DOI:
10.1039/C4RA14972F
(Paper)
RSC Adv., 2015,
5, 15341-15349
Fluorene-based novel gold(I) complexes with aggregation-induced emission (AIE) or aggregate fluorescence change characteristics: from green to white emission†
Received
21st November 2014
, Accepted 26th January 2015
First published on 26th January 2015
Abstract
In this paper, five fluorene-based gold(I) complexes are synthesized and characterized. They exhibit typical aggregation-induced emission (AIE) properties or significant aggregate fluorescence change behaviors. Their thin-film and solid state fluorescence spectra are consistent with the luminescence of all luminogens in DMF–H2O mixtures with high water content. The emission color changes with different numbers of fluorene units. Among them, it is remarkable that luminogens 4 and 5 emit a bright white light in the thin-film and solid state. In addition, their thin-film absolute fluorescence quantum yields are also investigated. The results indicate the luminogen 4 gold(I) complex, with three fluorene units, exhibits the highest thin-film emission quantum yield, which is up to 65.42%.
Introduction
Recently, the development of highly emissive fluorescent organic molecules has been a popular research topic, and it has attracted extensive attention due to their potential uses such as photoelectronic devices, displays, biotechnology and memory systems.1 However, light emissions of most luminogenic materials conspicuously decrease when aggregated in the condensed phase. It is well-known that the phenomenon is referred to as aggregation-caused quenching (ACQ),2 which has greatly hindered the practical applications of organic luminescent materials. Fortunately, Tang et al. observed some propeller-shaped molecules showed the phenomenon differing from the ACQ effect in 2001. The phenomenon was called aggregation-induced emission (AIE).3 In 2002, Park et al. reported that a new class of CN-MBE nanoparticles exhibited a strongly enhanced fluorescence emission, which was known as aggregation-induced emission enhancement (AIEE).4 Since then, many AIE or AIEE fluorescent materials have been proved promising as emitters for the manufacture of effective electroluminescent devices.5 Fluorene based oligomers and polymers are highly valuable candidates for application in light emitting diodes due to adjustable electronic properties and moderate structural fragility. Whereas ACQ is the chief bottleneck for achieving efficient use of these materials with fluorene as skeletons. Thus it is very significant and challenging to develop highly emissive luminogens based on fluorene in the aggregated state. To date, a majority of AIE materials have been organic compounds.6 Meanwhile, some metal-bearing AIE luminogens, such as rhenium(I),7 zinc(II),8 platinum(II)9 and iridium(III)10 complexes, have also been reported. However, the AIE luminogens based on gold(I) complexes have rarely been investigated.11 In fact, it is proverbial that gold(I) chemistry has aroused widespread interesting over the last two decades.12 Therefore, the study of gold(I) complexes with AIE behaviors is important and urgent. In addition, White light-emitting materials have attracted great attention due to their enormous potential in different lighting applications.13 Nevertheless, most white light materials reported to date realize white light emission via the combination of red/blue/green or blue/orange emitters.14 Unfortunately, these procedures include complex mixing or doping schemes, which may cause some problems such as various requirement for device fabricate. Compared to the multi-emitting-component white light sources, a single-emitting-component white light sources exhibit many merits, such as better stability, and a conciser fabrication process.15 Herein, five fluorene-based gold(I) complexes were designed and synthesized. Their synthesis and characterization are depicted, and their aggregate fluorescence change or AIE characteristics are investigated as well.
Result and discussion
Synthesis
The diisocyano-based binuclear gold(I) complexes 1, 3 and 4 were prepared according to the synthetic routes presented in Schemes 1 and 2. The tetraisocyano-based tetranuclear gold(I) complexes 2 and 5 were synthesized according to Scheme 3. The intermediate products 1c and 2e–5e as starting materials were reacted with C6F5Au(tht) (tht = tetrahydrothiophene) to provide the target gold(I) complexes 1–5 in high yields, respectively.
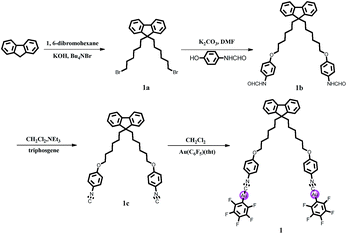 |
| Scheme 1 Synthesis route of luminogen 1. | |
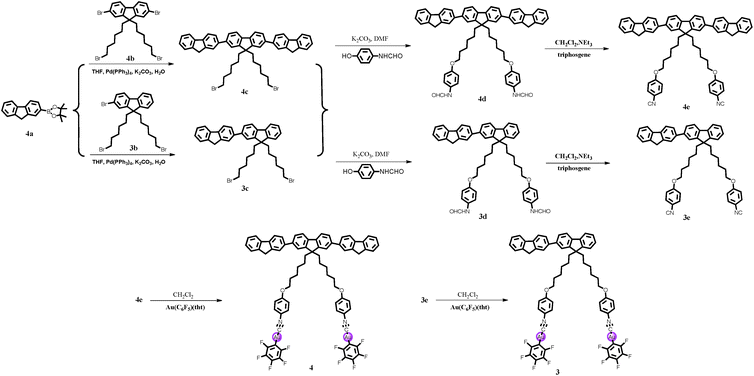 |
| Scheme 2 Synthesis routes of luminogens 3 and 4. | |
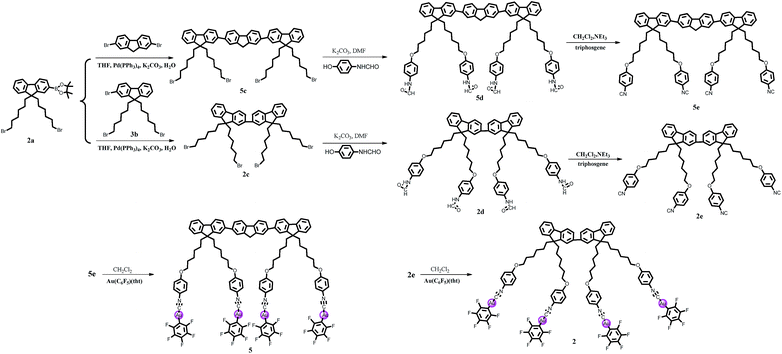 |
| Scheme 3 Synthesis routes of luminogens 2 and 5. | |
X-ray structure of complex 4
Single crystals of luminogen 4 which were appropriate for X-ray analysis were obtained via slow diffusion of n-hexane into a dichloromethane solution containing small amounts of complex 4. Detailed crystal data and further information on data collection have been described.16 As shown in Fig. 1b, molecules of 4 have no intramolecular π–π interactions and gold–gold interactions, with Au⋯Au distances of 13.090 Å. However, the existence of intermolecular π–π interactions (d = 3.048 Å, 2.392 Å, 3.299 Å, 3.360 Å, Fig. 1c) and intermolecular C–H⋯F interactions (dH⋯F = 2.485 Å, 2.665 Å, Fig. S1, ESI†) contribute to molecular packing.16
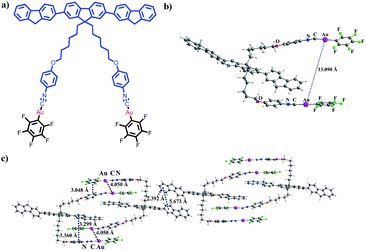 |
| Fig. 1 (a) The structure of complex 4. (b) The single crystal structure of complex 4. (c) The structural organization of complex 4. | |
Aggregation-induced emission (AIE) property of complex 1
Subsequently, the AIE behavior of luminogen 1 was investigated. The UV-Vis absorption spectra of 1 in DMF–H2O mixtures with various water fraction (fw) exhibited level-off tails in the visible region as the fw values were increased (Fig. S2, ESI†). Such tails are attributed to the Mie scattering effect,17 which is commonly observed in nanoparticle suspensions. As shown in Fig. 2, when a dilute DMF solution of 1 was excited at 330 nm, the photoluminescence (PL) signals were extremely weak, and the solution was hardly emissive under 365 nm UV illumination. However, when the water fraction in the DMF solution was increased to 30%, a green emission was observed, with λmax at 519 nm. The emission intensity was approximately 64-fold higher than the intensity in pure DMF (Fig. S3, ESI†). This is because luminogen 1 is insoluble in water, so increasing the water fraction in the mixed solvent leaded to the formation of aggregated particles. In fact, the aggregated particles obtained were characterized by DLS (dynamic light scattering). The results indicated the presence of nano-aggregates as a main component (Fig. S4, ESI†). The nano-aggregates caused the generation of gold–gold interactions, which resulted in the green emission. Therefore, luminogen 1 exhibited excellent AIE behavior. The changes to the PL spectra with further increases of fw values were negligible.
