DOI:
10.1039/D2RA03034A
(Paper)
RSC Adv., 2022,
12, 16745-16750
Metal- and base-free tandem sulfonylation/cyclization of 1,5-dienes with aryldiazonium salts via the insertion of sulfur dioxide†
Received
13th May 2022
, Accepted 31st May 2022
First published on 6th June 2022
Abstract
A metal- and base-free 5-endo-trig sulfonylative cyclization between 1,5-dienes, aryldiazonium salts and SO2 (from SOgen) is presented. This method could successfully produce sulfonylated pyrrolin-2-ones in one pot with excellent regioselectivity and good-to-excellent yields. This strategy features mild reaction conditions and broad substrate scope. Moreover, a scale-up reaction and three synthetic applications demonstrate the practicality of this method. Lastly, control experiments indicate that the 5-endo-trig sulfonylative cyclization may proceed in a radical pathway.
Introduction
Pyrrolin-2-ones and their N-heterocyclic compound derivatives, widely exist in natural plants,1 pharmaceuticals2 and bioactive molecules.3 Similarly, sulfonyl groups are frequently found in pharmaceuticals4 and photoelectric materials5 due to their unique chemical properties. Numerous studies have indicated that the incorporation of sulfonyl groups into heterocycles could enhance their pharmacological activity.6 Therefore, great efforts have been devoted to explore efficient and straightforward methods to build sulfone-containing N-heterocyclic frameworks.
Radical cascade cyclization reactions represent a powerful strategy for the synthesis of functionalized cyclic structure, characterized by multiple C–C/C–X bond-forming in one step.7 The incorporation of sulfonyl group into heterocycles by radical cascade cyclization reactions has aroused extensive interest among scientists.8 In recent years, many sulfone-containing heterocyclic frameworks have been constructed by radical cascade cyclization reactions, such as sulfonylindoles,9 sulfonylindolins,10 sulfonylated pyrrolidines,11 sulfonylated phenanthridines,12 sulfonylated benzofurans,13 sulfonated oxazolines,14 sulfonylated spirocycles15 and others.16 In 2021, sulfonylated pyrrolinones were synthesized via sulfonylation/cyclization of 1,5-dienes with sulfonyl chlorides or sodium sulfinates by Wang and co-workers (Fig. 1a and b).17 However, due to the limited accessibility of sulfonyl chlorides and sodium sulfinates, these two methods suffered from a narrow range of substrates. Besides, transition metal (Cu and Ag), base and elevated temperature were essential in these transformations.
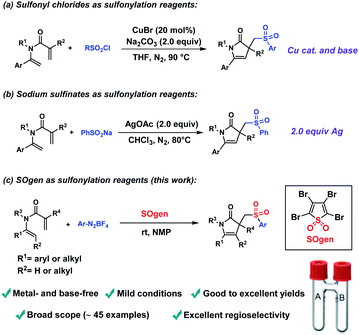 |
| Fig. 1 Overview of tandem sulfonylative cyclization of 1,5-dienes: (a) sulfonyl chlorides as sulfonylation reagents; (b) sodium sulfinates as sulfonylation reagents; (c) SOgen as sulfonylation reagents (this work). | |
On the other hand, direct insertion of sulfur dioxide (SO2) provides an alternative and efficient approach to introduce sulfonyl moiety into molecules.18,19 Recently, a cheap and bench-stable SO2 surrogate (SOgen) has been developed by our group, which has been successfully applied in several sulfonylation reactions.20 Inspired by Wang's work and our continuous interests in SO2 chemistry, we herein attempt to construct sulfonylated pyrrolinones using SOgen as SO2 surrogate (Fig. 1c). This transformation features metal- and base-free conditions and could proceed smoothly at room temperature to form sulfonylated pyrrolinones with excellent regioselectivity and good to excellent yields.
Results and discussion
We started the studies by evaluating the reaction between 1,5-diene (1a), 4-methylbenzenediazonium tetrafluoroborate (2a) and SO2 gas (from SOgen) under metal- and base-free conditions. Pleasingly, when the reaction was carried out in NMP at room temperature for 24 h, desired product 3a was successfully obtained in 91% yield with excellent regioselectivity (Table 1, entry 1).
