DOI:
10.1039/D3MA00042G
(Paper)
Mater. Adv., 2023,
4, 2119-2126
High birefringence liquid crystals with a wide temperature range and low melting point for augmented reality displays†
Received
25th January 2023
, Accepted 30th March 2023
First published on 31st March 2023
Abstract
A series of tolane liquid crystals terminated by but-3-enyl with high performance (such as high clearing point, low melting point, high birefringence Δn, low viscosity γ1, and broad nematic phase interval) were designed and synthesized in accordance with the phase-only liquid-crystal-on-silicon (LCoS) device requirements for AR displays. The effects of the lateral fluoro-substituent, central alkyne bond, terminal group, and flexible n-alkyl chain on the thermal properties were systematically investigated, and density functional theory (DFT) calculations of molecular conformation and aspect ratio were used to corroborate the experimental results. Further, their properties of phase transition temperature, birefringence, and viscosity were comprehensively evaluated in two typical LC mixtures (001, P02-F). The results indicate that our target compounds can effectively lower the melting point, broaden the working temperature interval, and maintain a high Δn and low γ1 for three LC mixtures. One new LC mixture by employing compound 2TFV as a LC dopant exhibited a high Δn (∼0.26), moderate γ1 and dielectric anisotropy (∼6.3), and broad temperature adaptability (−31.3 °C to 91.3 °C), and achieved 2.08π phase modulation, 5 V operation voltage, and 2.89 ms response time at 40 °C in a reflective LCoS device.
1. Introduction
Broad temperature adaptability is a key performance evaluator for the widespread applications of all optoelectronic devices, which have attracted significant attention in various fields, such as proton exchange membrane fuel cells,1,2 perovskite solar cells,3,4 lithium ion batteries,5–7 supercapacitors,8,9 and liquid crystal (LC) devices.10–13 Augmented reality (AR) displays, one of the emerging research hotspots of LC devices,14–17 are subject to device failure at low temperature resulting from the temperature-dependent properties of the LC materials used in AR displays. Therefore, there is an urgent need to find effective ways to lower the working temperature of LC devices to enhance their ambient temperature tolerance.
Currently, there are three common methods to improve the low-temperature performance of LC devices: high driving voltage,18 external auxiliary heating,19,20 and low-temperature reinforcement technology.21 Although their ambient temperature tolerance can be improved with the help of the outside world, the low-temperature performance of LC devices depends mainly on the LC mixtures used. Therefore, the most direct and effective way is to develop LC mixtures with a low melting point (Tm) and wide working temperature range. As we know, the LC materials used in AR displays require high birefringence (Δn) to obtain 2π phase modulation and a fast response time.22–25 Unfortunately, the high Δn property requires large π–π conjugations in LC molecules,26–28 which inevitably lead to increased intermolecular interaction, further increasing the Tm of LC mixtures and making them unusable. In order to develop practical LC mixtures for AR displays, we usually start with fine molecular engineering strategies to arrange the synthons for LC compounds with excellent performance and then blend them to form one LC mixture with the desired properties.29 LC mixtures are mainly formulated by mixing an optical regulatory component, an electrical regulatory component, a LC diluter, and a wide-temperature modulation component.30 The preferred optical regulatory components adopt a tolane or bis-tolane unit as the molecular skeleton to obtain high Δn and low Tm.31–33 Lateral fluorine substituents and terminal polar groups are utilized to obtain electrical regulatory components with large dielectric anisotropy (Δε) and low Tm.34,35 LC diluters containing an olefin terminal group have been reported to display low Tm and rotational viscosity (γ1).36 Wide-temperature modulation components with a high clearing point (Tc) and relatively low Tm are realized with three-ring molecular structures.37–39 Overall, these individual parameters (such as high Δn, large Δε, low γ1, high Tc, and low Tm) or combined parameters have been achieved in one component through molecular engineering strategies. However, there is still a lack of theoretical guidance to realize the required properties of high Δn, large Δε, low γ1, high Tc and low Tm in one LC mixture for AR displays.
From the aforementioned information, we proposed to design and synthesize target compounds with high performance (broadened nematic phase interval, low Tm, high Δn, high Tc and relatively low γ1) and further employ them to improve the ambient temperature tolerance of a high-Δn LC mixture for AR displays. As shown in Fig. 1, our molecular tailoring strategy is summarized as follows: (1) one tolane central core was adopted as the molecular skeleton to achieve high Δn, low Tm and low γ1; (2) many functional groups, including a trans-cyclohexyl core,40,41 ethylene bridge,41,42 buty-3-enyl terminal group43 and lateral fluorine atom,44–46 were introduced to decrease the melting point; (3) the three-ring molecular structure was utilized to maintain high Tc and the broaden LC phase interval.47 Meanwhile, the effects of the lateral fluoro-substituent, central alkyne bond, terminal group and flexible n-alkyl chain on the thermal properties were systematically investigated. Research results indicate that the target compounds meet our molecular design goal; thus, they were further employed as LC dopants in one LC mixture to achieve 2π phase modulation at 5 V driving voltage and a 240 Hz frame rate for AR displays.
