DOI:
10.1039/D2RA04603B
(Paper)
RSC Adv., 2022,
12, 26727-26732
Stereoselective synthesis of C3-tetrasubstituted oxindoles via copper catalyzed asymmetric propargylation†
Received
24th July 2022
, Accepted 10th September 2022
First published on 21st September 2022
Abstract
Herein, a copper catalyzed asymmetric propargylation of 2-oxindole-3-carboxylate esters with terminal propargylic esters is described. This strategy successfully provides a direct approach to constructing a broad range of chiral C3-tetrasubstituted oxindoles with contiguous tertiary and quaternary carbon stereocenters in high yields and excellent enantioselectivities (16 examples, up to 99% yield and 98% ee). Moreover, the diastereoisomers of the two newly formed stereocenters can be separated by silica gel chromatography, thereby providing a valuable stereoselective access to all four possible stereoisomers of C3-tetrasubstituted oxindoles.
Introduction
As privileged scaffolds in a large family of natural alkaloid compounds, pharmaceuticals and bioactive products, oxindoles bearing a tetrasubstituted carbon stereocenter at the C3 position widely exist in a range of structural frameworks and architectures owing to their potential biological and pharmacological activities (Fig. 1a).1 As a result, tremendous efforts have been devoted to achieving these motifs.2 With the development of asymmetric organocatalysis and transition metal catalysis, catalytic asymmetric protocols to directly construct C3-tetrasubstituted oxindoles with an all-carbon quaternary stereocenter using 3-substituted oxindoles as nucleophiles have received extensive attention in the past decades (Fig. 1b).3 Despite these impressive numerous works, the efficient synthesis of chiral C3-tetrasubstituted oxindoles bearing contiguous stereocenters remains one of the most difficult issues in asymmetric catalysis.
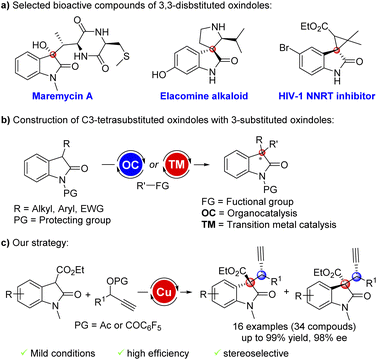 |
| Fig. 1 Representative examples of C3-tetrasubstituted oxindoles and our strategy. | |
Recently, asymmetric propargylation reactions of readily available racemic terminal propargylic esters catalyzed by non-precious chiral copper complexes have emerged as a promising, fruitful and attractive method to construct useful chiral propargyl compounds.4 However, although a variety of nucleophiles can participate in copper catalyzed propargylation substitution,5 the efficient construction of contiguous tertiary and all-carbon quaternary stereocenters is still a difficult problem for this methodology.6 In 2020, Hu and co-workers reported a copper catalyzed asymmetric propargylation reaction of 3-aryl substituted oxindoles affording 3,3-disubstituted oxindoles in highly diastereo- and enantioselective results.7 Recently, the stereodivergent synthesis of natural product frameworks via asymmetric catalytic transformations using simple starting materials has aroused widely concern and development.8 To the best of our knowledge, the stereoselective synthesis of chiral C3-tetrasubstituted oxindoles with contiguous tertiary and all-carbon quaternary stereocenters via copper catalyzed asymmetric propargylation reaction is still highly desirable. Herein, we report a copper catalyzed asymmetric propargylation substitution of 2-oxindole-3-carboxylate esters9 with terminal propargylic esters providing a concise strategy for the stereoselective synthesis of chiral C3-tetrasubstituted oxindoles bearing contiguous tertiary and quaternary carbon stereocenters.
Results and discussion
To investigate our study, we chose 2-oxindole-3-carboxylate esters 1a and terminal propargylic acetate 2a as model substrates in the presence of 10 mol% of copper catalyst in methanol (Table 1). A series of chiral Pybox ligands were first screened, we can successfully get the desired chiral propargylation products 3a in good yields and moderate enantioselectivities albeit in low diastereoselectivity, in which L(+)-leucinol-derived chiral ligand L6 is the best choice (Table 1, entries 1–7). Performing the reaction at lower temperature resulted in increased yield and enantioselectivity, but not diastereoselectivity (Table 1, entry 8). However, changing the base could not improve the reaction results (Table 1, entries 9 and 10). Ultimately, further lowering the reaction temperature to −15 °C gave excellent yield and enantioselectivity, but the diastereoselectivity remained unimproved (Table 1, entry 11). Although the diastereoselectivity was not so satisfactory, the two diastereomers (R,R)-3a and (S,R)-3a can be separated by silica gel chromatography.
