DOI:
10.1039/C5QO00374A
(Research Article)
Org. Chem. Front., 2016,
3, 217-221
Double carboxylation of o-alkynyl acetophenone with carbon dioxide†
Received
16th November 2015
, Accepted 6th December 2015
First published on 7th December 2015
Abstract
A copper-catalyzed double carboxylation of o-alkynyl acetophenone using carbon dioxide to afford 1(3H)-isobenzofuranylidene dicarboxylic esters in good yields is described. The reaction proceeds via a carboxylation/intramolecular cyclization/carboxylation sequence. Alkyl-substituted substrates show much higher double carboxylation product selectivities than aryl-substituted substrates.
The utilization of carbon dioxide in organic synthesis has gained significant attention since it provides the opportunity to convert this greenhouse gas into value-added fine chemicals.1 In the last few decades, various methodologies using carbon dioxide as a carboxylative reagent have been developed, offering attractive and powerful access to synthetically important carboxylic acids and derivatives.2–9 Generally, one single carbon dioxide molecule is incorporated into a product in these carboxylation reactions. Double carboxylation of a substrate using carbon dioxide is rarely reported, and electrochemical conditions,10 stoichiometric metal complexes11 or specific substrates12 are used to enable the second carbon dioxide insertion. Most recently, Tsuji et al. disclosed a nickel-catalyzed reductive double carboxylation of internal alkynes with carbon dioxide to produce maleic anhydrides.13 Herein, we describe a CuI-catalyzed double carboxylation of o-alkynyl acetophenone using carbon dioxide to give 1(3H)-isobenzofuranylidene dicarboxylic esters in good yields.
Yamada14 and our group15 previously found a silver-catalyzed sequential carboxylation/intramolecular cyclization reaction of o-alkynyl acetophenone with carbon dioxide, in which β-ketocarboxylate coming from the α-carboxylation of the carbonyl group16 initiated a nucleophilic attack towards the silver(I)-activated alkyne moiety to produce the 1(3H)-isobenzofuranylidene acetate product (Scheme 1, a). It is noteworthy that a new metal–carbon bond might be formed during the above cyclization reaction.17 We envisioned that with a judicious choice of catalytic and reaction systems, the second carbon dioxide molecule would insert into this newly-formed metal–carbon bond,18 and thus a double carboxylation product would be obtained via a carboxylation/intramolecular cyclization/carboxylation sequence (Scheme 1, a). Indeed, Ma once reported a copper-catalyzed carboxylation of a vinylic metallic intermediate generated by cyclic anti-azametallation of 2-alkynylaniline using carbon dioxide in the presence of dimethylzinc and cesium fluoride.18a Encouraged by the above mechanistic and experimental investigations, we chose the model reaction of 2-(phenylethynyl)phenylethanone (1a) with carbon dioxide as used previously15 to identify the optimal catalytic system for the double carboxylation reaction.
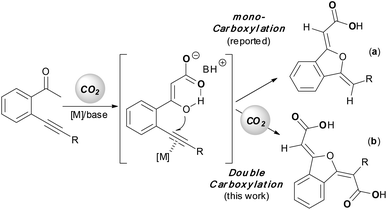 |
| Scheme 1 Reaction of o-alkynyl acetophenone with carbon dioxide. | |
As shown in Table 1, a combination of 10 mol% of CuI and 4 equiv. of DBU in DMF at 60 °C gave 86% yield of the mono-carboxylation product 3a, and no double carboxylation product 2a was observed (entry 1). When cesium carbonate was used as a base, although 3a was still the major product, 24% yield of 2a was isolated (entry 2). The structure of product 2a was determined unambiguously by single crystal X-ray diffraction (Fig. 1).19 The configuration of two double bonds in 2a was also confirmed by NOE studies. The reactions in DMAc and NMP also gave a much higher yield of 3a than that of 2a (entries 3 and 4). It's delightedly found that when DMSO was used as a solvent, 54% yield of double carboxylation product 2a was obtained as the major product (entry 5). A decrease in cesium carbonate's quantity from 4 equiv. to 2 equiv. obviously switches the product selectivity (entry 6). Cesium fluoride proved to be a less efficient base (entry 7), and potassium carbonate or tert-butoxide was not the suitable base for this reaction (entries 8 and 9). The reaction at a decreased temperature (25 °C) resulted in a low conversion and a lower yield of 2a was obtained (entry 10). Increasing the reaction temperature to 100 °C didn't enhance the yield of 2a (entry 11). Both introduction of a nitrogen ligand to the catalytic system and the use of the N-heterocyclic carbene copper(I) complex led to the major formation of the mono-carboxylation product 3a (entries 12–14).