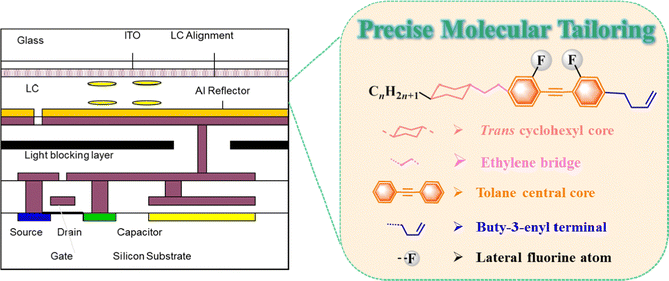 |
| Fig. 1 Molecular tailoring strategy of target compounds with many cooperating functional groups for LCoS devices in AR displays. | |
2. Experimental section
2.1. Materials
Tetrabutylammonium bromide (TBAB) and tetrakis(triphenylphosphine) palladium (Pd(PPh3)4) were purchased from Aladdin Reagent Company. Trimethylamine (Et3N), tetrahydrofuran (THF), potassium hydroxide (KOH), methylbenzene (PhMe) and other reagents were purchased from Sinophinese Chemical Reagent Company. The 2-(4-bromophenethyl)-1,3-dioxolane, 2-(4-bromo-3-fluorophenethyl)-1,3-dioxolane and methyltriphenylphosphonium bromide (CH3BrPPh3) were purchased from Xi’an Caijing Opto-Electrical Science & Technology Co. Ltd and used as received. The parent LC mixture (001, Δn = 0.0790, Tm = 9.4 °C, Tc = 112.9 °C, γ1 = 141.4 mPa s) was provided by Xi’an Modern Chemistry Research Institute. The synthesis procedures and characterized data of target compounds are shown in the ESI.†
2.2. Characterization and measurements
Four traditional spectral methods were used, including 1H-nuclear and 13C-nuclear magnetic resonance (1H NMR and 13C NMR) spectra (ECZ400R/S1, Nippon Electronics Co), Fourier transform infrared spectrometry (IR, FTIR Tensor27, Brucker, Germany) and gas phase mass spectrometry (GC-MS, DSQ II, Thermo Fisher Scientific Co., LTD). High performance liquid chromatography (HPLC, LC-20AT, Shimadzu International Trading Co., LTD) and gas chromatography (GC, GC-2014C, Shimadzu, Japan) were employed to detect the purities of the target compounds and intermediates.
Gaussian 09 software was employed to carry out DFT calculations for our target compounds by applying the B3LYP/6-311G (d,p) method48,49 and we obtained their molecular steric configurations and molecular aspect ratios. Their geometric data are listed in the electronic supplementary information. In addition, frequency calculations were performed to ascertain whether the optimized geometry is without any imaginary frequency. The mesomorphic properties of target compounds 4TV, nTFV, 4FTV and 4FTFV were tested by differential scanning calorimeter (DSC) and polarizing optical microscope (POM). Under an N2 atmosphere, the Shimadzu DSC-60 instrument was utilized to measure the phase transition temperatures with a heating/cooling rate of 10 °C min−1. The POM (LEICA DM2500P) instrument was equipped with a Linkam THMS600 hot stage to observe the liquid crystal phase state. The phase transition temperatures of LC mixtures were measured by DSC (TA Instruments Q100) in nitrogen at heating/cooling rate of 2 °C min−1.
The molecular structures and compositions of the fluoro-tolane-based LC mixture P02-F have been previously reported.36 The target compounds 4TV, 4TFV, 4FTV, 4FTFV and 2TFV were added into parent LC mixture 001 at a mass fraction of 15% to prepare five new LC mixtures, termed A, B, C, D and E, respectively. Their Δn values were measured at 20 °C by an Abbe refractometer using a 589 nm wavelength light source, then extrapolated experimental results were obtained by the host–guest method. Their γ1 values were measured at 25 °C by a multi-channel liquid crystal evaluation system (Model 6254, TOYO Corporation, Japan). Compound 2TFV (15 wt%) was dissolved in the LC mixture P02-F to prepare a new LC mixture F. The Δn and γ1 values of the LC mixtures (P02-F, F, SNUP03 and SNUP04) were calculated by the measured phase retardation and transient current methods.50 The dielectric anisotropy (Δε) values of LC mixtures SNUP03 and SNUP04 were measured with a multifrequency LCR meter (HP-4274). All measurements for LC mixtures (P02-F, F, SNUP03 and SNUP04) were carried out at a temperature of 40 °C and a wavelength of 633 nm.
3. Results and discussion
3.1. Synthesis and characterization
All the chemicals were from commercial suppliers and used without further purification. The aryl iodine compounds nxI were synthesized through sequential Grignard reaction, Wolff–Kishner-Huang-Minlon reduction reaction and iodization, in accordance with our previous work.51 Many efficient methods have been reported to create tolane LCs, mainly including the elimination reaction of alkenyl halide, the Sonogashira coupling reaction and the Fritsch–Buttenberg–Wiechell rearrangement. Currently, the most efficient method seems to be the Sonogashira reaction; therefore, the key intermediates nxyT were synthesized by palladium-catalysed Sonogashira reaction with different acetylenic alcohols and aryl bromides in one pot to gain a high yield of about 50% with purities higher than 99% (HPLC).
