DOI:
10.1039/C9SC01527B
(Edge Article)
Chem. Sci., 2019,
10, 6311-6315
A regioselectivity switch in Pd-catalyzed hydroallylation of alkynes†
Received
28th March 2019
, Accepted 16th May 2019
First published on 16th May 2019
Abstract
By exploiting the reactivity of a vinyl-Pd species, we control the regioselectivity in hydroallylation of alkynes under Pd-hydride catalysis. A monophosphine ligand and carboxylic acid combination promotes 1,5-dienes through a pathway involving isomerization of alkynes to allenes. In contrast, a bisphosphine ligand and copper cocatalyst favor 1,4-dienes via a mechanism that involves transmetalation. Our study highlights how to access different isomers by diverting a common organometallic intermediate.
Introduction
Inventing novel cross-couplings has relied upon our ability to divert readily accessible and common organometallic intermediates.1 For example, Pd(II) intermediates I (generated from organic halides or their analogues) can transmetallate with organoborons in a Suzuki–Miyaura cross-coupling2 or undergo insertion into olefins and subsequent β-hydride elimination in the Heck reaction (Scheme 1, top).3 Inspired by the power of this concept, we set out to divert the reactivity of a vinyl-Pd(II) intermediate II (generated from alkynes, Scheme 1, bottom) to achieve useful skipped dienes.
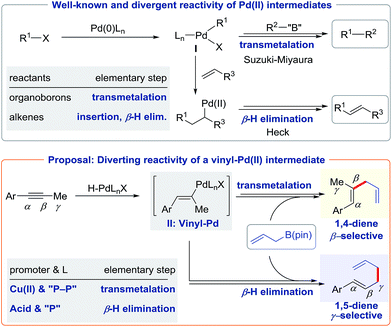 |
| Scheme 1 Divergent reactivity of Pd(II) species. | |
The hydroallylation of alkynes has attracted attention due to the occurrence of skipped dienes in bioactive compounds and natural products.4 While various catalysts have been developed to generate the 1,4-diene motif,5 access to the 1,5-diene isomer via hydroallylation of alkynes has been elusive. In considering this challenge, we were inspired by the work of Trost,6 Yamamoto,7 and Breit8 who have used alkynes as redox-neutral allyl precursors.9–12 Early studies established that Pd–H can add to a alkyne to generate the vinyl-Pd species II. With this in mind, we set out to manipulate the reactivity of this Pd(II) species II towards transmetalation or β-H elimination to enable selective access to 1,4 or 1,5-dienes, respectively (Scheme 1, bottom). Herein, we report the use of ligands and promoters to enable a regiodivergent synthesis of skipped dienes. Our study contributes to the art of diverging catalytic intermediates to access different constitutional isomers.13
Results and discussion
We began our study with 1-phenyl-1-propyne 1a and allyl-B(pin) 2a as the model substrates. After examining various combinations of ligands and additives, we obtained compelling results (Chart 1). In the presence of Pd2(dba)3 (2.5 mol%) and a proton source nBuOH (2.0 equiv.), we found that monophosphine ligands such as PPhCy2 and PCy3 could give 1,5-diene (Chart 1A) as the major product. With the aid of PCy3, 1,5-diene 3a could be obtained in 18% yield accompanied by trace amounts of 1,4-dienes 4a and 4a′ mixture. To improve the yield of 1,5-diene 3a, we chose Brønsted acid to facilitate the formation of an active Pd(II)–H catalyst.7 By adding 10 mol% 1-adamantanecarboxylic acid, we obtained 3a in 83% yield with excellent selectivity (Chart 1B). Bisphosphine ligands gave only trace amounts of 1,5-diene 3a because these ligands occupy the otherwise vacant sites needed for β-hydride elimination.1,14
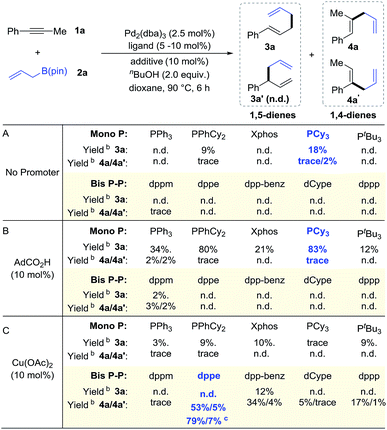 |
| Chart 1 Ligand and promoter effects on hydroallylation. a1a (0.20 mmol), 2a (2.0 equiv.), Pd2(dba)3 (2.5 mol%), monophosphine (10 mol%) or bis-phosphine (5 mol%), promoter (10 mol%), nBuOH (2.0 equiv.), dioxane (1.0 mL), 90 °C, and 6 h. bDetermined by 1H NMR or GC-FID with 1,3,5-trimethoxybenzene as the internal standard. cMeOH (3.0 equiv.) instead of nBuOH, dioxane (0.5 mL), 70 °C, and 24 h. | |
