DOI:
10.1039/C5QO00089K
(Research Article)
Org. Chem. Front., 2015,
2, 947-950
Sulfone promoted Rh(III)-catalyzed C–H activation and base assisted 1,5-H shift strategy for the construction of seven-membered rings†
Received
19th March 2015
, Accepted 5th June 2015
First published on 8th June 2015
Abstract
A facile synthesis of thiepine sulfones is described. It relies on a sequence of Rh(III)-catalyzed C–H cleavage, 1,5-H shift, and intramolecular allene insertion. As a result of extremely readily accessible starting materials and convenient operation, this protocol should be an appealing strategy in organic synthesis.
Introduction
Over the past few decades, directing group (DG) promoted transition-metal-catalyzed C–H activation has emerged as a versatile and efficient tool for the construction of cyclic compounds.1 With the chelation assistance of DG/metal, numerous C(sp2)–H activation-annulations have been successfully achieved.2,3 However, on the other hand, the chelation assistance limits the construction of cyclic compounds, because of the cyclometalated intermediate, generally with six to seven-membered sizes (Scheme 1a).4 Recently, Glorius's group and Zhao's group reported a rhodium catalyzed C–H activation/[4 + 3] annulation protocols, respectively, using α,β-unsaturated aldehydes (ketones) as the C3 sources (Scheme 1b).5 Herein we wish to report the realization of a new strategy in which the carbon–rhodium species, derived from ortho C–H activation, underwent an intramolecular insertion of the allene moiety in situ generated from a 1,5-H shift, affording thiepine sulfone derivatives (Scheme 1c).
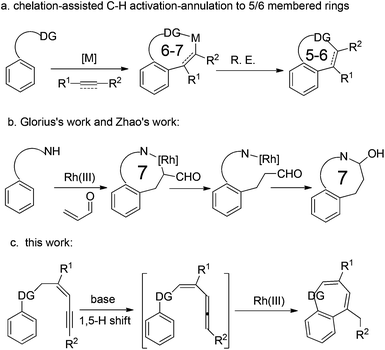 |
| Scheme 1 Proposal of Rh(III)-catalyzed C–H activation/intramolecular insertion of allene. | |
Results and discussion
Optimization of reaction conditions
On the basis of the understanding of base-assisted propargyl–allenyl isomerization,6 we initiated our studies by examining the reaction of 2-methyl-5-phenylpent-2-en-4-yn-1-yl phenyl sulfone (1a) in the presence of [(Cp*RhCl2)2] (0.025 eq.) and triethylamine (3 eq.) in toluene at 100 °C. Substrate 1a could be prepared readily via treatment of commercially available 5-bromo-4-methyl-1-phenylpent-3-en-1-yne with sodium benzenesulfinate and [Cp*RhIII] was chosen as the catalyst because of its high efficiency, mild reaction conditions and good functional group compatibility on C–H activation. This set of conditions afforded the desired product benzothiepine sulfone (2a) in 18% yield (Table 1, entry 1) and the structure of 2a was revealed by X-ray diffraction analysis (Fig. 1).7 We tested different additives in this system, and observed that Cu(OAc)2 (0.25 eq.) was the most efficient, affording 2a in 54% yield (Table 1, entry 8). Lowering the reaction temperature to 80 °C improved the yield slightly (Table 1, entry 9). Subsequent screening of solvents showed that dichloroethane was the best choice, giving 82% yield (Table 1, entry 11); and the use of other rhodium catalysts did not offer better results (Table 1, entries 15–17). Control experiments confirmed that the transformation did not occur in the absence of [(Cp*RhCl2)2] or triethylamine (Table 1, entries 18 and 19).
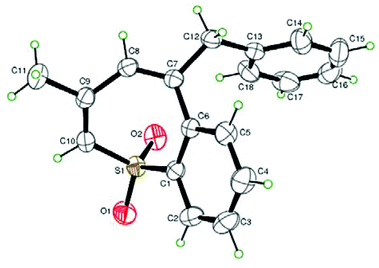 |
| Fig. 1 ORTEP representation of 2a. | |
Table 1 Optimization of the reaction conditionsa
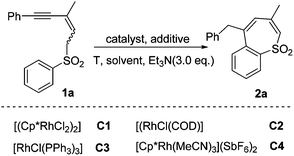
|
Entry |
Catalyst |
Additive (eq.) |
Solvent |
T (°C) |
Yield (%) |
Conditions: 1a (0.20 mmol), catalyst (0.025 eq.), Et3N (3 eq.), additive, and solvent (2.0 mL).
1a was recovered in 95% yield.