Using aryl iodine compounds as raw materials (Scheme 1), the target compounds were synthesized by sequential Heck coupling reaction, Sonogashira coupling reaction, deprotection of aldehyde and Wittig reaction. Classical organic synthetic reactions were employed in the above route to ensure the fast and efficient synthesis of target compounds; the synthesis processes and characterized data are shown in the supporting information. All target molecules and intermediate compounds were characterized and confirmed by 1H NMR spectroscopy, 13C NMR spectroscopy, mass spectroscopy and infrared spectroscopy.
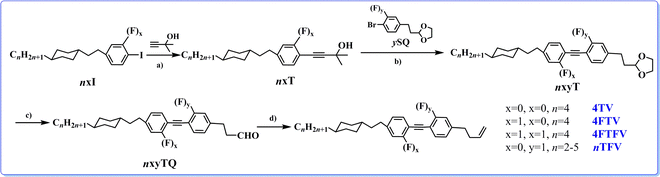 |
| Scheme 1 Synthetic routes of compounds nTFV, 4TV, 4FTV and 4FTFV. Reaction conditions: (a) Et3N, CuI, Pd(PPh3)4, 80 °C, 8 h; (b) PhMe: H2O (5 : 1, V/V), KOH, TBAB, Pd(PPh3)4, 80 °C, 8 h; (c) CHOOH, THF, 55 °C; (d) CH3BrPPh3, THF, −15 °C. | |
3.2. Liquid crystal characterization
The phase transition temperatures and associated enthalpy changes of target compounds nTFV, 4TV, 4FTV and 4FTFV were measured by differential scanning calorimetry (DSC). With reference to the reported mesophase textures and results from polarizing optical microscopy (POM), their liquid crystal phase textures were further confirmed and the corresponding data are summarized in Table S1 (ESI†). The POM pictures and DSC thermograms showed that all the target compounds display a stable nematic phase and a broad LC phase temperature range during the heating and cooling processes. In particular, the fluorine-substituted compound 4FTFV possessed the broadest nematic temperature interval of 110.2 °C upon heating.
The representative DSC thermograms of compounds 2TFV and 3TFV are presented in Fig. 2(a and d). Compound 2TFV exhibited a melting transition at 33.9 °C and a nematic to isotropic phase transition at 127.4 °C upon heating. The cooling process looks almost identical to the heating scan, except that a very large supercooled nematic mesophase range (119.1 °C) is observed, further indicating possible good low-temperature performance. Compound 2TFV exhibited the typical schlieren texture of nematic mesophase upon heating and cooling (Fig. 2(b and c)). Similar to those of compound 2TFV, the POM pictures and DSC thermogram of compound 3TFV show an enantiotropic nematic mesophase with a broad temperature interval.
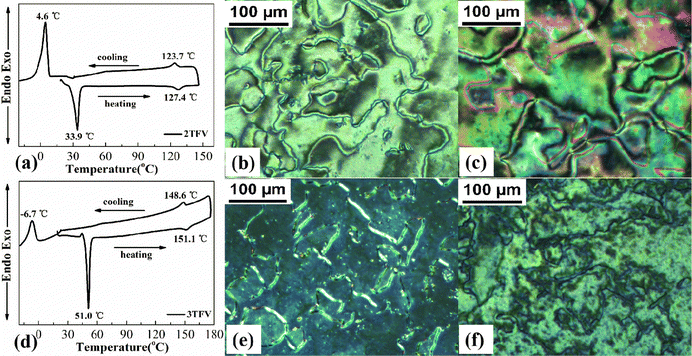 |
| Fig. 2 DSC curves and POM images (×200) of compounds 2TFV and 3TFV. (a) DSC curve of 2TFV; (b) schlieren texture of the nematic mesophase for 2TFV at 56 °C on cooling; (c) schlieren texture of the nematic mesophase for 2TFV at 120 °C on heating. (d) DSC curve of 3TFV; (e) a nematic texture with surface disclination lines for 3TFV at 127 °C on cooling; (f) a nematic thread-like texture for 3TFV at 136 °C on heating. | |
3.3. Thermal properties
The development of new LCs and an in-depth understanding of the correlation between molecular structure and thermal properties are essential for the further development of LC materials for LC photonics. Therefore, we investigated the effects of the lateral fluoro-substituent, central alkyne bond, terminal group and flexible n-alkyl chain on the thermal properties and the corresponding results are displayed in Fig. 3.
