Modeling low impact development in two Chicago communities
Received
28th April 2015
, Accepted 29th July 2015
First published on 4th August 2015
Abstract
Sustainable practices that will aid in reducing runoff volume and nutrient loading during storm events are needed in many urban areas. Continued assessment of the hydrologic impact of low impact development (LID) practices in urban communities is needed to support development and re-development of residential areas. An evaluation using the L-THIA LID model of two south-side Chicago communities with hypothetical implementation levels of LID features is presented in this study. The results revealed that incorporating LID practices such as porous pavement, and rain barrels, could have a noticeable impact on average annual runoff and the nonpoint source (NPS) pollutants carried in the runoff. Porous pavement on 50% of current parking lot space could result in an estimated 24% decrease in runoff volume. Additionally, the runoff reduction for all scenarios ranged from 2–24%. Hence, these results indicate that implementation of LID features in urban communities could provide substantial benefits.
Water impact
In the city of Chicago, low impact development (LID) can be implemented to decrease runoff and enhance water quality during development and/or re-development of high-density residential areas. There is a great deal of impervious surface area in Chicago, IL that lends itself to heavy runoff and non-point source pollution (NPS). In this study, various LID features (i.e. rain barrel, green roof, bio-retention, and porous pavement) were modeled using L-THIA to quantify the impact on runoff and NPS. The results revealed that these features could have a noticeable impact on average annual runoff and the nonpoint source (NPS) pollutants carried in the runoff. For example, porous pavement on 50% of current parking lot space could result in an estimated 24% decrease in runoff volume.
|
1 Introduction
In densely populated residential areas, such as the South Side of Chicago, Illinois (USA), activities associated with urbanization may contaminate water resources. The South Side of Chicago has undergone major residential changes resulting in impaired waterways.1 The United States Environmental Protection Agency (USEPA) identified urbanization as a major contributor of runoff and pollutant losses for water quality deterioration. Nineteen years ago, the USEPA reported that 14 of the 18 national estuaries contained significant nonpoint source pollution from urban runoff. According to Hayhoe et al.,2 Chicago is susceptible to “increases in temperature, particularly extremely hot days and extended heat waves, as well as projected increases in heavy rainfall events associated with flooding.” There are more than 160 kilometers of water bodies that form the Chicago Area Waterway System (CAWS), which connects Lake Michigan with the Mississippi River via the Lower Des Plaines and Illinois rivers.3 With the onset of residential and commercial development and recreational activities along the Chicago River, many discussions took place to improve water quality in the Chicago River. The USEPA estimated the cost of abatement at over US $44 billion; however, the quantitative importance of these sewer systems has not received a great deal of attention.4 The city of Chicago disseminated a water quality report where they samples seven sites six times in relation to the drinking water system in 2011.5
In the early 1900s, engineers diverted the flow of water from eastward to westward in order to prevent human and industrial waste from entering Lake Michigan.6 The diversion of water in the Chicagoland area led to major controversies that expanded to other states in the Midwest and even Canada. These states wanted the diversion stopped or reduced.6 Currently, billions of dollars have been spent on the Tunnel and Reservoir Plan (TARP) to reduce stormwater runoff in Chicago.7 The TARP plan was put in to place to relieve the combined sewer overflow (CSO) system during storm events. 0.33 inch of rain could overload the CSO system causing sewage and runoff to combine and pollute waterways that serves as drinking water sources. Major pollutants for the Chicago area include: oil and grease, road salt, metals and solids.8 In order to combat these pollutants the City of Chicago has implemented best management practices such as rooftop gardens, rain barrels, vegetated swales, and oil and great traps to name a few at various locations throughout the city.8
While the use of conventional stormwater management practices will remain a necessity (especially in older, built-out communities), adoption of innovative strategies such as low impact development (LID) practices can aid not only in reducing the costs for stormwater management but also in promoting natural hydrologic functions and environmental sustainability.9–13 Brabec14 indicated that impervious surfaces found in urban and suburban areas are not difficult for urban planners to incorporate when providing standards for roads, streets, driveways, and parking lots to help enhance water quality. The use of LID practices in Chicago and other communities would be a relatively simple and sustainable approach to reduce costs of stormwater management and protect water resources.
Van Roon15 indicated that water cycle management is imperative in urban settings. A holistic approach to tackling the sustainability of the economy, nature and overall quality of life should be considered. He also suggested that management of stormwater through natural systems rather than drains/pipes is increasingly a preferred approach to reduce runoff amount in sewer systems, reduce NPS pollutions, and improve community livability.
1.1 Low impact development
Low impact development techniques are increasingly being implemented in urbanized areas. LID is relatively new and in parts of the U.S. Midwest, there are few studies on LID implementation.16,17 Even fewer studies have explored the benefits of reduced runoff in urban green spaces such as Chicago.4 This is mainly due to projected costs of implementation and the belief that the amount of land that could be devoted to LID practices is minimal;18 on the other hand LID practices work best in high-density residential communities. One study showed that 40% of urban runoff came from urban roofs and private driveways.19 LID practices can be implemented in any setting (i.e. new development, redevelopment, or retrofitted to existing land) to preserve landscapes, reduce impervious surfaces, treat stormwater effectively by improving water quality, and recreating the natural movement of water within a watershed.12,13,20–30 Finally, EPA purports that LID implementation can “restore a watershed's hydrologic and ecological functions.”31
Porous (permeable) pavement can be implemented in place of traditional asphalt to decrease runoff. It is suitable for residential, commercial, and industrial areas but for light-duty purposes.32 A range of sustainable materials and techniques for permeable pavements with a base and sub-base allow the movement of stormwater through the surface. In addition to reducing runoff, this effectively traps suspended solids and filters pollutants from the water. A second type of LID practice is the green roof system, for which research has indicated that stormwater management improved habitats for wildlife, and residential energy usage declined for cooling.33 A third type of LID practice is the use of the rain barrel, which, works to collect and store rainwater from the roof that would otherwise be lost to runoff and diverted to storm drains and streams. Lawn and garden watering make up nearly 30% of total household water use during the summer.34 It provides an ample supply of free “soft water” to homeowners, containing no chlorine, lime or calcium making it ideal for gardens, flowerpots, and car and window washing. Another type of LID is bioretention systems, also called biofilters or rain gardens, which are vegetation planted to assist in the uptake of nutrients before they reach unsafe levels. They have been found to capture oil, grease, suspended solids, and heavy metals.35 They are also recommended for reducing runoff, groundwater recharge, and enhancing water quality.36 Bedan & Clausen37 found that implementing LID features in LID designed subdivision vs. a traditional subdivision in paired watersheds revealed a decrease in runoff and NPS.