Table 1 Optimization of reaction conditionsa

|
Entry |
Variation from std conditions |
Yield of 3ab (%) |
Standard conditions: chamber A, SOgen (0.80 mmol), 1-methyl-4-vinylbenzene (0.81 mmol), tetradecane (1.0 mL), at 100 °C for 10 min; chamber B, 1a (0.2 mmol, 1.0 equiv.), 2a (0.44 mmol, 2.2 equiv.), NMP (1.0 mL), at room temperature for 24 h under argon atmosphere. Yields were determined by 1H-NMR analysis using 1,3,5-trimethoxybenzene as an internal standard. Isolated yield in the parentheses. The reaction was set up in a 4 mL vial. |
1 |
None |
91 (87)c |
2 |
THF instead of NMP |
0 |
3 |
MeCN instead of NMP |
0 |
4 |
DCM instead of NMP |
0 |
5 |
Toluene instead of NMP |
0 |
6 |
MeOH instead of NMP |
0 |
7 |
DMSO instead of NMP |
21 |
8 |
DMF instead of NMP |
20 |
9 |
DMA instead of NMP |
56 |
10 |
3.5 equiv. of SO2 |
86 |
11 |
3.0 equiv. of SO2 |
80 |
12 |
2.5 equiv. of SO2 |
74 |
13 |
Air instead of Ar |
54 |
14d |
DABSO as SO2 surrogate |
71 |
15d |
Na2S2O5 as SO2 surrogate |
53 |
16d |
K2S2O5 as SO2 surrogate |
52 |
17d |
HOCH2SO2Na·H2O as SO2 surrogate |
0 |
Then we explored the influence of other solvents on this reaction, the target product (3a) was not obtained in most solvents, such as THF, MeCN, DCM, toluene and MeOH (Table 1, entries 2–6). When the solvent was DMA, DMF and DMSO, 3a was formed in only poor yields (Table 1, entries 7–9). Next, the amount of SO2 (from 2.5 equiv. to 4.0 equiv.) was investigated, and the results indicated that 4.0 equiv. was the best choice (Table 1, entries 10–12). Although this reaction could work under an air atmosphere, argon atmosphere proved to be more beneficial for the transformation (Table 1, entry 13). Finally, other sulfur dioxide surrogates were examined. The use of DABSO and inorganic SO2 surrogates (Na2S2O5 and K2S2O5) could both lead to the formation of product 3a but with lower yields (Table 1, entries 14–16). Unfortunately, rongalite reagent (HOCH2SO2Na·H2O) would hamper the reaction (Table 1, entry 17).
After determining the optimal reaction conditions, we began to explore the substrate scope of this reaction, and the results are summarized in Scheme 1. We first investigated the functional group compatibility of aryldiazonium tetrafluoroborates in the transformation. Alkyl substituted aryldiazonium tetrafluoroborates at the meta- or para-position of the phenyl ring proceeded well and afforded corresponding products 3b–3d in good yields (83–93%). While a methyl substituent at the ortho-position could lead to a lower yield (67%, 3e), possibly due to the steric hindrance. In addition, substrates with methoxy or phenoxy group delivered desired products 3f and 3g in 83% and 86% yield, respectively. Substrates bearing a biphenyl or 2-naphthyl group showed good reactivity, producing expected products (3h and 3i) in excellent yields. Notably, halogen groups were found to be well tolerated under the standard conditions (3j–3q). Moreover, substrates with electron-withdrawing groups such as MeCO–, PhCO–, CF3O–, MeSO2– were subject to the reaction conditions, and gave corresponding products (3r–3v) in good yields. In addition, heterocyclic diazonium salt was found to be compatible in the transformation (3w). Finally, aryldiazonium tetrafluoroborates with complicated substituent structures could also work smoothly to afford 3x and 3y in 92% and 90% yield, respectively.