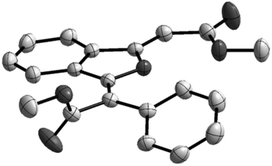 |
| Fig. 1 ORTEP plot of 2a shown with ellipsoids at the 30% probability level; hydrogen atoms were omitted for clarity. | |
Table 1 Reaction of 2-(phenylethynyl)phenylethanone with carbon dioxidea
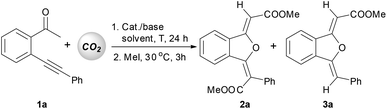
|
Entry |
Cat. (mol%) |
Base |
T/°C |
2a
/% |
3a
/% |
Reaction conditions: 1a (0.2 mmol), base (0.8 mmol), 2 mL DMSO as the solvent, 2.0 MPa of carbon dioxide, 24 h; then MeI (1.0 mmol), 30 °C, 3 h.
Isolated yield.
DMF as the solvent.
DMAc as the solvent.
NMP as the solvent.
2.0 equiv. cesium carbonate.
Cu(CH3CN)4PF6 as the catalyst.
0.1 MPa of carbon dioxide.
In the absence of carbon dioxide. L1: 1,10-phenanthroline. L2: 5-nitro-1,10-phenanthroline.
|
1c |
CuI (10) |
DBU |
60 |
— |
86 |
2c |
CuI (10) |
Cs2CO3 |
60 |
24 |
52 |
3d |
CuI (10) |
Cs2CO3 |
60 |
16 |
63 |
4e |
CuI (10) |
Cs2CO3 |
60 |
22 |
56 |
5 |
CuI (10) |
Cs2CO3 |
60 |
54 |
32 |
6f |
CuI (10) |
Cs2CO3 |
60 |
24 |
58 |
7 |
CuI (10) |
CsF |
60 |
33 |
10 |
8 |
CuI (10) |
K2CO3 |
60 |
— |
— |
9 |
CuI (10) |
KOtBu |
60 |
— |
— |
10 |
CuI (10) |
Cs2CO3 |
25 |
28 |
7 |
11 |
CuI (10) |
Cs2CO3 |
100 |
54 |
28 |
12 |
CuI + L1 (10) |
Cs2CO3 |
60 |
24 |
74 |
13 |
CuI + L2 (10) |
Cs2CO3 |
60 |
22 |
68 |
14 |
IPrCuCl (10) |
Cs2CO3 |
60 |
40 |
59 |
15 |
CuBr (10) |
Cs2CO3 |
60 |
33 |
45 |
16 |
CuCl (10) |
Cs2CO3 |
60 |
37 |
55 |
17g |
CuPF6 (10) |
Cs2CO3 |
60 |
28 |
43 |
18 |
CuCl2 (10) |
Cs2CO3 |
60 |
41 |
28 |
19 |
PdCl2 (10) |
Cs2CO3 |
60 |
37 |
11 |
20 |
AgI (10) |
Cs2CO3 |
60 |
19 |
59 |
21 |
CuI (2) |
Cs2CO3 |
60 |
51 |
35 |
22h |
CuI (10) |
Cs2CO3 |
60 |
36 |
39 |
23 |
— |
Cs2CO3 |
60 |
16 |
3 |
24 |
CuI (10) |
— |
60 |
— |
— |
25i |
CuI (10) |
Cs2CO3 |
60 |
— |
— |
Other copper(I) salts such as CuBr, CuCl, and Cu(CH3CN)4PF6 show inferior double product selectivities than CuI (entries 15–17). CuCl2 and PdCl2 can also catalyze the reaction to afford the major double carboxylation product (entries 18 and 19). The silver(I) catalyst previously used in our mono-carboxylation reaction15 shows a very low double carboxylation product selectivity (entry 20). When the catalyst loading decreased from 10 mol% to 2 mol%, a slightly lower yield of 2a was formed (entry 21). The product selectivity is sensitive to CO2 pressure and the reaction using atmospheric carbon dioxide gives both 36% yield of 2a and 39% yield of 3a (entry 22). The reaction in the absence of CuI proceeds very slowly, which clearly demonstrates the catalytic role of the copper salt (entry 23). The reaction without a base provides no product, which implies the essential role of the base to deprotonate the α-H of the carbonyl group and then trigger the following carboxylation reaction (entry 24). No carboxylation product was detected when the reaction was carried out in the absence of carbon dioxide, showing that the CO2 moiety in the carboxylation product comes from carbon dioxide, not from cesium carbonate (entry 25).