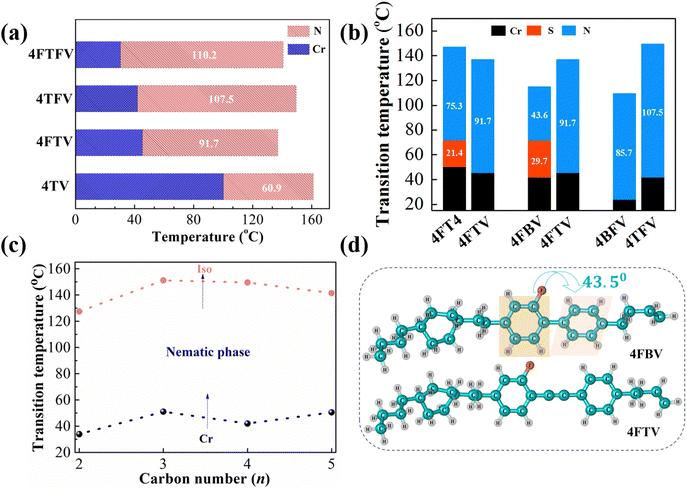 |
| Fig. 3 (a) The mesophase ranges of compounds 4FTFV, 4TFV, 4FTV, and 4TV during heating; (b) effect of the acetylene bond and terminal alkenyl on phase transition temperature; (c) transition behaviour of the compounds nTFV: dependence of the transition temperatures on the carbon number (n) of the terminal alkyl chain. (d) Molecular structures of compounds 4FBV and 4FTV optimized at the DFT/b3lyp/6-311G (d,p) level of theory. Cr: crystal; N: nematic phase interval; S: smectic phase interval; Iso: isotropic liquid. | |
Overall, Fig. 3a shows that the melting points of the fluorinated LCs are lower than that of the non-fluorinated LC. The melting point of the non-fluorinated compound 4TV is 100.2 °C and the melting points of the mono-fluorinated compounds 4TFV and 4FTV are respectively 58.2 °C and 54.8 °C lower than that of 4TV. Two ortho fluoro-substituents further decreased the melting point of 4TV to the lowest value (30.5 °C), which is respectively 11.5 °C and 14.9 °C lower than those of mono-fluorinated 4TFV and 4FTV. It can be seen that the introduction of a lateral fluorine substituent is beneficial in decreasing the melting point of LC molecules, probably because lateral fluorine substituents reduce the degree of compact stacking between liquid crystal molecules. Meanwhile, the nematic phase temperature intervals of the fluorinated LC molecules 4FTFV, 4TFV and 4FTV are respectively 49.3 °C, 46.6 °C and 30.8 °C wider than that of the non-fluorinated LC molecule 4TV. It can be found that the introduction of a lateral fluorine substituent broadens the LC phase intervals. Furthermore, compound 4TFV shows a lower melting point and a wider liquid crystal phase interval than compound 4FTV, meaning that the lateral fluorine substituent group located on the second benzene ring facilitates a broader nematic phase interval.43 This may be due to the lateral fluorine substituent located on the second benzene ring helping to increase the molecular aspect ratio (Table 1) and thus promoting the stability of the LC phase.45
Table 1 The DFT-calculated molecular length (L), width (W), and aspect ratios (L/W) of compounds 4TV, 4TFV, 4FTV and 4FTFV and their effects on the properties of LC mixture 001
Compd. |
L (Å) |
W (Å) |
L/W |
T
m (°C)a |
Nematic range (°C)a |
Δna |
γ
1 (mPa s)a |
Δnb |
85 wt% 001 + 15 wt% single compound.
Extrapolated experimental results.
|
4TV
|
27.29 |
4.34 |
6.28 |
8.1 |
114.9 |
0.1095 |
391.9 |
0.2823 |
4TFV
|
27.25 |
5.32 |
5.12 |
1.4 |
117.8 |
0.1081 |
227.7 |
0.2730 |
4FTV
|
27.26 |
5.47 |
4.98 |
1.7 |
119.6 |
0.1067 |
159.3 |
0.2636 |
4FTFV
|
27.24 |
5.35 |
5.09 |
−0.2 |
116.5 |
0.1032 |
115.0 |
0.2418 |
3BFV
|
23.54 |
4.50 |
5.23 |
−7.6 |
113.9 |
0.0981 |
145.6 |
0.2063 |
The molecular structures of reference LC compounds 4FBV, 4BFV, 3BFV and 4FT4 are shown in Fig. S5 (ESI†). From Fig. 3b, the melting and clearing points of compound 4FT4 with an n-butyl terminal group are 50.4 °C and 147.1 °C, respectively, with a liquid crystal phase interval of 96.7 °C and the presence of a smectic phase. Comparing 4FT4 with 4FTV, it is found that replacing the n-butyl terminal group with a buty-3-enyl group could decrease the melting point and limit the smectic phase while maintaining a high clearing point and broad nematic phase interval. It has been speculated that the buty-3-enyl group increases the π–π interactions of the carbon–carbon double bond to inhibit the intertwining of the terminal alkyl chain, further facilitating the stability of the nematic phase.43
In Fig. 3d, it is seen that the biphenyl rings of 4FBV are twisted to 43.5°, while the analogue 4FTV containing a central alkyne bond is almost planar, which is conducive to molecular length and π–π interactions. As shown in Fig. 3b, the melting and clearing points of compound 4FBV are 41.8 °C and 115.1 °C, respectively, with a liquid crystal phase interval of 73.3 °C and the presence of a smectic phase. Comparing 4FBV with 4FTV, it is seen that the insertion of the alkyne bond into the biphenyl rings broadened the mesomorphic phase temperature range, enhanced the melting and clearing points and suppressed the smectic phase.
