DOI:
10.1039/C6RA16802G
(Communication)
RSC Adv., 2016,
6, 75499-75504
High-throughput metabolomics analysis discovers salivary biomarkers for predicting mild cognitive impairment and Alzheimer's disease†
Received
29th June 2016
, Accepted 29th July 2016
First published on 1st August 2016
Abstract
Mild cognitive impairment (MCI) confers an increased risk of developing Alzheimer's disease (AD). There is high interest in the discovery of early diagnostic biomarkers that could predict MCI to AD progression, for which saliva metabolomics exhibits a great potential. In this work, the nontargeted metabolomic approach based on fast ultra-high performance liquid chromatography coupled with time-of-flight mass spectrometry (FUPLC-MS) was developed to examine metabolic differences in saliva samples from MCI subjects and age-matched AD subjects; multivariate analyses were used to define the differences between MCI and AD groups; and receiver operating characteristic analysis was used to evaluate the diagnostic power of the candidate biomarkers. Metabolic differences among AD and MCI subjects were identified by principal component analysis. Of note, ten metabolites in the saliva of AD subjects were significantly different from MCI subjects. According to the predictive model, five metabolites were selected as the candidate biomarkers for predicting conversion of MCI to AD. The major contributors were cytidine (P = 0.0003) and sphinganine-1-phosphate (P = 0.0009). The results demonstrated that saliva metabolite profiling may contribute to making early diagnosis and understanding the pathogenic mechanism of AD and MCI.
Introduction
Alzheimer's disease (AD) is the main cause of gradual cognitive impairment in elderly individuals.1 It is a growing challenge to the health care systems and economies of developed and developing countries.2,3 Mild cognitive impairment (MCI) is an intermediate state between healthy states and AD, and has an increased risk of developing AD.4 However, biomarker discovery for the accurate diagnosis of AD remains one of the urgent research initiatives. In AD research, prediction of the progression from MCI to AD is also of major interest. Recent research has concentrated on obtaining biomarkers to identify features that differentiate between those MCI subjects who will develop AD.5–9
Biomarkers are needed for early and accurate diagnosis, for prediction of conversion from pre-clinical MCI and for monitoring AD progression. Many diseases disrupt metabolism and result in changes that can be captured as metabolic signatures. Metabolomics is the systematic study of low molecular weight molecules and has become a powerful approach to understand the pathophysiological state.10–13 Metabolic signatures have been reported for several central nervous system disorders, including AD.14,15 Salivary metabolomics is an emerging and promising field that offers unique perspective on the use of oral fluids in molecular diagnostics.16 In this study, we used fast ultra-high performance liquid chromatography coupled with time-of-flight mass spectrometry (FUPLC-MS) to establish metabolomics method to obtain the low molecular weight metabolites in saliva samples from AD and MCI participants.
Experimental
Chemicals
Distilled water was purified using a Milli-Q system (Millipore, Billerica, USA). HPLC grade acetonitrile and methanol were provided by Fisher (USA). Formic acid (HPLC grade), lactic acid and carnitine were provided by J&K Chemical Ltd (Beijing, China). Leucine enkephalin was provided by Sigma-Aldrich (St. Louis, MO, USA).
Ethics statement
The saliva samples were conducted according to the Declaration of Helsinki and approved by the ethics committee of Heilongjiang University of Chinese Medicine (approval number: HUCM-2014-12619). All patients gave informed written consent.
Subjects
The AD patients and MCI patients were all recruited from the First Affiliated Hospital, Heilongjiang University of Chinese Medicine. Cases were classified as MCI (MCI-AD group) and AD (AD group). The detailed clinical characteristics of the attended subjects are provided in ESI Table 1.†
Saliva collection and preparation
Prior to samples collection, all the patients were asked to rinse their mouth thoroughly with water. The samples were collected between 9:00 and 11:00 a.m. The collected samples were centrifuged at 9000g for 20 min at 4 °C, and the supernatant were transferred to fresh tubes and frozen at −80 °C until analysis. The acetonitrile/methanol (75
:
25 v/v, 400 μL) was added to saliva to precipitating proteins. The mixture was then centrifuged at 9000 g for 20 min at 4 °C and filtered through 0.22 μm syringe filter until the FUPLC-MS analysis.