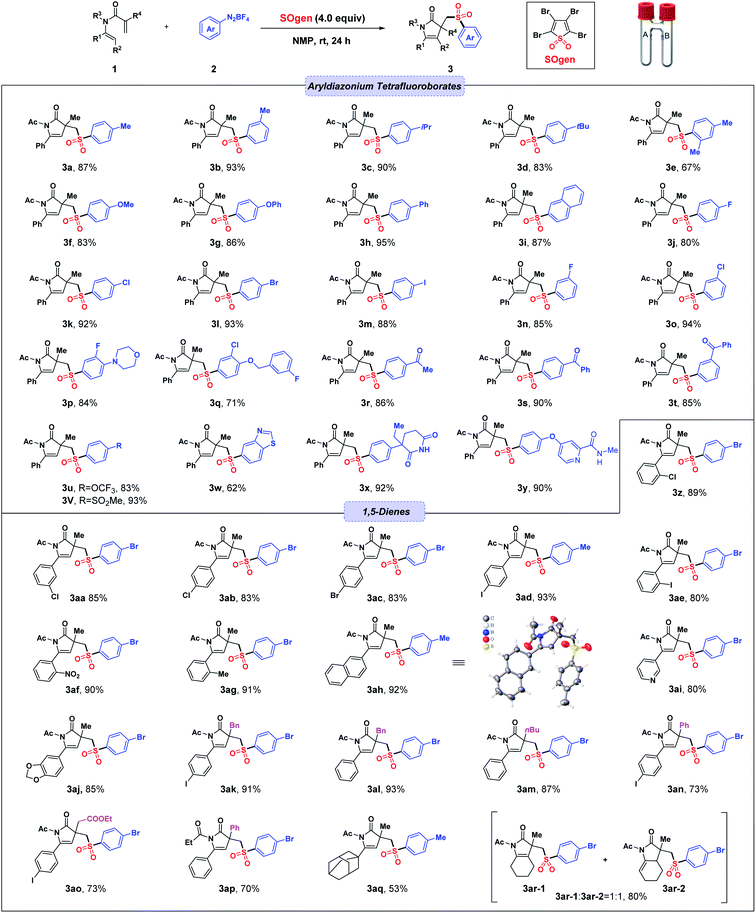 |
| Scheme 1 Substrate scopea. aReaction conditions: chamber A, SOgen (0.80 mmol), 1-methyl-4-vinylbenzene (0.81 mmol), tetradecane (1.0 mL), at 100 °C for 10 min; chamber B, 1 (0.2 mmol, 1.0 equiv.), 2 (0.44 mmol, 2.2 equiv.), NMP (1.0 mL), at rt for 24 h under argon atmosphere. All yields are isolated yields. | |
Next, the substrate scope of 1,5-dienes was investigated. The results showed that halogen groups (–Cl, –Br and –I) on 1,5-dienes had little effect on the reaction, and the corresponding products 3z–3ae were formed in 80–93% yields. Notably, 1,5-diene with a strongly electron-withdrawing group (–NO2) could deliver desired products 3af in an excellent yield (90%). Meanwhile, the one with an electron-donating group (–Me) could also give products 3ag in a similar yield (91%). Naphthalene ring was well tolerated, achieving 3ah in 92% yield and the configuration of compound 3ah was confirmed by X-ray crystallography. Pyridine moiety was also adapted to the reaction conditions and generated 3ai in 80% yield. In addition, it was found that benzodioxole moiety (3aj) could be well tolerated under the standard conditions. When R4 group was replaced by other substituents, such as benzyl, phenyl, n-butyl and –CH2COOEt, desired products (3ak–3ap) could still be made in good-to-excellent yields. When R1 group was alkyl, the sulfonylation reaction could still proceed, demonstrated by two successful examples (3aq and 3ar). It was worth noting that compound 3ar contained two isomers (3ar-1
:
3ar-1 = 1
:
1).
The practicality of this methodology was successfully illustrated by the production of 3a with 90% yield in a scale-up reaction (Scheme 2(1)). To further demonstrate the synthetic utility of this method, 3a was then applied in subsequent transformations. In the presence of diethylamine, the acetyl group on the nitrogen atom could be easily removed in a quantitative yield (Scheme 2(2)). Then, different substituents could be introduced on the N atom. For example, N–H could be transferred to N–Me (5) in 90% yield in a mixture of sodium hydride and iodomethane (Scheme 2(3)). In addition, the amide moiety of 3a could be reduced to hydroxy amine (6) via ring-opening by NaBH4 in excellent yield (Scheme 2(4)).