The substrate scope of the copper-catalyzed double carboxylation of o-alkynyl acetophenones was then investigated using 10 mol% CuI as the catalyst, 4 equiv. of cesium carbonate as the base in DMSO at 60 °C (Table 2). A variety of aryl-substituted o-alkynyl acetophenones conducted the double carboxylation reaction smoothly to give 1(3H)-isobenzofuranylidene dicarboxylic esters in moderate to good yields (2a–2g). The electron-deficient phenylethynyl substrates (1e, 1f) show higher double carboxylation product selectivities than the electron-rich phenylethynyl substrates (1b–1d, 1g).
Table 2 Substrate scopea,b
Reaction conditions: 1 (0.2 mmol), CuI (0.02 mmol), Cs2CO3 (0.8 mmol), 2 mL DMSO as the solvent, 2.0 MPa of carbon dioxide, 60 °C, 24 h; then MeI (1.0 mmol), 30 °C, 3 h.
Isolated yields (2/3) are given.
Compound 4 was isolated as the main product.
1q was used as a substrate.
|
|
Alkyl-substituted o-alkynyl acetophenones show much higher double carboxylation product selectivities than aryl-substituted substrates. When 1h was used as the substrate, 91% yield of the double carboxylation product 2h was isolated and only a trace mono-carboxylation product 3h was observed in mass spectroscopy. The steric factor has a profound effect on the product selectivity, and the bulky substitutes on the alkyne moiety lead to the decreased selectivity of the double carboxylation product (2k, 2m), presumably due to the hindrance of the insertion of the second carbon dioxide. When the tert-butyl substituted substrate 1n was used, only traces of carboxylative cyclization products were observed in mass spectroscopy. Instead, compound 4 was isolated as the major product. When 1q containing a chloride group to intramolecularly trap the carboxylate intermediate was used as a substrate, a lactone product 5 was formed exclusively in good yield.
Although the detailed mechanism is not clear, a possible pathway (Scheme 2) could initially involve a base-promoted carboxylation of o-alkynyl acetophenone with carbon dioxide.14–16 Then the copper(I)-catalyzed intramolecular cyclization would afford a vinylic copper intermediate A.18 The following insertion of the second carbon dioxide into the newly-formed carbon–copper bond would finally furnish the double carboxylation product 2. Meanwhile, the competitive protonation of the vinylic copper intermediate would irreversibly give the mono-carboxylation product 3. When the standard reaction was carried out in the presence of 1 equiv. of D2O, the yields of the 2a/3a evidently changed from 54/28% to 26/45% (Scheme 3, a). The mono-carboxylation product 3a′ cannot convert into the double carboxylation product under the standard reaction conditions (Scheme 3, b). When a mixture of 2a′ and 3a′ (molar ratio: 2a′/3a′ = 3.8
:
1) were subjected to the standard catalytic system, a decreased molar ratio of 2a/3a was obtained (Scheme 3, c), which demonstrated that the second carboxylation might be reversible. Therefore, the relatively high carbon dioxide pressure and large excess of the base are beneficial to the formation of the double carboxylation product.