FUPLC-MS analysis technology
Chromatography separation of saliva samples was performed on an ACQUITY UPLC™ BEH C18 column (50 mm × 2.1 mm i.d., 1.7 μm, Waters Co., USA) that was maintained at 40 °C. The injected sample volume was 2 μL for each run. The gradient elution was performed with 0.1% formic acid–water (A), acetonitrile (B). It was started as follows: 0–2.0 min, 1–15% A; 2.0–5.0 min, 15–25% A; 5.0–7.0 min, 25–50% A; 7.0–8.5 min, 50–95% A; 8.5–10 min, 95% A, 10–14 min, 1% A. Mass spectrometry (MS) was performed by faster TOF/MS (time of flight mass spectrometer) equipped with an negative ion source (Waters, Milford, USA), the scan range was from m/z 50 to 1500. The source temperature was set at 120 °C, and desolvation gas temperature was set at 400 °C. The flow rates of desolvation gas and cone were set at 500 L h−1 and 80 L h−1, respectively. Capillary and extraction cone voltages were set at 3.0 kV and 5.0 V, respectively. MS data was then introduced to EZinfo 2.0 software (Waters corp., Milford, USA) for multivariate statistical analysis including principal component analysis (PCA) and orthogonal partial least-squared discriminant analysis (OPLS-DA). VIP-plot of OPLS-DA was used to the discriminating variables between AD and MCI-AD groups. Metabolites were identified from databases by exact molecular weights.
Statistical analysis
GraphPad Prism Version 5.00 (GraphPad Software, San Diego, California, USA) was used for determining the diagnostic effectiveness of the metabolites, following the area under curve (AUC) of receiver operating characteristic curve (ROC). SPSS (version 19, IBM, Chicago, IL, http://www.spss.com) programs for Windows were used for data processing and were run on a PC Intel Core i7-2600K processor (4.0 GHz) with 64 bits.
Results
In this study, the clinical characteristics of participants between patients with MCI and age-matched AD are provided in ESI Table 1.† We found that the average level of A-amyloid protein was significantly higher than that of the MCI. In this work, the saliva samples were analyzed by FUPLC-MS. The typical BPI (base peak intensity) chromatograms of saliva samples in negative ion mode were shown in Fig. 1. 4917 peaks from comprehensive metabolomics profiling were obtained using MarkerLynx software and then were exported into EZinfo 2.0 software for multivariate data analysis. The classification resulted in excellent predictive abilities (R2(X) = 77.9%, R2(Y) = 98.6%, Q2(Y) = 98.3%) shown in Fig. 2. There are obvious separation between AD and MCI-AD. VIP (variable importance in the projection)-plot model of OPLS-DA was used as an important parameter to select the discriminating variables for AD relative to MCI-AD group. VIP value > 12 and P < 0.01 were considered as interesting biomarkers. VIP-plot of AD vs. MCI-AD patients was shown in Fig. 3. Finally, a total of 10 discriminating variables as candidate biomarkers were found in AD relative to the MCI-AD group. These candidate variables were highlighted in VIP-plot (VIP > 12 and P < 0.01), and confirmed by comparison with standards. They were identified and listed in Table 1. Seven discriminating biomarkers were up-regulated and three discriminating biomarkers were down-regulated in saliva of AD patients. Then, we performed a ROC analysis as described in the ‘Methods’ section to assess the feasibility of diagnosis. The detailed parameters were provided in Table 1 which listed the sensitivity, and specificity for AD early prediction. Cytidine, sphinganine 1-phosphate, and 3-dehydrocarnitine had the areas under curve (AUC) values of 0.995, 0.934, and 0907, respectively in AD patients vs. MCI-AD patients. ROC analysis showed a score of 0.90, indicating a high discrimination power and confirmed the potential value for diagnosing AD. For a single candidate biomarker, cytidine had a sensitivity of 99.7% and a specificity of 97.3% for diagnosing AD.