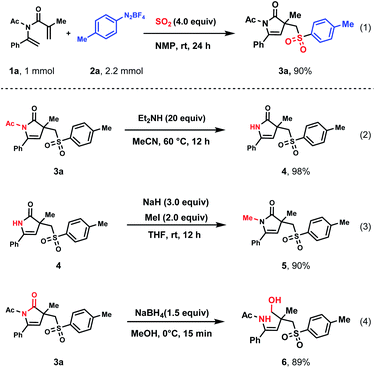 |
| Scheme 2 Scale-up reaction and synthetic applications. | |
In order to understand the mechanism of this reaction, three control experiments with radical scavengers were carried out. Firstly, in the presence of radical scavenger (TEMPO), the desired product (3a) was totally quenched and TEMPO adduct 7 was identified by LC-MS (Scheme 3a(1)). Secondly, when 1,1-diphenylethylene or BHT was added, the reaction showed similar result and corresponding aryl radicals adduct (8 or 10) sulfonyl radicals adduct (9 or 11) were identified, respectively (Scheme 3(2) and (3)). These results indicated that this transformation might proceed through a radical pathway.
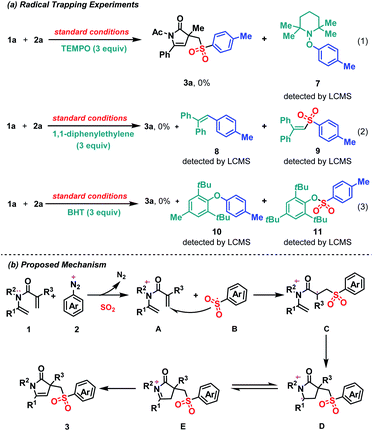 |
| Scheme 3 (a) Control experiments and (b) proposed mechanism. | |
Base on the control experiments and literature,20c a plausible reaction mechanism is proposed herein (Scheme 3b). One of lone-pair electrons on the N atom of 1,5-diene is transferred to aryldiazonium tetrafluoroborate, which leads to the formation of nitrogen radical cation species (A) and aryl radical. Then aryl radical is trapped by sulfur dioxide and gives aryl sulfone radical (B). Sulfonyl radical B selectively adds to the double bond of 1,5-diene and produces alkyl radical species C. Subsequently, intramolecular 5-endo-trig cyclization produces intermediate D, which has an equilibrium with E. Finally, the desired product (3) is produced via tautomerization from E.
Conclusions
In conclusion, a metal- and base-free sulfonylative cyclization of 1,5-dienes with aryldiazonium salts via the insertion of SO2 (from SOgen) has been developed. This method can work under mild conditions and provide the desired products in good yields with excellent regioselectivity. In addition, this approach greatly expands the substrates scope compared with previous reported work. Preliminary mechanism studies indicate that this 5-endo-trig sulfonylative cyclization may proceed in a radical pathway.
Conflicts of interest
There are no conflicts to declare.
Acknowledgements
This work is supported by National Natural Science Foundation of China (21901168), “1000-Youth Talents Plan”, Sichuan Science and Technology Program (2021YJ0395) and “1.3.5 Project for Disciplines of Excellence, West China Hospital, Sichuan University”. We also thank Jing Li from the Comprehensive Training Platform of Specialized Laboratory in College of Chemistry at Sichuan University for sample analysis.
Notes and references
- For selected examples, see:
(a) N. Dev, A. K. Das, M. A. Hossain and S. M. M. Rahman, Chemical Compositions of Different Extracts of Ocimum Basilicum Leaves, J. Sci. Res., 2011, 3, 197–206 CrossRef;
(b) S. Chu, S. Liu, W. Duan, Y. Cheng, X. Jiang, C. Zhu, K. Tang, R. Wang, L. Xu, X. Wang, X. Yu, K. Wu, Y. Wang, M. Wang, H. Huang and J. Zhang, The Anti-Dementia Drug Candidate, (−)-Clausenamide, Improves Memory Impairment Through Its Multi-target Effect, Pharmacol. Ther., 2016, 162, 179–187 CrossRef CAS PubMed.
- For selected examples, see:
(a) R. Kontnik and J. Clardy, Codinaeopsin, an Antimalarial Fungal Polyketide, Org. Lett., 2008, 10, 4149–4151 CrossRef CAS PubMed;
(b) S. Castellano, H. D. G. S. Fiji, S. S. Kinderman, M. Watanabe, P. deLeon, F. Tamanoi and O. Kwon, Small-Molecule Inhibitors of Protein Geranylgeranyltransferase Type I, J. Am. Chem. Soc., 2007, 129, 5843–5845 CrossRef CAS PubMed.