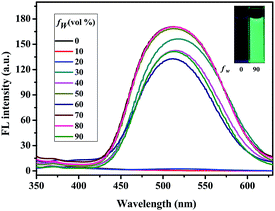 |
| Fig. 2 PL spectra of the dilute solutions of luminogen 1 (1.0 × 10−5 mol L−1) in DMF–H2O mixtures with different volume fractions of water. Excitation wavelength = 330 nm. The inset shows the emission images of 1 (1.0 × 10−5 mol L−1) in pure DMF as well as 90% water fraction. | |
Aggregate fluorescence change behaviors of luminogens 2 and 3
The level-off tails in the long-wavelength areas were also observed in the UV-Vis absorption spectra of luminogens 2 and 3 in DMF–H2O mixtures with high water contents (Fig. S5, ESI†). The phenomena are the signals of nano-aggregates formation as well.17 As is presented in Fig. 3a, in pure DMF, 2 exhibited two emission bands with λmax at 353 nm, 421 nm and 441 nm, corresponding to blue emission under 365 nm UV light. When fw reached 20%, one new emission band was observed with λmax at 516 nm. Correspondingly, the emission color changed from blue to green under 365 nm UV irradiation. Generation of the new emission band should be attributed to the formation of aggregation. Simultaneously, 2 are indeed different from traditional AIE molecules. It was not non-emissive in solution, but emitted a blue fluorescence. Therefore, it exhibited an aggregate fluorescence change phenomenon, which is an interesting and uncommon emission behavior.6b The fluorescence property of luminogen 3 in DMF–H2O mixtures with various fw was similar to that of 2 (Fig. 3c). It also displayed the aggregate fluorescence change property involving the fluorescence color transformation from blue to light green.
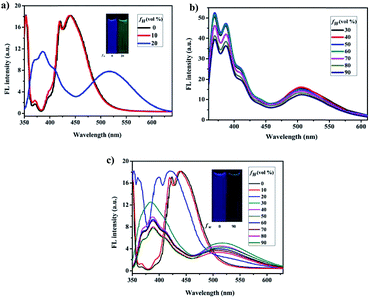 |
| Fig. 3 (a) PL spectra of the dilute solutions of luminogen 2 (1.0 × 10−5 mol L−1) in DMF–H2O mixtures with different volume fractions of water (0–20%). Excitation wavelength = 330 nm. The inset shows the emission images of 2 (1.0 × 10−5 mol L−1) in pure DMF as well as 20% water fraction. (b) PL spectra of the dilute solutions of luminogen 2 (1.0 × 10−5 mol L−1) in DMF–H2O mixtures with different volume fractions of water (30–90%). Excitation wavelength = 330 nm. (c) PL spectra of the dilute solutions of luminogen 3 (2.0 × 10−5 mol L−1) in DMF–H2O mixtures with different volume fractions of water (0–90%). Excitation wavelength = 330 nm. The inset shows the emission images of 3 (2.0 × 10−5 mol L−1) in pure DMF as well as 90% water fraction. | |
Aggregation-induced white light emission effects of luminogens 4 and 5
To study the aggregation-induced properties of luminogens 4 and 5, the UV spectra and photoluminescence spectra were determined in DMF–H2O mixtures with different fw. Luminogen 5 showed similar absorption spectra with absorption maxima (λmax) at around 272 nm and 352 nm in DMF–H2O mixtures with various water contents (Fig. S6, ESI†). As shown in Fig. 4a, luminogen 5 in pure DMF (1.0 × 10−5 mol L−1) exhibited two emission bands with λmax at 376 nm, 452 nm and 471 nm, and emitted a blue-green fluorescence under 365 nm UV illumination due to the presence of three fluorene units. However, when the water fraction in the DMF solution was increased to 20%, two new fluorescence bands were observed with λmax at 399 nm, 418 nm and 538 nm. Correspondingly, luminogen 5 displayed white light emission (Fig. 4c). Moreover, 5 emitted a bright white light when the water fraction exceeded 20%, and the emission bands were very broad and covered the whole visible range (Fig. 4b). Similarly, luminogen 4 is also a aggregation-induced white light-emitting gold(I) complex.16 It was speculated that intermolecular π–π interactions, C–H⋯F interactions and weak intermolecular aurophilic interactions were responsible for white light emission.
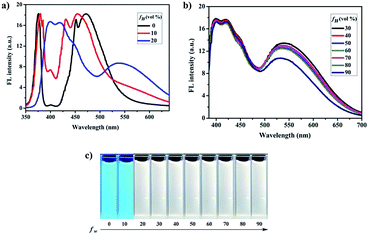 |
| Fig. 4 (a) PL spectra of the dilute solutions of luminogen 5 (1.0 × 10−5 mol L−1) in DMF–H2O mixtures with different volume fractions of water (0%, 10% and 20%). Excitation wavelength = 330 nm. (b) PL spectra of the dilute solutions of luminogen 5 (1.0 × 10−5 mol L−1) in DMF–H2O mixtures with different volume fractions of water (30–90%). Excitation wavelength = 365 nm. (c) The fluorescence images of luminogen 5 (concentration: 1.0 × 10−5 mol L−1) in different DMF–H2O mixtures with different water fractions (fw) under 365 nm UV light. | |
Thin-film and solid state fluorescence behaviors
Next, we evaluated the thin-film and solid fluorescence behaviors of luminogens 1, 2, 3, 4 and 5. As shown in Fig. 5, both the thin-film and solid fluorescence spectra of complex 1 showed single emission bands with λmax at around 499 nm. Thin-film and solid of 1 emitted strong green fluorescence under 365 nm UV light. Furthermore, the thin-film emission efficiency of 1 was superior with an absolute fluorescence quantum yield up to 49.56%. The thin-film fluorescence spectra of luminogens 2 and 3 exhibited two emission bands with λmax at around 389 nm and 501 nm (Fig. 6). Their solid state fluorescence spectra were also similar to the thin-film spectra. The thin-film absolute fluorescence quantum yields of 2 and 3 were 5.80% and 5.42%, respectively. As shown in Fig. 7, complex 5 exhibited very broad (white-light) emission covering the entire visible spectrum in the state of solid and thin-film. The thin-film absolute fluorescence quantum yield of 5 was 10.63%.
