DOI:
10.1039/C9SC00603F
(Edge Article)
Chem. Sci., 2019,
10, 6316-6321
A ruthenium(II)-catalyzed C–H allenylation-based approach to allenoic acids†
Received
2nd February 2019
, Accepted 4th May 2019
First published on 10th May 2019
Abstract
A Ru(II)-catalyzed direct access to various functionalized allenoic acids via C–H allenylation of readily available aryl carboxylic acids with propargylic acetates is reported. Axially chiral allenoic acids could be obtained in high ee by using optically active propargylic acetates through a chirality transfer strategy.
Allene moieties are not only present in natural products but also in precious building blocks due to their unique structures and multiple reactive sites.1 Allene chemistry has experienced an explosion during the last few decades.2 Thus, the synthesis of functionalized allenes is of crucial importance. Of particular interest is the synthesis of synthetically versatile allenoic acids.3 A common approach to 2,3-allenoic acids is the hydrolysis of 2,3-allenoates, which suffers from poor step-economy and a selectivity issue of forming allenoic acids and 3-alkynoic acids (Scheme 1a).4 The only example of the oxidation of allenols is realized through microbial transformation (Scheme 1b).5 Pd- or Ni-catalyzed carboxylation of propargylic compounds with CO in the presence of water (Scheme 1c)6 and the carboxylation of 2-alkynyl bromides or allenylmetallic reagents with CO2 (Scheme 1d)7 have also been reported. Crabbé homologation of o-methoxycarbonylphenylacetylene with paraformaldehyde results in the formation of methyl 2-propadienylbenzoate, which undergoes hydrolysis to afford the corresponding allenoic acid (Scheme 1e).8 The limitations are harsh conditions and the use of toxic carbon monoxide, stoichiometric amounts of reductants and limited substrates. On the other hand, C–H activation has been proven to be a powerful tool in synthetic chemistry because of the atom- and step-economy.9 The synthesis of allenes based on C–H activation is undoubtedly an ideal strategy.10 We reasoned that the most straightforward approach to allenoic acids would be the use of benzoic acids with carboxylic acid acting as an inherent directing group.11 Herein, we wish to report the realization of Ru-catalyzed synthesis of allenoic acids via direct C–H allenylation of benzoic acids (Scheme 1f).
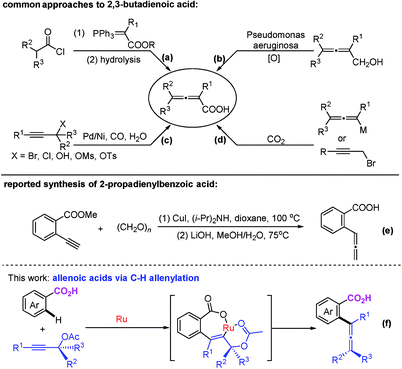 |
| Scheme 1 Approaches to allenoic acids. | |
Our initial attempt began with benzoic acid 1a and propargylic acetate 2a in the presence of [Ru(p-cymene)Cl2]2 and NaOAc at 50 °C using toluene as the solvent. To our delight, the monoallenylation product 3aa was generated in 7% yield together with 67% recovery of 2a (Table 1, entry 1). We then investigated the effect of the solvent (Table 1, entries 2–7). The reaction could proceed in dioxane, DCE, CH3CN, THF, and even in water, albeit affording the monoallenylation product 3aa and the double allenylation product 4aa in rather low yields (Table 1, entries 2–6). To our surprise, the yield could be greatly improved when the reaction was conducted in MeOH: 36% yield of the monoallenylation product 3aa and 16% yield of the double allenylation product 4aa were obtained (Table 1, entry 7). We next examined a series of additives as shown in entries 8–13: when K2CO3 was employed, the reaction gave a 64% combined yield of 3aa and 4aa (Table 1, entry 13). The reaction in EtOH under optimal conditions led to increased combined yield but a lower selectivity of 3aa/4aa (Table 1, entries 13–14). When 2.6 equiv. of benzoic acid were used, the yield was the highest with a selectivity of 58/14 (Table 1, entry 17). When the reaction was conducted in air, the influence was negligible (Table 1, entry 19). In the absence of the base, we only observed the decomposition of propargylic acetate.