Application of LID practices requires public awareness and education for successful implementation and maintenance.38 Brehm et al.,39 studied the adoption of best management practices (BMP) since households are major pollutant loading contributors that impact receiving water quality. The authors found that education and awareness were the two main factors that either prompts homeowners to implement BMPs or not implement. The United States Geologic Survey (USGS) carried out a small-scale study on implementing LID features such as porous pavement, green roofs, and rain gardens near the Ipswich River Basin in Massachusetts.40
1.2 Purpose of study
This study was conducted to determine potential use of LID practices for reducing runoff and NPS to improve water quality in two communities on Chicago, IL. The objectives are to bring to light the benefits of applying different LID practices in a series of hypothetical scenarios that could be beneficial to decreasing stormwater runoff, thus impacting the ongoing TARP project. Chicago has a high population density, which leads to an increased quantity of impervious surfaces for housing and vehicle traffic. The amount of construction ongoing in and around Chicago annually alone contributes quite heavily to reduced water quality. To learn more about water quality and monitoring in the Chicago area, please see Illinois EPA.41
1.3 L-THIA-LID model description
In this study, the Long-Term Hydrologic Impact Assessment (L-THIA LID) model42 (Ahiablame et al., 2013) was used in evaluating the effects of various LID scenarios in an urban setting. The L-THIA-LID model is a revised version of the original L-THIA model developed to assess changes in past and/or recommended land use changes impacting runoff, recharge, and non-point source pollution.43–45 The L-THIA-LID model extends L-THIA to model LID practices such as roads, parking lots, porous pavement, rain barrels, and rain garden features. L-THIA-LID uses the curve number method46 based on event mean concentration, soil attributes, land cover, and long-term rainfall data for the area of interest to estimate long-term average annual runoff and NPS pollutant load.43,47 L-THIA can be used as an alternative to more complex hydrologic models in providing a decision-support tool to explore possible future environmental impacts.48
L-THIA LID, in addition to L-THIA, has been widely used in many studies. The L-THIA and L-THIA LID models have previously been used in calibrated and uncalibrated modes, and in case studies to illustrate and inform planners or to mimic real-world conditions.47,49–51 Specifically, Grove et al.,52 found that estimated streamflow data in conjunction with the uncalibrated L-THIA model provided a reasonable estimate for average annual runoff. The L-THIA-LID model can simulate green roof, permeable patio, porous pavement, rain barrel/cistern, rain garden, and grass swale features on lot and watershed scales. The CN values were altered to reflect the effects of the above-mentioned LID practices related to hydrology and water quality.12,13 The basis for altering the CN values is that these values are essential to simulating LID practices. The altered CN values were based on Wilson and Weng53 study to adjust for the LID practices applied in the study area. Lim et al.,51 and Ahiablame et al.,42 also adjusted CN values to enhance pollutant estimation and runoff for their study areas.
Additionally, it has proven accurate for modeling direct runoff from watersheds throughout the Midwest with little or no calibration.42,48,52,54 Liu et al.,56 found that L-THIA LID could assist stakeholders in assessing the environmental impact of LID practices to make more effective stormwater management decisions. Stagge et al.,57 investigated the impact of large parking lots in urban areas and found that runoff and pollutant levels were increased. Ahiablame et al.,42 revealed that the L-THIA LID model illustrated that incorporating LID practices in urban settings were effective in reducing runoff and pollution levels. The L-THIA LID model is a useful tool in modeling the impact of LID practices in urban and rural settings.12,13,56
1.4 Model assumptions
The L-THIA-LID model assumes that one rain barrel will collect runoff from on housing unit with the assumption that the barrel has the appropriate size to handle one inch of rain. The barrel is represented by a curve number (CN) value that accounts for barrel effectiveness. The porous pavement component assumed that conventional streets, driveways, parking lots, side streets, and sidewalks were converted to porous pavement. During simulation of porous pavement, it was assumed that the pavement did not receive runoff from other impervious surfaces, but from rainfall directly. The simulated rain garden feature was implemented on available green space. For model simulation, only 25% of the available green space was treated with rain garden to capture and infiltrate runoff for both communities (i.e. SLOOP-24ha and Douglas-51ha). Previous LID modeling studies also used similar assumptions to model LID practices at community and watershed scales (e.g. Ahiablame et al., 2012a and 2012b; Di Vittorio and Ahiablame, 2015; Wang et al., 2005; Liu et al., 2008).12,13,55,58,59
2 Methods
A total of 26 ‘what-if scenarios’ (Table 1) were developed to assess the effectiveness of runoff reduction. In this study, the L-THIA LID model was used to determine daily estimates of direct runoff over the watershed from 1957 to 2001 using the distributed approach.52,60 The modeling process entailed creating the scenarios, gathering precipitation data, compiling curve number values for the various land uses, and computation of runoff and NPS pollutant loads. In addition, this project involved community impact and not watershed impact, so the need for analysis using a defined watershed was not critical.