Table 1 Optimization of the reaction conditionsa
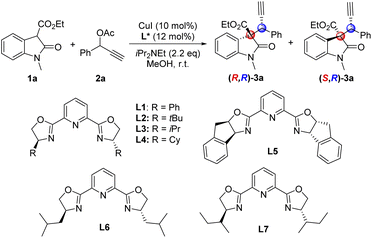
|
Entry |
Ligand |
Time (h) |
Yieldb (%) |
eec (%) |
dr [(R,R)/(S,R)]c (%) |
Unless noted, reactions were performed with 1a (0.20 mmol), 2a (0.3 mmol), CuI (0.02 mmol), L (0.022 mmol) and iPr2NEt (0.44 mmol) in anhydrous MeOH (3 mL) at r.t. for 1 h. Determined by 1H NMR of the reaction mixture containing 1,3,5-trimethoxybenzene as an internal standard. ee and dr [(R,R)/(S,R)], determined by chiral HPLC. Reaction performed at 0 °C. Using Et3N instead of iPr2NEt. Using K2CO3 instead of iPr2NEt. Reaction performed at −15 °C. Isolated yield in parentheses. |
1 |
L1 |
1 |
93 |
44/60 |
1 : 1.7 |
2 |
L2 |
1 |
66 |
27/4 |
1.1 : 1 |
3 |
L3 |
1 |
85 |
59/48 |
1.1 : 1 |
4 |
L4 |
1 |
73 |
27/4 |
1 : 1 |
5 |
L5 |
1 |
60 |
59/48 |
1.1 : 1 |
6 |
L6 |
1 |
90 |
71/79 |
1 : 1.2 |
7 |
L7 |
1 |
80 |
62/54 |
1.5 : 1 |
8d |
L6 |
5 |
92 |
90/94 |
1 : 1.5 |
9d,e |
L6 |
5 |
95 |
86/92 |
1 : 1.3 |
10d,f |
L6 |
5 |
40 |
54/75 |
1 : 1.5 |
11g |
L6 |
20 |
95(92)h |
91/95 |
1 : 1.6 |
With the optimized reaction conditions in hand, a series of 2-oxindole-3-carboxylate esters 1 were first evaluated, providing the corresponding products in moderate to excellent yields and enantioselectivities, but with low diastereoselectivities (Scheme 1: 3a–3h, up to 95% yield, 95% ee and 1
:
1.6 dr). As shown in Scheme 1, the reaction has a broad substrate scope and high functional group tolerance. Substituted groups at the 5-position of oxindole such as 5-Br, 5-Cl, 5-Me and 5-MeO reacted smoothly achieving the desired chiral products in high yields and enantioselectivities (3a–3e). The results revealed that the electron-withdrawing substituents performed better than the electron-donating substituents. At the same time, the substituent at the 6-position resulted in the same reactivity (3f). To further verify the compatibility of this transformation, the protecting group of oxindole was investigated. Ethyl- and allyl-protected 2-oxindole-3-carboxylate esters also proved to be suitable for this reaction (3g–3h), but one of the isomers of ethyl-protected chiral product showed lower enantioselectivity ((R,R)-3g: 67% ee). Next, the substrate scope with respect to terminal propargylic acetates 2 were investigated (Scheme 1: 3i–3p, up to 99% yield, 98% ee and 1
:
1.4 dr). The substituted groups on the phenyl ring of terminal propargylic acetates were evaluated affording the desired corresponding chiral C3-tetrasubstituted oxindoles in moderate to good yields and enantioselectivities (3i–3k). In addition, naphthyl and 2-bromo piperonyl also performed well with good results (3l–3m). When adjusting the protecting group of terminal propargylic esters, aliphatic substrates were compatible with the reaction, giving the corresponding chiral products in good to excellent yields and enantioselectivities (3n–3o). However, the cyclohexyl substituted substrate only gave moderate results, suggesting a sensitive effect of steric hindrance for this transformation (3p). Finally, the absolute configuration of the two isomers can be determined by X-ray structure analysis of (S,R)-3i (CCDC 2181912) and (R,S)-3m (CCDC 2181911).†
