DOI:
10.1039/C5QO00353A
(Research Article)
Org. Chem. Front., 2016,
3, 222-226
Copper-catalyzed aminotrifluoromethylation of alkenes: a facile synthesis of CF3-containing lactams†
Received
5th November 2015
, Accepted 5th December 2015
First published on 10th December 2015
Abstract
Copper-catalyzed aminotrifluoromethylation of alkenes using amides as nucleophiles has been developed. It provides a rapid and efficient access to a variety of CF3-containing lactams. The reaction proceeds under mild conditions with a good scope and functional group tolerance, offering a valuable method to prepare CF3-containing lactams that are of great potential in pharmaceuticals and agrochemicals.
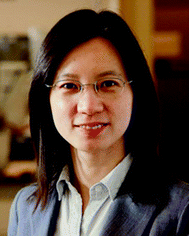 Qiu Wang | Professor Qiu Wang received her Ph.D. in organic chemistry from Emory University under the direction of Professor Albert Padwa (2005). She undertook postdoctoral trainings with Professor Andrew Myers at Harvard University (2005–2007) and Professor Stuart Schreiber at the Broad Institute of Harvard and MIT (2007–2011). She started her independent career in 2011 as an assistant professor of chemistry at Duke University. Her research interests focus on the synthesis and chemical biology of biologically important nitrogen-containing molecules. |
The pharmacological profile of organic molecules can be significantly improved through the introduction of fluorine.1 In particular, the trifluoromethyl group has attracted substantial attention in the fields of pharmacology and agrochemistry due to its favorable influence on lipophilicity, hydrophobicity, and metabolic stability.2 A variety of synthetic methods have been developed towards the installation of the trifluoromethyl group into organic molecules.2–4 Over the past several years, transition-metal-mediated alkene trifluoromethylation has emerged as a powerful approach.3 For example, copper-catalyzed allylic trifluoromethylation of terminal alkenes has been reported by several groups (Scheme 1a).5 In addition, multiple alkene difunctionalization reactions incorporating trifluoromethylation have been successfully established,6,7 including carbotrifluoromethylation,8 oxytrifluoromethylation,9 and aminotrifluoromethylation10 of simple alkenes (Scheme 1b). The Buchwald group reported an elegant study on the copper-catalyzed intramolecular oxytrifluoromethylation of alkenes with Togni's reagent, employing carboxylic acids, phenols or alcohols as nucleophiles to form oxygen-containing heterocycles.9d,e Recently, aminotrifluoromethylation of terminal alkenes with Togni's reagent has been reported by the Sodeoka10c and Liu10d,e groups using amines or protected amines as nucleophiles to construct trifluoromethylated aziridines, pyrrolidines and indolines. Though a wide range of trifluoromethylated scaffolds are accessible through current methods, there are no examples of the aminotrifluoromethylation of unsaturated amides towards the synthesis of CF3-containing lactams. In contrast to the successful oxytrifluoromethylation of unsaturated carboxylic acids, it remains challenging to employ amides as nucleophiles in trifluoromethylation-incorporated olefin difunctionalization.9d With the ubiquitous presence of lactams in synthetic building blocks, bioactive compounds, natural products and pharmaceuticals,11 it would be of great value to synthesize CF3-containing lactams. Herein, we report the first example of the introduction of the trifluoromethyl group into lactams via aminotrifluoromethylation of simple alkenes.