Table 1 Land use types and areas for SLOOP and Douglas communities in Chicago, IL. (Note: the impervious surfaces include paved streets (roads), parking lots, and flat roofs)
2006 NLCD land use for the Douglas community-Chicago, IL |
2006 NLCD land use for the SLOOP community-Chicago, IL |
Description |
Area (ha) |
% of land area |
Description |
Area (ha) |
% of land area |
Green space |
203 |
26% |
Green space |
97 |
17% |
Parking lot |
320 |
42% |
Parking lot |
144 |
25% |
Rooftop |
62 |
8% |
Rooftop |
93 |
16% |
Roads |
144 |
19% |
Roads |
139 |
24% |
Green space devoted to specific activities |
42 |
5% |
Green space devoted to specific activities |
102 |
18% |
Total |
771 |
|
Total |
575 |
|
2.1 Study area
The study area consists of two communities located in the South Side of Chicago: the South Loop (SLOOP) and Douglas communities. The SLOOP is approximately 575 hectares (about 2 square miles) and Douglas, approximately 771 hectares (about 1.6 square miles). In the state of Illinois, the average population density is 231.1 persons per square mile, while the SLOOP community has 13
211 people per square mile, and the Douglas community has 13
322 people per square mile.61 Both communities are south of Chicago's “Loop” and border Lake Michigan. The SLOOP was a sparsely populated industrial area, but is now a thriving community of young professionals and students of nearby colleges. The neighborhood features notable historical landmarks that include the 1836 Clarke House, one of Chicago's oldest residences, a diverse dining scene, blues clubs and other nightlife options, and the Museum Campus and Loop are a short stroll away. The SLOOP is also a very successful large-scale redevelopment. The Douglas community is located near the Illinois Institute of Technology and Illinois College of Optometry. During the 20th century, Douglas was known as the “Black Metropolis,” one of the nation's most significant landmarks of African-American urban history. Between 1910 and 1920, during the peak of the “Great Migration,” the population in the Douglas community increased when thousands of African-Americans migrated to Chicago in search of industrial jobs. Notably, between the 1940s and 1960s, a replacement of the “slums” with high-rise public housing projects occurred, managed by the Chicago Housing Authority, which isolated and concentrated underrepresented and minority populations in this section of the city. These were demolished in the late 1990s and early 21st century, with plans to redevelop the area with lower density housing which would be an ideal setting for LID.
2.2 Data used
For this project, ten years of daily rainfall (2001–2010) were extracted from Chicago Midway Airport, rain gauge station through the National Climatic Data Center.62 This station is located approximately 16 kilometers west of the two communities. The 2006 National Land Cover Database was utilized to extract land use data for the two communities. Aerial photo and geographic information data (i.e. roads, sidewalks, curbs, buildings, landuse type, water bodies) were extracted from the Chicago Data Portal (http://chicagodatawetpaint.com/page/GIS+Shapefiles). Manual digitization of land uses was completed with ArcMap 10 by overlaying an aerial photo of 2006 and the neighborhood maps obtained from the Chicago Data Portal City of Chicago website. Curve number values and NPS pollutant coefficients (i.e. total nitrogen, lead, cadmium, nickel, total phosphorus, biochemical oxygen demand, chemical oxygen demand, suspended solids, oil and grease) associated with different land use types were obtained from Wilson and Weng53 and incorporated into L-THIA LID.
2.3 LID simulation scenarios
After careful analysis of the aerial photos of the two communities, there were 5 prevalent land uses: parking lots, flat roofs, roads, and green space (i.e. soccer field, baseball fields). Thirteen scenarios using the various land use types (Tables 1 and 2) were developed for this study to assess runoff in the SLOOP and Douglas communities. The scenarios included individual as well as combinations of the land use types found in the two communities. The scenarios ranged in variations of 25%, 50% and 75% of land use area with applied LID practices. The only exception was within the green space land use, where the land was devoted to specific activities that would not be changed, so the amount of land hypothetically altered to apply LID features remained unchanged. For the green space that could be altered, 25% of the area in both communities was treated with rain garden for model simulation in scenarios 7–13.
Table 2 Low impact development implementation scenarios (SC) simulated in SLOOP and Douglas communities in Chicago, IL
|
SC 1 |
SC 2 |
SC 3 |
SC 4 |
SC 5 |
SC 6 |
SC 7 |
SC 8 |
SC 9 |
SC 10 |
SC 11 |
SC 12 |
SC 13 |
A – 25% of area treated with LID practice. B – 50% of area treated with LID practice. C – 75% of area not treated with LID practice. D – Area treated with rain garden LID practice. |
Land use |
|
|
|
|
|
|
|
|
|
|
|
|
|
Green space w/ rain garden |
|
|
|
|
|
|
D |
D |
D |
D |
D |
D |
D |
Roads |
|
|
|
C |
|
C |
|
|
|
C |
|
|
C |
Roads with porous pavement |
|
|
|
A |
|
A |
|
|
|
A |
|
|
A |
Roof |
|
|
A |
|
C |
C |
|
C |
C |
C |
|
|
|
Roof w/ rain barrel |
|
B |
C |
|
A |
A |
|
A |
A |
A |
B |
B |
B |
Parking lot |
|
|
|
|
C |
C |
|
|
C |
C |
|
C |
C |
Parking lot w/ porous pavement |
B |
|
|
|
A |
A |
|
|
A |
A |
|
A |
A |
The scenarios consisted of hypothetical percentages of land use implemented as various LID practices. We attempted to be as realistic as possible in taking into account the cost-effectiveness of each land use and the geography of the land in both communities. These two communities are densely residential with green space, large amounts of roofs, sidewalks, roads and parking lots. Varying the percentages conservatively provided a backdrop for a paradigm shift in residential design.