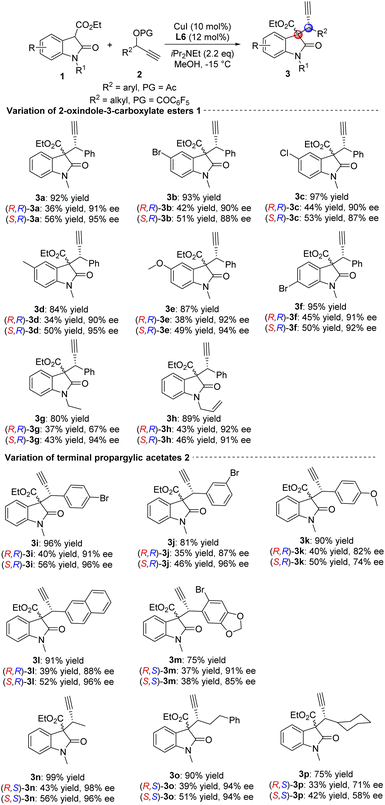 |
| Scheme 1 Substrate scope.a Unless noted, reactions were performed with 1 (0.20 mmol), 2 (0.3 mmol), CuI (0.02 mmol), L6 (0.022 mmol) and iPr2NEt (0.44 mmol) in anhydrous MeOH (3 mL) at −15 °C for 20 h. Isolated yields based on 1, and ee values were determined by chiral HPLC analysis. | |
To further demonstrated the utility of this transformation, synthetic transformations were carried out (Fig. 2). Under the standard reaction conditions, using L6 and its enantiomer ent-L6 as chiral ligands and 1f and 2a as substrates, we were able to obtain all four stereoisomers of chiral product 3f in moderate yields and excellent enantioselectivities (Fig. 2a). This offered an efficient method for the stereoselective synthesis of chiral C3-tetrasubstituted oxindoles bearing contiguous tertiary and quaternary carbon stereocenters. The click reaction of (S,R)-3f with anti-HIV drug Zidovudine resulted in chiral triazole product 4 in 90% yield and excellent diastereoselectivity (Fig. 2b).
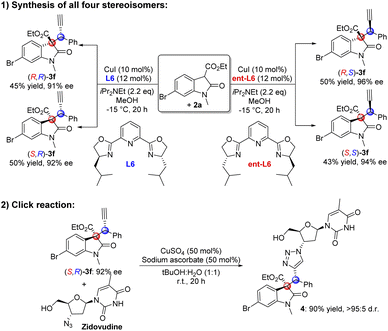 |
| Fig. 2 Synthetic transformations. | |
In order to get the insight of this reaction, we performed a set of experiments with ee-varied ligand L6 under the model reaction. As depicted in Fig. 3, a linear relationship between ee of ligand L6 and product 3a was observed, which indicated a mono-copper catalytic process.10 On the basis of prior studies6a,7a,9 and our observations, the proposed mechanism and stereocontrol models are depicted in Fig. 4. Firstly, the in situ formed chiral copper complex CuL* by CuI and L6 reacted with terminal propargylic acetate 2 with the assistance of iPr2NEt to generate copper allenylidene intermediate A or its resonance intermediate A′. In the presence of base, substrate 1 is transformed to its enolate 1′, followed by nucleophilic attack on the cationic intermediate A or A′ giving the intermediate B. Finally, the protolysis of intermediate B generates the desired chiral 3a and release the chiral copper complex. Based on the absolute configurations of the two isomers, rationalized stereocontrol models are proposed in Fig. 4. Owing to the steric hindrance of chiral ligand L6, the enolate 1′ favors nucleophilic attack from the top of the face of copper allenylidene intermediate, thus leading to good enantioselectivities. However, the two sides of the enolate 1′ (Si face and Re face) can attack on the face of copper allenylidene intermediate A, respectively, resulting in poor diastereoselectivities (Fig. 4, path a and b).