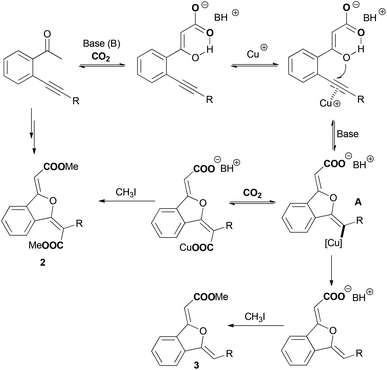 |
| Scheme 2 Possible mechanism. | |
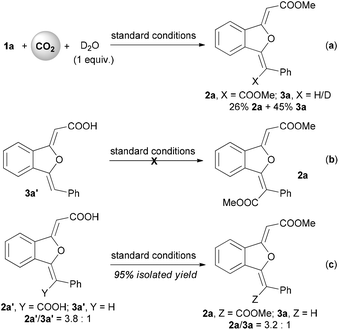 |
| Scheme 3 Control experiments. Standard conditions: CuI (10 mol%), Cs2CO3 (4 equiv.), DMSO (0.1 M), 2.0 MPa of carbon dioxide, 60 °C, 24 h; then MeI (5 equiv.), 30 °C, 3 h. | |
In summary, we have developed a copper-catalyzed double carboxylation of o-alkynyl acetophenone using carbon dioxide as a carboxylative reagent to give 1(3H)-isobenzofuranylidene dicarboxylic esters in moderate to good yields. This sequential reaction is triggered by α-carboxylation of the carbonyl group with carbon dioxide and proceeds via a carboxylation/intramolecular cyclization/second carboxylation sequence. The substitute in the alkyne moiety has a profound effect on the reaction product and alkyl-substituted substrates show much higher double carboxylation product selectivities than aryl-substituted substrates. Further mechanistic studies are currently under investigation in our laboratory.
Acknowledgements
This work was supported by the National Natural Science Foundation of China (21172026), the Fundamental Research Funds for the Central Universities (DUT15LAB21), and the Program for Changjiang Scholars and Innovative Research Team in University (IRT13008). X.-B. Lu gratefully acknowledges the Chang Jiang Scholars Program (no. T2011056) from Ministry of Education, People's Republic of China.
Notes and references
- For reviews, see:
(a) J. Louie, Curr. Org. Chem., 2005, 9, 605 CrossRef CAS;
(b) M. Mori, Eur. J. Org. Chem., 2007, 4981 CrossRef CAS;
(c) T. Sakakura, J.-C. Choi and H. Yasuda, Chem. Rev., 2007, 107, 2365 CrossRef CAS PubMed;
(d) A. Correa and R. Martin, Angew. Chem., Int. Ed., 2009, 48, 6201 CrossRef CAS PubMed;
(e) S. N. Riduan and Y. Zhang, Dalton Trans., 2010, 39, 3347 RSC;
(f) K. Huang, C.-L. Sun and Z.-J. Shi, Chem. Soc. Rev., 2011, 40, 2435 RSC;
(g) M. Cokoja, C. Bruckmeier, B. Rieger, W. A. Herrmann and F. E. Kuhn, Angew. Chem., Int. Ed., 2011, 50, 8510 CrossRef CAS PubMed;
(h) W.-Z. Zhang and X.-B. Lu, Chin. J. Catal., 2012, 33, 745 CrossRef CAS;
(i) Y. Tsuji and T. Fujihara, Chem. Commun., 2012, 48, 9956 RSC;
(j) L. Zhang and Z. Hou, Chem. Sci., 2013, 4, 3395 RSC;
(k) N. Kielland, C. J. Whiteoak and A. W. Kleij, Adv. Synth. Catal., 2013, 355, 2115 CrossRef CAS;
(l) X. Cai and B. Xie, Synthesis, 2013, 3305 CrossRef CAS;
(m) M. Aresta, A. Dibenedetto and A. Angelini, Chem. Rev., 2014, 114, 1709 CrossRef CAS PubMed;
(n) B. Yu and L.-N. He, ChemSusChem, 2015, 8, 52 CrossRef CAS PubMed;
(o) Q. Liu, L. Wu, R. Jackstell and M. Beller, Nat. Commun., 2015, 6, 5933, DOI:10.1038/ncomms6933.