Table 1 Optimization of the ortho-allenylation of benzoic acid 1aa

|
Entry |
x
|
Solvent |
Additive |
Time (h) |
Combined yield (3aa/4aa)b (%) |
Recovery of 2ab (%) |
The reaction was conducted with 1a, 2a (0.2 mmol), [Ru(p-cymene)Cl2]2 (0.004 mmol), and an additive (0.06 mmol) in a solvent (0.5 mL) at 50 °C.
Determined by 1H NMR analysis using CH2Br2 as the internal standard.
In air.
|
1 |
1.5 |
Toluene |
NaOAc |
46 |
7 (7/0) |
67 |
2 |
1.5 |
Dioxane |
NaOAc |
46 |
15 (12/3) |
20 |
3 |
1.5 |
DCE |
NaOAc |
46 |
14 (14/0) |
24 |
4 |
1.5 |
CH3CN |
NaOAc |
46 |
27 (23/4) |
26 |
5 |
1.5 |
THF |
NaOAc |
46 |
30 (23/7) |
6 |
6 |
1.5 |
H2O |
NaOAc |
46 |
19 (12/7) |
23 |
7 |
1.5 |
MeOH |
NaOAc |
46 |
52 (36/16) |
13 |
8 |
1.5 |
MeOH |
NaOAc |
12 |
57 (39/18) |
29 |
9 |
1.5 |
MeOH |
K3PO4 |
12 |
41 (30/11) |
7 |
10 |
1.5 |
MeOH |
t-BuONa |
12 |
22 (18/4) |
21 |
11 |
1.5 |
MeOH |
Na2CO3 |
12 |
57 (40/17) |
18 |
12 |
1.5 |
MeOH |
Cs2CO3 |
12 |
60 (42/18) |
20 |
13 |
1.5 |
MeOH |
K2CO3 |
12 |
64 (45/19) |
27 |
14 |
1.5 |
EtOH |
K2CO3 |
12 |
69 (36/33) |
9 |
15 |
2.0 |
MeOH |
K2CO3 |
12 |
66 (48/18) |
16 |
16 |
2.4 |
MeOH |
K2CO3 |
12 |
63 (50/13) |
16 |
17 |
2.6 |
MeOH |
K2CO3 |
12 |
72 (58/14) |
20 |
18 |
3.0 |
MeOH |
K2CO3 |
12 |
61 (52/9) |
21 |
19c |
2.6 |
MeOH |
K2CO3 |
12 |
67 (56/11) |
18 |
20c |
2.6 |
MeOH |
— |
12 |
0 |
42 |
We further investigated the effect of the leaving group (LG) by studying the reaction of benzoic acid 1a with several propargylic alcohol derivatives and found that OAc was still the best leaving group (Table 2).
Table 2 Effect of the leaving groups

|
Entry |
LG |
Combined yield (3aa/4aa)a (%) |
Recovery of 2aa (%) |
Determined by 1H NMR analysis using CH2Br2 as the internal standard.
|
1 |
OMe (2a1) |
0 (—/—) |
59 |
2 |
OCO2Me (2a2) |
54 (40/14) |
9 |
3 |
OCOEt (2a3) |
57 (47/10) |
20 |
4 |
OBoc (2a4) |
61 (47/14) |
21 |
5 |
OAc (2a) |
72 (58/14) |
20 |
With the optimized reaction conditions in hand, the scope of the reaction was investigated at the 1.0 mmol scale (eqn (1) and (2) and Table 3). The parent benzoic acid 1a afforded the monoallenylation product 3aa in 55% yield together with the diallenylation product 4aa in 10% yield (eqn (1)). 4-Bromobenzoic acid 1n was converted to the monoallenylation product 3na (45% yield) and the bisallenylation product 4ma (26% yield) under the standard conditions (eqn (2)).
| 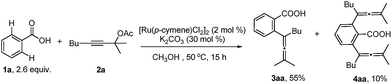 | (1) |
|  | (2) |

|
The reaction was conducted with 1 (2.4 mmol), 2 (1.0 mmol), [Ru(p-cymene)Cl2]2 (0.02 mmol), and K2CO3 (0.3 mmol) in EtOH (2.5 mL) at 50 °C.
4 mol% [Ru(p-cymene)Cl2]2.