3 Results and discussion
3.1 Runoff volume
The baseline for SLOOP annual runoff volume was 4500 m3 ha−1 per year, while Douglas results showed 4750 m3 ha−1 per year (Fig. 1). Runoff for both the SLOOP and Douglas communities revealed a decreasing trend from the baseline with implementation of the various LID practice scenarios described in Table 2 (Fig. 1 and 2). The addition of porous pavement on 50% of the parking lots (SC 1) resulted in the largest decline (24%) in average annual runoff if implemented in the Douglas community, while the SLOOP community required a diverse implementation of LID practices (SC 13) to attain a 20% decrease in annual runoff (Fig. 2). This could be attributed to the increased number of parking lots, types of buildings, and size of the community. A decline in annual runoff was also revealed when 25% of the parking lots contained porous pavement, 25% of roads contained porous pavement, and 25% of the roofs included rain barrels (SC 6, SC 10, SC 12, and SC 13 in Fig. 2). The results show minimum reduction in average annual runoff when only the rain garden feature was applied (SC 7; Table 2 and Fig. 2). Similar reductions were also observed when rain garden was added in combination with 25% porous pavement applied to roads and/or 25% roof with a rain barrel attached.
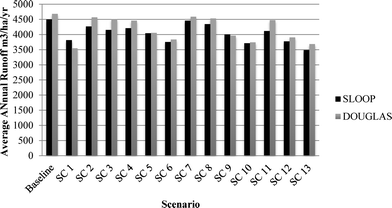 |
| Fig. 1 Comparison of SLOOP and Douglas average annual runoff volume for current baseline case and LID scenarios. | |
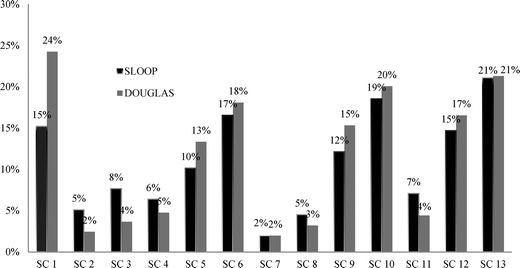 |
| Fig. 2 Percent change in runoff volume for baseline case and LID scenarios in the SLOOP and Douglas communities in Chicago, IL. | |
Percent change in predicted average annual runoff appeared high (17–24% reduction) in SC 1, SC 6, SC 10, SC 12 and SC 13, which follows the trend stated previously where a percentage of the parking lots have porous pavement (Fig. 3). The rain garden LID practice by itself or coupled with a rain barrel (SC 7) was not as impactful as expected. This scenario revealed the least change in average annual runoff for both communities (2–5%) as shown in Fig. 2. It appeared from the results that porous pavement alone could have a large impact on these two communities in reducing runoff.
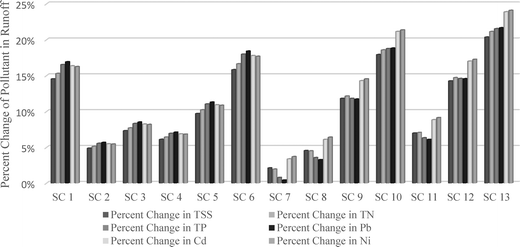 |
| Fig. 3 SLOOP percent change of pollutants in runoff volume for baseline case and LID scenarios. | |
3.2 Nonpoint source pollution
Pollutants in runoff followed the same trend as annual runoff volume in relation to each of the scenarios for both communities (Fig. 3 and 4). These pollutants include: total suspended solids, total nitrogen and total phosphorous. When all LID practices were applied (i.e. SC 12 & SC 13), there was a substantial decrease in nutrient loading and suspended solids as compared with the baseline. Additionally, with 50% of the parking lots covered with porous pavement (SC 1), the decrease in nutrient loading was comparable to SC 12 and SC 13 (Fig. 3 and 4). This decrease could be attributed to increased infiltration and corresponding decrease in runoff due to the porous pavement.
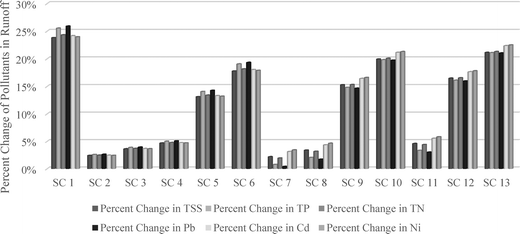 |
| Fig. 4 Douglas percent change in pollutants in runoff volume for baseline case and LID scenarios. | |
Biological oxygen demand (BOD), chemical oxygen demand (COD) and oil and grease (O&G) could also have significant reductions if the LID practices explored are implemented on the vast amount of parking lots in these two communities (Fig. 5 and 6). These three pollutants are major contributors to Chicago's water quality issue along with chlorides and road salt. Wilson and Weng (2010)53 found that areas with excessive human activities have greater concentrations of BOD, COD and O&G than areas with fewer human activities. With the large number of parking lots and roads in the two communities (SLOOP and Douglas), these pollutants could be reduced by 20% in the SLOOP community and 25% for the Douglas community (Fig. 5 and 6). Chapman and Horner63 found that with the use of rain garden systems in Seattle, WA, 92–96% of motor oil could be retained within the system.