- For the carboxylation of organotin reagents with CO2, see:
(a) M. Shi and K. M. Nicholas, J. Am. Chem. Soc., 1997, 119, 5057 CrossRef CAS;
(b) R. J. Franks and K. M. Nicholas, Organometallics, 2000, 19, 1458 CrossRef CAS;
(c) R. Johansson and O. F. Wendt, Dalton Trans., 2007, 488 RSC;
(d) J.-G. Wu and N. Hazari, Chem. Commun., 2011, 47, 1069 RSC;
(e) T. Mita, J. Chen, M. Sugawara and Y. Sato, Angew. Chem., Int. Ed., 2011, 50, 1393 CrossRef CAS PubMed;
(f) X. Feng, A. Sun, S. Zhang, X. Yu and M. Bao, Org. Lett., 2013, 15, 108 CrossRef CAS PubMed.
- For the carboxylation of organoboron reagents with CO2, see:
(a) K. Ukai, M. Aoki, J. Takaya and N. Iwasawa, J. Am. Chem. Soc., 2006, 128, 8706 CrossRef CAS PubMed;
(b) J. Takaya, S. Tadami, K. Ukai and N. Iwasawa, Org. Lett., 2008, 10, 2697 CrossRef CAS PubMed;
(c) T. Ohishi, M. Nishiura and Z. Hou, Angew. Chem., Int. Ed., 2008, 47, 5792 CrossRef CAS PubMed;
(d) H. Ohmiya, M. Tanabe and M. Sawamura, Org. Lett., 2011, 13, 1086 CrossRef CAS PubMed;
(e) T. Ohishi, L. Zhang, M. Nishiura and Z. Hou, Angew. Chem., Int. Ed., 2011, 50, 8114 CrossRef CAS PubMed;
(f) X. Zhang, W.-Z. Zhang, L.-L. Shi, C.-X. Guo, L.-L. Zhang and X.-B. Lu, Chem. Commun., 2012, 48, 6292 RSC.
- For the carboxylation of organozinc reagents with CO2, see:
(a) H. Ochiai, M. Jang, K. Hirano, H. Yorimitsu and K. Oshima, Org. Lett., 2008, 10, 2681 CrossRef CAS PubMed;
(b) C. S. Yeung and V. M. Dong, J. Am. Chem. Soc., 2008, 130, 7826 CrossRef CAS PubMed;
(c) K. Kobayashi and Y. Kondo, Org. Lett., 2009, 11, 2035 CrossRef CAS PubMed.
- For the carboxylation of terminal alkynes with CO2, see:
(a) Y. Fukue, S. Oi and Y. Inoue, J. Chem. Soc., Chem. Commun., 1994, 2091 RSC;
(b) N. Eghbali, J. Eddy and P. T. Anastas, J. Org. Chem., 2008, 73, 6932 CrossRef CAS PubMed;
(c) L. J. Goossen, N. Rodriguez, F. Manjolinho and P. P. Lange, Adv. Synth. Catal., 2010, 352, 2913 CrossRef CAS;
(d) D. Yu and Y. Zhang, Proc. Natl. Acad. Sci. U. S. A., 2010, 107, 20184 CrossRef CAS PubMed;
(e) D. Yu and Y. Zhang, Green Chem., 2011, 13, 1275 RSC;
(f) W.-Z. Zhang, W.-J. Li, X. Zhang, H. Zhou and X.-B. Lu, Org. Lett., 2010, 21, 4748 CrossRef PubMed;
(g) X. Zhang, W.-Z. Zhang, X. Ren, L.-L. Zhang and X.-B. Lu, Org. Lett., 2011, 13, 2402 CrossRef CAS PubMed;
(h) M. Arndt, E. Risto, T. Krause and L. J. Goossen, ChemCatChem, 2012, 4, 484 CrossRef CAS;
(i) D. Yu, M. X. Tan and Y. Zhang, Adv. Synth. Catal., 2012, 354, 969 CrossRef CAS;
(j) K. Inamoto, N. Asano, K. Kobayashi, M. Yonemoto and Y. Kondo, Org. Biomol. Chem., 2012, 10, 1514 RSC;
(k) X. Zhang, W.-Z. Zhang, L.-L. Shi, C. Zhu, J.-L. Jiang and X.-B. Lu, Tetrahedron, 2012, 68, 9085 CrossRef CAS;
(l) F. Manjolinho, M. Arndt, K. Goossen and L. J. Goossen, ACS Catal., 2012, 2, 2014 CrossRef CAS.