Next, we aimed to selectively prepare the 1,4-diene 4a, which is the minor isomer that results from direct coupling of alkyne 1a and allylboron 2a. To alter regioselectivity, we pursued a co-catalyst that would accelerate transmetalation between the allyl species and the vinyl-Pd intermediate, and therefore enable the synthesis of 1,4-dienes. An evaluation of co-catalysts revealed that Cu(OAc)2 promoted the formation of 4a/4a′ (Chart 1C). Moreover, in contrast to the monophosphine ligands that promotes the formation of 3a, bisphosphine ligands such as dppe and dpp-benz gave a higher yield of 4a/4a′. When dppe was used as the ligand, 4a/4a′ was delivered in 58% combined yield as a 10
:
1 mixture of regioisomers. Using methanol instead of butanol as the proton source improved the yield of 4a to 79%. In the absence of palladium, no products were detected, which indicates that this transformation is not catalyzed by copper alone.
As shown in Table 1A, we obtained various 1,5-dienes in moderate to good yields and high selectivities (>20
:
1, 3vs.4). Substrates with electron-donating groups proceed smoothly to deliver 1,5-dienes 3b–3d. Fluoro and chloro groups are also well tolerated, yielding products 3e and 3f in 74% and 78% yield. Aryl alkynes bearing electron-withdrawing substituents (CF3, Ph, Ac, and CO2Me) show slightly higher reactivities, providing linear products (3g–3j) in high yields (81–90%). High selectivities are still obtained for meta-substituted 1-arylalkyne 1k–1m. The steric hindrance of alkyne influences the reactivity and offers 3n with 52% yield. On replacing the phenyl group with 2-naphthalenyl groups, the substrate transforms into a mixture of linear product 3o and branched product 3o′ in a 1
:
1.7 ratio. The branched product 3o′ probably originates from the formed η3-π-benzyl-palladium intermediate.15 No desired 1,5-diene product is obtained when alkyl-substituted alkyne 1p or 1q is subjected to standard conditions. Instead, the isomerization product 1-phenyl-1,3-butadiene is observed for the conversion of 1p. Notably, substrates bearing pyridine rings, which were incompatible in previously reported palladium catalysis,16 also lead to 3r and 3s in moderate yields. Finally, the late-stage modification of the estrone derivative 1t affords 3t in 38% yield. This 1,5-diene synthesis complements known allyl–allyl couplings that require pre-functionalized allyl precursors such as allyl chlorides or carbonates.16,17
Table 1 Regioselective hydroallylation of alkynesa
Isolated yield of the all isomers. Unless otherwise noted, selectivity > 20 : 1.
Accompanied by a small amount of inseparable alkyne 1, and the yield of the product has been adjusted accordingly.
Isolated product together with 1-phenyl-1,3-butadiene in a 4.0 : 1 ratio, and the yield of the product has been adjusted accordingly.
|
|
Then, we examined the substrate scope for the synthesis of 1,4-dienes (Table 1B). Various alkynes can be transformed into 1,4-dienes using the Pd/Cu catalyst combo. Although substrates bearing electron-donating groups lead to skipped dienes with moderate to good regioselectivities (4b–4d, 4k, and 4m), electron-withdrawing substrates perform well in terms of yields and selectivities (4e–4j, 4l). Ortho-substituted alkyne 1n exhibits no reactivity due to steric hindrance. It is noteworthy that 4p and 4p′ are successfully acquired in 49% yield, accompanied by a small amount of isomerization side product (1-phenyl-1,3-butadiene). Comparatively, the cross-coupling between 1-phenyl-1-hexyne 1q and 2a provides 1,4-diene products 4q/4q′ without any 1,3-diene side product. Heterocyclic substituted alkynes (1r and 1s) and estrone derivatives all successfully deliver the 1,4-diene products.