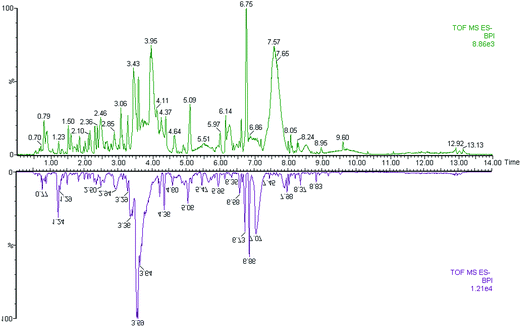 |
| Fig. 1 Typical BPI chromatograms of mild cognitive impairment subjects (up) and Alzheimer's disease patients (down) by FUPLC/MS platform. | |
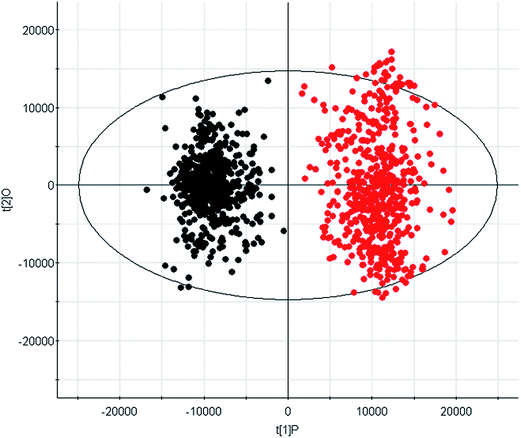 |
| Fig. 2 Score plot of the principal component analysis model of the FUPLC/MS data from mild cognitive impairment patients (black) and Alzheimer's disease group (red). It could also observe a difference between AD and MCI-AD. All saliva samples were divided into two groups: MCI-AD group vs. AD group. | |
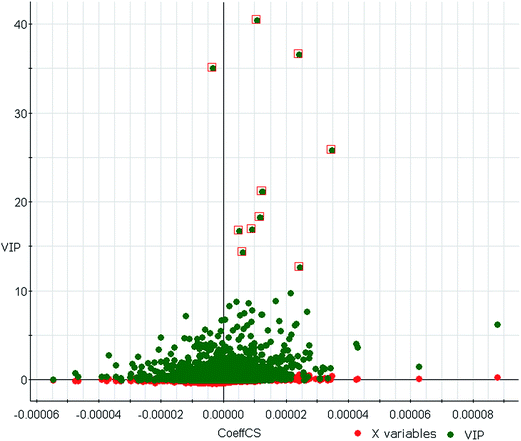 |
| Fig. 3 VIP (variable importance in the projection)-plot for selection of interesting variables for patients with Alzheimer's disease and age-matched mild cognitive impairment patients. | |
Table 1 Information of salivary biomarkers for the predicting conversion of MCI to probable AD detected by FUPLC/MS
No |
Rt (min) |
m/z |
Compound ID |
Formula |
Mass error (ppm) |
Description |
Anova (p) |
Max fold change |
Trend |
VIP |
AUC |
Sensitivity (%) |
Specificity (%) |
1 |
2.04 |
242.0794 |
HMDB00089 |
C9H13N3O5 |
0.55 |
Cytidine |
0.0003 |
5.21 |
↑ |
14.34 |
0.995 |
99.7 |
97.3 |
2 |
3.64 |
380.2556 |
HMDB01383 |
C18H40NO5P |
−1.06 |
Sphinganine-1-phosphate |
0.0009 |
6.11 |
↑ |
40.45 |
0.964 |
98.5 |
96.1 |
3 |
0.98 |
158.0824 |
HMDB12154 |
C7H13NO3 |
1.14 |
3-Dehydrocarnitine |
0.0011 |
8.21 |
↓ |
21.18 |
0.927 |
91.9 |
87.8 |
4 |
1.54 |
165.0558 |
HMDB00779 |
C9H10O3 |
0.69 |
Phenyllactic acid |
0.0008 |
4.54 |
↑ |
35.11 |
0.780 |
76.8 |
83.7 |
5 |
0.56 |
128.0354 |
HMDB00267 |
C5H7NO3 |
0.55 |
Pyroglutamic acid |
0.0007 |
5.64 |
↑ |
16.96 |
0.865 |
90.2 |
89.9 |
6 |
0.51 |
146.0470 |
HMDB00148 |
C5H9NO4 |
0.86 |
L-Glutamic acid |
0.0010 |
7.12 |
↑ |
16.80 |
0.757 |
84.8 |
90.4 |
7 |
0.63 |
131.0825 |
HMDB00214 |
C5H12N2O2 |
−0.52 |
Ornithine |
0.0007 |
4.22 |
↑ |
36.62 |
0.868 |
90.2 |
87.0 |
8 |
2.12 |
203.0803 |
HMDB00929 |
C11H12N2O2 |
1.29 |
L-Tryptophan |
0.0019 |
2.92 |
↑ |
12.66 |
0.677 |
71.9 |
80.2 |
9 |
2.24 |
267.0731 |
HMDB00195 |
C10H12N4O5 |
−1.49 |
Inosine |
0.0004 |
14.85 |
↓ |
25.88 |
0.631 |
90.2 |
87.0 |
10 |
0.77 |
135.0299 |
HMDB00157 |
C5H4N4O |
−2.58 |
Hypoxanthine |
0.0000 |
12.09 |
↓ |
18.29 |
0.640 |
86.8 |
74.9 |
Discussion
In our work, FUPLC-MS combined with multivariate statistical analysis was a useful tool to help us to find metabolites differences from AD patients and MCI-AD patients. Of note, ten metabolites including cytidine, sphinganine-1-phosphate, 3-dehydrocarnitine, phenyllactic acid, pyroglutamic acid, L-glutamic acid, ornithine, L-tryptophan, inosine and hypoxanthine were obtained. In addition, two candidate biomarkers were found to differentiating the AD. Description for the selected biomarkers of AD was grouped as up-regulated and down-regulated metabolites. For up-regulated metabolites, cytidine is a pyrimidine that can serve as substrate for the salvage pathway of pyrimidine nucleotide synthesis; as precursor of the cytidine triphosphate (CTP) needed in the phosphatidylcholine and phosphatidylethanolamine biosynthetic pathway. The transports of cytidine into the brain's extracellular fluid, and then into neurons, are essential prerequisites for cytidine to be utilized in brain. The biosynthesis of PC, the most abundant phosphatide in the brain, via the Kennedy pathway requires phosphocholine