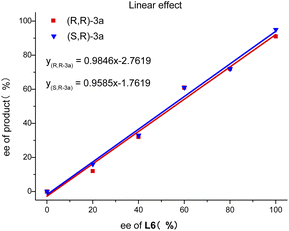 |
| Fig. 3 The linear effect. | |
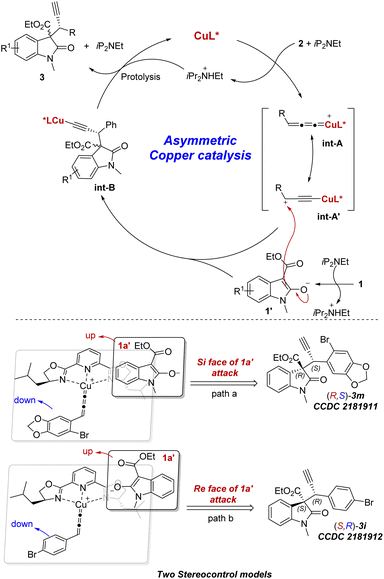 |
| Fig. 4 The proposed mechanism and stereocontrol models. | |
Conclusions
In conclusion, we have reported an efficient copper catalyzed asymmetric propargylation substitution of 2-oxindole-3-carboxylate esters with terminal propargylic acetates. This protocol provides a concise and facile synthetic approach to construct a broad range of chiral C3-tetrasubstituted oxindoles bearing contiguous tertiary and quaternary carbon stereocenters. Furthermore, both isomers can be separated by silica gel chromatography. So, this method provides a valuable access for the stereoselective synthesis of four possible C3-tetrasubstituted oxindole stereoisomers. We hope this transformation would inspire the development of new asymmetric and highly practical synthetic methods for the synthesis of chiral C3-tetrasubstituted oxindole derivatives.
Author contributions
Jiaomei Wang and Yu Zhao contributed equally to this work.
Conflicts of interest
There are no conflicts to declare.
Acknowledgements
We are grateful for financial support by the National Natural Science Foundation of China (Grants No. 22101102, 22161047), Scientific Research Foundation of Xuzhou Institute of Technology (XKY2019210), Natural Science Foundation of Jiangsu Province (BK20210915).
Notes and references
- For selected examples, see:
(a) T. Matsuura, L. E. Overman and D. J. Poon, J. Am. Chem. Soc., 1998, 120, 6500–6503 CrossRef CAS;
(b) S. Edmondson, S. J. Danishefsky, L. Sepp-Lorenzino and N. Rosen, J. Am. Chem. Soc., 1999, 121, 2147–2155 CrossRef CAS;
(c) L. E. Overman and M. D. Rosen, Angew. Chem., Int. Ed., 2000, 39, 4596–4599 CrossRef CAS;
(d) P. R. Sebahar and R. M. Williams, J. Am. Chem. Soc., 2000, 122, 5666–5667 CrossRef CAS;
(e) A. Lerchner and E. M. Carreira, J. Am. Chem. Soc., 2002, 124, 14826–14827 CrossRef CAS PubMed;
(f) C. Marti and E. M. Carreira, Eur. J. Org. Chem., 2003, 2003, 2209–2219 CrossRef;
(g) H. Lin and S. J. Danishefsky, Angew. Chem., Int. Ed., 2003, 42, 36–51 CrossRef CAS;
(h) J. F. Austin, S.-G. Kim, C. J. Sinz, W.-J. Xiao and D. W. C. MacMillan, Proc. Natl. Acad. Sci. U. S. A., 2004, 101, 5482–5487 CrossRef CAS PubMed;
(i) A. Huang, J. J. Kodanko and L. E. Overman, J. Am. Chem. Soc., 2004, 126, 14043–14053 CrossRef CAS PubMed;
(j) F. Y. Miyake, K. Yakushijin and D. A. Horne, Angew. Chem., Int. Ed., 2004, 43, 5357–5360 CrossRef CAS;
(k) M. M.-C. Lo, C. S. Neumann, S. Nagayama, E. O. Perlstein and S. L. Schreiber, J. Am. Chem. Soc., 2004, 126, 16077–16086 CrossRef CAS PubMed;
(l) C. Marti and E. M. Carreira, J. Am. Chem. Soc., 2005, 127, 11505–11515 CrossRef CAS PubMed;
(m) J. A. May and B. Stoltz, Tetrahedron, 2006, 62, 5262–5271 CrossRef CAS;
(n) T. Kawasaki, M. Shinada, D. Kamimura, M. Ohzono and A. Ogawa, Chem. Commun., 2006, 420–422 RSC;
(o) B. M. Trost and Y. Zhang, J. Am. Chem. Soc., 2006, 128, 4590–4591 CrossRef CAS PubMed;
(p) B. M. Trost and D. T. Stiles, Org. Lett., 2007, 9, 2763–2766 CrossRef CAS PubMed;
(q) C. V. Galliford and K. A. Scheidt, Angew. Chem., Int. Ed., 2007, 46, 8748–8758 CrossRef CAS PubMed;
(r) J. T. Mohr, M. R. Krout and B. M. Stoltz, Nature, 2008, 455, 323–332 CrossRef CAS PubMed;
(s) P. Siengalewicz, T. Gaich and J. Mulzer, Angew. Chem., Int. Ed., 2008, 47, 8170–8176 CrossRef CAS PubMed.
