only branched products can be achieved.
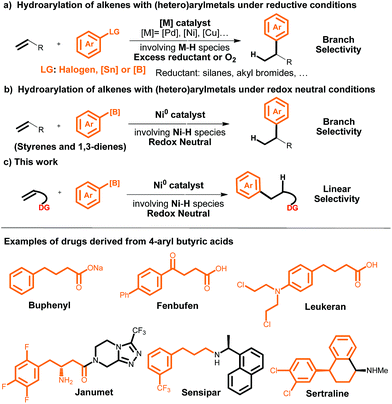 |
| Scheme 1 Transition-metal-catalyzed hydroarylation of alkenes involving M–H and examples of drugs derived from 4-aryl butyric acids. | |
Inspired by recent work on olefin functionalization reactions in which the reactivity and regioselectivity are controlled via the introduction of a coordinating group,7,8 we have developed the first method for directing-group-controlled linear-selective hydroarylation of unactivated alkenes and styrenes with aryl boronic acids under redox-neutral conditions (Scheme 1c). This efficient, scalable reaction serves as a method for modular assembly of structurally diverse alkyl arenes, including γ-aryl butyric acid derivatives, which are widely utilized as chemical building blocks for the synthesis of various drugs and other biologically active compounds (Scheme 1). γ-Aryl butyric acids are traditionally prepared by Friedel–Crafts reactions between arenes and butyrolactones,9 but this method suffers from disadvantages such as harsh reaction conditions, limited substrate scope (it is suitable only for electron-rich arenes), and poor regioselectivity.
Results and discussion
In a preliminary experiment, we found that treatment of 3-butenoic acid derivative 1a (0.2 mmol), which bears an 8-aminoquinoline (AQ) directing group, with Ni(COD)2 (5 mol%), PCy3 (10 mol%), and 2-naphthalenylboronic acid 2a (0.3 mmol) in MeOH (1 mL) at 70 °C for 24 h gave linear product 3a in 21% yield (Table 1, entry 1). Replacing MeOH with t-amyl alcohol slightly improved the yield (entry 2), and adding 1.5 equiv. of K2CO3 improved the yield even further (to 38%, entry 3). Evaluation of different bases revealed that CsOPiv was the best choice (entries 3–7). We also found that changing the 1a/2a molar ratio to 1
:
2 increased the yield to 61% (entry 8). Finally, various phosphine ligands were investigated (entries 9–12); switching from PCy3 to PPh3 dramatically improved the yield (to 85%, entry 9). A control experiment confirmed that the reaction did not occur in the absence of Ni(COD)2 (entry 13).
Table 1 Optimization of directed hydroarylation of unactivated alkene 1a with 2-naphthalenylboronic acid 2aa
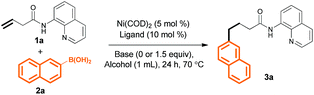
|
Entry |
Ligand |
Base |
Solvent (H source) |
Yieldb [%] |
Reactions were carried out with Ni(COD)2 (5 mol%), ligand (10 mol%), base (0 or 1.5 equiv.), 1a (0.2 mmol), and 2-naphthalenylboronic acid (2a, 0.3 mmol) in solvent (1 mL) for 48 h at 25 °C under a N2 atmosphere.
Yields were determined by NMR spectroscopy with an internal standard. NR = no reaction.
2.0 equiv. of 2a was used.
The isolated yield is given in parenthesis.
Reaction without Ni(COD)2.