Fig. 3c presents the effect of terminal alkyl chains (n = 2–5) on the melting and clearing points of compounds nTFV during the heating process. For the melting point, a clear even–odd effect is exhibited: compounds nTFV with even terminal chain lengths (n = 2, 4) exhibit lower melting points than those with odd terminal chain lengths (n = 3, 5). Meanwhile, compound 2TFV has a lower clearing point than compound 3TFV, but the longer the terminal alkyl chains (n = 4, 5) are, the lower the clearing points.
3.4. Physical properties
The fast response speed of liquid crystals is mainly associated with their properties of low viscosity and high Δn.22,23 Therefore, we investigate the relationship between their molecular structures and their properties of birefringence and viscosity to lay the theoretical foundation for designing high Δn and low viscosity LC molecules. The new LC mixtures were prepared by separately adding compounds 4TV, 4TFV, 4FTV, 4FTFV and 3BFV to the parent LC mixture 001 at a mass ratio of 15% and their Δn values all increased, as shown in Table 1. Compared to reference compound 3BFV, the target molecules 4TV, 4TFV, 4FTV and 4FTFV exhibit larger Δn values of 0.28, 0.27, 0.26 and 0.24, respectively. It is obvious that the Δn value decreases from 0.28 to 0.24 with the increase of the lateral fluorine substitution number, which is attributable to the introduction of fluorine atoms into the benzene ring increasing the molecular width and weakening the intermolecular π–π conjugation.44,49 Meanwhile, the numerical order of the viscosity value is consistent with that of birefringence, which indicates that large π-conjugation is beneficial to high birefringence, but not to low viscosity.
In addition, we evaluated the effects on the melting point and nematic phase temperature range of LC mixture 001 (Fig. 4). The parent LC mixture 001 has a melting point of 9.4 °C, a clearing point of 112.9 °C and a nematic phase temperature interval of 103.5 °C. Each of the five compounds 4TV, 4FTV, 4TFV, 4FTFV and 2TFV (15 wt%) was dissolved in LC mixture 001, yielding five new LC mixtures, termed A, B, C, D and E, respectively. It was found that, compared with parent LC mixture 001, the melting points of the new mixtures were lower and their clearing points were increased, displaying wider nematic phase temperature intervals. Among these five compounds, compound 2TFV decreased the original melting point of 9.4 °C to the lowest temperature (−1.6 °C) and maintained the widest nematic range (119.7 °C) of the LC mixtures.
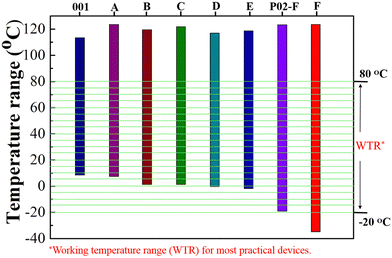 |
| Fig. 4 Effect of target compounds on the temperature range of LC mixtures. Each of five compounds 4TV, 4FTV, 4TFV, 4FTFV and 2TFV (15 wt%) was dissolved in LC mixture 001, yielding new LC mixtures A, B, C, D and E. Compound 2TFV (15 wt%) was dissolved in the LC mixture P02-F, yielding new LC mixture F. | |
3.5. Properties in high-Δn LC mixtures
The above results show that the target compounds display low melting point, wide nematic phase temperature interval, large birefringence and low rotational viscosity. They also effectively lower the melting point of LC mixture 001, broaden its liquid crystal phase temperature interval, enhance its birefringence and maintain low rotational viscosity. To further evaluate their properties in high-Δn LC mixtures, we selected the high-Δn LC mixture P02-F containing fluoro-tolane to investigate the effects on the properties of birefringence, rotational viscosity, low temperature and LC phase temperature interval (Table S2 and Fig. 4, ESI†).
Compound 2TFV possesses the advantages of low melting point, wide nematic phase temperature interval and high birefringence and, therefore, it was selected as a typical representative of the target compounds to carry out the next study. Further experimental results showed that the addition of compound 2TFV could lower the melting point of LC mixture P02-F to −34 °C, broaden its LC phase interval to 157.4 °C, and reduce its rotational viscosity to 185.5 mPa s while maintaining high birefringence (0.28). This predicts that the target compounds may have positive effects on the fast response time and wide operating temperature range of high-Δn LC mixtures. Thus, we prepared two LC mixtures SNUP03 and SNUP04 (Table S4 and S5, ESI†) according to the performance requirements of phase-only LCoS devices for AR displays22,23 and further evaluated the comprehensive effect of compound 2TFV on the performance of a high-Δn LC mixture; the test results are listed in Table 2.