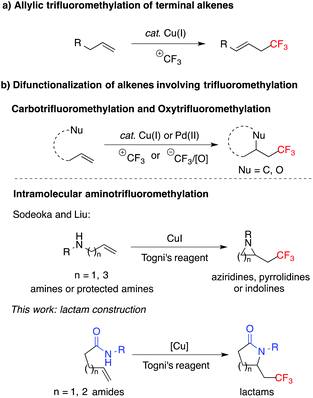 |
| Scheme 1 Alkene trifluoromethylation. | |
Recently, our group has reported a copper-catalyzed alkene diamination reaction in which the protecting group on the amide played a critical role in the formation of the lactam products.12 We postulated that the protecting group on the amide would once again be critical in the proposed aminotrifluoromethylation reaction. Thus, we examined the copper-catalyzed reactions of 2-vinylbenzamides 1 containing different protecting groups using Togni's reagent 2 (Table 1). Interestingly, two types of trifluoromethylation products were observed depending on the protecting groups. In the case of 1a–1c with H, Ph, or Bn groups, trifluoromethylated alkenes 3a′–3c′ were formed.13 However, in the reaction of 1d with an OMe group, aminotrifluoromethylated lactam 3d was successfully formed. These results suggested that the alkoxyl group on the nitrogen played an important role in the trifluoromethylation reaction and facilitating the radical cyclization via nitrogen trapping.
Table 1 Trifluoromethylation reactions of 2-vinylbenzamidesa
Reaction conditions: 1 (0.2 mmol, 1 equiv.), 2 (0.3 mmol, 1.5 equiv.), Cu(CH3CN)4PF6 (0.04 mmol, 20 mol%), MeOH (1 mL), 80 °C, and 2 h.
|
|
With our preliminary success, we next used unactivated 2-allyl-N-methoxybenzamide 1e as the model substrate to optimize the aminotrifluoromethylation conditions (Table 2). In contrast to the successful formation of isoindolinone product 3d, aminotrifluoromethylated product 3,4-dihydroisoquinolinone 3e was formed in only 17% yield under the initial conditions with Cu(CH3CN)4PF6 as the catalyst in MeOH at 80 °C (Table 2, entry 1). Among the set of copper catalysts examined, Cu(acac)2 was found to be the best, providing 3e in 49% yield (Table 2, entries 1–8). Methanol proved to be the optimal solvent for the formation of the desired product 3e (Table 2, entries 8–15). Lowering the reaction temperatures resulted in a significant decrease in efficiency (Table 2, entries 16–18). Finally, increasing the amount of Togni's reagent (2 equivalents) led to a much improved yield of 3e (72%), which was chosen as the standard conditions for alkene aminotrifluoromethylation.
Table 2 Condition optimization for alkene aminotrifluoromethylationa

|
Entry |
Catalyst |
Solvent |
Temp. (°C) |
Yieldb |
Reaction conditions: 1 (0.2 mmol, 1 equiv.), 2 (0.30 mmol, 1.5 equiv.), Cu(acac)2 (0.04 mmol, 20 mol%), MeOH (2 mL), 80 °C, and 12 h, unless otherwise noted.
Yields determined by 19F NMR with CF3Ph as an internal standard.
2 (0.4 mmol, 2 equiv.), 12 h.
|
1 |
Cu(CH3CN)4PF6 |
MeOH |
80 |
17% |
2 |
CuOTf |
MeOH |
80 |
14% |
3 |
CuOAc |
MeOH |
80 |
33% |
4 |
CuTc |
MeOH |
80 |
32% |
5 |
CuCN |
MeOH |
80 |
17% |
6 |
CuCl |
MeOH |
80 |
28% |
7 |
Cu(OAc)2 |
MeOH |
80 |
30% |
8 |
Cu(acac)2 |
MeOH |
80 |
49% |
9 |
Cu(acac)2 |
DMF |
80 |
7% |
10 |
Cu(acac)2 |
Toluene |
80 |
5% |
11 |
Cu(acac)2 |
DCE |
80 |
11% |
12 |
Cu(acac)2 |
1,4-Dioxane |
80 |
3% |
13 |
Cu(acac)2 |
THF |
80 |
3% |
14 |
Cu(acac)2 |
MTBE |
80 |
25% |
15 |
Cu(acac)2 |
CH3CN |
80 |
8% |
16 |
Cu(acac)2 |
MeOH |
60 |
45% |
17 |
Cu(acac)2 |
MeOH |
40 |
18% |
18 |
Cu(acac)2 |
MeOH |
rt |
3% |
19
|
Cu(acac)
2
|
MeOH
|
80
|
72%
|
With these optimized conditions, we examined the scope of this alkene aminotrifluoromethylation (Table 3). Both 5- and 6-membered lactam products (isoindolinone 3d and 3,4-dihydroisoquinolinone 3e) were readily formed in 82% and 89% yields, respectively. The reaction showed compatibility with a wide range of substitutions on the aryl group, including those that were electron-donating (3f), electron-withdrawing (3g), or located at a sterically obstructing ortho position (3h). In addition to N-methoxybenzamides 1d–1h, N-methoxyamides 1i–1n bearing different substituents on the alkenyl chain underwent smooth 5-exo cyclization to afford γ-lactams 3i–3n. In the amide substrates that contained a stereocenter, moderate to good diastereoselectivity was observed in the formation of lactams 3l–3n. Finally, the formation of 6-membered lactam 3o was also effective.