|
1 |
PCy3 |
— |
MeOH |
21 |
2 |
PCy3 |
— |
t-AmylOH |
29 |
3 |
PCy3 |
K2CO3 |
t-AmylOH |
38 |
4 |
PCy3 |
KHCO3 |
t-AmylOH |
23 |
5 |
PCy3 |
K3PO4 |
t-AmylOH |
43 |
6 |
PCy3 |
NaOtBu |
t-AmylOH |
NR |
7 |
PCy3 |
CsOPiv |
t-AmylOH |
53 |
8c |
PCy3 |
CsOPiv |
t-AmylOH |
61 |
9
|
PPh
3
|
CsOPiv
|
t-AmylOH
|
86(85)
|
10c |
CyPPh2 |
CsOPiv |
t-AmylOH |
77 |
11c |
S-Phos |
CsOPiv |
t-AmylOH |
33 |
12c |
XantPhos |
CsOPiv |
t-AmylOH |
59 |
13e |
PPh3 |
CsOPiv |
t-AmylOH |
NR |
Next, we investigated the substrate scope with respect to the aryl boronic acid by carrying out reactions with 3-butenoic acid derivative 1a as the alkene (Scheme 2). To our delight, electron-rich, electron-poor, and sterically hindered aryl boronic acids all afforded the desired products in good to excellent yields (3a–3u). Heteroaryl boronic acids were also reactive (3v and 3w), as was an alkenyl boronic acid, which selectively afforded (E)-6-phenylhexenoic acid derivative 3x under the optimized conditions. In addition, we found that a high yield of 3b could be obtained by hydroarylation of 1a with an aryl boroxine or an aryl boronic ester as the aryl source. It is important to stress that these reaction conditions were compatible with a remarkable variety of functional groups on the aryl boronic acids, including halogens (F, Cl, and Br) and acetyl, cyano, and methoxy groups, yielding products (3i–3s) that could be subjected to further synthetic transformations. In addition, the reaction could be carried out on gram scale without a decrease in the yield (1.5 g of 3b, 86%). The structure of 3b was confirmed by X-ray diffraction analysis of a single crystal.10
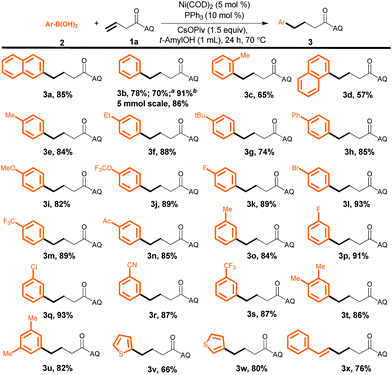 |
| Scheme 2 The reactions of diverse aryl boronic acids 2 with 3-butenoic acid derivative 1a. Yield of isolated product is given. a 5,5-Dimethyl-2-phenyl-1,3,2-dioxaborinane was used as the aryl source. b Triphenylboroxine was used as the aryl source. | |
We next evaluated the utility of this method for various unactivated alkenes (Scheme 3). Terminal alkenes bearing a single substituent at the α- or β-position reacted smoothly to afford the desired products in good to excellent yields (4a and 4b). A sterically congested α,α-disubstituted terminal alkene was also reactive, furnishing desired product 4c in moderate yield, although a higher temperature (125 °C) and a higher loading(10 mol%) of a different ligand, PhPMe2, were required. Furthermore, internal alkenes bearing a variety of substituents at the γ-position could also be efficiently converted into the corresponding products (4d–4l); the steric and electronic effects of the substituents appeared to be negligible. Notably, the yields of 4e from trans- and cis-3-hexenoic acid were essentially the same. In addition, α,γ-disubstituted alkenes gave desired products 4i and 4j. Surprisingly, a γ,γ-disubstituted alkene could also be hydroarylated to produce compound 4l (88%), which has a quaternary carbon center.
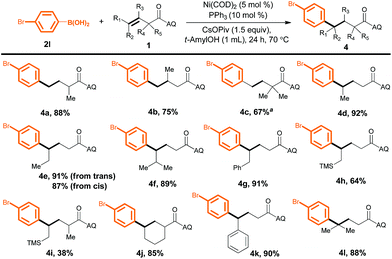 |
| Scheme 3 Reactions of unactivated alkenes with aryl boronic acid 2l. Yield of isolated product is given. a PhPMe2 (10 mol%) as the ligand at 125 °C. | |
We wondered whether the introduction of the AQ directing group at the ortho-position of styrene would result in linear selectivity rather than the branched selectivity reported by Sigman et al. and Zhou et al. for hydroarylation of styrenes with aryl metals.4,6 After extensive investigation, we found, to our delight, that reactions of styrene derivative 1m with various aryl boronic acids occurred smoothly with completely linear selectivity and in moderate yield by employment of PhPCy2 as the ligand and higher catalyst loading (10 mol% Ni(COD)2), higher temperature (125 °C), and longer reaction time (Scheme 4). This result clearly proved that the directing group controlled the regioselectivity of the hydrometalation.