5.0 mL of EtOH as the solvent.
|
|
For mono-o-substituted benzoic acids, electron withdrawing groups, such as halogen atoms (including fluorine, chlorine, bromine, and iodine), CF3, and OCF3, were all well tolerated (Table 3, 3ba–3gf). The structure of 3ba was confirmed by X-ray diffraction study.12 Mono-o-substituted benzoic acids containing the electron-donating groups methoxy and phenoxy afforded allenylation products 3hb in 71% yield and 3ia in 61% yield, respectively. 2,3-Dichlorobenzoic acid (3ja), 2-methyl-3-nitrobenzoic acid (3kf and 3kg) and 2-chloro-4-bromobenzoic acid (3lf) were also allenylated in moderate to good yields. Notably, when the reaction was conducted with β-naphthoic acid, which has more than one C–H bond, 3-allenylation products 3ma and 3me were obtained exclusively. The scope of C–H allenylation with regard to propargylic acetates was also investigated affording 3cb, 3nb, 3dc, 3dd, 3ee, 3le, 3kf, 3gf, or 3kg smoothly.
A gram scale reaction using 2-bromobenzoic acid 1d with 2g afforded the allenylation product 3dg in 67% yield (eqn (3)).
| 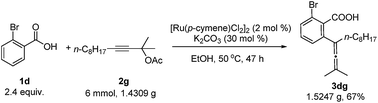 | (3) |
In addition, when the optically active acetate (S)-2f (99% ee) was applied, axially chiral allenoic acids (Sa)-3gf (99% ee) and (Sa)-3kf (97% ee) could be obtained with highly efficient chirality transfer (Scheme 2a). This method may open a new avenue for developing practical and synthetically useful methodologies for the synthesis of optically active allenoic acids. Meanwhile, this result indicated that the coordination of acetates and ruthenium species dictated the regioselectivity of alkyne insertion and the stereoselectivity of β-OAc elimination.
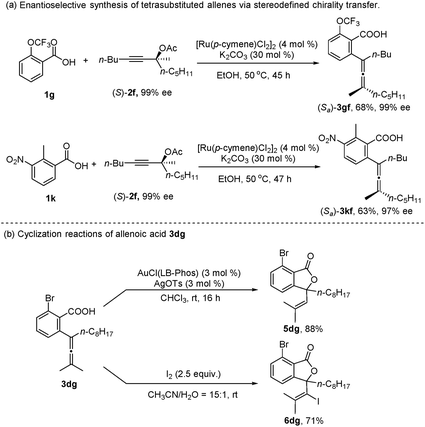 |
| Scheme 2 Synthetic applications. | |
To further explore the synthetic utility of this method, several synthetic applications were studied (Scheme 2b). The allenoic acid 3dg was easily transformed into the lactone 5dg by treatment with AuCl(LB-Phos) and AgOTs.13 This allenoic acid may also undergo an iodolactonization reaction with iodine to afford 6dg in 71% yield.
To gain insight into the mechanism of this methodology, several control experiments were carried out. Firstly, when 2-fluorobenzoic acid 1b was added to a mixture of CD3OD and D2O (2
:
1), the corresponding benzoic acid D-1b with 95% deuterium incorporation was obtained (Scheme 3a), indicating that the C–H activation step was reversible in the catalytic system. Subsequently, the parallel reactions of 1b and D-1b with 2a were conducted. We measured the reaction rate (k) of both 1b and D-1b by monitoring the concentration of the product 3ba by NMR from 2.5 h to 8 h (Scheme 3b and Fig. 1). Then, the primary kinetic isotope effect of 3.6 was observed. These results suggest that C–H bond cleavage is the rate-determining step.14
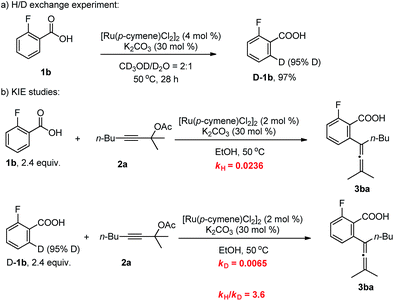 |
| Scheme 3 Mechanistic studies. | |
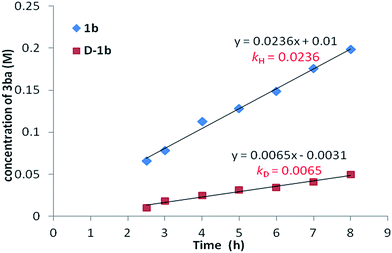 |
| Fig. 1 Plot of the concentrations of 3bavs. time. | |
In addition, a first-order dependence of the initial rate on the amount of the Ru catalyst was established (Fig. 2a, see the ESI† for details). The reaction orders of each reactant were also measured by using 2-fluorobenzoic acid 1b and propargylic acetate 2a. Both 1b and 2a follow the first-order reaction rate law, according to the linear relationship with ln([1b]) vs. reaction time: ln([1b]) = −k1t + ln[1b0] (Fig. 2b) and ln([2a]) = −k2t + ln[2a0] (Fig. 2c). Based on these data, we may give the rate equation as d[3ba]/dt = k[Ru]·[1b]·[2a].