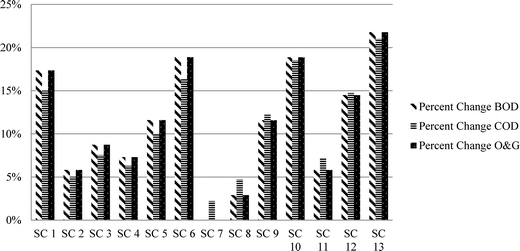 |
| Fig. 5 SLOOP results for percent change in comparison to baseline for BOD, COD, and oil and grease for scenarios described in Table 2. | |
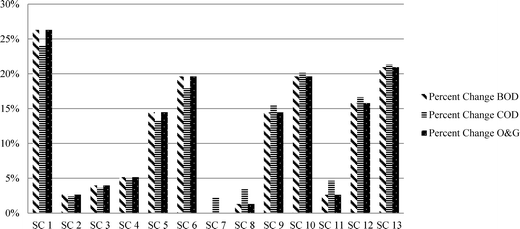 |
| Fig. 6 Douglas results for percent change in comparison to baseline for BOD, COD, and oil and grease for scenarios described in Table 2. | |
3.3 Comparison of SLOOP and Douglas
Both communities followed the same trend of a reduction in average annual runoff and nutrient loading for all 13 scenarios (Fig. 1 and 2). The land use mix had an impact on the amount of runoff and nutrient loading for both communities as expected. SLOOP (2–15% reduction-Fig. 2) did not have as much land devoted to parking lots as the Douglas (2–24% reduction-Fig. 2) community, which showed lower reductions in annual runoff and nutrient loading. SLOOP showed slightly higher reductions than the Douglas community for average annual runoff in SC 1 and SC 9 (24% and 15%, respectively-Fig. 2) due to the area of land devoted to parking lots being significantly less in the SLOOP community. Additionally, these two communities have a high population density with high traffic and a plethora of construction sites that are constantly building residential and business structures that contribute to NPS in the catchment area. EPA stated “agricultural runoff, construction site runoff, urban runoff, hydrologic modifications, and resource extraction activities are the major sources of NPS pollution that impair Illinois' surface water and groundwater resources.”41
The results revealed that in the SLOOP community (Fig. 1), change in runoff was most apparent in SC 13 (i.e. 25% of the parking lots treated with porous pavement, 50% of rooftops have rain barrels, 25% of parking lots treated with porous pavement, 25% of roads treated with porous pavement, and rain garden features in both communities). The results also showed that with only a rain garden feature, runoff had little change. This could be due to the small area (see Table 1) of green space devoted to rain garden in the SLOOP community for this scenario. The SLOOP community had greater reduction in runoff in SC 2, SC 3, SC 4, SC 8, and SC 11 (Fig. 2). This could be attributed to the type of buildings, quantity of roads, buildings in close proximity, and more high-rises. There are more single family home, parking lots and flat roofs within the Douglas community.
In the Douglas community (Fig. 1), change in runoff was most apparent in SC 13 (25% of the parking lots treated with porous pavement, 50% of rooftops have rain barrels, 25% of parking lots treated with porous pavement, 25% of roads treated with porous pavement, and rain garden features). Similar to the SLOOP community, the results also showed that with only a rain garden feature, runoff in the Douglas community had little change as the green space (see Table 1) devoted to rain garden in the Douglas was also relatively small and drainage was assumed to infiltrate. The Douglas community displayed a greater change in runoff in SC 2, SC 3, SC 4, SC 8, and SC 11 (Fig. 2). Runoff reduction in the Douglas community was highest for LID practice implementation, which could be due to the area of parking lots and roads that could be converted to porous pavement. The Douglas area had greater change in runoff than SLOOP in SC 1, SC 5, SC 6, SC 9, SC 10, and SC 12 (Fig. 2). Overall, the highest percent decrease in runoff was shown with porous pavement implemented on parking lots (SC 1-Fig. 3) and adoption of all LID practices (SC 13-Fig. 3). Although the percentage reductions show the greatest difference as 24%, this could have major impact on stormwater management if these LID practices could be adopted and managed appropriately.