- For selected examples, see:
(a) S. S. Ariya, J. Baby, V. Santhanam and H. Waheeta, Computational Analysis of Compounds form Ocimum Sanctum for Anticancer Activity Against Oral Squamous Cell Carcinoma, Asian J. Pharm. Clin. Res., 2019, 12, 168–172 CrossRef CAS;
(b) V. R. Macherla, S. S. Mitchell, R. R. Manam, K. A. Reed, T. H. Chao, B. Nicholson, G. Deyanat-Yazdi, B. Mai, P. R. Jensen, W. F. Fenical, S. T. C. Neuteboom, K. S. Lam, M. A. Palladino and B. C. M. Potts, Structure–Activity Relationship Studies of Salinosporamide A (NPI-0052), a Novel Marine Derived Proteasome Inhibitor, J. Med. Chem., 2005, 48, 3684–3687 CrossRef CAS PubMed.
- For selected examples, see:
(a) A. Guerrini, A. Tesei, C. Ferroni, G. Paganelli, A. Zamagni, S. Carloni, M. D. Donato, G. Castoria, C. Leonetti, M. Porru, M. De Cesare, N. Zaffaroni, G. Luca Beretta, A. Del Rio and G. Varchi, A New Avenue toward Androgen Receptor Pan-antagonists: C2 Sterically Hindered Substitution of Hydroxy-propanamides, J. Med. Chem., 2014, 57, 7263–7279 CrossRef CAS PubMed;
(b) K. G. Liu, A. J. Robichaud, R. C. Bernotas, Y. Yan, J. R. Lo, M. Y. Zhang, Z. A. Hughes, C. Huselton, G. M. Zhang, J. Y. Zhang, D. M. Kowal, D. L. Smith, L. E. Schechter and T. A. Comery, 5-Piperazinyl-3-Sulfonylindazoles as Potent and Selective 5-Hydroxytryptamine-6 Antagonists, J. Med. Chem., 2010, 53, 7639–7646 CrossRef CAS PubMed.
-
(a) A. Ulman, C. S. Willand, W. Kohler, D. R. Robello, D. J. Williams and L. Handley, New Sulfonyl-containing Materials for Nonlinear Optics: Semiempirical Calculations, Synthesis, and Properties, J. Am. Chem. Soc., 1990, 112, 7083–7090 CrossRef CAS;
(b) Y. Huang, L. j. Huo, S. Q. Zhang, X. Guo, C. C. Han, Y. F. Li and J. H. Hou, Sulfonyl: a New Application of Electron-Withdrawing Substituent in Highly Efficient Photovoltaic Polymer, Chem. Commun., 2011, 47, 8904–906 RSC.
- For selected examples, see:
(a) W. Grell, R. Hurnaus, G. Griss, R. Sauter, E. Rupprecht, M. Mark, P. Luger, H. Nar, H. Wittneben and P. Müller, Repaglinide and Related Hypoglycemic Benzoic Acid Derivatives, J. Med. Chem., 1998, 41, 5219–5246 CrossRef PubMed;
(b) T. Uchida, K. Hayashi, H. Kido and M. Watanabe, Diuretic Action of the Novel Loop Diuretic Torasemide in the Presence of Angiotensin II or Endothelin-1 in Anaesthetized Dogs, J. Pharm. Pharmacol., 2011, 44, 39–43 CrossRef PubMed.
- For selected examples, see:
(a) N. Fuentes, W. Kong, L. Fernandez-Sanchez, E. Merino and C. Nevado, Cyclization Cascades via N-Amidyl Radicals toward Highly Functionalized Heterocyclic Scaffolds, J. Am. Chem. Soc., 2015, 137, 964–973 CrossRef CAS PubMed;
(b) J. Xuan and A. Studer, Radical Cascade Cyclization of 1,n-enynes and Diynes for the Synthesis of Carbocycles and Heterocycles, Chem. Soc. Rev., 2017, 46, 4329–4346 RSC;
(c) H. M. Huang, J. J. W. McDouall and D. J. Procter, SmI2-catalysed cyclization cascades by radical relay, Nat. Catal., 2019, 2, 211–218 CrossRef CAS;
(d) C. Z. Zhang, D. S. Wang, W. C. C. Lee, A. M. McKillop and X. P. Zhang, Controlling Enantioselectivity and Diastereoselectivity in Radical Cascade Cyclization for Construction of Bicyclic Structures, J. Am. Chem. Soc., 2021, 143, 11130–11140 CrossRef CAS PubMed;
(e) Y. Liu, J. L. Zhang, R. J. Song, P. C. Qian and J. H. Li, Cascade Nitration/Cyclization of 1,7-Enynes with tBuONO and H2O: One-Pot Self-Assembly of Pyrrolo[4,3,2-de]quinolinones, Angew. Chem., Int. Ed., 2014, 53, 9017–9022 CrossRef CAS PubMed.