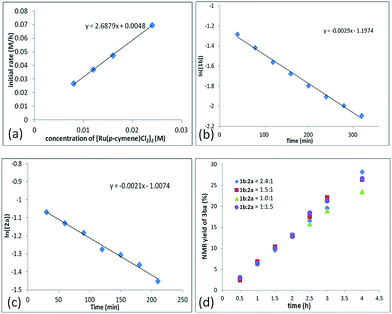 |
| Fig. 2 The dependence of the initial reaction rate on [Ru(p-cymene)Cl2]2 (a), 2-fluorobenzoic acid 1b (b), and propargylic acetate 2a (c). NMR yield of 3bavs. time with different molar ratios of 1b and 2a (d). | |
To further understand the role of benzoic acid on the reaction, four experiments were conducted by using different molar ratios of 2-fluorobenzoic acid 1bvs. propargylic acetate 2a (Fig. 2d). The yield vs. time profile is almost the same in the initial four hours, indicating that the loading of benzoic acid has a very limited effect on the formation of the final product; the excess benzoic acid did not accelerate the formation of the product greatly.15 In addition, we observed that the reaction failed to afford the expected product in the absence of k2CO3, indicating a CMD process for the C–H cleavage. However, due to its catalytic nature, the role of benzoic acid as a Brønsted acid to promote the insertion process from Int 2 to Int 3 cannot be excluded.16
Based on these investigations above, the proposed catalytic cycle is illustrated in Scheme 4. Firstly, the C–H activation step leads to the formation of the cyclic intermediate Int 1via a CMD process. Subsequently, Int 2 is generated by the coordination of the carbonyl unit in acetate with the Ru in the cycloruthenated species, which subsequently undergoes the syn-insertion of a C–C triple bond to afford Int 3. After a syn-β-OAc elimination step, the allenylation product was generated and the ruthenium species was released to restart the cycle. It should be noted that the acetate plays an important role in the syn-insertion as well as the syn-β-OAc elimination step.
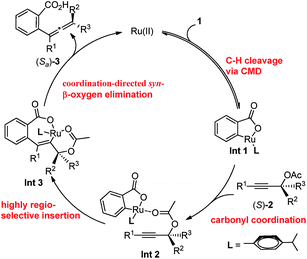 |
| Scheme 4 A possible mechanism. | |
Conclusions
In conclusion, we have established a new strategy to access allenoic acids, which is based on ruthenium catalysed carboxylic acid-directed C–H allenylation of benzoic acids with propargylic acetates. The reaction is compatible with air and synthetically useful functional groups such as Cl, Br, I, and OCF3 are all tolerated. Optically active allenoic acids could also be prepared through highly efficient chirality transfer. The formed allenoic acids could be transformed to lactones efficiently under mild conditions.
Conflicts of interest
There are no conflicts to declare.
Acknowledgements
Financial support from the National Natural Science Foundation of China (21690063) and National Basic Research Program of China (2015CB856600) is greatly appreciated. Shengming Ma is a Qiu Shi Adjunct Professor at Zhejiang University. We thank Anni Qin in our group for reproducing the results of 3dc, 3hb, and (Sa)-3gf.
Notes and references
-
(a)
Modern Allene Chemistry, ed. N. Krause and A. S. K.Hashmi, Wiley-VCH, Weinheim, 2004 Search PubMed;
(b)
Cumulenes and Allenes in Science of Synthesis, ed. N. Krause, Thieme, Stuttgart, 2008, vol. 44 Search PubMed;
(c)
Handbook of Cyclization Reactions, ed. S. Ma, Wiley-VCH, Weinheim, 2010, vol. 1 Search PubMed.