Besides internal alkynes, terminal alkyne 1u couples with allylB(pin) 2a to yield 1,5-diene 3a (Scheme 2). The bis-allylations of di(prop-1-yn-1-yl)benzene 1v and 1w proceed smoothly with high selectivities and moderate yields. These olefin products are potential monomers for polymerization.18
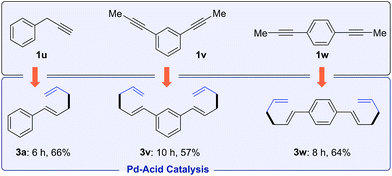 |
| Scheme 2 Hydroallylation of terminal and bis-alkynes. | |
We also tested the scope of allylborons under our Pd-acid conditions (Table 2). Generally, substrates 2b–2d were less reactive in allyl–allyl couplings. This agrees with previous work reported by Morken's group that substituted allylborons were comparatively reluctant in Pd-catalyzed allyl–allyl coupling reactions.17g To improve the reactivity, Cu(OAc)2 was employed as an additional promoter to facilitate transmetalation. The yields of 3x and 3y were successfully increased to 34% and 38%, respectively. It should be noted that these reactions all give interesting linear-branched coupling products, and this selectivity is rare in Suzuki-type allyl–allyl coupling reactions.17
Table 2 The scope of allylborons
While further studies are warranted, we propose the following mechanisms on the basis of literature7 and our own observations (Fig. 1A, right). First, the oxidative addition of the carboxylic acid with a Pd(0) precursor generates Pd(II)-hydride species A. Syn-Migratory insertion of alkyne 1 into Pd(II)–H A affords vinyl-Pd intermediate B. For the 1,5-diene pathway, a vacant coordination site of complex B is spared for β-hydride elimination in the presence of the monophosphine ligand. Allene 5 is subsequently produced and undergoes reinsertion into Pd(II)–H forming the π-allyl-Pd intermediate C. Then, electrophilic intermediate C reacts with allylB(pin) 2 to deliver bis(allyl)Pd species D.19 Reductive elimination yields the allyl–allyl coupling product 3a and turns over the Pd(0) catalyst (allyl–allyl coupling cycle).
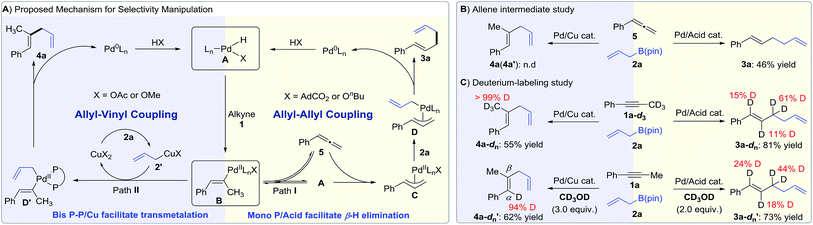 |
| Fig. 1 Divergent reactivity of Pd(II) species. | |
In the presence of a Cu(II) co-catalyst, we propose that transmetalation of allylboron 2a to generate allylcopper species 2′ is favored.5i,17k In this protocol, vinyl-Pd species B is also generated by a syn-migratory of alkyne 1 into Pd(II)–H A (Fig. 1A, left). However, when coordinated with a bidentate ligand, vinyl-Pd intermediate B prefers direct transmetalation with allylcopper species 2′ to form D′ rather than undergoing β-hydride elimination to produce allene 5. Reductive elimination from D′ yields the product 1,4-diene 4a (allyl–vinyl coupling cycle).