Table 3 . Aminotrifluoromethylation of unactivated alkenesa
Reaction conditions: 1 (0.3 mmol, 1 equiv.), 2 (0.6 mmol, 2 equiv.), Cu(acac)2 (0.06 mmol, 20 mol%), MeOH (3 mL), 80 °C, and 2–5 h. Isolation yields.
dr = diastereomeric ratio, determined by 19F NMR of the crude reaction mixture. The isolated yield includes both isomers.
dr determined by GC-MS of the crude reaction mixture, only one diastereomer is isolated.
|
|
When 1,1-disubstituted terminal alkenes 1p and 1q were examined under standard conditions, interestingly, the desired aminotrifluoromethylation products were not observed; rather the oxytrifluoromethylation products 3p and 3q were formed upon the subsequent acid-catalyzed hydrolysis. These results suggest that O-trapping is favored over N-trapping upon the increased steric hindrance (Scheme 2).14
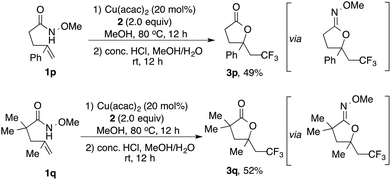 |
| Scheme 2 Oxytrifluoromethylation of 1,1-disubstituted alkenes. | |
While the mechanistic details of this copper-catalyzed aminotrifluoromethylation reaction remain unclear at present, the current results suggest it would be analogous to copper-catalyzed oxytrifluoromethylation.9d,e Furthermore, the addition of 2,2,6,6-tetramethylpiperidine-1-oxyl (TEMPO), a known radical scavenger, was found to largely inhibit the aminotrifluoromethylation reaction.15
To demonstrate the synthetic utility of the products derived from this reaction, 3,4-dihydroisoquinolinone 3e was treated with Mo(CO)6, readily providing free lactam 4e in 82% yield (Scheme 3). Furthermore, reduction using LiAlH4 afforded the CF3-containing tetrahydroisoquinoline 5e, providing effective access to trifluoromethylated piperidine derivatives.
 |
| Scheme 3 Deprotection of N-methoxyamide 3e. | |
Conclusions
In summary, an efficient copper-catalyzed aminotrifluoro-methylation of alkenes has been achieved using amides as nucleophiles under mild conditions. These reactions provide CF3-containing lactams in good yields. It offers a useful method to access a variety of CF3-containing lactams, which are valuable building blocks in organic synthesis and drug discovery. Further investigations of the reaction mechanism are currently underway.
Acknowledgements
We acknowledge financial support of Duke University and the National Science Foundation (CHE-1455220) to this work.