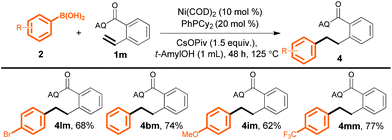 |
| Scheme 4 Reactions of styrene derivative 1m with aryl boronic acids. | |
The products obtained by the method described herein could provide access to structurally diverse synthetic building blocks by means of additional transformations (Scheme 5, upper panel). Specifically, removal of the AQ directing group of 3b smoothly provided 4-phenylbutanoic acid (5b) in 97% yield. Acid 5b could undergo intramolecular cyclization to yield tetralin-derivative 6a, or the carboxyl group could be replaced with an iodine atom (6b). Five-membered lactone 6c could be obtained by treatment of 5b with a hypervalent iodine reagent, and 5b readily underwent attack by CH3NO2 to afford corresponding ketone derivative 6d. To further demonstrate the synthetic usefulness of our method, we synthesized the drug Sensipar from 3s (Scheme 5, lower panel). First, free acid 5s was synthesized by removal of the AQ directing group, and then the acid was transformed into the corresponding alkyl iodide (6s) by treatment with NIS/I2 in DCE. Simple amination of 6s gave Sensipar in 97% yield.
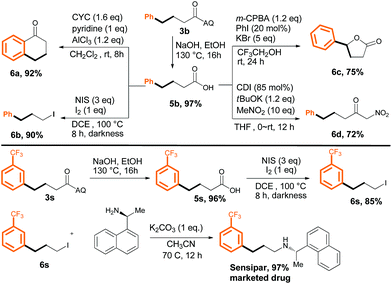 |
| Scheme 5 Transformations of hydroarylation products 3b and 3s. | |
To probe the reaction mechanism, we carried out a control experiment and a series of deuteration experiments (Scheme 6). We found that no reaction occurred when N-(naphthalenyl)butenamide 7 and 2-naphthalenylboronic acid (1a) were used as the substrates (eqn (1)). This result indicates that the presence of the AQ directing group on the unactivated alkene was indispensable. Treatment of PhB(OD)22b-D with alkene 1a in MeOH or treatment of (PhBO)3 with 1a in CD3OH gave undeuterated product 3b (eqn (2) and (3)). However, the use of MeOD as the solvent led to the formation of 3b that was only partially deuterated at both the α- and β-positions (eqn (4)), which reveals that the hydrogen atom of the Ni–H intermediate A (Scheme 7) came from the methanol O–H group and hydrometalation of Ni–H is irreversible in the presence of aryl boronic acid. Further experiment suggests that hydrometalation of starting material 1avia Ni–H species would also happen to form 5-membered nickelacycle and is reversible in the absence of aryl boronic acid under our reaction conditions (eqn (5) and (6)). Additionally, the deuteration of α-positions might be because of CsOPiv base.
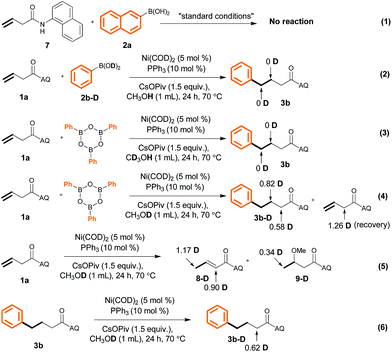 |
| Scheme 6 Control experiment and deuteration experiments. | |
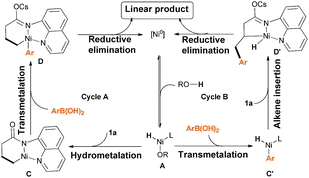 |
| Scheme 7 Proposed mechanism of the Ni(0)-catalyzed directing-group-controlled hydroarylation of unactivated alkenes with aryl boronic acids. | |
On the basis of literature reports and our own mechanistic experiments, we propose two catalytic cycles that starts with the reversible formation of Ni–H species A (Scheme 7). For path A, coordination and subsequent hydrometalation of the C
C bond affords the six-membered nickelacyclic intermediate C, and then transmetalation of the aryl boronic acid generates intermediate D.11 Finally, reductive elimination gives the desired product and regenerates the Ni(0) catalyst. Alternatively, intermediate A could also undergo transmetalation first to form Aryl-Ni–H species C′, and then alkene insertion to form five-membered nickelacyclic intermediate D′, which would involve reductive elimination to yield the same linear product. Note, that we have detected the production of naphthalene under our reaction condition. It indicated that cycle B might be the real process. However, at this stage, we still can't rule out cycle A.