and CTP, a cytidine nucleotide, which is involved in the rate-limiting step. Sphinganine-1-phosphate is an intermediate in the metabolism of glycosphingolipids and sphingolipids.17 It is a critical substrate for sphingosine kinase. Sphingolipid metabolism is thus seen to be abnormal in AD patients compared to MCI. The salivary sphinganine-1-phosphate content is higher compared with healthy subjects probably due to the increased expression of the process of sphingosine kinase. Compared with MCI, a higher level of phenyllactic acid was observed in the early stage of AD patients.
Phenyllactic acid, a product of phenylalanine catabolism, is likely produced from phenylpyruvate via the action of lactate dehydrogenase.18 Metabolites of this transamination reaction include phenylacetate, phenylpyruvate and phenethylamine. Phenylalanine accumulation disrupts brain development, leading to mental retardation.19,20 Elevated level of phenyllactic acid appears in the saliva of AD patients probably due to the disorders of phenylalanine metabolism. Pyroglutamic acid is a cyclized derivative of L-glutamic acid. It is formed nonenzymatically from glutamate, glutamine, and gamma-glutamylated peptides, but it can also be produced by the action of gamma-glutamylcyclotransferase on an L-amino acid. Elevated blood levels may be associated with problems of glutamine or glutathione metabolism. This compound is found in substantial amounts in brain tissue. L-Glutamic acid (Glu), a key molecule in cellular metabolism, is the most abundant fast excitatory neurotransmitter in the mammalian nervous system. Glu is involved in cognitive functions like learning and memory in the brain. In brain injury, it can work in reverse and excess glutamate can accumulate outside cells. Ornithine is an amino acid produced in the urea cycle by the splitting off of urea from arginine.21 It is a central part of the urea cycle, which allows for the disposal of excess nitrogen. We found that the levels of ornithine were markedly higher in patients with AD than in healthy controls. Ornithine is also a precursor of citrulline and arginine.22 In order for ornithine produced in the cytosol to be converted to citrulline, it must first cross the inner mitochondrial membrane into the mitochondrial matrix where it is carbamylated by ornithine transcarbamylase. L-Tryptophan is an essential amino acid which is the precursor of serotonin that is a brain neurotransmitter. Metabolism of tryptophan to serotonin requires nutrients such as vitamin B6, niacin and glutathione. For down-regulated metabolites, 3-dehydrocarnitine is a member of the carnitine family that is an intermediate in carnitine degradation. Carnitine is a quaternary ammonium compound biosynthesized from the amino acids lysine and methionine.23 In living cells, it is required for the transport of fatty acids from the cytosol into the mitochondria during the breakdown of lipids for the generation of metabolic energy. Inosine is a purine nucleoside that has hypoxanthine linked by the N9 nitrogen to the C1 carbon of ribose.24 It is an intermediate in the degradation of purines and purine nucleosides to uric acid and in pathways of purine salvage. Hypoxanthine is a spontaneous deamination product of adenine.25 The obvious decrease of inosine and hypoxanthine reveals an abnormal purine metabolism in AD patients.