Notes and references
-
(a)
P. Kirsch, Modern Fluoroorganic Chemistry: Synthesis Reactivity, Applications, Wiley-VCH, Weinheim, 2004 Search PubMed;
(b) K. Müller, C. Faeh and F. Diederich, Science, 2007, 317, 1881 CrossRef PubMed;
(c) J.-A. Ma and D. Cahard, Chem. Rev., 2008, 108, PR1 CrossRef CAS PubMed;
(d) D. O'Hagan, Chem. Soc. Rev., 2008, 37, 308 RSC;
(e) S. Purser, P. R. Moore, S. Swallow and V. Gouverneur, Chem. Soc. Rev., 2008, 37, 320 RSC;
(f) K. Uneyama, T. Katagiri and H. Amii, Acc. Chem. Res., 2008, 41, 817 CrossRef CAS PubMed;
(g) W. K. Hagmann, J. Med. Chem., 2008, 51, 4359 CrossRef CAS PubMed;
(h)
I. Ojima, Fluorine in Medicinal Chemistry and Chemical Biology, Wiley-Blackwell, Chichester, UK, 2009 Search PubMed;
(i) S. Lectard, Y. Hamashima and M. Sodeoka, Adv. Synth. Catal., 2010, 352, 2708 CrossRef CAS;
(j) T. Furuya, A. S. Kamlet and T. Ritter, Nature, 2011, 473, 470 CrossRef CAS PubMed;
(k) T. Liang, C. N. Neumann and T. Ritter, Angew. Chem., Int. Ed., 2013, 52, 8214 CrossRef CAS PubMed.
- For reviews on trifluoromethylation reactions, see:
(a) T. Umemoto, Chem. Rev., 1996, 96, 1757 CrossRef CAS PubMed;
(b) S. G. K. Prakash and A. K. Yudin, Chem. Rev., 1997, 97, 757 CrossRef PubMed;
(c) M. Shimizu and T. Hiyama, Angew. Chem., Int. Ed., 2005, 44, 214 CrossRef CAS PubMed;
(d) M. Schlosser, Angew. Chem., Int. Ed., 2006, 45, 5432 CrossRef CAS PubMed;
(e) J.-A. Ma and D. Cahard, J. Fluorine Chem., 2007, 128, 975 CrossRef CAS;
(f) Y. Zheng and J.-A. Ma, Adv. Synth. Catal., 2010, 352, 2745 CrossRef CAS;
(g) S. Roy, B. T. Gregg, G. W. Gribble, V.-D. Le and S. Roy, Tetrahedron, 2011, 67, 2161 CrossRef CAS;
(h) O. A. Tomashenko and V. V. Grushin, Chem. Rev., 2011, 111, 4475 CrossRef CAS PubMed;
(i) X.-F. Wu, H. Neumann and M. Beller, Chem. – Asian J., 2012, 7, 1744 CrossRef CAS PubMed;
(j) A. Studer, Angew. Chem., Int. Ed., 2012, 51, 8950 CrossRef CAS PubMed;
(k) Y. Ye and M. S. Sanford, Synlett, 2012, 2005 CAS.
- For recent reviews, see:
(a) Z. Jin, G. B. Hammond and B. Xu, Aldrichimica Acta, 2012, 45, 67 CAS;
(b) P. Chen and G. Liu, Synthesis, 2013, 2919 CAS.