Conclusions
In conclusion, we have described the first method for Ni(0)-catalyzed directing-group-controlled linear-selective hydroarylation of unactivated alkenes and styrenes with aryl boronic acids under redox-neutral conditions. Our efficient, scalable method provides a general route to structurally diverse alkyl arene derivatives, including γ-aryl butyric acid derivatives, which are widely utilized as chemical feedstocks for the preparation of drugs and other biologically active compounds.
Conflicts of interest
There are no conflicts to declare.
Acknowledgements
We are grateful for the financial support from the National Natural Science Foundation of China (21602115), 1000-Talent Youth Program (020/BF180181), the Natural Science Foundation of Tianjin (18JCYBJC20400), the Fundamental Research Funds for the Central Universities and Nankai University.
Notes and references
- For some reviews on metal-hydride chemistry, see:
(a) S. Rendler and M. Oestreich, Angew. Chem., Int. Ed., 2007, 46, 498 CrossRef PubMed;
(b) C. Deutsch, N. Krause and B. H. Lipshutz, Chem. Rev., 2008, 108, 2916 CrossRef PubMed;
(c) M. T. Pirnot, Y.-M. Wang and S. L. Buchwald, Angew. Chem., Int. Ed., 2016, 55, 48 CrossRef PubMed;
(d) M. D. Greenhalgh, A. S. Jones and S. P. Thomas, ChemCatChem, 2015, 7, 190 CrossRef;
(e) R. M. Maksymowicz, A. J. Bissette and S. P. Fletcher, Chem.–Eur. J., 2015, 21, 5668 CrossRef PubMed;
(f) S. W. M. Crossley, C. Obradors, R. M. Martinez and R. A. Shenvi, Chem. Rev., 2016, 116, 8912 CrossRef PubMed;
(g) K. D. Nguyen, B. Y. Park, T. Luong, H. Sato, V. J. Garza and M. J. Krische, Science, 2016, 354, 300 CrossRef PubMed.
- For some reviews on hydroarylation of alkenes, see:
(a) F. Kakiuchi and S. Murai, Acc. Chem. Res., 2002, 35, 826 CrossRef PubMed;
(b) S. Pan and T. Shibata, ACS Catal., 2013, 3, 704 CrossRef;
(c) X. Zeng, Chem. Rev., 2013, 113, 6864 CrossRef PubMed;
(d) L. Yang and H. Huang, Chem. Rev., 2015, 115, 3468 CrossRef PubMed;
(e) G. E. M. Crisenza and J. F. Bower, Chem. Lett., 2016, 45, 2 CrossRef;
(f) Z. Dong, Z. Ren, S. J. Thompson, Y. Xu and G. Dong, Chem. Rev., 2017, 117, 9333 CrossRef PubMed.