-
(a) A. Hoffmann-Röder and N. Krause, Angew. Chem., Int. Ed., 2002, 41, 2933 CrossRef;
(b) A. Hoffmann-Röder and N. Krause, Angew. Chem., Int. Ed., 2004, 43, 1196 CrossRef PubMed;
(c) S. Ma, Chem. Rev., 2005, 105, 2829 CrossRef PubMed;
(d) S. Yu and S. Ma, Chem. Commun., 2011, 47, 5384 RSC;
(e) S. Yu and S. Ma, Angew. Chem., Int. Ed., 2012, 51, 3074 CrossRef CAS PubMed;
(f) R. Zimmer and H.-U. Reissig, Chem. Soc. Rev., 2014, 43, 2888 RSC;
(g) B. Alcaide, P. Almendros and C. Aragoncillo, Chem. Soc. Rev., 2014, 43, 3106 RSC.
- For selected reviews, see:
(a) S. Ma, Acc. Chem. Res., 2003, 36, 701 CrossRef CAS;
(b) S. Ma, Acc. Chem. Res., 2009, 42, 1679 CrossRef CAS PubMed;
(c) B. Alcaide, P. Almendros and T. M. Campo, Chem.–Eur. J., 2010, 16, 5836 CrossRef CAS PubMed;
(d) J. Ye and S. Ma, Acc. Chem. Res., 2014, 47, 989 CrossRef CAS PubMed. For selected examples on cyclization of allenoic acids, see:
(e) S. Ma, Z. Yu and S. Wu, Tetrahedron, 2001, 57, 1585 CrossRef CAS;
(f) S. Ma and Z. Yu, Angew. Chem., Int. Ed., 2002, 41, 1775 CrossRef CAS;
(g) S. Ma and Z. Yu, Angew. Chem., Int. Ed., 2003, 42, 1955 CrossRef CAS PubMed;
(h) S. Ma and Z. Gu, J. Am. Chem. Soc., 2005, 127, 6182 CrossRef CAS PubMed;
(i) Z. Gu and S. Ma, Angew. Chem., Int. Ed., 2006, 45, 6002 CrossRef CAS PubMed.
-
(a) R. W. Lang and H. J. Hansen, Helv. Chim. Acta, 1980, 63, 438 CrossRef CAS;
(b) J. A. Marshall, E. D. Robinson and A. Zapata, J. Org. Chem., 1989, 54, 5854 CrossRef CAS;
(c) C. Li, X. Wang, X. Sun, Y. Tang, J. Zheng, Z. Xu, Y. Zhou and L. Dai, J. Am. Chem. Soc., 2007, 129, 1494 CrossRef CAS PubMed.
- E. Ferre, G. Gil, M. Bertrand and J. L. Petit, Appl. Microbiol. Biotechnol., 1985, 21, 258 CrossRef CAS.
-
(a) H. Arzoumanian, F. Cochini, D. Nuel, J. F. Petrignani and N. Rosas, Organometallics, 1992, 11, 493 CrossRef CAS;
(b) K. Matsushita, T. Komori, S. Oi and Y. Inoue, Tetrahedron Lett., 1994, 35, 5889 CrossRef CAS;
(c) J. A. Marshall, M. A. Wolf and E. M. Wallace, J. Org. Chem., 1997, 62, 367 CrossRef CAS;
(d) W.-F. Zheng, W. Zhang, J. Huang, Y. Yu, H. Qian and S. Ma, Org. Chem. Front., 2018, 5, 1900 RSC.
-
(a) J. H. Ford, C. D. Thompson and C. S. Marvel, J. Am. Chem. Soc., 1935, 57, 2619 CrossRef;
(b) J. C. Clinet and G. Linstrumelle, Synthesis, 1981, 875 CrossRef CAS;
(c) B. Miao, G. Li and S. Ma, Chem.–Eur. J., 2015, 21, 17224 CrossRef CAS.
-
(a) P. Crabbé, D. André and H. Fillion, Tetrahedron Lett., 1979, 20, 893 CrossRef;
(b) B. M. Trost and A. McClory, Org. Lett., 2006, 8, 3627 CrossRef CAS PubMed. For a recent account, see
(c) X. Huang and S. Ma, Acc. Chem. Res., 2019 DOI:10.1021/acs.accounts.9b00023.