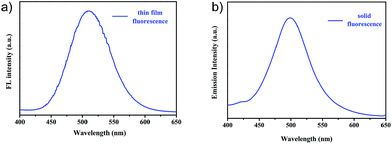 |
| Fig. 5 (a) Thin-film fluorescence spectrum of luminogen 1 (excitation wavelength = 365 nm). (b) Solid fluorescence spectrum of luminogen 1 (excitation wavelength = 365 nm). | |
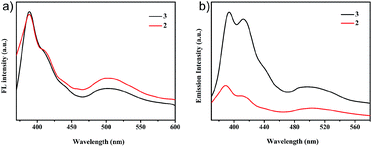 |
| Fig. 6 (a) Thin-film fluorescence spectra of luminogens 2 and 3 (excitation wavelength = 330 nm). (b) Solid fluorescence spectra of luminogens 2 and 3 (excitation wavelength = 330 nm). | |
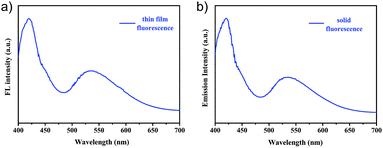 |
| Fig. 7 (a) Thin-film fluorescence spectrum of luminogen 5 (excitation wavelength = 365 nm). (b) Solid fluorescence spectrum of 5 (excitation wavelength = 365 nm). | |
Besides, thin-film and solid of luminogen 4 showed the white light-emitting as well.16 Hence, complexes 4 and 5 emitted direct white light in the solid state without involving complicated mixing or doping procedures. Moreover, the thin-film emission efficiency of 4 was most superior among these luminogens with an fluorescence quantum yield of 65.42%. The solid and thin-film luminescence of all luminogens were consistent with their fluorescence in DMF–H2O mixtures with high water fraction.
Experimentals
General
All manipulations were completed under an argon atmosphere via using standard Schlenk techniques, unless otherwise stated. All starting materials were purchased commercially as analytical-grade and used without further purification. Compounds 1a,18 2a,19 3b,20 4a,19 4b,18 N-(4-hydroxyphenyl)formamide21 and C6F5Au(tht)22 were prepared by procedures described in the corresponding literatures. EI-MS was obtained using Thermo scientific DSQII. 1H NMR (400 MHz) and 13C NMR (100.6 MHz) spectra were collected on American Varian Mercury Plus 400 spectrometer (400 MHz). 1H NMR spectra are reported as followed: chemical shift in ppm (δ) relative to the chemical shift of TMS at 0.00 ppm, integration, multiplicities (s = singlet, d = doublet, t = triplet, m = multiplet), and coupling constant (Hz). 13C NMR chemical shifts reported in ppm (δ) relative to the central line of triplet for CDCl3 at 77 ppm. 19F NMR chemical shifts are relative to C6F6 (δ = −163.00). Elemental analyses (C, H, N) were performed by the Microanalytical Services, College of Chemistry, CCNU. UV-Vis spectra were obtained on U-3310 UV Spectrophotometer. Fluorescence spectra were recorded on a Fluoromax-P luminescence spectrometer (HORIBA JOBIN YVON INC.). The absolute fluorescence quantum yields of thin-films were measured by Edinburgh Instruments FLS900. Dynamic light scattering (DLS) measurements were performed on the Zetasizer instrument ZEN3600 (Malvern, UK) with a 173° back scattering angle and He–Ne laser (λ = 633 nm). The X-ray crystal-structure determination of complex 4 was obtained on a Bruker APEX DUO CCD system. Column chromatographic separations were carried out on silica gel (200–300 mesh). TLC was performed by using commercially prepared 100–400 mesh silica gel plates (GF254) and visualization was effected at 254 nm.
Synthesis of luminogen 1
Synthesis of 1b. A mixture of compounds 1a (2.2 mmol, 1.1 g), N-(4-hydroxyphenyl)formamide (6.6 mmol), K2CO3 (26.4 mmol) were stirred in DMF (50 mL) for overnight under an argon atmosphere at 60 °C. After completion of present reaction, DMF was removed from reaction system by vacuum distillation. The residual mixtures were extracted with dichloromethane (3 × 20 mL). The combined organic layers were washed with brine, dried (Na2SO4), and concentrated in vacuo. The residues were purified by column chromatography, affording the expected light yellow liquid product in a yield of 66.7%. 1H NMR (400 MHz, CDCl3): δ (ppm) = 8.49–8.39 (m, 2H), 8.25 (s, 1H), 7.70 (t, J = 8 Hz, 3H), 7.39 (d, J = 12 Hz, 2H), 7.33–7.25 (m, 6H), 6.97 (d, J = 8 Hz, 2H), 6.77 (t, J = 8 Hz, 4H), 3.77 (d, J = 4 Hz, 4H), 1.96 (d, J = 8 Hz, 4H), 1.55 (d, J = 4 Hz, 4H), 1.19 (s, 4H), 1.09 (s, 4H), 0.64 (s, 4H). 13C NMR (100 MHz, CDCl3): δ (ppm) = 163.22, 159.09, 156.94, 156.04, 150.31, 140.97, 129.74, 129.31, 126.97, 126.70, 122.69, 121.65, 121.35, 119.59, 115.31, 114.65, 68.12, 68.02, 54.82, 40.17, 29.56, 28.94, 25.50, 23.56. EI-MS: m/z = 604.76 [M]+. Anal. calcd for C39H44N2O4: C, 77.45; H, 7.33; N, 4.63. Found: C, 77.59; H, 7.48; N, 4.50.
Synthesis of 1c. A CH2Cl2 suspension (15 mL) of 1b (0.7 g, 1.2 mmol) and triethylamine (5 mL) was cooled to 0 °C. To the mixture was added dropwise a CH2Cl2 solution (10 mL) of triphosgene (2.6 mmol). The mixture was refluxed for 3 h, then 10% aq. Na2CO3 (50 mL) was added dropwise at room temperature. The mixture was extracted with dichloromethane (3 × 20 mL). The combined organic layers were washed with brine, dried (Na2SO4), and concentrated in vacuo. The residues were purified by column chromatography, affording the expected light green liquid product in a yield of 57.1%. 1H NMR (400 MHz, CDCl3): δ (ppm) = 7.68 (d, J = 8 Hz, 2H), 7.33–7.22 (m, 10H), 6.74 (d, J = 8 Hz, 4H), 3.78 (t, J = 6 Hz, 4H), 1.98 (d, J = 8 Hz, 4H), 1.55 (t, J = 6 Hz, 4H), 1.18–1.09 (m, 8H), 0.63 (s, 4H). 13C NMR (100 MHz, CDCl3): δ (ppm) = 162.43, 159.25, 150.21, 140.97, 127.52, 126.94, 126.70, 122.62, 119.58, 119.06, 114.84, 68.11, 54.79, 40.18, 29.50, 28.73, 25.43, 23.52. EI-MS: m/z = 568.68 [M]+. Anal. calcd for C39H40N2O2: C, 82.36; H, 7.09; N, 4.93. Found: C, 82.51; H, 7.01; N, 4.99.