- For selected examples, see:
(a) W. T. Wei, M. B. Zhou, J. H. Fan, W. Liu, R. J. Song, Y. Liu, M. Hu, P. Xie and J. H. Li, Synthesis of Oxindoles by Iron-Catalyzed Oxidative 1,2-Alkylarylation of Activated Alkenes with an Aryl C(sp2)–H Bond and a C(sp3)–H Bond Adjacent to a Heteroatom, Angew. Chem., Int. Ed., 2013, 52, 1–5 CrossRef;
(b) N. Fuentes, W. Kong, L. Fernandez-Sanchez, E. Merino and C. Nevado, Cyclization Cascades via N-Amidyl Radicals toward Highly Functionalized Heterocyclic Scaffolds, J. Am. Chem. Soc., 2015, 137, 964–973 CrossRef CAS PubMed;
(c) M. Hu, R. J. Song and J. H. Li, Metal-Free Radical 5-exo-dig Cyclizations of Phenol-Linked 1,6-Enynes for the Synthesis of Carbonylated Benzofurans, Angew. Chem., Int. Ed., 2014, 53, 608–613 Search PubMed;
(d) Z. Z. Chen, S. Liu, W. J. Hao, G. Xu, S. Wu, J. N. Miao, B. Jiang, S. L. Wang, S. J. Tu and G. Li, Catalytic Arylsulfonyl Radical-Triggered 1,5-Enyne-Bicyclizations and Hydrosulfonylation of α,β-Conjugates, Chem. Sci., 2015, 6, 6654–6658 RSC;
(e) B. Huang, L. Zeng, Y. Shen and S. Cui, Nickel-Catalyzed Acetamidation and Lactamization of Arylboronic Acids, Chem. Commun., 2017, 53, 11996–11999 RSC;
(f) C. Z. Zhang, D. S. Wang, W. C. Cindy Lee, A. M. McKillop and X. P. Zhang, Controlling Enantioselectivity and Diastereoselectivity in Radical Cascade Cyclization for Construction of Bicyclic Structures, J. Am. Chem. Soc., 2021, 143, 11130–11140 CrossRef CAS PubMed;
(g) D. M. Zeng, M. Wang, W. P. Deng and X. F. Jiang, The Same Oxygenation-State Introduction of Hypervalent Sulfur under Transition-metal-free Conditions, Org. Chem. Front., 2020, 7, 3956–3966 RSC.
- H. J. Chen, M. L. Liu, G. Y. S. Qiu and J. Wu, A Three-Component Reaction of Aryldiazonium Tetrafluoroborates, Sulfur Dioxide, and 1-(Prop-2-yn-1-yl)Indoles under Catalyst-Free Conditions, Adv. Synth. Catal., 2019, 361, 146–150 CrossRef CAS.
- T. Liu, D. Q. Zheng and J. Wu, Synthesis of 3-((Arylsulfonyl)methyl)Indolin-2-Ones via Insertion of Sulfur Dioxide using Anilines as the Aryl Source, Org. Chem. Front., 2017, 4, 1079–1083 RSC.
- L. Zheng, Z. Z. Zhou, Y. T. He, L. H. Li, J. W. Ma, Y. F. Qiu, P. X. Zhou, X. Y. Liu, P. F. Xu and Y. M. Liang, Iodine-Promoted Radical Cyclization in Water: A Selective Reaction of 1,6-Enynes with Sulfonyl Hydrazides, J. Org. Chem., 2016, 81, 66–76 CrossRef CAS PubMed.
- X. F. Wang, Y. W. Li, G. Y. S. Qiu and J. Wu, Synthesis of 6-(Sulfonylmethyl)Phenanthridines through a Reaction of Aryldiazonium Tetrafluoroborates, Sulfur Dioxide, and Vinyl Azides, Org. Chem. Front., 2018, 5, 2555–2559 RSC.
- J. Xu, X. Yu, J. Yan and Q. Song, Synthesis of 3-(Arylsulfonyl)benzothiophenes and Benzoselenophenes via TBHP-Initiated Radical Cyclization of 2-Alkynylthioanisoles or -selenoanisoles with Sulfinic Acid, Org. Lett., 2017, 19, 6292–6295 CrossRef CAS PubMed.