- Recent selected examples on trifluoromethylation of aromatic compounds:
(a) M. Oishi, H. Kondo and H. Amii, Chem. Commun., 2009, 1909 RSC;
(b) X. Wang, L. Truesdale and J.-Q. Yu, J. Am. Chem. Soc., 2010, 132, 3648 CrossRef CAS PubMed;
(c) E. J. Cho, T. D. Cenecal, T. Kinzel, Y. Zhang, D. A. Watson and S. L. Buchwald, Science, 2010, 328, 1679 CrossRef CAS PubMed;
(d) R. Shimizu, H. Egami, T. Nagi, J. Chae, Y. Hamashima and M. Sodeoka, Tetrahedron Lett., 2010, 51, 5947 CrossRef CAS;
(e) M. S. Wiehn, E. V. Vinogradova and A. Togni, J. Fluorine Chem., 2010, 131, 951 CrossRef CAS;
(f) L. Chu and F.-L. Qing, Org. Lett., 2010, 12, 5060 CrossRef CAS PubMed;
(g) T. D. Senecal, A. T. Parson and S. L. Buchwald, J. Org. Chem., 2011, 76, 1174 CrossRef CAS PubMed;
(h) H. Morimoto, T. Tsubogo, N. D. Litvinas and J. F. Hartwig, Angew. Chem., Int. Ed., 2011, 50, 3793 CrossRef CAS PubMed;
(i) T. Knauber, F. Arikan, G.-V. Röschenthaler and L. J. Gooßen, Chem. – Eur. J., 2011, 17, 2689 CrossRef CAS PubMed;
(j) J. Xu, D.-F. Luo, B. Xiao, Z.-J. Liu, T.-J. Gong, Y. Fu and L. Liu, Chem. Commun., 2011, 47, 4300 RSC;
(k) T. Liu and Q. Shen, Org. Lett., 2011, 13, 2342 CrossRef CAS PubMed;
(l) X. Mu, S. Chen, X. Zhen and G. Liu, Chem. – Eur. J., 2011, 17, 6039 CrossRef CAS PubMed;
(m) D. A. Nagib and D. W. C. MacMillan, Nature, 2011, 480, 224 CrossRef CAS PubMed;
(n) Z. Weng, R. Lee, W. Jia, Y. Yuan, W. Wang, X. Feng and K.-W. Huang, Organometallics, 2011, 30, 3229 CrossRef CAS;
(o) A. T. Parsons, T. D. Senecal and S. L. Buchwald, Angew. Chem., Int. Ed., 2012, 51, 2947 CrossRef CAS PubMed;
(p) Z. He, T. Luo, M. Hu, Y. Cao and J. Hu, Angew. Chem., Int. Ed., 2012, 51, 3944 CrossRef CAS PubMed;
(q) L. Chu and F.-L. Qing, J. Am. Chem. Soc., 2012, 134, 1298 CrossRef CAS PubMed;
(r) Y. Ye and M. S. Sanford, J. Am. Chem. Soc., 2012, 134, 9034 CrossRef CAS PubMed;
(s) X.-G. Zhang, H.-X. Dai, M. Wasa and J.-Q. Yu, J. Am. Chem. Soc., 2012, 134, 11948 CrossRef CAS PubMed.
-
(a) A. T. Parsons and S. L. Buchwald, Angew. Chem., Int. Ed., 2011, 50, 9120 CrossRef CAS PubMed;
(b) J. Xu, Y. Fu, D. Luo, Y. Jiang, B. Xiao, Z. Liu, T. Gong and L. Liu, J. Am. Chem. Soc., 2011, 133, 15300 CrossRef CAS PubMed;
(c) X. Wang, Y. Ye, S. Zhang, J. Feng, Y. Xu, Y. Zhang and J. Wang, J. Am. Chem. Soc., 2011, 133, 16410 CrossRef CAS PubMed;
(d) R. Shimizu, H. Egami, Y. Hamashima and M. Sodeoka, Angew. Chem., Int. Ed., 2012, 51, 4577 CrossRef CAS PubMed;
(e) L. Chu and F. Qing, Org. Lett., 2012, 14, 2106 CrossRef CAS PubMed;
(f) S. Mizuta, O. Galicia-López, K. M. Engle, S. Verhoog, K. Wheelhouse, G. Rassias and V. Gouverneur, Chem. – Eur. J., 2012, 18, 8583 CrossRef CAS PubMed;
(g) S. Mizuta, K. M. Engle, S. Verhoog, O. Galicia-López, M. O'Duill, M. Médebielle, K. Wheelhouse, G. Rassias, A. L. Thompson and V. Gouverneur, Org. Lett., 2013, 15, 1250 CrossRef CAS PubMed.