Synthesis of 1. A mixture of C6F5Au(tht) (1 mmol) and 1c (0.3 g, 0.5 mmol) was stirred in CH2Cl2 (20 mL) over night at room temperature. After completion of present reaction, the solvent was evaporated. A small amount of CH2Cl2 was added, and then a lot of n-hexane was added. Collecting the white solid product by suction filtration. Yield = 80%. 1H NMR (400 MHz, CDCl3): δ (ppm) = 7.71 (d, J = 8 Hz, 2H), 7.42 (d, J = 8 Hz, 4H), 7.32 (t, J = 8 Hz, 6H), 6.87 (d, J = 8 Hz, 4H), 3.86 (t, J = 6 Hz, 4H), 1.99 (s, 4H), 1.59 (s, 4H), 1.21–1.11 (m, 8H), 0.63 (s, 4H). 19F NMR (CDCl3): δ (ppm) = −116.92, −158.42, −163.26. Anal. calcd for C51H40Au2F10N2O2: C, 47.24; H, 3.11; N, 2.16. Found: C, 47.38; H, 3.19; N, 2.01.
Synthesis of luminogens 3 and 4
Synthesis of 3c. A mixture of 3b (4.4 mmol, 2.5 g), 4a (5.5 mmol), K2CO3 (32.8 mmol), Pd(PPh3)4 (0.1 mmol) were stirred in THF (50 mL) and H2O (5 mL) for two days at 80 °C. After completion of present reaction, the mixtures were extracted with dichloromethane (3 × 20 mL). The combined organic layers were washed with brine, dried (Na2SO4), and concentrated in vacuo. The residues were purified by column chromatography, affording the expected white solid product in a yield of 60.2%. 1H NMR (400 MHz, CDCl3): δ (ppm) = 7.72 (t, J = 8 Hz, 4H), 7.66–7.58 (m, 3H), 7.53 (d, J = 8 Hz, 2H), 7.46 (d, J = 4 Hz, 1H), 7.30–7.21 (m, 4H), 3.87 (s, 2H), 3.15 (t, J = 6 Hz, 4H), 1.92 (s, 4H), 1.53 (t, J = 8 Hz, 4H), 1.08 (d, J = 4 Hz, 4H), 0.98 (d, J = 8 Hz, 4H), 0.58 (d, J = 8 Hz, 4H). 13C NMR (100 MHz, CDCl3): δ (ppm) = 150.96, 150.49, 143.88, 143.38, 141.33, 140.79, 140.32, 140.21, 140.10, 127.04, 126.88, 126.77, 126.66, 126.06, 125.97, 125.01, 123.70, 122.72, 121.23, 120.08, 119.92, 119.74, 54.98, 40.21, 36.98, 33.92, 32.57, 29.00, 27.69, 23.51. EI-MS: m/z = 656.58 [M]+. Anal. calcd for C38H40Br2: C, 69.52; H, 6.14. Found: C, 69.68; H, 6.01.
Synthesis of 4c. A mixture of 4b (3.8 mmol, 2.5 g), 4a (9.6 mmol), K2CO3 (28.8 mmol), Pd(PPh3)4 (0.04 mmol) were stirred in THF (50 mL) and H2O (5 mL) for two days under an argon atmosphere at 80 °C. After completion of present reaction, the mixture was extracted with dichloromethane (3 × 20 mL). The combined organic layers were washed with brine, dried (Na2SO4), and concentrated in vacuo. The residues were purified by column chromatography, affording the expected white solid product in a yield of 60.0%. 1H NMR (400 MHz, CDCl3): δ (ppm) = 7.89–7.79 (m, 8H), 7.72–7.66 (m, 4H), 7.63 (s, 2H), 7.58 (d, J = 8 Hz, 2H), 7.40 (d, J = 8 Hz, 2H), 7.34 (d, J = 8 Hz, 2H), 4.01 (s, 4H), 3.27 (t, J = 6 Hz, 4H), 2.10 (s, 4H), 1.65 (d, J = 8 Hz, 4H), 1.23 (s, 4H), 1.12 (s, 4H), 0.76 (s, 4H). 13C NMR (100 MHz, CDCl3): δ (ppm) = 151.31, 143.93, 143.42, 141.38, 140.88, 140.38, 140.13, 139.97, 126.80, 126.69, 126.22, 125.99, 125.02, 123.70, 121.30, 120.10, 119.93, 55.19, 40.27, 37.01, 33.81, 32.60, 29.01, 27.70, 23.62. EI-MS: m/z = 820.63 [M]+. Anal. calcd for C51H48Br2: C, 74.63; H, 5.89. Found: C, 74.69; H, 5.85.
Synthesis of 4d. A mixture of compounds 4c (1.8 mmol, 1.5 g), N-(4-hydroxyphenyl)formamide (5.5 mmol), K2CO3 (21.9 mmol) were stirred in DMF (50 mL) for overnight at 60 °C. After completion of present reaction, DMF was removed from reaction system by vacuum distillation. The residual mixture was extracted with dichloromethane (3 × 20 mL). The combined organic layers were washed with brine, dried (Na2SO4), and concentrated in vacuo. The residues were purified by column chromatography, affording the expected yellow solid product in a yield of 58.0%. 1H NMR (400 MHz, CDCl3): δ (ppm) = 8.39 (d, J = 12 Hz, 1H), 8.21 (s, 1H), 7.84–7.65 (m, 13H), 7.56 (d, J = 8 Hz, 2H), 7.40–7.24 (m, 8H), 7.12 (d, J = 12 Hz, 1H), 6.86 (d, J = 8 Hz, 2H), 6.71 (d, J = 8 Hz, 4H), 3.94 (s, 4H), 3.74 (d, J = 4 Hz, 4H), 2.11 (s, 4H), 1.54 (s, 4H), 1.25 (d, J = 4 Hz, 4H), 1.16 (d, J = 4 Hz, 4H), 0.79 (s, 4H). 13C NMR (100 MHz, CDCl3): δ (ppm) = 162.64, 158.57, 151.46, 143.98, 143.47, 141.42, 140.87, 140.28, 129.05, 126.87, 126.17, 126.01, 125.10, 123.72, 121.65, 121.42, 120.10, 119.97, 115.44, 114.86, 68.21, 55.31, 40.25, 37.03, 29.50, 28.98, 25.49, 23.64. EI-MS: m/z = 933.71 [M]+. Anal. calcd for C65H60N2O4: C, 83.66; H, 6.48; N, 3.00. Found: C, 83.70; H, 6.40; N, 3.02.
Synthesis of 3d. A mixture of compounds 3c (1.5 mmol, 1.0 g), N-(4-hydroxyphenyl)formamide (4.6 mmol), K2CO3 (18.3 mmol) were stirred in DMF (50 mL) for overnight at 60 °C. After completion of present reaction, DMF was removed from reaction system by vacuum distillation. The residual mixture was extracted with dichloromethane (3 × 20 mL). The combined organic layers were washed with brine, dried (Na2SO4), and concentrated in vacuo. The residues were purified by column chromatography, affording the expected yellow solid product in a yield of 48.2%. 1H NMR (400 MHz, CDCl3): δ (ppm) = 8.43 (d, J = 12 Hz, 1H), 8.28 (s, 1H), 7.83–7.56 (m, 10H), 7.41–7.33 (m, 7H), 6.99 (s, 1H), 6.92 (d, J = 8 Hz, 2H), 6.76 (d, J = 8 Hz, 4H), 3.95 (s, 2H), 3.78 (d, J = 4 Hz, 4H), 2.04 (s, 4H), 1.56 (s, 4H), 1.23 (s, 4H), 1.13 (s, 4H), 0.71 (s, 4H). 13C NMR (100 MHz, CDCl3): δ (ppm) = 163.06, 158.93, 156.96, 156.07, 151.11, 150.63, 143.88, 143.39, 141.33, 140.73, 140.26, 129.69, 129.24, 127.01, 126.84, 125.99, 125.92, 125.03, 123.65, 122.77, 121.61, 121.36, 120.07, 119.89, 119.72, 115.28, 114.66, 68.13, 68.03, 55.03, 40.15, 36.92, 29.50, 28.93, 25.46, 23.57. EI-MS: m/z = 768.85 [M]+. Anal. calcd for C52H52N2O4: C, 81.22; H, 6.82; N, 3.64. Found: C, 81.38; H, 6.70; N, 3.71.