- Z. C. Chen, H. Zhang, S. F. Zhou and X. L. Cui, Photoredox-Catalyzed Synthesis of Sulfonated Oxazolines from N-allylamides through the Insertion of Sulfur Dioxide, Org. Chem. Front., 2022, 9, 364–369 RSC.
- W. Dong, L. Qi, J. Y. Song, J. M. Chen, J. X. Guo, S. Shen, L. J. Li, W. Li and L. J. Wang, Direct Synthesis of Sulfonylated Spiro[indole-3,3’-pyrrolidines] by Silver-Mediated Sulfonylation of Acrylamides Coupled with Indole Dearomatization, Org. Lett., 2020, 22, 1830–1835 CrossRef CAS PubMed.
-
(a) Z. C. Chen, H. Zhang, S. F. Zhou and X. L. Cui, K2S2O8-Initiated Cascade Cyclization of 2-Alkynylnitriles with Sodium Sulfinates: Access to Fused Cyclopenta[gh]phenanthridines, Chin. J. Org. Chem., 2020, 40, 3866–3872 CrossRef CAS;
(b) Z. Cao, Q. Zhu, Y. W. Lin and W. M. He, The Concept of Dual Roles Design in Clean Organic Preparation, Chin. Chem. Lett., 2019, 30, 2132–2144 CrossRef CAS;
(c) K. D. Zhou, J. Zhang, G. Y. S. Qiu and J. Wu, Copper(II)-Catalyzed Reaction of 2,3-Allenoic Acids, Sulfur Dioxide, and Aryldiazonium Tetrafluoroborates: Route to 4-Sulfonylated Furan-2(5H)-ones, Org. Lett., 2019, 21, 275–278 CrossRef CAS PubMed;
(d) F. Herrera, P. Esteban, A. Luna and P. Almendros, Metal-Catalyzed Reactivity Reversal in the Sulfonylation Reaction of α-Allenols: Controlled Synthesis of 4-(Arylsulfonyl)-2,5-Dihydrofurans, Adv. Synth. Catal., 2021, 363, 3952–3956 CrossRef CAS.
-
(a) R. Ding, M. H. Mao, W. Z. Jia, J. M. Fu, L. Liu, Y. Y. Mao, Y. Guo and P. L. Wang, Synthesis of Sulfonylated Pyrrolines and Pyrrolinones via Ag-Mediated Radical Cyclization of Olefinic Enamides with Sodium Sulfinates, Asian J. Org. Chem., 2021, 10, 366–370 CrossRef CAS;
(b) R. Ding, Y. L. Liu, H. Hao, C. Y. Chen, L. Liu, N. S. Chen, Y. Guo and P. L. Wang, Regioselective, Copper(I)-Catalyzed, Tandem Sulfonylation-Cyclization of 1,5-Dienes with Sulfonyl Chlorides, Org. Chem. Front., 2021, 8, 3123–3127 RSC.
- For selected reviews, see:
(a) E. J. Emmett and M. C. Willis, The Development and Application of Sulfur Dioxide Surrogates in Synthetic Organic Chemistry, Asian J. Org. Chem., 2015, 4, 602–611 CrossRef CAS;
(b) G. S. Qiu, K. D. Zhou, L. Gao and J. Wu, Insertion of Sulfur Dioxide Via a Radical Process: An Efficient Route to Sulfonyl Compounds, Org. Chem. Front., 2018, 5, 691–705 RSC;
(c) K. Hofman, N. W. Liu and G. Manolikakes, Radicals and Sulfur Dioxide: A Versatile Combination for the Construction of Sulfonyl-Containing Molecules, Chem.–Eur. J., 2018, 24, 11852–11863 CrossRef CAS PubMed;
(d) D. Joseph, M. A. Idris, J. Chen and S. Lee, Recent Advances in the Catalytic Synthesis of Arylsulfonyl Compounds, ACS Catal., 2021, 11, 4169–4204 CrossRef CAS.