3.4 Implications on urban planning
Moglen and Kim64 stated that if more than 15% of impervious surface is present in a watershed, undesirable changes in natural hydrology may occur. The authors argue that a 10% impervious threshold be set for all LID studies to allow for future comparisons of NPS and runoff. The studied communities have large amounts of impervious surface (69% in Douglas community and 65% in SLOOP community) and thus would benefit from LID practices. With the percent imperviousness in the two communities high above the threshold that Moglen and Kim64 discussed, the results revealed that low impact development could decrease runoff and NPS by as much as 20%. Although in this study, we did not reach a 10% threshold for these communities due to the reality of costs in applying porous pavement on the many roads that encompass these communities, the model did show a decrease in runoff volume and NPS. Even adding 25% porous pavement to both parking lots and roads that run throughout the SLOOP and Douglas neighborhoods would aid in reducing runoff by at least 20% with strategic low impact development features (Fig. 1 and 2). With the onset of new developments in both communities, developers can add rain gardens in addition to porous pavement on sidewalks and parking lots, and connect rooftops to rain barrels in order to increase infiltration. In these two communities, the quantity of available green space for treatment with rain gardens was low (i.e. only 25% of available green space for each area was simulated – SLOOP area treated with rain garden and Douglas area treated with rain garden), which is reflected in the results for SC 7 where runoff and NPS did not decrease significantly. Since development in Chicago has been mainly condominiums and mixed income apartment style buildings, these LID practices can have a positive impact in reducing costs of stormwater management by reducing runoff and NPS pollution (Fig. 3 and 4). In 2000, the city of Seattle, Washington found that by reducing impervious surface by 11% in one of their neighborhoods could produce drastic results in reducing stormwater runoff. The city implemented roadside swales and planted 1200 shrubs and trees to reduce stormwater runoff by 99%.65 In a recent study by the Natural Resources Defense Council (NRDC) on the impact of implementing green infrastructure in an area on the northwest side of Chicago, revealed that implementing bioswales, street trees, hanging gardens, rain barrels, and permeable pavement could potentially reduce runoff and NPS to the sewer system by as much as 30%.66
4 Conclusions
In order to enhance water quality in urban communities such as the SLOOP and Douglas, implementation of LID practices may be an effective tool. This study examined the impact of hypothetical scenarios if two Chicago communities (SLOOP and Douglas) were to adopt LID practices (i.e. rain garden, porous pavement, green roof, and rain barrel). The L-THIA-LID model was used to explore scenarios due to its capability to model diverse LID conditions in a rather rapid and an efficient manner. The results revealed that implementation of LID practices may reduce runoff volume and NPS pollutants for Chicago. The highest reduction was shown when porous pavement was implemented in parking lots. In these two communities, the amount of impervious surface in each community was above 60%; however, with the introduction of porous pavement and/or rain garden features, the amount of runoff from these sites can be reduced considerably. Proper planning will be a major factor in implementing porous pavement on road sides and parking lots; however, new residential development could use porous pavement for small residential parking lots and sidewalks. The ability to establish a simple but quantitative approach to assess the estimation of stormwater runoff and pollutant loads from alternative land use scenarios may help homeowners, planners, and policy makers build the understanding of how to adopt stormwater management practices. However, future field studies should be conducted to assure that the multifaceted nature of a watershed is accounted for before implementation. Additionally, curve number values were obtained from scholarly literature which could over or underestimate the low impact development features implemented in this study. Finally, the study's results imply that with a combination of rain garden, rain barrel, and porous pavement features could be beneficial in improving water quality in these two communities.
Acknowledgements
This project was supported by the School for New Learning at DePaul University and the Department of Agricultural & Biological Engineering (ABE) at Purdue University. The authors would like to thank Larry Theller of ABE at Purdue University for his assistance with GIS data processing.
References
- City of Chicago, (2014), Chicago Green Stormwater Infrastructure, http://www.cityofchicago.org/content/dam/city/progs/env/ChicagoGreenStormwaterInfrastructureStrategy.pdf, (accessed July 2015).
- K. Hayhoe, M. Robson, J. Rogula, M. Auffhammer, N. Miller and D. Wuebbles,
et al., An integrated framework for quantifying and valuing climate change impacts on urban energy and infrastructure: A Chicago case study, J. Great Lakes Res., 2010, 36, 94–105 CrossRef PubMed.
-
USEPA, Chicago River, http://www.epa.gov/region5/chicagoriver/index.html, (accessed July 2015).
- G. Zhang, J. Hamlett, P. Reed and Y. Tang, Multi-objective optimization of low impact development designs in an urbanizing watershed, Open J. Optim., 2013, 2(4), 95–108 CrossRef.
-
City of Chicago. (2011). City of Chicago Emerging Contaminant Study, http://www.cityofchicago.org/city/en/depts/water/supp_info/water_quality_resultsandreports/city_of_chicago_emergincontaminantstudy.html, (accessed July 2015).
- S. Changnon and M. Glantz, The Great Lakes diversion at Chicago and its implications for climate change, Clim. Change, 1996, 32(2), 199–214 CrossRef.
-
City of Chicago, (2014), Stormwater Manuel, http://www.cityofchicago.org/content/dam/city/depts/water/general/Engineering/SewerConstStormReq/2014StormwaterManual.pdf, (accessed July 2015).
-
City of Chicago- Department of the Environment, (2003), http://water.epa.gov/polwaste/nps/stormwater/upload/2003_03_26_NPS_natlstormwater03_21Malec.pdf, (accessed July 2015).
- G. Doubleday, A. Sebastian, T. Luttenschlager and P. Bedient, Modeling hydrologic benefits of low impact development: A distributed hydrologic model of the Woodlands, Texas, J. Am. Water Resour. Assoc., 2013, 49(6), 1444–1455 CrossRef PubMed.
- Y. Liu, L. Ahiablame, V. Bralts and B. Engel, Enhancing a rainfall-runoff model to assess the impacts of BMPs and LID practices on storm runoff, J. Environ. Manage., 2015, 147, 12–23 CrossRef PubMed.
- G. Zhang, J. Hamlett, P. Reed and Y. Tang, Multi-objective optimization of low impact development designs in an urbanizing watershed, Open J. Optim., 2013, 2(4), 95–108 CrossRef.
- L. Ahiablame, B. Engel and I. Chaubey, Effectiveness of low impact development practices: Literature review and suggestions for future research, Water, Air, Soil Pollut., 2012, 223(7), 4253–4273 CrossRef CAS.
- L. Ahiablame, B. Engel and I. Chaubey, Representation and evaluation of low impact development practices with L-THIA-LID: An example for site planning, Environ. Pollut., 2012, 1(2), 1–13 Search PubMed.
- E. Brabec, S. Schulte and P. L. Richards, Impervious surfaces and water quality: A review of current literature and its implications for watershed planning, J. Plan. Lit., 2002, 16(4), 499–514 CrossRef PubMed.
- M. Van Roon, Emerging approaches to urban ecosystem management, the potential of low impact urban design and development principles, JEAPM, 2005, 7(1), 125–148 Search PubMed.