- For some examples on hydroarylation of alkenes using arenes as the aryl source, see:
(a) Z.-M. Sun, J. Zhang, R. S. Manan and P. Zhao, J. Am. Chem. Soc., 2010, 132, 6935 CrossRef PubMed;
(b) Y. Nakao, Y. Yamada, N. Kashihara and T. Hiyama, J. Am. Chem. Soc., 2010, 132, 13666 CrossRef PubMed;
(c) K. Gao and N. Yoshikai, J. Am. Chem. Soc., 2011, 133, 400 CrossRef PubMed;
(d) B.-T. Guan and Z. Hou, J. Am. Chem. Soc., 2011, 133, 18086 CrossRef PubMed;
(e) J. Oyamada and Z. Hou, Angew. Chem., Int. Ed., 2012, 51, 12828 CrossRef PubMed;
(f) S. Pan, N. Ryu and T. Shibata, J. Am. Chem. Soc., 2012, 134, 17474 CrossRef PubMed;
(g) P.-S. Lee and N. Yoshikai, Angew. Chem., Int. Ed., 2013, 52, 1240 CrossRef PubMed;
(h) T. Andou, Y. Saga, H. Komai, S. Matsunaga and M. Kanai, Angew. Chem., Int. Ed., 2013, 52, 3213 CrossRef PubMed;
(i) W. Xu and N. Yoshikai, Angew. Chem., Int. Ed., 2014, 53, 14166 CrossRef PubMed;
(j) G. E. M. Crisenza, N. G. McCreanor and J. F. Bower, J. Am. Chem. Soc., 2014, 136, 10258 CrossRef PubMed;
(k) J. S. Bair, Y. Schramm, A. G. Sergeev, E. Clot, O. Eisenstein and J. F. Hartwig, J. Am. Chem. Soc., 2014, 136, 13098 CrossRef PubMed;
(l) G. E. M. Crisenza, O. O. Sokolova and J. F. Bower, Angew. Chem., Int. Ed., 2015, 54, 14866 CrossRef PubMed;
(m) Y. Schramm, M. Takeuchi, K. Semba, Y. Nakao and J. F. Hartwig, J. Am. Chem. Soc., 2015, 137, 12215 CrossRef PubMed;
(n) Y. Ebe and T. Nishimura, J. Am. Chem. Soc., 2015, 137, 5899 CrossRef PubMed;
(o) C. M. Filloux and T. Rovis, J. Am. Chem. Soc., 2015, 137, 508 CrossRef PubMed;
(p) M. Hatano, Y. Ebe, T. Nishimura and H. Yorimitsu, J. Am. Chem. Soc., 2016, 138, 4010 CrossRef PubMed;
(q) N. Kimura, T. Kochi and F. Kakiuchi, J. Am. Chem. Soc., 2017, 139, 14849 CrossRef PubMed;
(r) J. Loup, D. Zell, J. C. A. Oliveira, H. Keil, D. Stalke and L. Ackermann, Angew. Chem., Int. Ed., 2017, 56, 14197 CrossRef PubMed.
-
(a) K. M. Gligorich, S. A. Cummings and M. S. Sigman, J. Am. Chem. Soc., 2007, 129, 14193 CrossRef PubMed;
(b) Y. Iwai, K. M. Gligorich and M. S. Sigman, Angew. Chem., Int. Ed., 2008, 47, 3219 CrossRef PubMed;
(c) L. Liao and M. S. Sigman, J. Am. Chem. Soc., 2010, 132, 10209 CrossRef PubMed.
-
(a) K. Semba, K. Ariyama, H. Zheng, R. Kameyama, S. Sakaki and Y. Nakao, Angew. Chem., Int. Ed., 2016, 55, 6275 CrossRef PubMed;
(b) S. D. Friis, M. T. Pirnot and S. L. Buchwald, J. Am. Chem. Soc., 2016, 138, 8372 CrossRef PubMed;
(c) S. D. Friis, M. T. Pirnot, L. N. Dupuis and S. L. Buchwald, Angew. Chem., Int. Ed., 2017, 56, 7242 CrossRef PubMed;
(d) X. Lu, B. Xiao, Z. Zhang, T. Gong, W. Su, J. Yi, Y. Fu and L. Liu, Nat. Commun., 2016, 7, 11129 CrossRef PubMed;
(e) S. A. Green, J. L. M. Matos, A. Yagi and R. A. Shenvi, J. Am. Chem. Soc., 2016, 138, 12779 CrossRef PubMed;
(f) Y. He, Y. Cai and S. Zhu, J. Am. Chem. Soc., 2017, 139, 1061 CrossRef PubMed;
(g) F. Chen, K. Chen, Y. Zhang, Y. He, Y.-M. Wang and S. Zhu, J. Am. Chem. Soc., 2017, 139, 13929 CrossRef PubMed;
(h) W. Li, J. K. Boon and Y. Zhao, Chem. Sci., 2018, 9, 600 RSC.