Table 2 Measured properties of the two LC mixtures at T = 40 °C, λ = 633 nm, and f = 1 kHz
No. |
T
m (°C) |
T
c (°C) |
Δn |
Δε |
γ
1 (mPa s) |
K
11 (pN) |
V
th (V) |
SNUP03
|
−7.5 |
89.9 |
0.27 |
7.25 |
75.1 |
8.5 |
1.04 |
SNUP04
|
−31.3 |
91.3 |
0.26 |
6.31 |
97.4 |
7.9 |
1.18 |
As expected, adulteration with compound 2TFV lowers the melting point of LC mixture SNUP03 to −31.3 °C and broadens its LC phase temperature interval to 122.6 °C, meeting the operating temperature requirements for most indoor device applications. To further evaluate its effect on the response time of a phase-only liquid-crystal-on-silicon (LCoS) device for AR displays, we divided the phase change into nine levels (Table S3 and Fig. S5, ESI†) to evaluate the grayscale phase response. The phase-to-phase (PTP) response time was measured between every two phase levels and the experimental results are summarized in Table S4 (ESI†). In our experiment, the 2.08π phase change was achieved at an operation voltage of 5 V with a 3.12 μm transmissive LC cell. Since the response time is proportional to the square of the LC cell gap, the response time of the 1.56 μm reflective cell should be 4 times faster than that of the 3.12 μm transmissive cell. Therefore, the extrapolated average PTP response time of a 1.56 μm reflective LCoS panel is 2.89 ms, meaning that this phase-only LCoS could realize a 240 Hz refresh rate without overdrive circuitry. In recent years, the state-of-the-art LC material development for AR displays has reached a sub-millisecond response time for intensity modulation23,25 and a ∼2 ms response time for 2π phase modulation22,23,52 at 40 °C. To achieve broad temperature adaptability and fast response time, further research should focus on effective molecular tailoring strategies.
4. Conclusion
The molecular tailoring strategy was applied to obtain novel fluorinated tolane-liquid crystals comprised of a tolane central core, trans cyclohexyl core, ethylene bridge, buty-3-enyl terminal group, lateral fluorine atom and terminal alkyl chain. These target compounds exhibited enantiotropic nematic mesophases with a broadest nematic temperature interval of 110.2 °C and lowest melting point of 30.5 °C and the melting points of compounds nTFV exhibited a clear even–odd effect. The correlation between molecular structure and thermal properties indicated that the lateral fluoro-substituent and the buty-3-enyl terminal group helped to broaden the nematic phase interval, decrease the melting point and suppress the smectic phase, which corroborates the DFT calculations. Meanwhile, the fluorinated tolane liquid crystals with the buty-3-enyl terminal group displayed high Δn values (0.24–0.27) and can effectively lower the melting point, broaden the LC phase temperature interval, and maintain the high Δn and low γ1 of three LC mixtures (001, P02-F and SNUP03). Finally, we developed a new LC mixture (SNUP04) by mixing compound 2TFV and other compounds for phase-only LCoS-based AR displays, which could enable a 240 Hz frame rate at an operation voltage of 5 V and 2.08π phase change. This molecular tailoring strategy can be an effective way to accomplish the integration of many desired properties into one compound and the compound developed herein is confirmed to be an effective LC dopant to improve the ambient temperature tolerance of LC mixtures.
Conflicts of interest
There are no conflicts to declare.
Acknowledgements
The authors thank for the financial support by the National Natural Science Foundation of China (62105194, 52273186 and 51873100), the China Postdoctoral Science Foundation (2022T150394), Sanqin scholars innovation teams in Shaanxi Province, China, International Science and Technology Cooperation Project of Shaanxi Province, China (2021KW-20) for financial support of this work. We are grateful to Professor Wenliang Wang at Shaanxi Normal University for theoretical calculations.
References
- K. S. Lee, S. Maurya and Y. S. Kim,
et al., Intermediate temperature fuel cells via an ion-pair coordinated polymer electrolyte, Energy Environ. Sci., 2018, 11, 979–987 RSC.
- X. Zhang, X. Fu and S. Yang,
et al., Design of sepiolite-supported ionogel-embedded composite membranes without proton carrier wastage for wide-temperature-range operation of proton exchange membrane fuel cells, J. Mater. Chem. A, 2019, 7(25), 15288–15301 RSC.
- Y. Chen, S. Tan and N. Li,
et al., Self-elimination of intrinsic defects improves the low-temperature performance of perovskite photovoltaics, Joule, 2020, 4(9), 1961–1976 CrossRef CAS.
- G. Li, Z. Su and M. Li,
et al., Structure and Performance Evolution of Perovskite Solar Cells under Extreme Temperatures, Adv. Energy Mater., 2022, 12(48), 2202887 CrossRef CAS.
- C. S. Rustomji, Y. Yang and T. K. Kim,
et al., Liquefied gas electrolytes for electrochemical energy storage devices, Science, 2017, 356, eaal4263 CrossRef PubMed.
- L. Cheng, Y. Wang and J. Yang,
et al., An Ultrafast and Stable Li-Metal Battery Cycled at – 40 °C, Adv. Funct. Mater., 2022, 2212349 Search PubMed.
- T. Ma, Y. Ni and Q. Wang,
et al., Optimize Lithium Deposition at Low Temperature by Weakly Solvating Power Solvent, Angew. Chem., Int. Ed., 2022, 134, e202207927 CrossRef.
- Q. Liu, A. Zhao and X. He,
et al., Full Temperature All Solid State Ti3C2Tx/Aramid Fiber Supercapacitor with Optimal Balance of Capacitive Performance and Flexibility, Adv. Funct. Mater., 2021, 31, 2010944 CrossRef CAS.