- J. Crick and L. Prokopy, Prevalence of conservation design in an agriculture-dominated landscape: The case of northern Indiana, Environ. Manage., 2009, 43(6), 1048–1060 CrossRef PubMed.
- J. R. Miller, M. Groom, G. R. Hess, T. Steelman, D. L. Stokes and J. Thompson, Biodiversity conservation in local planning, Conserv. Biol., 2009, 23(1), 53–63 CrossRef PubMed.
- F. Montalto, C. Behr, C. K. Alfredo, M. Wolf, M. Arye and M. Walsh, Rapid assessment of the cost-effectiveness of low impact development for CSO control, Landsc. Urban Plan., 2007, 82(3), 117–131 CrossRef PubMed.
-
J. Heaney, L. Wright and D. Sample, Sustainable urban water management, in, Innovative Wet-Weather Flow Collection/Control/Treatment Systems For Newly Urbanizing Areas In The 21st Century, ed. J. Heaney, R. Field and R. Pitt, Technomics, Lancaster, PA, 1999, ch 3 Search PubMed.
-
USEPA, Low impact development. A literature review. Washington, D.C., Office of Water, EPA-841-B-00-005, http://nepis.epa.gov/Exe/ZyPURL.cgi?Dockey=P1001B6V.txt, (accessed July 2015).
-
USEPA, Low-impact development design strategies, An integrated design approach. Prince George's County, MD. Department of Environmental Resources. EPA-841-B-00-003, http://nepis.epa.gov/Exe/ZyPURL.cgi?Dockey=20004JX4.txt, (accessed July 2015).
-
USEPA, Low-impact development hydrolysis analysis. Prince George's County, MD. Department of Environmental Resources. EPA-841-B-00-002, http://nepis.epa.gov/Exe/ZyPURL.cgi?Dockey=20004JTW.txt, (accessed July 2015).
- W. Liu, W. Chen and C. Peng, Advances in low impact development technology for urban stormwater management, (English), Yingyong Shengtai Xuebao, 2015, 26(6), 1901–1912 Search PubMed.
- X. Wang, W. Shuster, C. Pal, S. Buchberger, J. Bonta and K. Avadhanula, Low impact development design- integrating suitability analysis and site planning for reduction of post-development stormwater quantity, Sustainability, 2010, 2(8), 2467–2482 CrossRef PubMed.
- A. Davis, M. Shokouhian, H. Sharma and C. Minami, Water quality improvement through bioretention media: Nitrogen and phosphorus removal, Water Environ. Res., 2006, 78(3), 284–293 CrossRef CAS.
- M. Dietz, Low impact development practices: A review of current research and recommendations for future directions, Water, Air, Soil Pollut., 2007, 186, 351–363 CrossRef CAS.
- D. Line and W. Hunt, Performance of a bioretention area and a level spreader-grass filter strip at two highway sites in North Carolina, J. Irrig. Drain. Div., Am. Soc. Civ. Eng., 2009, 135(2), 217–224 CrossRef.
- A. Roy-Poirier, P. Champagne and Y. Filion, Review of bioretention system research and design, past, present, and future, J. Environ. Eng., 2010, 136(9), 878–889 CrossRef CAS.
- J. Huang, L. Yu, N. Shuai and S. Zhou, Assessing the performances of low impact development alternatives by long-term simulation for a semi-arid area in Tianjin, northern China, Water Sci. Technol., 2014, 70(11), 1740–1745 CrossRef PubMed.
- B. Palanisamy and T. M. Chui, Rehabilitation of concrete canals in urban catchments using low impact development techniques, J. Hydrol., 2015, 523, 309–319 CrossRef PubMed.
-
USEPA, Urban Nonpoint Source Fact Sheet, http://water.epa.gov/polwaste/nps/urban_facts.cfm, (accessed July 2015).
- M. Scholz and P. Grabowiecki, Review of permeable pavement systems, Build. Sci., 2006, 42(11), 3830–3836 Search PubMed.
- E. Oberndorfer, J. Lundholm, B. Bass, R. Coffman, H. Doshi and B. Row,
et al., Green Roofs as Urban Ecosystems, Ecological Structures, Functions, and Services, Bioscience, 2007, 57(10), 823–833 CrossRef.
-
USEPA, Conserving Water, http://www.epa.gov/greenhomes/ConserveWater.htm, (accessed July 2015).
- E. Passeport, W. F. Hunt, D. E. Line, R. A. Smith and R. A. Brown, Field study of the ability of two grassed bioretention cells to reduce storm-water runoff pollution, J. Irrig. Drain. Div., Am. Soc. Civ. Eng., 2009, 135(4), 505–510 CrossRef.
- J. Stagge, A. Davis, E. Jamil and H. Kim, Performance of grass swales for improving water quality from highway runoff, Water Res., 2012, 46(20), 6731–6742 CrossRef CAS PubMed.
- E. Bedan and J. Clausen, Stormwater runoff quality and quantity from traditional and low impact development watersheds, J. Am. Water Resour. Assoc., 2009, 45(4), 998–1008 CrossRef CAS PubMed.
- S. Echols, Artful rainwater design in the urban landscape, J. Green. Build., 2007, 2(4), 103–122 Search PubMed.
- J. Brehm, D. Pasko and B. Eisenhauer, Identifying key factors in homeowner's adoption of water quality best management practices, Environ. Manage., 2013, 52(1), 113–122 CrossRef PubMed.
- USGS, Effects of low-impact-development (LID) practices on streamflow, runoff quantity, and runoff quality in the Ipswich River Basin, Massachusetts, A summary of field and modeling studies, http://pubs.usgs.gov/circ/1361/pdf/circ1361.pdf, (accessed July 2015).