- For the hydro-, sila- or bora-carboxylation of unsaturated compounds with CO2, see:
(a) J. Takaya and N. Iwasawa, J. Am. Chem. Soc., 2008, 130, 15254 CrossRef CAS PubMed;
(b) C. M. Williams, J. B. Johnson and T. Rovis, J. Am. Chem. Soc., 2008, 130, 14936 CrossRef CAS PubMed;
(c) S. Li, W. Yuan and S. Ma, Angew. Chem., Int. Ed., 2011, 50, 2578 CrossRef CAS PubMed;
(d) T. Fujihara, T. Xu, K. Semba, J. Terao and Y. Tsuji, Angew. Chem., Int. Ed., 2011, 50, 523 CrossRef CAS PubMed;
(e) M. North, Angew. Chem., Int. Ed., 2009, 48, 4104 CrossRef CAS PubMed;
(f) Y. Zhang and S. N. Riduan, Angew. Chem., Int. Ed., 2011, 50, 6210 CrossRef CAS PubMed;
(g) M. D. Greenhalgh and S. P. Thomas, J. Am. Chem. Soc., 2012, 134, 11900 CrossRef CAS PubMed;
(h) S. Li and S. Ma, Org. Lett., 2011, 13, 6046 CrossRef CAS PubMed;
(i) S. Li and S. Ma, Adv. Synth. Catal., 2012, 354, 2387 CrossRef CAS;
(j) L. Zhang, J. Cheng, B. Carry and Z. Hou, J. Am. Chem. Soc., 2012, 134, 14314 CrossRef CAS PubMed;
(k) T. Fujihara, Y. Tani, K. Semba, J. Terao and Y. Tsuji, Angew. Chem., Int. Ed., 2012, 51, 11487 CrossRef CAS PubMed;
(l) S. Li, B. Miao, W. Yuan and S. Ma, Org. Lett., 2013, 15, 977 CrossRef CAS PubMed;
(m) T. G. Ostapowicz, M. Schmitz, M. Krystof, J. Klankermayer and W. Leitner, Angew.
Chem., Int. Ed., 2013, 52, 12119 CAS;
(n) Y. Tani, T. Fujihara, J. Terao and Y. Tsuji, J. Am. Chem. Soc., 2014, 136, 17706 CrossRef CAS PubMed;
(o) X. Wang, M. Nakajima and R. Martin, J. Am. Chem. Soc., 2015, 137, 8924 CrossRef CAS PubMed.
- For the carboxylation of C–H bonds with CO2, see:
(a) I. I. F. Boogaerts and S. P. Nolan, J. Am. Chem. Soc., 2010, 132, 8858 CrossRef CAS PubMed;
(b) L. Zhang, J. Cheng, T. Ohishi and Z. Hou, Angew. Chem., Int. Ed., 2010, 49, 8670 CrossRef CAS PubMed;
(c) I. I. F. Boogaerts, G. C. Fortman, M. R. L. Catherine and S. P. Nolan, Angew. Chem., Int. Ed., 2010, 49, 8674 CrossRef CAS PubMed;
(d) H. Mizuno, J. Takaya and N. Iwasawa, J. Am. Chem. Soc., 2011, 133, 1251 CrossRef CAS PubMed;
(e) D. M. Dalton and T. Rovis, Nat. Chem., 2010, 2, 710 CrossRef CAS PubMed;
(f) I. I. F. Boogaerts and S. P. Nolan, Chem. Commun., 2011, 47, 3021 RSC;
(g) K. Sasano, J. Takaya and N. Iwasawa, J. Am. Chem. Soc., 2013, 135, 10954 CrossRef CAS PubMed.