- X. Hou, Q. Zhang and L. Wang,
et al., Low-temperature-resistant flexible solid supercapacitors based on organohydrogel electrolytes and microvoid-incorporated reduced graphene oxide electrodes, ACS Appl. Mater. Interfaces, 2021, 13, 12432–12441 CrossRef CAS PubMed.
- G. E. Bauman, J. M. McCracken and T. J. White, Actuation of Liquid Crystalline Elastomers at or Below Ambient Temperature, Angew. Chem., Int. Ed., 2022, e202202577 CAS.
- Y. Chen, C. Zheng and W. Yang,
et al., Over 200 °C Broad Temperature Lasers Reconstructed from a Blue Phase Polymer Scaffold, Adv. Mater., 2022, 2206580 CrossRef CAS PubMed.
- W. Hu, L. Wang and M. Wang,
et al., Ultrastable liquid crystalline blue phase from molecular synergistic self-assembly, Nat. Commun., 2021, 12, 1440 CrossRef CAS PubMed.
- N. Gao, S. Jing and X. Kong,
et al., Low-temperature properties of nematic liquid crystal materials for display, Liq. Cryst., 2021, 48(11), 1593–1607 CrossRef CAS.
- J. Xiong, E. L. Hsiang and Z. He,
et al., Augmented reality and virtual reality displays: emerging technologies and future perspectives, Light: Sci. Appl., 2021, 10, 216 CrossRef CAS PubMed.
- Y. Li, Q. Yang and J. Xiong,
et al., 3D displays in augmented and virtual realities with holographic optical elements, Opt. Express, 2021, 29, 42696–42712 CrossRef CAS.
- K. Yin, E. L. Hsiang and J. Zou,
et al., Advanced liquid crystal devices for augmented reality and virtual reality displays: principles and applications, Light: Sci. Appl., 2022, 11, 1–22 CrossRef PubMed.
- Y. Li, S. Chen and H. Liang,
et al., Ultracompact multifunctional metalens visor for augmented reality displays, PhotoniX, 2022, 3, 29 CrossRef.
- M. Mrukiewicz, K. Kowiorski and P. Perkowski,
et al., Threshold voltage decrease in a thermotropic nematic liquid crystal doped with graphene oxide flakes, J. Biomed. Nanotechnol., 2019, 10, 71–78 CAS.
- M. Yousefi, S. M. Rozati and N. Najafi,
et al., Investigation of the effect of temperature on the structural, optical, electrical, and self-cleaning properties of ITO thin films, Appl. Phys. A: Mater. Sci. Process., 2020, 126(4), 455–459 Search PubMed.
- S. Yang, S. Wang and H. Liao,
et al., Improving the performance of Cu2ZnSnS4 thin film solar cell by engineering the ITO film thickness, Physica B, 2020, 589(10), 412196 CrossRef CAS.
- L. Yu and Y. S. Qian Technology, of rugged AM-LCD under lower temperature, Chin. J. Liq. Cryst. Disp., 2003, 18(5), 342–347 Search PubMed.
- R. Chen, Y. Huang and J. Li,
et al., High-frame-rate liquid crystal phase modulator for augmented reality displays, Liq. Cryst., 2019, 46(2), 309–315 CrossRef CAS.
- Y. Huang, Z. He and S. T. Wu, Fast-response liquid crystal phase modulators for augmented reality displays, Opt. Express, 2017, 25(26), 32757–32766 CrossRef CAS.
- Q. Yang, J. Zou and Y. Li,
et al., Fast-response liquid crystal phase modulators with an excellent photostability, Crystals, 2020, 10(9), 765 CrossRef CAS.
- H. Chen, F. Gou and S. T. Wu, Submillisecond-response nematic liquid crystals for augmented reality displays, Opt. Mater. Express, 2017, 7(1), 195–201 CrossRef CAS.
- R. Chen, L. Wang and Z. An,
et al., Effect of π-conjugation units on the liquid crystal and photovoltaic performance of heterocyclic pyridine-based compounds, Liq. Cryst., 2021, 48(15), 2178–2187 CrossRef CAS.
- R. Chen, Y. Qin and Z. An,
et al., The effect of phenyl ring on the physical properties of liquid crystals containing 4-pyridyl terminal group, Liq. Cryst., 2018, 45, 1825–1833 CrossRef CAS.
- N. Xie, S. Du and R. Chen,
et al., Synthesis and properties of benzoxazole-terminated mesogenic compounds containing tolane with high birefringence and large dielectric anisotropy, Liq. Cryst., 2021, 48, 1978–1991 CrossRef CAS.
- R. Dąbrowski, J. Dziaduszek and A. Ziółek,
et al., Low viscosity, high birefringence liquid crystalline compounds and mixtures, Opto-Electron. Rev., 2007, 15(1), 47–51 Search PubMed.
- H. Chen, Y. Liu and M. Chen,
et al., Research of Liquid-Crystal Materials for a High-Performance FFS-TFT Display, Molecules, 2023, 28, 754 CrossRef CAS PubMed.