- For selected reviews see:
(a) M. Bandini and A. Eichholzer, Angew. Chem., Int. Ed., 2009, 48, 9608–9644 CrossRef CAS;
(b) F. Zhou, Y.-L. Liu and J. Zhou, Adv. Synth. Catal., 2010, 352, 1381–1407 CrossRef CAS;
(c) Z.-Y. Cao, Y.-H. Wang, X.-P. Zeng and J. Zhou, Tetrahedron Lett., 2014, 55, 2571–2584 CrossRef CAS;
(d) R. Dalpozzo, Adv. Synth. Catal., 2017, 359, 1772–1810 CrossRef CAS;
(e) Z.-Y. Cao, F. Zhou and J. Zhou, Acc. Chem. Res., 2018, 51, 1443–1454 CrossRef CAS PubMed.
- For selected examples, see:
(a) T. B. K. Lee and G. S. K. Wong, J. Org. Chem., 1991, 56, 872–875 CrossRef CAS;
(b) B. M. Trost and M. U. Frederiksen, Angew. Chem., Int. Ed., 2005, 44, 308–310 CrossRef CAS;
(c) B. M. Trost and Y. Zhang, J. Am. Chem. Soc., 2006, 128, 4590–4591 CrossRef CAS;
(d) B. M. Trost and Y. Zhang, J. Am. Chem. Soc., 2007, 129, 14548–14549 CrossRef CAS PubMed;
(e) S. Ogawa, N. Shibata, J. Inagaki, S. Nakamura, T. Toru and M. Shiro, Angew. Chem., Int. Ed., 2007, 46, 8666–8669 CrossRef CAS;
(f) X. Tian, K. Jiang, J. Peng, W. Du and Y.-C. Chen, Org. Lett., 2008, 10, 3583–3586 CrossRef CAS;
(g) K. Jiang, J. Peng, H.-L. Cui and Y.-C. Chen, Chem. Commun., 2009, 3955–3957 RSC;
(h) A. M. Taylor, R. A. Altman and S. L. Buchwald, J. Am. Chem. Soc., 2009, 131, 9900–9901 CrossRef CAS PubMed;
(i) T. Bui, S. Syed and C. F. Barbas III, J. Am. Chem. Soc., 2009, 131, 8758–8759 CrossRef CAS PubMed;
(j) Y. Kato, M. Furutachi, Z. Chen, H. Mitsunuma, S. Matsunaga and M. Shibasaki, J. Am. Chem. Soc., 2009, 131, 9168–9169 CrossRef CAS PubMed;
(k) R. He, S. Shirakawa and K. Maruoka, J. Am. Chem. Soc., 2009, 131, 16620–16621 CrossRef CAS PubMed;
(l) L. Cheng, L. Liu, H. Jia, D. Wang and Y.-J. Chen, J. Org. Chem., 2009, 74, 4650–4653 CrossRef CAS PubMed;
(m) R. He, C. Ding and K. Maruoka, Angew. Chem., Int. Ed., 2009, 48, 4559–4561 CrossRef CAS;
(n) P. Galzerano, G. Bencivenni, F. Pesciaioli, A. Mazzanti, B. Giannichi, L. Sambri, G. Bartoli and P. Melchiorre, Chem.–Eur. J., 2009, 15, 7846–7849 CrossRef CAS PubMed;
(o) X. Li, Z.-G. Xi, S. Luo and J.-P. Cheng, Org. Biomol. Chem., 2010, 8, 77–82 RSC;
(p) K. Shen, X. Liu, K. Zheng, W. Li, X. Hu, L. Lin and X. Feng, Chem.–Eur. J., 2010, 16, 3736–3742 CrossRef CAS;
(q) B. M. Trost and Y. Zhang, Chem.–Eur. J., 2010, 16, 296–303 CrossRef CAS;
(r) M. Ding, F. Zhou, Y.-L. Liu, C.-H. Wang, X.-L. Zhao and J. Zhou, Chem. Sci., 2011, 2, 2035–2039 RSC;
(s) S.-W. Duan, J. An, J.-R. Chen and W.-J. Xiao, Org. Lett., 2011, 13, 2290–2293 CrossRef CAS PubMed;
(t) S.-W. Duan, Y.-Y. Liu, W. Ding, T.-R. Li, D.-Q. Shi, J.-R. Chen and W.-J. Xiao, Synthesis, 2013, 45, 1647–1653 CrossRef CAS;
(u) A. Boucherif, S.-W. Duan, Z.-G. Yuan, L.-Q. Lu and W.-J. Xiao, Adv. Synth. Catal., 2016, 358, 2594–2598 CrossRef CAS , and references therein.