Synthesis of 4e. A CH2Cl2 suspension (15 mL) of 4d (0.60 g, 0.6 mmol) and triethylamine (5 mL) was cooled to 0 °C. To the mixture was added dropwise a CH2Cl2 solution (10 mL) of triphosgene (1.42 mmol). The mixture was refluxed for 3 h, then 10% aq. Na2CO3 (50 mL) was added dropwise at room temperature. The mixture was extracted with dichloromethane (3 × 20 mL). The combined organic layers were washed with brine, dried (Na2SO4), and concentrated in vacuo. The residues were purified by column chromatography, affording the expected yellow solid product in a yield of 71.0%. 1H NMR (400 MHz, CDCl3): δ (ppm) = 7.85–7.79 (m, 8H), 7.70–7.64 (m, 6H), 7.58 (d, J = 8 Hz, 2H), 7.41 (d, J = 8 Hz, 2H), 7.35 (d, J = 8 Hz, 2H), 7.18 (d, J = 4 Hz, 4H), 6.70 (d, J = 8 Hz, 4H), 3.96 (s, 4H), 3.75 (d, J = 8 Hz, 4H), 2.11 (s, 4H), 1.56 (s, 4H), 1.23 (s, 4H), 1.15 (s, 4H), 0.77 (s, 4H). 13C NMR (100 MHz, CDCl3): δ (ppm) = 162.72, 159.36, 151.37, 143.97, 143.39, 141.32, 140.97, 140.37, 140.14, 140.08, 127.59, 126.93, 126.19, 125.95, 125.08, 123.67, 121.36, 120.12, 119.96, 114.94, 68.27, 55.27, 40.32, 37.03, 29.68, 28.83, 25.46, 23.67. EI-MS: m/z = 897.09 [M]+. Anal. calcd for C65H56N2O2: C, 87.02; H, 6.29; N, 3.12. Found: C, 87.05; H, 6.35; N, 3.05.
Synthesis of 3e. A CH2Cl2 suspension (15 mL) of 3d (0.16 g, 0.2 mmol) and triethylamine (5 mL) was cooled to 0 °C. To the mixture was added dropwise a CH2Cl2 solution (10 mL) of triphosgene (0.46 mmol). The mixture was refluxed for 3 h, then 10% aq. Na2CO3 (50 mL) was added dropwise at room temperature. The mixture was extracted with dichloromethane (3 × 20 mL). The combined organic layers were washed with brine, dried (Na2SO4), and concentrated in vacuo. The residues were purified by column chromatography, affording the expected yellow solid product in a yield of 65.3%. 1H NMR (400 MHz, CDCl3): δ (ppm) = 7.82–7.55 (m, 10H), 7.40–7.32 (m, 4H), 7.19 (d, J = 8 Hz, 4H), 6.72 (d, J = 8 Hz, 4H), 3.94 (s, 2H), 3.75 (d, J = 4 Hz, 4H), 2.03 (s, 4H), 1.55 (s, 4H), 1.20–1.12 (m, 8H), 0.70 (s, 4H). 13C NMR (100 MHz, CDCl3): δ (ppm) = 162.40, 159.24, 151.01, 150.50, 143.85, 143.28, 141.20, 140.80, 140.73, 140.23, 140.00, 127.52, 127.00, 126.84, 126.75, 125.98, 125.85, 125.00, 123.59, 122.70, 121.19, 120.04, 119.95, 119.87, 119.72, 119.07, 114.82, 68.11, 55.00, 40.22, 36.91, 29.48, 28.75, 25.42, 23.55. EI-MS: m/z = 732.59 [M]+. Anal. calcd for C52H48N2O2: C, 85.21; H, 6.60; N, 3.82. Found: C, 85.33; H, 6.73; N, 3.70.
Synthesis of 4. A mixture of C6F5Au(tht) (0.46 mmol) and 4e (0.2 g, 0.22 mmol) was stirred in CH2Cl2 (20 mL) over night at room temperature. After completion of present reaction, the solvent was evaporated. A small amount of CH2Cl2 was added, and then a lot of n-hexane was added. Collecting the white solid product by suction filtration. Yield = 91%. 1H NMR (400 MHz, CDCl3): δ (ppm) = 7.81 (t, J = 12 Hz, 9H), 7.68 (t, J = 8 Hz, 6H), 7.58 (d, J = 4 Hz, 2H), 7.43 (t, J = 8 Hz, 2H), 7.35 (t, J = 8 Hz, 2H), 7.22 (t, J = 8 Hz, 3H), 6.72 (d, J = 12 Hz, 4H), 3.94 (s, 4H), 3.76 (t, J = 6 Hz, 4H), 2.13 (s, 4H), 1.55 (s, 4H), 1.16 (d, J = 8 Hz, 4H), 0.88 (t, J = 8 Hz, 4H), 0.78 (s, 4H). 19F NMR (CDCl3): δ (ppm) = −116.95, −158.37, −163.21. Anal. calcd for C77H56Au2F10N2O2: C, 56.91; H, 3.47; N, 1.72. Found: C, 57.02; H, 3.42; N, 1.63.
Synthesis of 3. A mixture of C6F5Au(tht) (0.17 mmol) and 3e (0.06 g, 0.08 mmol) was stirred in CH2Cl2 (20 mL) over night at room temperature. After completion of present reaction, the solvent was evaporated. A small amount of CH2Cl2 was added, and then a lot of n-hexane was added. Collecting the white solid product by suction filtration. Yield = 80.1%. 1H NMR (400 MHz, CDCl3): δ (ppm) = 7.83–7.58 (m, 11H), 7.45–7.34 (m, 7H), 6.81 (d, J = 8 Hz, 4H), 3.95 (s, 2H), 3.81 (s, 4H), 2.05 (s, 4H), 1.55 (s, 4H), 1.23 (s, 4H), 1.14 (s, 4H), 0.70 (s, 4H). 19F NMR (CDCl3): δ (ppm) = −116.99, −158.49, −163.33. Anal. calcd for C64H48Au2F10N2O2: C, 52.61; H, 3.31; N, 1.92. Found: C, 52.78; H, 3.42; N, 1.78.
Synthesis of luminogens 2 and 5
Synthesis of 2c. A mixture of 3b (3.9 mmol, 2.2 g), 2a (4.8 mmol), K2CO3 (28.9 mmol), Pd(PPh3)4 (0.1 mmol) were stirred in THF (50 mL) and H2O (5 mL) for two days at 80 °C. After completion of present reaction, the mixture was extracted with dichloromethane (3 × 20 mL). The combined organic layers were washed with brine, dried (Na2SO4), and concentrated in vacuo. The residues were purified by column chromatography, affording the expected white solid product in a yield of 67.6%. 1H NMR (400 MHz, CDCl3): δ (ppm) = 7.80–7.73 (m, 4H), 7.65 (d, J = 8 Hz, 2H), 7.61 (s, 2H), 7.36–7.30 (m, 6H), 3.28 (t, J = 6 Hz, 8H), 2.05 (s, 8H), 1.70–1.63 (m, 8H), 1.25–1.09 (m, 16H), 0.71 (d, J = 4 Hz, 8H). 13C NMR (100 MHz, CDCl3): δ (ppm) = 151.07, 150.52, 140.71, 140.39, 140.33, 127.08, 126.91, 126.14, 122.79, 121.14, 119.95, 119.77, 55.03, 40.18, 33.92, 32.56, 29.00, 27.69, 23.54. Anal. calcd for C50H62Br4: C, 61.11; H, 6.36. Found: C, 61.25; H, 6.21.