- M. Bremer, M. Klasen Memmer and D. Pauluth,
et al., Novel liquid crystal materials with negative dielectric anisotropy for TV application, J. Soc. Inf. Disp., 2006, 14, 517–521 CrossRef CAS.
- M. Hu, J. Li and Z. Yang,
et al., Synthesis and properties of difluoromethyleneoxy-bridged liquid crystals terminated by 2,2-difluorovinyloxy group, Liq. Cryst., 2015, 42, 383–389 CrossRef CAS.
- Y. Arakawa, S. Kang and H. Tsuji,
et al., The design of liquid crystalline bistolane-based materials with extremely high birefringence, RSC Adv., 2016, 6, 92845–92851 RSC.
- N. Li, Z. Li and X. Zhang,
et al., Synthesis of 1, 4-bis (phenylethynyl) benzenes and their application as blue phase liquid crystal composition, Int. J. Mol. Sci., 2013, 14, 23257–23273 CrossRef PubMed.
- J. Li, X. Yang and N. Gan,
et al., The effect of lateral fluorination on the properties of phenyl-tolane liquid crystals, Liq. Cryst., 2015, 42, 397–403 CrossRef CAS.
- Q. Weng, L. Zhao and R. Chen,
et al., Syntheses of new diluents for medium birefringence liquid crystals materials, Liq. Cryst., 2019, 46, 700–707 CrossRef CAS.
- J. Li, M. Hu and R. Chen,
et al., Low dielectric loss and good miscibility of the tolane liquid crystals by tuning their lateral substituents, J. Mol. Liq., 2021, 325, 115236 CrossRef CAS.
- R. Dąbrowski, P. Kula and J. Herman, High birefringence liquid crystals, Crystals, 2013, 3, 443–482 CrossRef.
- R. Chen, L. Zhao and Z. An,
et al., Synthesis and properties of allyloxy-based tolane liquid crystals with high negative dielectric anisotropy, Liq. Cryst., 2017, 44, 2184–2191 CrossRef CAS.
- P. Kirsch and M. Bremer, Nematic liquid crystals for active matrix displays: molecular design and synthesis, Angew. Chem., Int. Ed., 2000, 39, 4216–4235 CrossRef CAS PubMed.
- M. Schadt, Linear and non-linear liquid crystal materials, electro-optical effects and surface interactions. Their application in present and future devices, Liq. Cryst., 1993, 14(1), 73–104 CrossRef CAS.
- Y. Goto, K. Kitano and T. Ogawa, Liquid crystals of some new
tolane derivatives containing a 1, 2-ethylene linkage, Liq. Cryst., 1989, 5(1), 225–232 CrossRef CAS.
- Y. Jiang, Z. An and P. Chen,
et al., Synthesis and mesomorphic properties of but-3-enyl-based fluorinated biphenyl liquid crystals, Liq. Cryst., 2012, 39(4), 457–465 CrossRef CAS.
- R. Chen, Z. An and W. Wang,
et al., Improving UV stability of tolane-liquid crystals in photonic applications by the ortho fluorine substitution, Opt. Mater. Express, 2016, 6(1), 97–105 CrossRef CAS.
- R. Chen, Z. An and F. Li,
et al., Synthesis and physical properties of tolane liquid crystals containing 2, 3-difluorophenylene and terminated by a tetrahydropyran moiety, Liq. Cryst., 2016, 43(4), 564–572 CrossRef CAS.
- S. Zhu, R. Chen and W. Zhang,
et al., Dissecting terminal fluorinated regulator of liquid crystals for fine-tuning intermolecular interaction and molecular configuration, J. Mol. Liq., 2020, 310, 113225 CrossRef CAS.
- M. Hu, Z. An and J. Li,
et al., Low mid-infrared absorption tolane liquid crystals terminated by 2, 2-difluorovinyloxyl: synthesis, characterization and properties, J. Mater. Chem. C, 2016, 4, 4939–4945 RSC.
- R. Chen, Z. An and W. Wang,
et al., Lateral substituent effects on UV stability of high-birefringence liquid crystals with the diaryl-diacetylene core: DFT/TD-DFT study, Liq. Cryst., 2017, 44(10), 1515–1524 CrossRef CAS.
- R. Chen, Y. Jiang and J. Li,
et al., Dielectric and optical anisotropy enhanced by 1, 3-dioxolane terminal substitution on tolane-liquid crystals, J. Mater. Chem. C, 2015, 3, 8706–8711 RSC.
- Z. Luo, F. Peng and H. Chen,
et al., Fast-response liquid crystals for high image quality wearable displays, Opt. Mater. Express, 2015, 5(3), 603–610 CrossRef.
- R. Chen, Z. An and X. Chen,
et al., Syntheses and properties of four-ring liquid crystals with ethylene and internal alkyne bridge, Chem. J. Chin. Univ., 2014, 35, 1433–1438 CAS.
- Y. Huang, E. Liao and R. Chen,
et al., Liquid-crystal-on-silicon for augmented reality displays, Appl. Sci., 2018, 8(12), 2366 CrossRef CAS.
|
This journal is © The Royal Society of Chemistry 2023 |