- For review, see: H. Egami and M. Sodeoka, Angew. Chem., Int. Ed., 2014, 53, 8294 CrossRef CAS PubMed.
- Examples of hydrotrifluoromethylation of alkenes:
(a) X. Wu, L. Chu and F. Qing, Angew. Chem., Int. Ed., 2013, 52, 2198 CrossRef CAS PubMed;
(b) S. Mizuta, S. Verhoog, K. M. Engle, T. Khotavivattana, M. O'Duill, K. Wheelhouse, G. Rassias, M. Médebielle and V. Gouverneur, J. Am. Chem. Soc., 2013, 135, 2505 CrossRef CAS PubMed.
-
(a) X. Mu, T. Wu, H.-Y. Wang, Y.-L. Guo and G. Liu, J. Am. Chem. Soc., 2012, 134, 878 CrossRef CAS PubMed;
(b) H. Egami, R. Shimizu, S. Kawamura and M. Sodeoka, Angew. Chem., Int. Ed., 2013, 52, 4000 CrossRef CAS PubMed;
(c) H. Egami, R. Shimizu and M. Sodeoka, J. Fluorine Chem., 2013, 152, 51 CrossRef CAS;
(d) X. Dong, R. Sang, Q. Wang, X.-Y. Tang and M. Shi, Chem. – Eur. J., 2013, 19, 16910 CrossRef CAS PubMed;
(e) P. Xu, J. Xie, Q. Xue, C. Pan, Y. Cheng and C. Zhu, Chem. – Eur. J., 2013, 19, 14039 CrossRef CAS PubMed;
(f) P. Gao, X.-B. Yan, T. Tao, F. Yang, T. He, X.-R. Song, X.-Y. Liu and Y.-M. Liang, Chem. – Eur. J., 2013, 19, 14420 CrossRef CAS PubMed;
(g) H. Egami, R. Shimizu, Y. Usui and M. Sodeoka, Chem. Commun., 2013, 49, 7346 RSC;
(h) W. Kong, M. Casimiro, E. Merino and C. Nevado, J. Am. Chem. Soc., 2013, 135, 14480 CrossRef CAS PubMed;
(i) N. O. Ilchenko, P. G. Janson and K. J. Szabó, J. Org. Chem., 2013, 78, 11087 CrossRef CAS PubMed;
(j) X. Liu, F. Xiong, X. Huang, L. Xu, P. Li and X. Wu, Angew. Chem., Int. Ed., 2013, 52, 6962 CrossRef CAS PubMed;
(k) Z.-M. Chen, W. Bai, S.-H. Wang, B.-M. Yang, Y.-Q. Tu and F.-M. Zhang, Angew. Chem., Int. Ed., 2013, 52, 9781 CrossRef CAS PubMed;
(l) F. Yang, P. Klumphu, Y.-M. Liang and B. H. Lipshutz, Chem. Commun., 2014, 50, 936 RSC;
(m) Y.-T. He, L.-H. Li, Y.-F. Yang, Z.-Z. Zhou, H.-L. Hua, X.-Y. Liu and Y.-M. Liang, Org. Lett., 2014, 16, 270 CrossRef CAS PubMed.