Synthesis of 5c. A mixture of 2,7-dibromofluorene (2.6 mmol, 0.85 g), 2a (6.6 mmol), K2CO3 (19.7 mmol), Pd(PPh3)4 (0.03 mmol) were stirred in THF (50 mL) and H2O (5 mL) for two days at 80 °C. After completion of present reaction, the mixtures were extracted with dichloromethane (3 × 20 mL). The combined organic layers were washed with brine, dried (Na2SO4), and concentrated in vacuo. The residues were purified by column chromatography, affording the expected white solid product in a yield of 56.7%. 1H NMR (400 MHz, CDCl3): δ (ppm) = 7.91 (t, J = 8 Hz, 4H), 7.80–7.63 (m, 10H), 7.35 (s, 6H), 4.10 (s, 2H), 3.28 (t, J = 8 Hz, 8H), 2.04 (s, 8H), 1.67 (t, J = 6 Hz, 8H), 1.21–1.10 (m, 16H), 0.68 (s, 8H). 13C NMR (100 MHz, CDCl3): δ (ppm) = 150.99, 150.50, 144.17, 140.74, 140.51, 140.27, 140.14, 127.08, 126.90, 126.08, 123.76, 122.73, 121.21, 120.20, 119.74, 55.00, 42.24, 37.10, 33.94, 32.58, 29.10, 27.70, 23.51. Anal. calcd for C63H70Br4: C, 65.98; H, 6.15. Found: C, 65.91; H, 6.23.
Synthesis of 2d. A mixture of compounds 2c (1.4 mmol, 1.4 g), N-(4-hydroxyphenyl)formamide (8.5 mmol), K2CO3 (34.2 mmol) were stirred in DMF (50 mL) for overnight at 60 °C. After completion of present reaction, DMF was removed from reaction system by vacuum distillation. The residual mixture was extracted with dichloromethane (3 × 20 mL). The combined organic layers were washed with brine, dried (Na2SO4), and concentrated in vacuo. The residues were purified by column chromatography, affording the expected white solid product in a yield of 50.8%. 1H NMR (400 MHz, CDCl3): δ (ppm) = 8.44–8.27 (m, 4H), 7.73–7.63 (m, 10H), 7.35 (s, 12H), 6.92 (s, 4H), 6.75 (s, 8H), 3.76 (s, 8H), 2.03 (s, 8H), 1.55 (s, 8H), 1.21 (s, 8H), 1.13 (s, 8H), 0.72 (s, 8H). 13C NMR (100 MHz, CDCl3): δ (ppm) = 163.26, 159.33, 156.75, 155.87, 151.10, 150.54, 140.53, 140.14, 129.79, 129.33, 126.99, 126.79, 125.92, 122.73, 121.64, 121.09, 119.88, 119.65, 115.17, 114.50, 68.00, 67.91, 54.95, 40.10, 29.51, 28.89, 25.46, 23.60. Anal. calcd for C78H86N4O8: C, 77.58; H, 7.18; N, 4.64. Found: C, 77.71; H, 7.32; N, 4.54.
Synthesis of 5d. A mixture of compounds 5c (1.5 mmol, 1.7 g), N-(4-hydroxyphenyl)formamide (8.9 mmol), K2CO3 (35.6 mmol) were stirred in DMF (50 mL) for overnight at 60 °C. After completion of present reaction, DMF was removed from reaction system by vacuum distillation. The residual mixtures were extracted with dichloromethane (3 × 20 mL). The combined organic layers were washed with brine, dried (Na2SO4), and concentrated in vacuo. The residues were purified by column chromatography, affording the expected orange red solid product in a yield of 51.8%. 1H NMR (400 MHz, CDCl3): δ (ppm) = 8.46–8.26 (m, 3H), 7.86 (d, J = 4 Hz, 3H), 7.79–7.60 (m, 12H), 7.37–7.30 (m, 14H), 6.94 (d, J = 8 Hz, 4H), 6.79–6.75 (m, 8H), 4.02 (s, 2H), 3.79 (t, J = 6 Hz, 8H), 2.05 (d, J = 8 Hz, 8H), 1.57 (s, 8H), 1.26–1.14 (m, 16H), 0.71 (s, 8H). 13C NMR (100 MHz, CDCl3): δ (ppm) = 163.10, 159.02, 156.94, 156.04, 151.14, 150.62, 144.17, 140.69, 140.30, 140.13, 129.69, 129.21, 127.05, 126.86, 126.01, 123.72, 122.78, 121.63, 121.39, 120.19, 120.01, 119.75, 115.29, 114.64, 68.09, 68.00, 55.04, 40.26, 29.57, 28.95, 25.52, 23.64. Anal. calcd for C91H94N4O8: C, 79.68; H, 6.91; N, 4.08. Found: C, 79.62; H, 6.86; N, 4.15.
Synthesis of 2e. A CH2Cl2 suspension (15 mL) of 2d (0.5 g, 0.4 mmol) and triethylamine (5 mL) was cooled to 0 °C. To the mixture was added dropwise a CH2Cl2 solution (10 mL) of triphosgene (1.8 mmol). The mixture was refluxed for 3 h, then 10% aq. Na2CO3 (50 mL) was added dropwise at room temperature. The mixture was extracted with dichloromethane (3 × 20 mL). The combined organic layers were washed with brine, dried (Na2SO4), and concentrated in vacuo. The residues were purified by column chromatography, affording the expected light yellow solid product in a yield of 55.8%. 1H NMR (400 MHz, CDCl3): δ (ppm) = 7.74 (d, J = 8 Hz, 4H), 7.66–7.62 (m, 4H), 7.38–7.32 (m, 7H), 7.23 (d, J = 8 Hz, 7H), 6.75 (d, J = 8 Hz, 8H), 3.79 (t, J = 6 Hz, 8H), 2.05 (s, 8H), 1.57 (s, 8H), 1.22 (s, 8H), 1.13 (s, 8H), 0.73 (s, 8H). 13C NMR (100 MHz, CDCl3): δ (ppm) = 162.79, 159.27, 151.16, 150.49, 140.61, 140.36, 140.22, 127.51, 127.08, 126.92, 125.96, 122.78, 121.05, 119.89, 119.71, 119.14, 114.91, 68.15, 55.02, 40.15, 29.47, 28.74, 25.42, 23.60. Anal. calcd for C78H78N4O4: C, 82.51; H, 6.92; N, 4.93. Found: C, 82.67; H, 6.83; N, 4.81.