-
Illinois Environmental Protection Agency. Illinois' nonpoint source management program, http://www.epa.state.il.us/water/watershed/publications/nps-management-program/index.pdf, (accessed July 2015).
- L. Ahiablame, B. Engel and I. Chaubey, Effectiveness of low impact development
practices in two urbanized watersheds: Retrofitting with rain barrel/cistern and porous pavement, J. Environ. Manage., 2013, 119, 151–161 CrossRef PubMed.
- J. Harbor, A practical method for estimating the impact of land-use change on surface runoff, groundwater, J. Am. Plann. Assoc., 1994, 60(1), 91–104 Search PubMed.
-
B. Engel, L-THIA NPS long-term hydrologic impact assessment and nonpoint source pollutant model, version 2.1, Purdue University and US Environmental Protection Agency, 2001 Search PubMed.
-
B. Engel and J. Hunter, L-THIA LID long-term hydrologic impact assessment low impact Development Model, Spreadsheet Version Purdue University, 2009 Search PubMed.
- NRCS (National Resource Conservation Service), Urban hydrology for small watersheds. Technical Release 55, USDA Natural Resources Conservation Service, 1986 Search PubMed.
-
S. Pandey, R. Gunn, K. J. Lim, B. Engel and J. Harbor, Developing a Web-enabled Tool to Assess Long-term Hydrologic Impacts of Landuse Change: Information Technology Issues and a Case Study, URISA, Washington DC, 2000, vol. 12, issue 4, pp. 5–22 Search PubMed.
- Z. Tang, B. A. Engel, B. C. Pijanowski and K. J. Lim, Forecasting land use change and its environmental impact at a watershed scale, J. Environ. Manage., 2005, 76(1), 35–45 CrossRef CAS PubMed.
- B. Bhaduri, J. Harbor, B. Engel and M. Grove, Assessing watershed-scale, long-term hydrologic impacts of land-use change using a GIS-NPS model, Environ. Manage., 2000, 26(6), 643–658 CrossRef CAS PubMed.
- Y. Kim, B. Engel, K. Lim, V. Larson and B. Duncan, Runoff impacts of land-use change in Indian River Lagoon watershed, J. Hydrol. Eng., 2002, 7(3), 245–251 CrossRef.
- K. Lim, B. Engel, Z. Tang, S. Muthukrishnan, J. Choi and K. Kim, Effects of calibration on L-THIA GIS runoff and pollutant estimation, J. Environ. Manage., 2006, 78(1), 35–43 CrossRef CAS PubMed.
- M. Grove, J. Harbor, B. Engel and S. Muthukrishnan, Impacts of urbanization on surface hydrology, Little Eagle Creek, Indiana, and analysis of L-THIA model sensitivity to data resolution, Phys. Geogr., 2001, 22, 135–153 Search PubMed.
- C. Wilson and Q. Weng, Assessing surface water quality and its relation with urban land cover changes in the Lake Calumet Area, Greater Chicago, Environ. Manage., 2010, 45, 1096–1111 CrossRef PubMed.
- J. L. Kyoung, B. Engel, S. Muthukrishnan and J. Harbor, Effects of initial abstraction and urbanization on estimated runoff using CN technology, J. Am. Water Resour. Assoc., 2006, 42(3), 629–644 CrossRef PubMed.
- D. Di Vittorio and L. Ahiablame, Spatial translation and scaling up of low impact development designs in an urban watershed, Journal of Water Management Modeling DOI:10.14796/JWMM.C388.
- Y. Liu, L. Ahiablame, V. Bralts and B. Engel, Enhancing a rainfall-runoff model to assess the impacts of BMPs and LID practices on storm runoff, J. Environ. Manage., 2015, 147, 12–23 CrossRef PubMed.
- J. Stagge, A. Davis, E. Jamil and H. Kim, Performance of grass swales for improving water quality from highway runoff, Water Res., 2012, 46(20), 6731–6742 CrossRef CAS PubMed.
- Y. Wang, W. Choi and B. Deal, Long-term impacts of land-use change on non-point source pollutant loads for the St. Louis metropolitan area, USA, Environ. Manage., 2005, 35(2), 194–205 CrossRef PubMed.
- L. Yang, M. Ke-Ming, G. Qing-Hai and B. Xue, Evaluating long-term hydrological impacts of regional urbanisation in Hanyang, China, using a GIS model and remote sensing, Int. J. Sust. Dev. World, 2008, 15(4), 350–356 CrossRef.
- E. Peters, Improving the practice of modeling urban hydrology, Stormwater, 2010, 11(2), 32–37 Search PubMed.
- City of Chicago. (2015), http://www.city-data.com/neighborhood/, (accessed July 2015).
- NCDC, http://http:www.ncdc.noaa.gov, (accessed July 2015).
- C. Chapman and R. Horner, Performance assessment of a street-drainage bioretention system, Water Environ. Res., 2010, 82(2), 109–119 CrossRef CAS.
- G. E. Moglen and S. Kim, Limiting Imperviousness, J. Am. Plann. Assoc., 2007, 73(2), 161–171 CrossRef PubMed.
-
USEPA (2000d), Effectiveness of low impact development proven LID technologies can work for your community, http://water.epa.gov/polwaste/green/upload/bbfs5effectiveness.pdf, (accessed July 2015).
- NRDC (Natural Resources Defense Council.) (2010). Re-envisioning the Chicago River: Adopting comprehensive regional solutions to the invasive species crisis, https://www.nrdc.org/water/Chicagoriver/files/Chicago%20River.pdf, (accessed July 2015).
|
This journal is © The Royal Society of Chemistry 2015 |
Click here to see how this site uses Cookies. View our privacy policy here.