To probe the feasibility of an allene intermediate, phenylallene 5 was subjected to couple with allyl-B(pin) 2a under two standard conditions (Fig. 1B, see the ESI for details†). The allene was transformed into 1,5-diene 3a in 46% yield. No formation of 4a or 4a′ under the Pd–Cu conditions supports that the 1,4-diene products do not arise from the addition of allylB(pin) 2a to allene 5. Hydroallylation was also performed with deuterated alkyne 1a-d3 or methanol (Fig. 1C). Under Pd/acid catalysis, we found that the deuterium label was scrambled into the α-, β-, and γ-positions of 1,5-diene 3a-dn using 1a-d3. Similar deuterium scrambling was observed when conducting reaction with deuterated methanol as a proton source. This observation supports a reversible hydrometallation of the internal π-system of the allene in the synthesis of 1,5-dienes. When experiments were carried out under Pd–Cu catalysis, the deuterium label remained intact in 1,4-diene 4a-dn with deuterated alkyne 1a-d3. Only an α-deuterated product was achieved using deuterated methanol (Fig. 1C). This indicates that β-hydride elimination is not involved in Pd/Cu catalysis.
Conclusions
Our work complements other alkyne hydroallylation methods for the synthesis of 1,4-dienes including those developed by Hilt, Hartwig, Lalic and Zhang.5 The key to the success of this method is the switchable reactivity of vinyl-Pd intermediates. Acid additive promotes the β-hydride elimination pathway for allyl–allyl coupling with the aid of a monophosphine ligand, whereas the Cu co-catalyst facilitates the direct transmetalation for vinyl-allyl coupling in the presence of a bisphosphine ligand. Transmetalation and β-hydride elimination are two elementary steps featured in many well-known organometallic mechanisms, including Suzuki–Miyaura and Heck cross-coupling. Insights from this study will guide the future development of related regiodivergent methods in catalysis.
Conflicts of interest
There are no conflicts to declare.
Acknowledgements
We thank Prof. Yong-Gui Zhou (DICP) and Dr Kevin G. M. Kou (UCR) for helpful discussion and paper revisions. Q. A. C thanks the Dalian Institute of Chemical Physics and the National Natural Science Foundation of China (21702204), and acknowledges the LiaoNing Revitalization Talents Program (XLYC1807181) and the “Thousand Youth Talents Plan” for financial support. V. M. D thanks the National Institutes of Health (R35GM127071) for financial support.
Notes and references
- For a book, see: J. F. Hartwig, Organotransition Metal Chemistry: From Bonding to Catalysis, University Science Books, Sausalito, 2010 Search PubMed.
- For selected reviews on Suzuki–Miyaura cross-coupling, see:
(a) N. Miyaura and A. Suzuki, Chem. Rev., 1995, 95, 2457 CrossRef CAS;
(b) A. F. Littke and G. C. Fu, Angew. Chem., Int. Ed., 2002, 41, 4176 CrossRef CAS;
(c) R. Jana, T. P. Pathak and M. S. Sigman, Chem. Rev., 2011, 111, 1417 CrossRef CAS PubMed;
(d) A. Suzuki, Angew. Chem., Int. Ed., 2011, 50, 6722 CrossRef CAS PubMed.
- For selected reviews on Heck reaction, see:
(a) I. P. Beletskaya and A. V. Cheprakov, Chem. Rev., 2000, 100, 3009 CrossRef CAS PubMed;
(b) R. F. Heck, Acc. Chem. Res., 1979, 12, 146 CrossRef CAS.
-
(a) M. Jie, M. K. Pasha and M. S. K. SyedRahmatullah, Nat. Prod. Rep., 1997, 14, 163 RSC;
(b)