Synthesis of 5e. A CH2Cl2 suspension (15 mL) of 5d (0.37 g, 0.27 mmol) and triethylamine (5 mL) was cooled to 0 °C. To the mixture was added dropwise a CH2Cl2 solution (10 mL) of triphosgene (1.18 mmol). The mixture was refluxed for 3 h, then 10% aq. Na2CO3 (50 mL) was added dropwise at room temperature. The mixture was extracted with dichloromethane (3 × 20 mL). The combined organic layers were washed with brine, dried (Na2SO4), and concentrated in vacuo. The residues were purified by column chromatography, affording the expected light yellow solid product in a yield of 71.4%. 1H NMR (400 MHz, CDCl3): δ (ppm) = 7.86 (d, J = 8 Hz, 4H), 7.79–7.63 (m, 10H), 7.33 (t, J = 8 Hz, 6H), 7.23 (t, J = 8 Hz, 8H), 6.75 (d, J = 12 Hz, 8H), 4.02 (s, 2H), 3.79 (t, J = 6 Hz, 8H), 2.06 (d, J = 4 Hz, 8H), 1.57 (t, J = 8 Hz, 8H), 1.22–1.14 (m, 16H), 0.70 (s, 8H). 13C NMR (100 MHz, CDCl3): δ (ppm) = 162.57, 159.35, 151.11, 150.58, 144.12, 140.81, 140.49, 140.39, 140.22, 127.59, 127.05, 126.91, 126.10, 123.69, 122.77, 121.26, 120.20, 119.79, 119.14, 114.94, 68.23, 55.10, 40.31, 29.55, 28.81, 25.49, 23.63, 22.63. Anal. calcd for C91H86N4O4: C, 84.10; H, 6.67; N, 4.31. Found: C, 84.13; H, 6.71; N, 4.25.
Synthesis of 2. A mixture of C6F5Au(tht) (0.22 mmol) and 2e (0.06 g, 0.05 mmol) was stirred in CH2Cl2 (20 mL) over night at room temperature. After completion of present reaction, the solvent was evaporated. A small amount of CH2Cl2 was added, and then a lot of n-hexane was added. Collecting the white solid product by suction filtration. Yield = 78.3%. 1H NMR (400 MHz, CDCl3): δ (ppm) = 7.73 (s, 4H), 7.65 (s, 4H), 7.37 (d, J = 8 Hz, 14H), 6.83 (d, J = 8 Hz, 8H), 3.84 (s, 8H), 2.06 (s, 8H), 1.63–1.58 (m, 8H), 1.26 (s, 8H), 1.15 (s, 8H), 0.73 (s, 8H). 19F NMR (CDCl3): δ (ppm) = −116.99, −158.39, −163.27. Anal. calcd for C102H78Au4F20N4O4: C, 47.27; H, 3.03; N, 2.16. Found: C, 47.39; H, 3.15; N, 2.02.
Synthesis of 5. A mixture of C6F5Au(tht) (0.19 mmol) and 5e (0.06 g, 0.046 mmol) was stirred in CH2Cl2 (20 mL) over night at room temperature. After completion of present reaction, the solvent was evaporated. A small amount of CH2Cl2 was added, and then a lot of n-hexane was added. Collecting the white solid product by suction filtration. Yield = 81.6%. 1H NMR (400 MHz, CDCl3): δ (ppm) = 7.86 (s, 3H), 7.79–7.67 (m, 8H), 7.62 (s, 2H), 7.34 (d, J = 12 Hz, 15H), 6.81 (d, J = 8 Hz, 8H), 4.00 (s, 2H), 3.82 (s, 8H), 2.04 (s, 8H), 1.23 (s, 12H), 1.13 (s, 12H), 0.69 (s, 8H). 19F NMR (CDCl3): δ (ppm) = −116.78, −158.39, −163.15. Anal. calcd for C115H86Au4F20N4O4: C, 50.12; H, 3.15; N, 2.03. Found: C, 50.05; H, 3.23; N, 1.95.
Conclusions
In this work, we reported a series of novel fluorene-based gold(I) complexes and characterized their structures. Through study of their aggregation-induced properties it was discovered that complex 1 exhibited excellent aggregation-induced emission (AIE) property. Complexes 2 and 3, containing two fluorene units, showed aggregate fluorescence change behaviors. More importantly, Complexes 4 and 5, containing three fluorene units, were single-component white light-emitting luminogens with aggregate fluorescence change characteristics. Thus, the two novel luminogens have potential value of applications in the field of white organic light-emitting diodes (WOLEDS). Furthermore, in thin-films and in the solid state, these luminogens with different fluorene units showed intense green, light-green or white emission. Therefore, we can adjust the solid state light-emitting of these gold(I) complexes from green to white via introducing different numbers of fluorene units. These complexes have potential applications in the field of organic light-emitting diodes (OLED). Further studies on the design of new white light emitting systems are in progress.
Acknowledgements
The authors acknowledge financial support from National Natural Science Foundation of China (21272088, 21472059 and 21402057) and the Program for Academic Leader in Wuhan Municipality (201271130441). The work was also supported by the Scientific Research Foundation for the Returned Overseas Chinese Scholars, Ministry of Education, and the Natural Science Foundation of Hubei Province (2013CFB207) and financially supported by self-determined research funds of CCNU from the colleges' basic research and operation of MOE (CCNU14A05009 and CCNU14F01003).
Notes and references
-
(a) Y. Sagara and T. Kato, Nat. Chem., 2009, 1, 605–610 CrossRef CAS PubMed
;
(b) M. Wang, G. Zhang, D. Zhang, D. Zhu and B. Z. Tang, J. Mater. Chem., 2010, 20, 1858–1867 RSC
;
(c) C. Weder, J. Mater. Chem., 2011, 21, 8235–8236 RSC
;
(d) J. Wu, W. Liu, J. Ge, H. Zhang and P. Wang, Chem. Soc. Rev., 2011, 40, 3483–3495 RSC
;
(e) Y. Hong, L. Meng, S. Chen, C. W. T. Leung, L.-T. Da, M. Faisal, D.-A. Silva, J. Liu, J. W. Y. Lam, X. Huang and B. Z. Tang, J. Am. Chem. Soc., 2012, 134, 1680–1689 CrossRef CAS PubMed
. -
(a) S. A. Jenekhe and J. A. Osaheni, Science, 1994, 265, 765–768 CAS
;
(b) C.-T. Chen, Chem. Mater., 2004, 16, 4389–4400 CrossRef CAS
;
(c) Y. Hong, J. W. Y. Lam and B. Z. Tang, Chem. Soc. Rev., 2011, 40, 5361–5388 RSC
. - J. Luo, Z. Xie, J. W. Y. Lam, L. Cheng, H. Chen, C. Qiu, H. S. Kwok, X. Zhan, Y. Liu, D. Zhu and B. Z. Tang, Chem. Commun., 2001, 1740–1741 RSC