- For selected examples, see:
(a) D. Q. Zheng, Y. Y. An, Z. H. Li and J. Wu, Metal-Free Aminosulfonylation of Aryldiazonium Tetrafluoroborates with DABCO·(SO2)2 and Hydrazines, Angew. Chem., Int. Ed., 2014, 53, 2451–2454 CrossRef CAS PubMed;
(b) B. Nguyen, E. J. Emmett and M. C. Willis, Palladium-Catalyzed Aminosulfonylation of Aryl Halides, J. Am. Chem. Soc., 2010, 132, 16372–16373 CrossRef CAS PubMed;
(c) Y. Meng, M. Wang and X. Jiang, Multicomponent Reductive Cross-Coupling of an Inorganic Sulfur Dioxide Surrogate: Straightforward Construction of Diversely Functionalized Sulfones, Angew. Chem., Int. Ed., 2020, 59, 1346–1353 CrossRef CAS PubMed;
(d) W. Li, H. Li, P. Langer, M. Beller and X. F. Wu, Palladium-Catalyzed Aminosulfonylation of Aryl Iodides by using Na2SO3 as the SO2 Source, Eur. J. Org. Chem., 2014, 3101–3103 CrossRef;
(e) H. B. Zhu, Y. J. Shen, D. H. Wen, Z. G. Le and T. Tu, Selective Synthesis of ortho-Substituted Diarylsulfones by Using NHC-Au Catalysts under Mild Conditions, Org. Lett., 2019, 21, 974–979 CrossRef CAS PubMed;
(f) Y. Wang, L. L. Deng, J. Zhou, X. C. Wang, H. B. Mei, J. L. Han and Y. Pan, Synthesis of Chiral Sulfonyl Lactones via Copper-Catalyzed Asymmetric Radical Reaction of DABCO·(SO2), Adv. Synth. Catal., 2018, 360, 1060–1065 CrossRef CAS;
(g) A. Shavnya, K. D. Hesp, V. Mascitti and A. C. Smith, Palladium-Catalyzed Synthesis of (Hetero)Aryl Alkyl Sulfones from (Hetero)Aryl Boronic Acids, Unactivated Alkyl Halides, and Potassium Metabisulfite, Angew. Chem., Int. Ed., 2015, 54, 13571–13575 CrossRef CAS PubMed;
(h) N. von Wolff, J. Char, X. Frogneux and T. Cantat, Synthesis of Aromatic Sulfones from SO2 and Organosilanes Under Metal-free Conditions, Angew. Chem., Int. Ed., 2017, 56, 5616–5619 CrossRef CAS PubMed.
-
(a) X. W. Jia, S. Kramer, T. Skrydstrup and Z. Lian, Design and Applications of a SO2 Surrogate in Palladium-Catalyzed Direct Aminosulfonylation between Aryl Iodides and Amines, Angew. Chem., Int. Ed., 2021, 60, 7353–7359 CrossRef CAS PubMed;
(b) Y. Li, L. Shen, M. Zhou, B. J. Xiong, X. M. Zhang and Z. Lian, Copper-Catalyzed Chloro-Arylsulfonylation of Styrene Derivatives via the Insertion of Sulfur Dioxide, Org. Lett., 2021, 23, 5880–5884 CrossRef CAS PubMed;
(c) L. Chen, M. Zhou, L. Shen, X. C. He, X. Li, X. M. Zhang and Z. Lian, Metal- and Base-Free C(sp2)–H Arylsulfonylation of Enamides for Synthesis of (E)-β-Amidovinyl Sulfones via the Insertion of Sulfur Dioxide, Org. Lett., 2021, 23, 4991–4996 CrossRef CAS PubMed;
(d) G. Chen, J. Xu, B. J. Xiong, H. Z. Song, X. M. Zhang, X. L. Ma and Z. Lian, Copper-Catalyzed Trifluoromethylthio-arylsulfonylation of Styrene Derivatives via the Insertion of Sulfur Dioxide, Org. Lett., 2022, 24(5), 1207–1212 CrossRef CAS PubMed;
(e) X. W. Jia, C. X. Huang, X. M. Zhang and Z. Lian, Metal-free Sulfonylative Annulations of Alkyl Diiodides with Sulfur Dioxide: Synthesis of Cyclic Aliphatic Sulfones, Org. Chem. Front., 2021, 8, 5310–5315 RSC.
Footnotes |
† Electronic supplementary information (ESI) available: Experimental procedures, characterization data of products, and NMR spectra, and X-ray crystallographic data. CCDC 2131001 (3ah). For ESI and crystallographic data in CIF or other electronic format see https://doi.org/10.1039/d2ra03034a |
‡ These authors contributed equally. |
|
This journal is © The Royal Society of Chemistry 2022 |