E. Breitmaier, Terpenes: Flavors, Fragrances, Pharmaca, Pheromones, Wiley-VCH, Weinheim, Germany, 2006 CrossRef.
- For selected examples for hydroallylation of alkynes, see:
(a) K. Oshima, T. Nishikawa and H. Yorimitsu, Synlett, 2004, 1573 CrossRef;
(b) H. Yasui, T. Nishikawa, H. Yorimitsu and K. Oshima, Bull. Chem. Soc. Jpn., 2006, 79, 1271 CrossRef CAS;
(c) G. Hilt and J. Treutwein, Angew. Chem., Int. Ed., 2007, 46, 8500 CrossRef CAS PubMed;
(d) G. Hilt, F. Erver and K. Harms, Org. Lett., 2011, 13, 304 CrossRef CAS PubMed;
(e) L. Ilies, T. Yoshida and E. Nakamura, J. Am. Chem. Soc., 2012, 134, 16951 CrossRef CAS PubMed;
(f) Y. Yamamoto, S. Yamada and H. Nishiyama, Chem.–Eur. J., 2012, 18, 3153 CrossRef CAS PubMed;
(g) W. Kong, C. Che, L. Kong and G. Zhu, Tetrahedron Lett., 2015, 56, 2780 CrossRef CAS;
(h) D. P. Todd, B. B. Thompson, A. J. Nett and J. Montgomery, J. Am. Chem. Soc., 2015, 137, 12788 CrossRef CAS PubMed;
(i) Y. Yamamoto, E. Ohkubo and M. Shibuya, Green Chem., 2016, 18, 4628 RSC;
(j) M. Mailig, A. Hazra, M. K. Armstrong and G. Lalic, J. Am. Chem. Soc., 2017, 139, 6969 CrossRef CAS PubMed;
(k) K. Troshin and J. F. Hartwig, Science, 2017, 357, 175 CrossRef CAS PubMed;
(l) G. Xu, H. Zhao, B. Fu, A. Cang, G. Zhang, Q. Zhang, T. Xiong and Q. Zhang, Angew. Chem., Int. Ed., 2017, 56, 13130 CrossRef CAS PubMed.
- B. M. Trost, W. Brieden and K. H. Baringhaus, Angew. Chem., Int. Ed., 1992, 31, 1335 CrossRef.
-
(a) I. Kadota, A. Shibuya, Y. S. Gyoung and Y. Yamamoto, J. Am. Chem. Soc., 1998, 120, 10262 CrossRef CAS;
(b) I. Kadota, A. Shibuya, L. M. Lutete and Y. Yamamoto, J. Org. Chem., 1999, 64, 4570 CrossRef CAS PubMed;
(c) L. M. Lutete, I. Kadota and Y. Yamamoto, J. Am. Chem. Soc., 2004, 126, 1622 CrossRef CAS PubMed.
- For reviews, see:
(a) P. Koschker and B. Breit, Acc. Chem. Res., 2016, 49, 1524 CrossRef CAS PubMed;
(b) A. M. Haydl, B. Breit, T. Liang and M. J. Krische, Angew. Chem., Int. Ed., 2017, 56, 11312 CrossRef CAS PubMed.
- For selected Pd-catalyzed allylation with alkynes, see:
(a) S. Gao, Z. Wu, X. Fang, A. Lin and H. Yao, Org. Lett., 2016, 18, 3906 CrossRef CAS PubMed;
(b) C. J. Lu, H. Chen, D. K. Chen, H. Wang, Z. P. Yang, J. Gao and H. Jin, Org. Biomol. Chem., 2016, 14, 10833 RSC;
(c) S. Gao, H. Liu, Z. Wu, H. Yao and A. Lin, Green Chem., 2017, 19, 1861 RSC;
(d) S. Gao, H. Liu, C. Yang, Z. Fu, H. Yao and A. Lin, Org. Lett., 2017, 19, 4710 CrossRef CAS PubMed;
(e) G. Cera, M. Lanzi, D. Balestri, N. Della Ca, R. Maggi, F. Bigi, M. Malacria and G. Maestri, Org. Lett., 2018, 20, 3220 CrossRef CAS PubMed;
(f) X. Fang, Q. Li, R. Shi, H. Yao and A. Lin, Org. Lett., 2018, 20, 6084 CrossRef CAS PubMed;
(g) J. T. D. Lee and Y. Zhao, Chem.–Eur. J., 2018, 24, 9520 CrossRef CAS PubMed;
(h) Y. Minami, Y. Furuya and T. Hiyamera, Asian J. Org. Chem., 2018, 7, 1343 CrossRef CAS;
(i) Y. L. Su, L. L. Li, X. L. Zhou, Z. Y. Dai, P. S. Wang and L. Z. Gong, Org. Lett., 2018, 20, 2403 CrossRef CAS PubMed;
(j) Z. Wu, X. Fang, Y. Leng, H. Yao and A. Lin, Adv. Synth. Catal., 2018, 360, 1289 CrossRef CAS;
(k) J. Zheng and B. Breit, Org. Lett., 2018, 20, 1866 CrossRef CAS PubMed.