- L.-J. Xiao, L. Cheng, W.-M. Feng, M.-L. Li, J.-H. Xie and Q.-L. Zhou, Angew. Chem. Int. Ed., 2018, 57, 461 CrossRef PubMed.
- For some recent examples on directed unactivated alkenes functionalization without metal hydride species, see:
(a) E. P. A. Talbot, T. A. Fernandes, J. M. McKenna and F. D. Toste, J. Am. Chem. Soc., 2014, 136, 4101 CrossRef PubMed;
(b) A. Archambeau and T. Rovis, Angew. Chem., Int. Ed., 2015, 54, 13337 CrossRef PubMed;
(c) Z. Liu, T. Zeng, K. S. Yang and K. M. Engle, J. Am. Chem. Soc., 2016, 138, 15122 CrossRef PubMed;
(d) K. S. Yang, J. A. Gurak Jr, Z. Liu and K. M. Engle, J. Am. Chem. Soc., 2016, 138, 14705 CrossRef PubMed;
(e) J. A. Gurak Jr, K. S. Yang, Z. Liu and K. M. Engle, J. Am. Chem. Soc., 2016, 138, 5805 CrossRef PubMed;
(f) J. Derosa, V. T. Tran, M. N. Boulous, J. S. Chen and K. M. Engle, J. Am. Chem. Soc., 2017, 139, 10657 CrossRef PubMed;
(g) Z. Liu, Y. Wang, Z. Wang, T. Zeng, P. Liu and K. M. Engle, J. Am. Chem. Soc., 2017, 139, 11261 CrossRef PubMed;
(h) J. A. Gurak Jr and K. M. Engle, Synlett, 2017, 28, 2057 CrossRef;
(i) B. Shrestha, P. Basnet, R. K. Dhungana, S. Kc, S. Thapa, J. M. Sears and R. Giri, J. Am. Chem. Soc., 2017, 139, 10653 CrossRef PubMed;
(j) S. Guin, A. Deb, P. Dolui, S. Chakraborty, V. K. Singh and D. Maiti, ACS Catal., 2018, 8, 2664 CrossRef;
(k) A. Deb, A. Hazra, Q. Peng, R. S. Paton and D. Maiti, J. Am. Chem. Soc., 2017, 139, 763 CrossRef PubMed;
(l) A. Deb, S. Bag, R. Kancherla and D. Maiti, J. Am. Chem. Soc., 2014, 136, 13602 CrossRef PubMed.
- For some directed hydrofunctionalizations involving M–H species, see: hydrogenation:
(a) R. H. Crabtree and M. W. Davis, J. Org. Chem., 1986, 51, 2655 CrossRef;
(b) S. Song, S.-F. Zhu, Y.-B. Yu and Q.-L. Zhou, Angew. Chem., Int. Ed., 2013, 52, 1556 CrossRef PubMed ; hydrosilyation: ;
(c) J. B. Perales and D. L. Van Vranken, J. Org. Chem., 2001, 66, 7270 CrossRef PubMed;
(d) Y. Kawasaki, Y. Ishikawa, K. Igawa and K. Tomooka, J. Am. Chem. Soc., 2011, 133, 20712 CrossRef PubMed ; hydroboration: ;
(e) D. A. Evans and G. C. Fu, J. Am. Chem. Soc., 1991, 113, 4042 CrossRef;
(f) C. E. Garrett and G. C. Fu, J. Org. Chem., 1998, 63, 1370 CrossRef;
(g) M. Rubina, M. Rubin and V. Gevorgyan, J. Am. Chem. Soc., 2003, 125, 7198 CrossRef PubMed;
(h) S. M. Smith and J. M. Takacs, J. Am. Chem. Soc., 2010, 132, 1740 CrossRef PubMed;
(i) C. Sun, B. Potter and J. P. Morken, J. Am. Chem. Soc., 2014, 136, 6534 CrossRef PubMed;
(j) Z.-D. Yang, R. Pal, G. L. Hoang, X. C. Zeng and J. M. Takacs, ACS Catal., 2014, 4, 763 CrossRef PubMed;