-
(a) P. G. Janson, I. Ghoneim, N. O. Ilchenko and K. J. Szabó, Org. Lett., 2012, 14, 2882 CrossRef CAS PubMed;
(b) Y. Li and A. Studer, Angew. Chem., Int. Ed., 2012, 51, 8221 CrossRef CAS PubMed;
(c) Y. Yasu, T. Koike and M. Akita, Angew. Chem., Int. Ed., 2012, 51, 9567 CrossRef CAS PubMed;
(d) R. Zhu and S. L. Buchwald, J. Am. Chem. Soc., 2012, 134, 12462 CrossRef CAS PubMed;
(e) R. Zhu and S. L. Buchwald, Angew. Chem., Int. Ed., 2013, 52, 12655 CrossRef CAS PubMed;
(f) Y.-T. He, L.-H. Li, Y.-F. Yang, Y.-Q. Wang, J.-Y. Luo, X.-Y. Liu and Y.-M. Liang, Chem. Commun., 2013, 49, 5687 RSC;
(g) Q. Yu and S. Ma, Chem. – Eur. J., 2013, 19, 13304 CrossRef CAS PubMed;
(h) D.-F. Lu, C.-L. Zhu and H. Xu, Chem. Sci., 2013, 4, 2478 RSC;
(i) X.-Y. Jiang and F.-L. Qing, Angew. Chem., Int. Ed., 2013, 52, 14177 CrossRef CAS PubMed.
-
(a) E. Kim, S. Choi, H. Kim and E. J. Cho, Chem. – Eur. J., 2013, 19, 6209 CrossRef CAS PubMed;
(b) Y. Yasu, T. Koike and M. Akita, Org. Lett., 2013, 15, 2136 CrossRef CAS PubMed;
(c) H. Egami, S. Kawamura, A. Miyazaki and M. Sodeoka, Angew. Chem., Int. Ed., 2013, 52, 7841 CrossRef CAS PubMed;
(d) J.-S. Lin, Y.-P. Xiong, C.-L. Ma, L.-J. Zhao, B. Tan and X.-Y. Liu, Chem. – Eur. J., 2014, 20, 1332 CrossRef CAS PubMed;
(e) J.-S. Lin, X.-G. Liu, X.-L. Zhu, B. Tan and X.-Y. Liu, J. Org. Chem., 2014, 79, 7084 CrossRef CAS PubMed.
- Recent reviews on lactams:
(a)
T. Janecki, Natural Lactones and Lactams: Synthesis, Occurrence and Biological Activity, Wiley-VCH, Weinheim, 2013 Search PubMed;
(b) L.-W. Ye, C. Shu and F. Gagosz, Org. Biomol. Chem., 2014, 12, 1833 RSC and references cited therein.
-
(a) K. Shen and Q. Wang, Chem. Sci., 2015, 6, 4279 RSC. Other examples using N-methoxyamides as substrates, see:
(b) D. J. Wardrop, E. G. Bowen, R. E. Forslund, A. D. Sussman and S. L. Weerasekera, J. Am. Chem. Soc., 2010, 132, 1188 CrossRef CAS PubMed;
(c) G. Yang, C. Shen and W. Zhang, Angew. Chem., Int. Ed., 2011, 51, 9141 CrossRef PubMed;
(d) Z.-Q. Zhang and F. Liu, Org. Biomol. Chem., 2015, 13, 6690 RSC.
-
(a) H. Egami, R. Shimizu and M. Sodeoka, Tetrahedron Lett., 2012, 53, 5503 CrossRef CAS;
(b) X.-P. Wang, J.-H. Lin, C.-P. Zhang, J.-C. Xiao and X. Zheng, Beilstein J. Org. Chem., 2013, 9, 2635 CrossRef PubMed;
(c) C. Feng and T.-P. Loh, Chem. Sci., 2012, 3, 3458 RSC;
(d) C. Feng and T.-P. Loh, Angew. Chem., Int. Ed., 2013, 52, 12414 CrossRef CAS PubMed.
- The observed O-trapping pathway may also arise from the different electronic nature of the 1,1-disubstituted alkenes.
- The formation of 3e was decreased significantly (12% 1H NMR yield) with the addition of TEMPO under the optimized reaction conditions, indicating the involvement of radical intermediates.
.
Footnote |
† Electronic supplementary information (ESI) available. See DOI: 10.1039/c5qo00353a |
|
This journal is © the Partner Organisations 2016 |