- For selected Rh-catalyzed allylation with alkynes, see:
(a) A. Lumbroso, P. Koschker, N. R. Vautravers and B. Breit, J. Am. Chem. Soc., 2011, 133, 2386 CrossRef CAS PubMed;
(b) U. Gellrich, A. Meissner, A. Steffani, M. Kahny, H. J. Drexler, D. Heller, D. A. Plattner and B. Breit, J. Am. Chem. Soc., 2014, 136, 1097 CrossRef CAS PubMed;
(c) K. Xu, V. Khakyzadeh, T. Bury and B. Breit, J. Am. Chem. Soc., 2014, 136, 16124 CrossRef CAS PubMed;
(d) Q.-A. Chen, Z. Chen and V. M. Dong, J. Am. Chem. Soc., 2015, 137, 8392 CrossRef CAS PubMed;
(e) P. Koschker, M. Kahny and B. Breit, J. Am. Chem. Soc., 2015, 137, 3131 CrossRef CAS PubMed;
(f) Z. Liu and B. Breit, Angew. Chem., Int. Ed., 2016, 55, 8440 CrossRef CAS PubMed;
(g) F. A. Cruz, Y. Zhu, Q. D. Tercenio, Z. Shen and V. M. Dong, J. Am. Chem. Soc., 2017, 139, 10641 CrossRef CAS PubMed;
(h) J. Kuang, S. Parveen and B. Breit, Angew. Chem., Int. Ed., 2017, 56, 8422 CrossRef CAS PubMed;
(i) W.-F. Zheng, Q.-J. Xu and Q. Kang, Organometallics, 2017, 36, 2323 CrossRef CAS;
(j) J. Zheng and B. Breit, Angew. Chem., Int. Ed., 2019, 58, 3392 CrossRef CAS PubMed.
- For Ir-catalyzed allylation with alkynes, see:
(a) Y. Obora, S. Hatanaka and Y. Ishii, Org. Lett., 2009, 11, 3510 CrossRef CAS PubMed;
(b) Y. Obora, T. Sawaguchi, K. Tsubakimoto, H. Yoshida, S. Ogawa and S. Hatanaka, Synthesis, 2013, 45, 2115 CrossRef CAS;
(c) T. Liang, W. Zhang and M. J. Krische, J. Am. Chem. Soc., 2015, 137, 16024 CrossRef CAS PubMed.
- For Ru-catalyzed allylation with alkynes, see:
(a) B. Y. Park, K. D. Nguyen, M. R. Chaulagain, V. Komanduri and M. J. Krische, J. Am. Chem. Soc., 2014, 136, 11902 CrossRef CAS PubMed;
(b) Q. A. Chen, F. A. Cruz and V. M. Dong, J. Am. Chem. Soc., 2015, 137, 3157 CrossRef CAS PubMed;
(c) T. Liang, K. D. Nguyen, W. Zhang and M. J. Krische, J. Am. Chem. Soc., 2015, 137, 3161 CrossRef CAS PubMed;
(d) T. Liang, W. Zhang, T. Y. Chen, K. D. Nguyen and M. J. Krische, J. Am. Chem. Soc., 2015, 137, 13066 CrossRef CAS PubMed.
- For selected examples, see:
(a) R. Y. Liu, Y. Yang and S. L. Buchwald, Angew. Chem., Int. Ed., 2016, 55, 14077 CrossRef CAS PubMed;
(b) S. R. Sardini and M. K. Brown, J. Am. Chem. Soc., 2017, 139, 9823 CrossRef CAS PubMed;
(c) K. B. Smith and M. K. Brown, J. Am. Chem. Soc., 2017, 139, 7721 CrossRef CAS PubMed;
(d) J. W. Park, B. Kang and V. M. Dong, Angew. Chem., Int. Ed., 2018, 57, 13598 CrossRef CAS PubMed;
(e) A. M. Bergmann, S. K. Dorn, K. B. Smith, K. M. Logan and M. K. Brown, Angew. Chem., Int. Ed., 2019, 58, 1719 CrossRef CAS PubMed;
(f) X. H. Yang, R. T. Davison, S. Z. Nie, F. A. Cruz, T. M. McGinnis and V. M. Dong, J. Am. Chem. Soc., 2019, 141, 3006 CrossRef CAS PubMed;
(g) Y. Ye, S. T. Kim, J. Jeong, M. H. Baik and S. L. Buchwald, J. Am. Chem. Soc., 2019, 141, 3901 CrossRef CAS PubMed;
(h) Y. C. Hu, D. W. Ji, C. Y. Zhao, H. Zheng and Q. A. Chen, Angew. Chem., Int. Ed., 2019, 58, 5438 CrossRef CAS PubMed.