Conclusions
Here, we used a comprehensive FUPLC/MS platform to analyze saliva samples from MCI-AD and AD patients, aim to discriminate MCL and AD patients, and then key metabolite signatures were identified as possible biomarkers. PCA score model yielded class separation for AD and MCI-AD group. Cytidine and sphinganine-1-phosphate of the AD subjects were significantly different from the MCI-AD subjects. ROC model revealed cytidine and sphinganine-1-phosphate to be potent discriminators between AD and MCI-AD patients. Our study suggested that FUPLC/MS metabolomics as a platform to identify saliva signatures for AD.
Conflict of interest
The authors declare no competing financial interests.
Acknowledgements
We thank BGI for excellent technical assistance. This work was supported by grants from the Key Program of Natural Science Foundation of State (Grant No. 81470196, 81302905).
References
- A. Aguzzi, Neurodegeneration: Alzheimer's disease under strain, Nature, 2014, 512(7512), 32–34 CrossRef CAS PubMed
. - L. H. Tsai and R. Madabhushi, Alzheimer's disease: a protective factor for the ageing brain, Nature, 2014, 507(7493), 439–440 CrossRef CAS PubMed
. - Q. Liang, H. Liu, T. Zhang, Y. Jiang, H. Xing and A. Zhang, Discovery of serum metabolites for diagnosis of mild cognitive impairment to Alzheimer's disease progression using an optimized metabolomics method, RSC Adv., 2016, 6, 3586–3591 RSC
. - J. C. Chen, M. J. Alvarez and F. Talos, et al., Identification of causal genetic drivers of human disease through systems-level analysis of regulatory networks, Cell, 2014, 159(2), 402–414 CrossRef CAS PubMed
. - M. Zhou, F. Zhang and L. Zhao, Entorhinal cortex: a good biomarker of mild cognitive impairment and mild Alzheimer's disease, Rev. Neurosci., 2016, 27(2), 185–195 Search PubMed
. - A. Koppara, S. Wolfsgruber and L. Kleineidam, The latent dementia phenotype δ is associated with cerebrospinal fluid biomarkers of Alzheimer's disease and predicts conversion to dementia in subjects with mild cognitive impairment, J. Alzheimer's Dis., 2015, 49(2), 547–560 CrossRef PubMed
. - A. Lleó, E. Cavedo and L. Parnetti, Cerebrospinal fluid biomarkers in trials for Alzheimer and Parkinson diseases, Nat. Rev. Neurol., 2015, 11(1), 41–55 CrossRef PubMed
. - K. Blennow, N. Mattsson and M. Schöll, Amyloid biomarkers in Alzheimer's disease, Trends Pharmacol. Sci., 2015, 36(5), 297–309 CrossRef CAS PubMed
. - P. Wang, B. Zhou and H. Yao, et al., Aberrant intra-and inter-network connectivity architectures in Alzheimer's disease and mild cognitive impairment, Sci. Rep., 2015, 5, 14824 CrossRef CAS PubMed
. - Q. Liang, H. Liu, Y. Jiang, H. Xing, T. Zhang and Z. Ai-hua, High-throughput metabolic profiling for discovering metabolic biomarkers of sepsis-induced acute lung injury, RSC Adv., 2016, 6(13), 11008–11013 RSC
. - T. J. Wang, M. G. Larson and R. S. Vasan, Metabolite profiles and the risk of developing diabetes, Nat. Med., 2011, 17(4), 448–453 CrossRef CAS PubMed
. - Q. Liang, H. Liu, T. Zhang, Y. Jiang, H. Xing and A. H. Zhang, Potential urine biomarkers from a high throughput metabolomics study of severe sepsis in a large Asian cohort, RSC Adv., 2015, 5(124), 102204–102209 RSC