- For selected reviews, see:
(a) Y. Miyake, S. Uemura and Y. Nishibayashi, ChemCatChem, 2009, 1, 342–356 CrossRef CAS;
(b) N. Ljungdahl and N. Kann, Angew. Chem., Int. Ed., 2009, 48, 642–644 CrossRef CAS;
(c) R. J. Detz, H. Hiemstra and J. H. Van Maarseveen, Eur. J. Org. Chem., 2009, 2009, 6263–6276 CrossRef;
(d) C.-H. Ding and X.-L. Hou, Chem. Rev., 2011, 111, 1914–1937 CrossRef CAS PubMed;
(e) Y. Nishibayashi, Synthesis, 2012, 2012, 489–503 CrossRef;
(f) X.-H. Hu, Z.-T. Liu, L. Shao and X.-P. Hu, Synthesis, 2015, 47, 913–923 CrossRef CAS;
(g) D.-Y. Zhang and X.-P. Hu, Tetrahedron Lett., 2015, 56, 283–295 CrossRef CAS;
(h) K. Sakata and Y. Nishibayashi, Catal. Sci. Technol., 2018, 8, 12–25 RSC;
(i) R. Roy and S. Saha, RSC Adv., 2018, 8, 31129–31193 RSC;
(j) S. W. Roh, K. Choi and C. Lee, Chem. Rev., 2019, 119, 4293–4356 CrossRef CAS;
(k) H. Tsuji and M. Kawatsura, Asian J. Org. Chem., 2020, 9, 1924–1941 CrossRef CAS;
(l) M.-M. Zhang, B.-L. Qu, B. Shi, W.-J. Xiao and L.-Q. Lu, Chem. Soc. Rev., 2022, 51, 4146–4174 RSC.
- For selected examples, see:
(a) R. J. Detz, M. M. E. H. Delville, H. Hiemstra and J. H. van Maarseveen, Angew. Chem., Int. Ed., 2008, 47, 3777–3780 CrossRef CAS PubMed;
(b) G. Hattori, H. Matsuzawa, Y. Miyake and Y. Nishibayashi, Angew. Chem., Int. Ed., 2008, 47, 3781–3783 CrossRef CAS PubMed;
(c) P. Fang and X.-L. Hou, Org. Lett., 2009, 11, 4612–4615 CrossRef CAS PubMed;
(d) G. Hattori, K. Sakata, H. Matsuzawa, Y. Tanabe, Y. Miyake and Y. Nishibayashi, J. Am. Chem. Soc., 2010, 132, 10592–10608 CrossRef CAS PubMed;
(e) A. Yoshida, G. Hattori, Y. Miyake and Y. Nishibayashi, Org. Lett., 2011, 13, 2460–2463 CrossRef CAS PubMed;
(f) C. Zhang, Y.-H. Wang, X.-H. Hu, Z. Zheng, J. Xu and X.-P. Hu, Adv. Synth. Catal., 2012, 354, 2854–2858 CrossRef CAS;
(g) C. Zhang, X.-H. Hu, Y.-H. Wang, Z. Zheng, J. Xu and X.-P. Hu, J. Am. Chem. Soc., 2012, 134, 9585–9588 CrossRef CAS;
(h) F.-Z. Han, F.-L. Zhu, Y.-H. Wang, Y. Zou, X.-H. Hu, S. Chen and X.-P. Hu, Org. Lett., 2014, 16, 588–591 CrossRef CAS PubMed;