-
(a) J. H. Kirchhoff, M. R. Netherton, I. D. Hills and G. C. Fu, J. Am. Chem. Soc., 2002, 124, 13662 CrossRef CAS PubMed;
(b) A. V. Vorogushin, X. H. Huang and S. L. Buchwald, J. Am. Chem. Soc., 2005, 127, 8146 CrossRef CAS PubMed.
-
(a) S. Lu, Z. Xu, M. Bao and Y. Yamamoto, Angew. Chem., Int. Ed., 2008, 47, 4366 CrossRef CAS PubMed;
(b) B. Peng, X. Feng, X. Zhang, S. Zhang and M. Bao, J. Org. Chem., 2010, 75, 2619 CrossRef CAS PubMed;
(c) S. Zhang, A. Ullah, Y. Yamamoto and M. Bao, Adv. Synth. Catal., 2017, 359, 2723 CrossRef CAS;
(d) M. Komatsuda, K. Muto and J. Yamaguchi, Org. Lett., 2018, 20, 4354 CrossRef CAS PubMed.
- E. Ferrer Flegeau, U. Schneider and S. Kobayashi, Chem.–Eur. J., 2009, 15, 12247 CrossRef PubMed.
-
(a) P. Zhang, L. A. Brozek and J. P. Morken, J. Am. Chem. Soc., 2010, 132, 10686 CrossRef CAS PubMed;
(b) L. A. Brozek, M. J. Ardolino and J. P. Morken, J. Am. Chem. Soc., 2011, 133, 16778 CrossRef CAS PubMed;
(c) A. Jimenez-Aquino, E. F. Flegeau, U. Schneider and S. Kobayashi, Chem. Commun., 2011, 47, 9456 RSC;
(d) P. Zhang, H. Le, R. E. Kyne and J. P. Morken, J. Am. Chem. Soc., 2011, 133, 9716 CrossRef CAS PubMed;
(e) M. B. Li, Y. Wang and S. K. Tian, Angew. Chem., Int. Ed., 2012, 51, 2968 CrossRef CAS PubMed;
(f) V. Hornillos, M. Perez, M. Fananas-Mastral and B. L. Feringa, J. Am. Chem. Soc., 2013, 135, 2140 CrossRef CAS PubMed;
(g) M. J. Ardolino and J. P. Morken, J. Am. Chem. Soc., 2014, 136, 7092 CrossRef CAS PubMed;
(h) J. Y. Hamilton, N. Hauser, D. Sarlah and E. M. Carreira, Angew. Chem., Int. Ed., 2014, 53, 10759 CrossRef CAS PubMed;
(i) J. Y. Hamilton, D. Sarlah and E. M. Carreira, J. Am. Chem. Soc., 2014, 136, 3006 CrossRef CAS PubMed;
(j) K. Semba, N. Bessho, T. Fujihara, J. Terao and Y. Tsuji, Angew. Chem., Int. Ed., 2014, 53, 9007 CrossRef CAS PubMed;
(k) Y. Yasuda, H. Ohmiya and M. Sawamura, Angew. Chem., Int. Ed., 2016, 55, 10816 CrossRef CAS PubMed;
(l) X. Wang, X. Wang, Z. Han, Z. Wang and K. Ding, Angew. Chem., Int. Ed., 2017, 56, 1116 CrossRef CAS PubMed.
-
(a) J. A. Smith, K. R. Brzezinska, D. J. Valenti and K. B. Wagener, Macromolecules, 2000, 33, 3781 CrossRef CAS;
(b) J. Pecher and S. Mecking, Macromolecules, 2007, 40, 7733 CrossRef CAS;
(c) B. Inci, I. Lieberwirth, W. Steffen, M. Mezger, R. Graf, K. Landfester and K. B. Wagener, Macromolecules, 2012, 45, 3367 CrossRef CAS.
- For a review, see: K. J. Szabo, Chem.–Eur. J., 2004, 10, 5268 CrossRef CAS PubMed.
Footnote |
† Electronic supplementary information (ESI) available. See DOI: 10.1039/c9sc01527b |
|
This journal is © The Royal Society of Chemistry 2019 |