. - Y. Tian, X. Nie and S. Xu, et al., Integrative metabonomics as potential method for diagnosis of thyroid malignancy, Sci. Rep., 2015, 5, 14869 CrossRef CAS PubMed
. - R. González-Domínguez, F. Javier Rupérez and T. García-Barrera, et al., Metabolomic-Driven Elucidation of Serum Disturbances Associated with Alzheimer's Disease and Mild Cognitive Impairment, Curr. Alzheimer Res., 2016, 13(6), 641–653 CrossRef
. - S. F. Graham, O. P. Chevallier and C. T. Elliott, et al., Untargeted Metabolomic Analysis of Human Plasma Indicates Differentially Affected Polyamine and L-Arginine Metabolism in Mild Cognitive Impairment Subjects Converting to Alzheimer's Disease, PLoS One, 2015, 10(3), e0119452 Search PubMed
. - Q. Liang, H. Liu and T. Zhang, et al., Metabolomics-based screening of salivary biomarkers for early diagnosis of Alzheimer's disease, RSC Adv., 2015, 5(116), 96074–96079 RSC
. - C. Tomlinson, M. Rafii and R. O. Ball, et al., Arginine can be synthesized from enteral proline in healthy adult humans, J. Nutr., 2011, 141(8), 1432–1436 CrossRef CAS PubMed
. - T. Fujita, H. D. Nguyen and T. Ito, et al., Microbial monomers custom-synthesized to build true bio-derived aromatic polymers, Appl. Microbiol. Biotechnol., 2013, 97(20), 8887–8894 CrossRef CAS PubMed
. - R. Cools, S. J. G. Lewis and L. Clark, et al., L-DOPA disrupts activity in the nucleus accumbens during reversal learning in Parkinson's disease, Neuropsychopharmacology, 2007, 32(1), 180–189 CrossRef CAS PubMed
. - A. Pinna, J. Bonaventura and D. Farré, et al., l-DOPA disrupts adenosine A 2A–cannabinoid CB 1–dopamine D 2 receptor heteromer cross-talk in the striatum of hemiparkinsonian rats: Biochemical and behavioral studies, Exp. Neurol., 2014, 253, 180–191 CrossRef CAS PubMed
. - L. Boon, W. J. C. Geerts and A. Jonker, et al., High protein diet induces pericentral glutamate dehydrogenase and ornithine aminotransferase to provide sufficient glutamate for pericentral detoxification of ammonia in rat liver lobules, Histochem. Cell Biol., 1999, 111(6), 445–452 CrossRef CAS PubMed
. - C. Tomlinson, M. Rafii and R. O. Ball, et al., Arginine can be synthesized from enteral proline in healthy adult humans, J. Nutr., 2011, 141(8), 1432–1436 CrossRef CAS PubMed
. - M. D. He, S. C. Xu and Y. H. Lu, et al., L-carnitine protects against nickel-induced neurotoxicity by maintaining mitochondrial function in Neuro-2a cells, Toxicol. Appl. Pharmacol., 2011, 253(1), 38–44 CrossRef CAS PubMed
. - H. Zhao, C. R. Chiaro and L. Zhang, et al., Quantitative analysis of purine nucleotides indicates that purinosomes increase de novo purine biosynthesis, J. Biol. Chem., 2015, 290(11), 6705–6713 CrossRef CAS PubMed
. - O. Sidorkina, M. Saparbaev and J. Laval, Effects of nitrous acid treatment on the survival and mutagenesis of Escherichia coli cells lacking base excision repair (hypoxanthine-DNA glycosylase-ALK A protein) and/or nucleotide excision repair, Mutagenesis, 1997, 12(1), 23–28 CrossRef CAS PubMed
.
Footnote |
† Electronic supplementary information (ESI) available. See DOI: 10.1039/c6ra16802g |
|
This journal is © The Royal Society of Chemistry 2016 |
Click here to see how this site uses Cookies. View our privacy policy here.