(i) M. Shibata, K. Nakajima and Y. Nishibayashi, Chem. Commun., 2014, 50, 7874–7877 RSC;
(j) W. Shao, H. Li, C. Liu, C. J. Liu and S. L. You, Angew. Chem., Int. Ed., 2015, 54, 7684–7687 CrossRef CAS PubMed;
(k) D.-Y. Zhang, L. Shao, J. Xu and X.-P. Hu, ACS Catal., 2015, 5, 5026–5030 CrossRef CAS;
(l) K. Nakajima, M. Shibata and Y. Nishibayashi, J. Am. Chem. Soc., 2015, 137, 2472–2475 CrossRef CAS PubMed;
(m) K. Tsuchida, Y. Senda, K. Nakajima and Y. Nishibayashi, Angew. Chem., Int. Ed., 2016, 55, 9728–9732 CrossRef CAS PubMed;
(n) Z.-T. Liu, Y.-H. Wang, F.-L. Zhu and X.-P. Hu, Org. Lett., 2016, 18, 1190–1194 CrossRef CAS PubMed;
(o) L. Shao, D.-Y. Zhang, Y.-H. Wang and X.-P. Hu, Adv. Synth. Catal., 2016, 358, 2558–2563 CrossRef CAS;
(p) H. Xu, L. Laraia, L. Schneider, K. Louven, C. Strohmann, A. P. Antonchick and H. Waldmann, Angew. Chem., Int. Ed., 2017, 56, 11232–11236 CrossRef CAS;
(q) K. Zhang, L.-Q. Lu, S. Yao, J.-R. Chen, D.-Q. Shi and W.-J. Xiao, J. Am. Chem. Soc., 2017, 139, 12847–12854 CrossRef CAS PubMed;
(r) L.-J. Cheng, A. P. N. Brown and C. J. Cordier, Chem. Sci., 2017, 8, 4299–4305 RSC;
(s) J. E. Gómez, W. Guo, S. Gaspa and A. W. Kleij, Angew. Chem., Int. Ed., 2017, 56, 15035–15038 CrossRef PubMed;
(t) R.-Z. Li, H. Tang, L. Wan, X. Zhang, Z. Fu, J. Liu, S. Yang, D. Jia and D. Niu, Chem, 2017, 3, 834–845 CrossRef CAS;
(u) F. Jiang, X. Feng, R. Wang, X. Gao, H. Jia, Y. Xiao, C. Zhang and H. Guo, Org. Lett., 2018, 20, 5278–5281 CrossRef CAS PubMed;
(v) L. Li, Z.-T. Liu and X.-P. Hu, Chem. Commun., 2018, 54, 12033–12036 RSC;
(w) Y.-C. Zhang, B.-W. Zhang, R.-L. Geng and J. Song, Org. Lett., 2018, 20, 7907–7911 CrossRef CAS PubMed;
(x) X. Gao, R. Cheng, Y.-L. Xiao, X.-L. Wan and X. Zhang, Chem, 2019, 5, 2987–2999 CrossRef CAS;
(y) L. Yang, X. Pu, D. Niu, Z. Fu and X. Zhang, Org. Lett., 2019, 21, 8553–8557 CrossRef CAS;
(z) S. Liu, K. Nakajima and Y. Nishibayashi, RSC Adv., 2019, 9, 18918–18922 RSC , and references therein.