. - B.-K. An, S.-K. Kwon, S.-D. Jung and S. Y. Park, J. Am. Chem. Soc., 2002, 124, 14410–14415 CrossRef CAS PubMed
. -
(a) Y. Hong, J. W. Y. Lam and B. Z. Tang, Chem. Commun., 2009, 4332–4353 RSC
;
(b) Y. S. Zhao, H. Fu, A. Peng, Y. Ma, D. Xiao and J. Yao, Adv. Mater., 2008, 20, 2859–2876 CrossRef CAS
. -
(a) Z. Yang, Z. Chi, T. Yu, X. Zhang, M. Chen, B. Xu, S. Liu, Y. Zhang and J. Xu, J. Mater. Chem., 2009, 19, 5541–5546 RSC
;
(b) Z. Chen, J. Liang, X. Han, J. Yin, G.-A. Yu and S. H. Liu, Dyes Pigm., 2015, 112, 59–66 CrossRef CAS PubMed
. -
(a) B. Manimaran, P. Thanasekaran, T. Rajendran, R.-J. Lin, I.-J. Chang, G.-H. Lee, S.-M. Peng, S. Rajagopal and K.-L. Lu, Inorg. Chem., 2002, 41, 5323–5325 CrossRef CAS PubMed
;
(b) V. Sathish, A. Ramdass, Z.-Z. Lu, M. Velayudham, P. Thanasekaran, K.-L. Lu and S. Rajagopal, J. Phys. Chem. B, 2013, 117, 14358–14366 CrossRef CAS PubMed
. -
(a) T. L. Bandrowsky, J. B. Carroll and J. Braddock-Wilking, Organometallics, 2011, 30, 3559–3569 CrossRef CAS
;
(b) K. Kokado and Y. Chujo, Dalton Trans., 2011, 40, 1919–1923 RSC
;
(c) B. Xu, Z. Chi, X. Zhang, H. Li, C. Chen, S. Liu, Y. Zhang and J. Xu, Chem. Commun., 2011, 47, 11080–11082 RSC
. -
(a) M.-X. Zhu, W. Lu, N. Zhu and C.-M. Che, Chem.–Eur. J., 2008, 14, 9736–9746 CrossRef CAS PubMed
;
(b) S. Y.-L. Leung and V. W.-W. Yam, Chem. Sci., 2013, 4, 4228–4234 RSC
;
(c) A. Y.-Y. Tam, K. M.-C. Wong and V. W.-W. Yam, J. Am. Chem. Soc., 2009, 131, 6253–6260 CrossRef CAS PubMed
. -
(a) Y. You, H. S. Huh, K. S. Kim, S. W. Lee, D. Kim and S. Y. Park, Chem. Commun., 2008, 3998–4000 RSC
;
(b) Q. Zhao, L. Li, F. Li, M. Yu, Z. Liu, T. Yi and C. Huang, Chem. Commun., 2008, 685–687 RSC
;
(c) K. Huang, H. Wu, M. Shi, F. Li, T. Yi and C. Huang, Chem. Commun., 2009, 1243–1245 RSC
;
(d) C. H. Shin, J. O. Huh, S. J. Baek, S. K. Kim, M. H. Lee and Y. Do, Eur. J. Inorg. Chem., 2010, 3642–3651 CrossRef CAS
;
(e) G.-G. Shan, D.-X. Zhu, H.-B. Li, P. Li, Z.-M. Su and Y. Liao, Dalton Trans., 2011, 40, 2947–2953 RSC
. -
(a) Z. Luo, X. Yuan, Y. Yu, Q. Zhang, D. T. Leong, J. Y. Lee and J. Xie, J. Am. Chem. Soc., 2012, 134, 16662–16670 CrossRef CAS PubMed
;
(b) J. Liang, Z. Chen, J. Yin, G.-A. Yu and S. H. Liu, Chem. Commun., 2013, 49, 3567–3569 RSC
;
(c) J. Liang, Z. Chen, L. Xu, J. Wang, J. Yin, G.-A. Yu, Z.-N. Chen and S. H. Liu, J. Mater. Chem. C, 2014, 2, 2243–2250 RSC
;
(d) Z. Chen, J. Zhang, M. Song, J. Yin, G.-A. Yu and S. H. Liu, Chem. Commun., 2015, 51, 326–329 RSC
. -
(a) G. J. Hutchings, M. Brust and H. Schmidbaur, Chem. Soc. Rev., 2008, 37, 1759–1765 RSC
;
(b) V. W.-W. Yam and E. C.-C. Cheng, Chem. Soc. Rev., 2008, 37, 1806–1813 RSC
;
(c) W.-Y. Wong, K.-H. Choi, G.-L. Lu, J.-X. Shi, P.-Y. Lai and S.-M. Chan, Organometallics, 2001, 20, 5446–5454 CrossRef CAS
;
(d) W. J. Hunks, M. C. Jennings and R. J. Puddephatt, Inorg. Chem., 2000, 39, 2699–2702 CrossRef CAS
;
(e) W. Lu, W.-M. Kwok, C. Ma, C. T.-L. Chan, M.-X. Zhu and C.-M. Che, J. Am. Chem. Soc., 2011, 133, 14120–14135 CrossRef CAS PubMed
;
(f) H. Schmidbaur and A. Schier, Chem. Soc. Rev., 2012, 41, 370–412 RSC
;
(g) E. R. T. Tiekink, Coord. Chem. Rev., 2014, 275, 130–153 CrossRef CAS PubMed
. -
(a) G. M. Farinola and R. Ragni, Chem. Soc. Rev., 2011, 40, 3467–3482 RSC
;
(b) B. Zhang, G. Tan, C.-S. Lam, B. Yao, C.-L. Ho, L. Liu, Z. Xie, W.-Y. Wong, J. Ding and L. Wang, Adv. Mater., 2012, 24, 1873–1877 CrossRef CAS PubMed
. - Y. Uchida and T. Taguchi, Opt. Eng., 2005, 44, 124003 CrossRef PubMed
. -
(a) B.-P. Yan, C. C. C. Cheung, S. C. F. Kui, H.-F. Xiang, V. A. L. Roy, S.-J. Xu and C.-M. Che, Adv. Mater., 2007, 19, 3599–3603 CrossRef CAS
;
(b) Y. Liu, M. Nishiura, Y. Wang and Z. Hou, J. Am. Chem. Soc., 2006, 128, 5592–5593 CrossRef CAS PubMed
;
(c) Z. Zhao, Y. Zhao, P. Lu and W. Tian, J. Phys. Chem. C, 2007, 111, 6883–6888 CrossRef CAS
;
(d) Z. Zhao, B. Xu, Z. Yang, H. Wang, X. Wang, P. Lu and W. Tian, J. Phys. Chem. C, 2008, 112, 8511–8515 CrossRef CAS
;
(e) X.-H. Jin, C. Chen, C.-X. Ren, L.-X. Cai and J. Zhang, Chem. Commun., 2014, 50, 15878–15881 RSC
. - Z. Chen, D. Wu, X. Han, J. Liang, J. Yin, G.-A. Yu and S. H. Liu, Chem. Commun., 2014, 50, 11033–11035 RSC
. - C. W. T. Leung, Y. Hong, S. Chen, E. Zhao, J. W. Y. Lam and B. Z. Tang, J. Am. Chem. Soc., 2013, 135, 62–65 CrossRef CAS PubMed
. - S. Liu, K. Zhang, J. Lu, J. Zhang, H.-L. Yip, F. Huang and Y. Cao, J. Am. Chem. Soc., 2013, 135, 15326–15329 CrossRef CAS PubMed
. - B. Liu and S. K. Dishari, Chem.–Eur. J., 2008, 14, 7366–7375 CrossRef CAS PubMed
. - B. Liu, B. S. Gaylord, S. Wang and G. C. Bazan, J. Am. Chem. Soc., 2003, 125, 6705–6714 CrossRef CAS PubMed
. - K. Bahrami, M. M. Khodaei, H. Targhan and M. S. Arabi, Tetrahedron Lett., 2013, 54, 5064–5068 CrossRef CAS PubMed
. - R. Uson, A. Laguna and M. Laguna, Inorg. Synth., 1989, 26, 85–91 CrossRef CAS
.
Footnote |
† Electronic supplementary information (ESI) available: UV-Vis absorption spectra of luminogens 1, 2, 3, 4 and 5 in DMF–H2O mixtures with different water contents, and characterization datas mentioned in the paper. See DOI: 10.1039/c4ra14972f |
|
This journal is © The Royal Society of Chemistry 2015 |
Click here to see how this site uses Cookies. View our privacy policy here.