- For the direct carboxylation of electrophiles with CO2, see:
(a) A. Correa and R. Martin, J. Am. Chem. Soc., 2009, 131, 15974 CrossRef CAS PubMed;
(b) T. Fujihara, K. Nogi, T. Xu, J. Terao and Y. Tsuji, J. Am. Chem. Soc., 2012, 134, 9106 CrossRef CAS PubMed;
(c) T. Leon, A. Correa and R. Martin, J. Am. Chem. Soc., 2013, 135, 1221 CrossRef CAS PubMed;
(d) T. Moragas, J. Cornella and R. Martin, J. Am. Chem. Soc., 2014, 136, 17702 CrossRef CAS PubMed;
(e) X. Wang, Y. Liu and R. Martin, J. Am. Chem. Soc., 2015, 137, 6476 CrossRef CAS PubMed.
- For the transition-metal-free incorporation of CO2, see:
(a) H. Yoshida, H. Fukushima, J. Ohshita and A. Kunai, J. Am. Chem. Soc., 2006, 128, 11040 CrossRef CAS PubMed;
(b) H. Zhou, W.-Z. Zhang, C.-H. Liu, J.-P. Qu and X.-B. Lu, J. Org. Chem., 2008, 73, 8039 CrossRef CAS PubMed;
(c) S. N. Riduan, Y. Zhang and J. Y. Ying, Angew. Chem., Int. Ed., 2009, 48, 3322 CrossRef CAS PubMed;
(d) Y. Kayaki, M. Yamamoto and T. Ikariya, Angew. Chem., Int. Ed., 2009, 48, 4194 CrossRef CAS PubMed;
(e) G. Chen, C. Fu and S. Ma, Org. Lett., 2009, 11, 2900 CrossRef CAS PubMed;
(f) O. Vechorkin, N. Hirt and X. Hu, Org. Lett., 2010, 12, 3567 CrossRef CAS PubMed;
(g) S. Minakata, I. Sasaki and T. Ide, Angew. Chem., Int. Ed., 2010, 49, 1309 CrossRef CAS PubMed;
(h) G. Chen, C. Fu and S. Ma, Org. Biomol. Chem., 2011, 9, 105 RSC;
(i) C. Das Neves Gomes, O. Jacquet, C. Villiers, P. Thuery, M. Ephritikhine and T. Cantat, Angew. Chem., Int. Ed., 2012, 51, 187 CrossRef CAS PubMed;
(j) K. Inamoto, N. Asano, Y. Nakamura, M. Yonemoto and Y. Kondo, Org. Lett., 2012, 14, 2622 CrossRef CAS PubMed;
(k) W.-J. Yoo, M. G. Capdevila, X. Du and S. Kobayashi, Org. Lett., 2012, 14, 5326 CrossRef CAS PubMed;
(l) L.-L. Zhao, S.-Y. Wang, X.-P. Xu and S.-J. Ji, Chem. Commun., 2013, 49, 2569 RSC;
(m) Y.-B. Wang, Y.-M. Wang, W.-Z. Zhang and X.-B. Lu, J. Am. Chem. Soc., 2013, 135, 11996 CrossRef CAS PubMed;
(n) W. Xiong, C. Qi, H. He, L. Ouyang, M. Zhang and H. Jiang, Angew. Chem., Int. Ed., 2015, 54, 3084 CrossRef CAS PubMed;
(o) Z. Xin, C. Lescot, S. D. Friis, K. Daasbjerg and T. Skrydstrup, Angew. Chem., Int. Ed., 2015, 54, 6862 CrossRef CAS PubMed;
(p) W.-Z. Zhang, T. Xia, X.-T. Yang and X.-B. Lu, Chem. Commun., 2015, 51, 6175 RSC.
-
(a) S. Derien, J.-C. Clinet, E. Dunach and J. Perichon, Tetrahedron, 1992, 48, 5235 CrossRef CAS;
(b) H. Senboku, H. Komatsu, Y. Fujimura and M. Tokuda, Synlett, 2001, 418 CAS;
(c) G.-Q. Yuan, H.-F. Jiang and C. Lin, Tetrahedron, 2008, 64, 5866 CrossRef CAS.
- M. Takimoto and M. Mori, J. Am. Chem. Soc., 2001, 123, 2895 CrossRef CAS PubMed.
- M. Takimoto, M. Kawamura, M. Mori and Y. Sato, Synlett, 2005, 2019 CAS.