- For selected examples, see:
(a) L. Zhao, G. Huang, B. Guo, L. Xu, J. Chen, W. Cao, G. Zhao and X. Wu, Org. Lett., 2014, 16, 5584–5587 CrossRef CAS PubMed;
(b) D.-Y. Zhang, F.-L. Zhu, Y.-H. Wang, X.-H. Hu, S. Chen, C.-J. Hou and X.-P. Hu, Chem. Commun., 2014, 50, 14459–14462 RSC;
(c) C. Zhang, Y.-Z. Hui, D.-Y. Zhang and X.-P. Hu, RSC Adv., 2016, 6, 14763–14767 RSC;
(d) Q. Wang, T.-R. Li, L.-Q. Lu, M.-M. Li, K. Zhang and W.-J. Xiao, J. Am. Chem. Soc., 2016, 138, 8360–8363 CrossRef CAS;
(e) W. Shao and S.-L. You, Chem.–Eur. J., 2017, 23, 12489–12493 CrossRef CAS PubMed;
(f) T.-R. Li, L.-Q. Lu, Y.-N. Wang, B.-C. Wang and W.-J. Xiao, Org. Lett., 2017, 19, 4098–4101 CrossRef CAS;
(g) J. Song, Z.-J. Zhang and L.-Z. Gong, Angew. Chem., Int. Ed., 2017, 56, 5212–5216 CrossRef CAS;
(h) R.-Z. Li, H. Tang, K. R. Yang, L.-Q. Wan, X. Zhang, J. Liu, Z. Fu and D. Niu, Angew. Chem., Int. Ed., 2017, 56, 7213–7217 CrossRef CAS PubMed;
(i) X.-H. Lu, L. Ge, C. Cheng, J. Chen, W.-G. Cao and X.-Y. Wu, Chem.–Eur. J., 2017, 23, 7689–7693 CrossRef CAS PubMed;
(j) H. Chen, X.-H. Lu, X.-J. Xia, Q.-Q. Zhu, Y.-H. Song, J. Chen, W.-G. Cao and X.-Y. Wu, Org. Lett., 2018, 20, 1760–1763 CrossRef CAS;
(k) Z. Fu, N. Deng, S.-N. Su, H. Li, R.-Z. Li, X. Zhang, J. Liu and D. Niu, Angew. Chem., Int. Ed., 2018, 57, 15217–15221 CrossRef CAS;
(l) A. K. Simlandy, B. Ghosh and S. Mukherjee, Org. Lett., 2019, 21, 3361–3366 CrossRef CAS PubMed;
(m) Y.-W. Xu and X.-P. Hu, Org. Lett., 2019, 21, 8091–8096 CrossRef CAS;
(n) Q. Zhu, B. Meng, C. Gu, Y. Xu, J. Chen, C. Lei and X. Wu, Org. Lett., 2019, 21, 9985–9989 CrossRef CAS PubMed;
(o) Z.-J. Zhang, L. Zhang, R.-L. Geng, J. Song, X.-H. Chen and L.-Z. Gong, Angew. Chem., Int. Ed., 2019, 58, 12190–12194 CrossRef CAS.
-
(a) J. T. Xia and X. P. Hu, Org. Lett., 2020, 22, 1102–1107 CrossRef CAS PubMed;
(b) Y.-W. Xu, L. Li and X.-P. Hu, Org. Lett., 2020, 22, 9534–9538 CrossRef CAS PubMed.
- For selected reviews, see:
(a) S. Krautwald and E. M. Carreira, J. Am. Chem. Soc., 2017, 139, 5627–5639 CrossRef CAS;
(b) L. L. Lin and X. M. Feng, Chem.–Eur. J., 2107, 23, 6464–6482 CrossRef PubMed;
(c) I. P. Beletskaya, C. Nájera and M. Yus, Chem. Rev., 2018, 118, 5080–5200 CrossRef CAS PubMed.
-
(a) X.-J. Chen, W. Zhu, W.-K. Qian, E.-G. Feng, Y. Zhou, J.-F. Wang, H.-L. Jiang, Z.-J. Yao and H. Liu, Adv. Synth. Catal., 2012, 354, 2151–2156 CrossRef CAS;
(b) J. Zhou, L.-N. Jia, L. Peng, Q.-L. Wang, F. Tian, X.-Y. Xu and L.-X. Wang, Tetrahedron, 2014, 70, 3478–3484 CrossRef CAS;
(c) J. Gao, J.-R. Chen, S.-W. Duan, T.-R. Li, L.-Q. Lu and W.-J. Xiao, Asian J. Org. Chem., 2014, 3, 530–535 CrossRef CAS;
(d) J.-H. Mao, Y.-B. Wang, L.-M. Yang, S.-H. Xiang, Q.-H. Wu, Y. Cui, Q. Lu, J. Lv, S.-Y. Li and B. Tan, Nat. Chem., 2021, 13, 982–991 CrossRef CAS PubMed.
- C. Girard and H. B. Kagan, Angew. Chem., Int. Ed., 1998, 37, 2922–2959 CrossRef PubMed.
Footnotes |
† Electronic supplementary information (ESI) available. CCDC 2181911 and 2181912. For ESI and crystallographic data in CIF or other electronic format see https://doi.org/10.1039/d2ra04603b |
‡ Jiaomei Wang and Yu Zhao contributed equally to this work. |
|
This journal is © The Royal Society of Chemistry 2022 |
Click here to see how this site uses Cookies. View our privacy policy here.