- For selected books and reviews on C–H functionalization, see:
(a)
C–H activation, Topics in Current Chemistry, ed. J.-Q. Yu and Z. Shi, Springer-Verlag, Berlin Heidelberg, 2010, vol. 292 Search PubMed;
(b) O. Daugulis, H.-Q. Do and D. Shabashov, Acc. Chem. Res., 2009, 42, 1074 CrossRef CAS PubMed;
(c) J. Yamaguchi, A. D. Yamaguchi and K. Itami, Angew. Chem., Int. Ed., 2012, 51, 8960 CrossRef CAS PubMed;
(d) Z. Chen, B. Wang, J. Zhang, W. Yu, Z. Liu and Y. Zhang, Org. Chem. Front., 2015, 2, 1107 RSC;
(e) J. He, M. Wasa, K. S. L. Chan, Q. Shao and J.-Q. Yu, Chem. Rev., 2017, 117, 8754 CrossRef CAS PubMed;
(f) C. G. Newton, S.-G. Wang, C. C. Oliveira and N. Cramer, Chem. Rev., 2017, 117, 8908 CrossRef CAS PubMed.
-
(a) R. Zeng, S. Wu, C. Fu and S. Ma, J. Am. Chem. Soc., 2013, 135, 18284 CrossRef CAS;
(b) S. Wu, X. Huang, W. Wu, P. Li, C. Fu and S. Ma, Nat. Commun., 2015, 6, 7946 CrossRef;
(c) S. Wu, X. Huang, C. Fu and S. Ma, Org. Chem. Front., 2017, 4, 2002 RSC;
(d) Q. Lu, S. Greßies, F. J. R. Klauck and F. Glorius, Angew. Chem., Int. Ed., 2017, 56, 6660 CrossRef CAS PubMed;
(e) M. Sen, P. Dahiya, J. R. Premkumar and B. Sundararaju, Org. Lett., 2017, 19, 3699 CrossRef CAS PubMed.
- For selected reviews on carboxylic acids as directing groups, see:
(a) S. D. Sarkar, W. Liu, S. I. Kozhushkov and L. Ackermann, Adv. Synth. Catal., 2014, 356, 1461 CrossRef;
(b) M. P. Drapeau and L. J. Gooßen, Chem.–Eur. J., 2016, 22, 18654 CrossRef PubMed;
(c) M. Font, J. M. Quibell, G. J. P. Perry and I. Larrosa, Chem. Commun., 2017, 53, 5584 RSC; For C–H functionalization based allylation, see:
(d) A. S. Trita, A. Biafora, M. P. Drapeau, P. Weber and L. J. Gooßen, Angew. Chem., Int. Ed., 2018, 57, 14580 CrossRef CAS.
-
3ba: C16H19FO2, MW = 262.32, monoclinic, space group P121/c 1, final R indices [I > 2\s(I)], R1 = 0.0602, wR2 = 0.1483; R indices (all data), R1 = 0.0962, wR2 = 0.1764; a = 10.7724(13) Å, b = 15.7829(12) Å, c = 9.9065(12) Å, α = 90.00°, β = 116.778(15)°, γ = 90.00°, V = 1503.7(3) Å3, T = 296(2) K, Z = 4, reflections collected/unique 5576/2735 (Rint = 0.0294), number of observations [>2σ(I)]: 1722, parameters: 179. Supplementary crystallographic data have been deposited at the Cambridge Crystallographic Data Centre, CCDC 1877103.†.
- J. Zhou, C. Fu and S. Ma, Nat. Commun., 2018, 9, 1654 CrossRef.
- E. M. Simmons and J. F. Hartwig, Angew. Chem., Int. Ed., 2012, 51, 3066 CrossRef CAS.
- For reviews on ruthenium-catalyzed C–H activations, see:
(a) P. B. Arockiam, C. Bruneau and P. H. Dixneuf, Chem. Rev., 2012, 112, 5879 CrossRef CAS;
(b) L. Ackermann, Acc. Chem. Res., 2014, 47, 281 CrossRef CAS PubMed;
(c) P. Nareddy, F. Jordan and M. Szostak, ACS Catal., 2017, 7, 5721 CrossRef CAS. For acid-mediated Ru-catalyzed C–H activation, see:
(d) E. F. Flegeau, C. Bruneau, P. H. Dixneuf and A. Jutand, J. Am. Chem. Soc., 2011, 133, 10161 CrossRef CAS PubMed.
- We thank the referee for the suggestion of this possibility..
Footnote |
† Electronic supplementary information (ESI) available. CCDC 1877103. For ESI and crystallographic data in CIF or other electronic format see DOI: 10.1039/c9sc00603f |
|
This journal is © The Royal Society of Chemistry 2019 |
Click here to see how this site uses Cookies. View our privacy policy here.