- T. Fujihara, Y. Horimoto, T. Mizoe, F. B. Sayyed, Y. Tani, J. Terao, S. Sakaki and Y. Tsuji, Org. Lett., 2014, 16, 4960 CrossRef CAS PubMed.
- K. Sekine, A. Takayanagi, S. Kikuchi and T. Yamada, Chem. Commun., 2013, 49, 11320 RSC.
- W.-Z. Zhang, L.-L. Shi, C. Liu, X.-T. Yang, Y.-B. Wang, Y. Luo and X.-B. Lu, Org. Chem. Front., 2014, 1, 275 RSC.
- For the α-carboxylation of carbonyl compounds with CO2, see:
(a) E. J. Corey and R. H. K. Chen, J. Org. Chem., 1973, 38, 4086 CrossRef CAS;
(b) E. Haruki, M. Arakawa, N. Matsumura, Y. Otsuji and E. Imoto, Chem. Lett., 1974, 427 CrossRef CAS;
(c) H. Sakurai, A. Shirahata and A. Hosomi, Tetrahedron Lett., 1980, 21, 1967 CrossRef CAS;
(d) Y. Hirai, T. Aida and S. Inoue, J. Am. Chem. Soc., 1989, 111, 3062 CrossRef CAS;
(e) K. Chiba, H. Tagaya, S. Miura and M. Karasu, Chem. Lett., 1992, 923 CrossRef CAS;
(f) B. J. Flowers, R. Gautreau-Service and P. G. Jessop, Adv. Synth. Catal., 2008, 350, 2947 CrossRef CAS;
(g) E. J. Beckmana and P. Munshi, Green Chem., 2011, 13, 376 RSC;
(h) B. R. Van Ausdall, N. F. Poth, V. A. Kincaid, A. M. Arif and J. Louie, J. Org. Chem., 2011, 76, 8413 CrossRef CAS PubMed;
(i) K. Sekine, T. Ishida and T. Yamada, Angew. Chem., Int. Ed., 2012, 51, 6989 CrossRef PubMed.
- For reviews, see:
(a) S. Ma, Acc. Chem. Res., 2003, 36, 701 CrossRef CAS PubMed;
(b) G. Zeni and R. C. Larock, Chem. Rev., 2004, 104, 2285 CrossRef CAS PubMed;
(c) F. Alonso, I. P. Beletskaya and M. Yus, Chem. Rev., 2004, 104, 3079 CrossRef CAS PubMed;
(d) J.-M. Weibel, A. L. Blanc and P. Pale, Chem. Rev., 2008, 108, 3149 CrossRef CAS PubMed;
(e) M. Alvarez-Corral, M. Munoz-Dorado and I. Rodriguez-Garcia, Chem. Rev., 2008, 108, 3174 CrossRef CAS PubMed;
(f) N. T. Patil and Y. Yamamoto, Chem. Rev., 2008, 108, 3395 CrossRef CAS PubMed;
(g) P. Belmont and E. Parker, Eur. J. Org. Chem., 2009, 6075 CrossRef CAS;
(h) C. Aubert, L. Fensterbank, P. Garcia, M. Malacria and A. Simonneau, Chem. Rev., 2011, 111, 1954 CrossRef CAS PubMed;
(i) A. V. Gulevich, A. S. Dudnik, N. Chernyak and V. Gevorgyan, Chem. Rev., 2013, 113, 3084 CrossRef CAS PubMed.
-
(a) S. Li and S. Ma, Adv. Synth. Catal., 2012, 354, 2387 CrossRef CAS;
(b) K. Inamoto, N. Asano, Y. Nakamura, M. Yonemoto and Y. Kondo, Org. Lett., 2012, 14, 2622 CrossRef CAS PubMed;
(c) W.-J. Yoo, T. V. Q. Nguyen and S. Kobayashi, Angew. Chem., Int. Ed., 2014, 53, 10213 CrossRef CAS PubMed.
- CCDC 1406654 (2a).
Footnote |
† Electronic supplementary information (ESI) available: Experimental details and data. CCDC 1406654. For ESI and crystallographic data in CIF or other electronic format see DOI: 10.1039/c5qo00374a |
|
This journal is © the Partner Organisations 2016 |
Click here to see how this site uses Cookies. View our privacy policy here.