(k) G. L. Hoang, Z.-D. Yang, S. M. Smith, R. Pal, J. L. Miska, D. E. Pérez, L. S. W. Pelter, X. C. Zeng and J. M. Takacs, Org. Lett., 2015, 17, 940 CrossRef PubMed;
(l) V. M. Shoba, N. C. Thacker, A. J. Bochat and J. M. Takacs, Angew. Chem., Int. Ed., 2016, 55, 1465 CrossRef PubMed;
(m) S. Chakrabarty and J. M. Takacs, J. Am. Chem. Soc., 2017, 139, 6066 CrossRef PubMed ; hydroacylation; ;
(n) M. M. Coulter, P. K. Dornan and V. M. Dong, J. Am. Chem. Soc., 2009, 131, 6932 CrossRef PubMed;
(o) M. M. Coulter, K. G. M. Kou, B. Galligan and V. M. Dong, J. Am. Chem. Soc., 2010, 132, 16330 CrossRef PubMed;
(p) S. K. Murphy, A. Bruch and V. M. Dong, Angew. Chem., Int. Ed., 2014, 53, 2455 CrossRef PubMed;
(q) S. K. Murphy, A. Brucha and V. M. Dong, Chem. Sci., 2015, 6, 174 RSC ; hydroformylation; ;
(r) C. L. Joe, T. P. Blaisdell, A. F. Geoghan and K. L. Tan, J. Am. Chem. Soc., 2014, 136, 8556 CrossRef PubMed;
(s) I. J. Krauss, C. C.-Y. Wang and J. L. Leighton, J. Am. Chem. Soc., 2001, 123, 11514 CrossRef PubMed;
(t) A. D. Worthy, L. Joe, T. E. Lightburn and K. L. Tan, J. Am. Chem. Soc., 2010, 132, 14757 CrossRef PubMed;
(u) B. Breit and D. Breuninger, J. Am. Chem. Soc., 2004, 126, 10244 CrossRef PubMed.
-
(a) A. F. Döbber, R. H. Phoa, B. W. Abbassi, B. W. Stringer, T. G. Day, M. Johns, C. P. Abadleh and L. Munoz, ACS Med. Chem. Lett., 2017, 8, 395 CrossRef PubMed;
(b) P. Prusevich, J. H. Kalin, S. A. Ming, M. Basso, J. Givens, X. Li, J. Hu, M. S. Taylor, A. M. Cieniewicz, P.-Y. Hsiao, R. Huang, H. Roberson, N. Adejola, L. B. Avery, R. A. Casero Jr, S. D. Taverna, J. Qian, A. J. Tackett, R. R. Ratan, O. G. McDonald, A. P. Feinberg and P. A. Cole, ACS Chem. Biol., 2014, 9, 1284 CrossRef PubMed;
(c) Y.-Z. Xu, X.-Y. Gu, S.-J. Peng, J.-G. Fang, Y.-M. Zhang, D.-J. Huang, J.-J. Chen and K. Gao, Eur. J. Med. Chem., 2015, 94, 284 CrossRef PubMed.
- CCDC 1838875 (3b) contains the supplementary crystallographic data.†.
-
(a) G. Huang and P. Liu, ACS Catal., 2016, 6, 809 CrossRef;
(b) M. Liu, P. Yang, M. K. Karunananda, Y. Wang, P. Liu and K. M. Engle, J. Am. Chem. Soc., 2018, 140, 5805 CrossRef PubMed;
(c) Z. Liu, H.-Q. Ni, T. Zeng and K. M. Engle, J. Am. Chem. Soc., 2018, 140, 3223 CrossRef PubMed.
Footnote |
† Electronic supplementary information (ESI) available. CCDC 1838875. For ESI and crystallographic data in CIF or other electronic format see DOI: 10.1039/c8sc02101e |
|
This journal is © The Royal Society of Chemistry 2018 |