The reaction was conducted without triethylamine and 1a was recovered in 88% yield.
|
1 |
C1
|
— |
PhMe |
100 |
18 |
2 |
C1
|
AgOAc (0.5) |
PhMe |
100 |
25 |
3 |
C1
|
Ag2CO3 (0.5) |
PhMe |
100 |
22 |
4 |
C1
|
CuBr2 (0.5) |
PhMe |
100 |
25 |
5 |
C1
|
CuSO4 (0.5) |
PhMe |
100 |
19 |
6 |
C1
|
AgBF4 (0.5) |
PhMe |
100 |
28 |
7 |
C1
|
Cu(OAc)2 (0.5) |
PhMe |
100 |
48 |
8 |
C1
|
Cu(OAc)2 (0.25) |
PhMe |
100 |
54 |
9 |
C1
|
Cu(OAc)2 (0.25) |
PhMe |
80 |
61 |
10 |
C1
|
Cu(OAc)2 (0.25) |
PhMe |
60 |
41 |
11
|
C1
|
Cu(OAc)
2
(0.25)
|
DCE
|
80
|
82
|
12 |
C1
|
Cu(OAc)2 (0.25) |
CH3CN |
80 |
57 |
13 |
C1
|
Cu(OAc)2 (0.25) |
DMF |
80 |
28 |
14 |
C1
|
Cu(OAc)2 (0.25) |
1,4-Dioxane |
80 |
25 |
15 |
C2
|
Cu(OAc)2 (0.25) |
DCE |
80 |
15 |
16 |
C3
|
Cu(OAc)2 (0.25) |
DCE |
80 |
19 |
17 |
C4
|
Cu(OAc)2 (0.25) |
DCE |
80 |
43 |
18 |
— |
Cu(OAc)2 (0.25) |
DCE |
80 |
0b |
19 |
C1
|
Cu(OAc)2 (0.25) |
DCE |
80 |
0c |
Scope of the Rh(III)-catalyzed C–H activation
With the optimized conditions in hand, we examined the scope of this reaction and obtained polyfunctionalized benzothiepine sulfones (2) in moderate to good yields under mild conditions (Table 2). The R2 can be a phenyl group optionally substituted with either an electron-withdrawing or an electron-donating group (2b–2f), a naphthalenyl group (2g and 2h), or an alkyl group (2p and 2q). The substrates containing an electron-donating or electron-withdrawing group on the phenyl ring adjacent to sulfone also reacted efficiently (2h–2l).
Table 2 Synthesis of benzothiepine sulfonesa
Conditions: 1 (0.20 mmol), [(Cp*RhCl2)2] (0.005 mmol), Et3N (0.6 mmol), Cu(OAc)2 (0.05 mmol), and DCE (2.0 mL) at 80 °C.
|
|
Then our attention was diverted to the synthesis of heterocycle-annulated thiepine sulfones. The substrates with furanyl and pyrrolyl sulfone moieties decomposed under the standard conditions; while stable thiophenyl sulfone substrates reacted smoothly and afforded desired products in good yields (Table 3).
Table 3 Synthesis of thieno[2,3-b]thiepine sulfonesa
Conditions: 3 (0.20 mmol), [(Cp*RhCl2)2] (0.005 mmol), Et3N (0.6 mmol), Cu(OAc)2 (0.05 mmol), and DCE (2.0 mL) at 80 °C.
|
|
Mechanistic discussion
The C–H activation using sulfone as a coordinating directing group has not been well documented, probably due to its weak Lewis basicity.8 To probe the mechanism of the C–H activation–annulation, we prepared d5-1a for the control experiments. First, we measured the value of KH/D = 3.17, indicating that C–H bond cleavage is the rate-determining step [Scheme 2, (1)]. The KIE value was calculated by dividing the 1H NMR yields of the isotopic products after 6 h (approximation to initial rate ratios). Then, we conducted the reaction using d5-1a as the substrate under similar conditions. One of the ortho deuterium atoms on the benzene ring moved to the methylene group of 2a, demonstrating that an intramolecular insertion had occurred [Scheme 2, (2)].
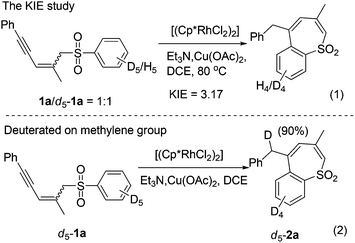 |
| Scheme 2 Deuterium labeling studies. | |
Based on these results, a plausible mechanism is proposed in Scheme 3. Coordination of sulfone 1a to a [Cp*RhIII] species for the C–H bond cleavage affords A, which experiences a base-assisted 1,5-H shift to form allene intermediate B.9 The rhodacycle moiety of intermediate B coordinates intramolecularly the allene moiety and undergoes insertion giving intermediate C.10 Subsequently, protonolysis of C delivers the product 2a.
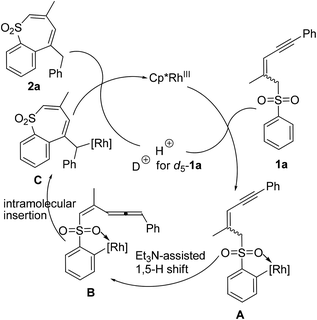 |
| Scheme 3 Plausible mechanism. | |
Conclusion
In conclusion, a sequence of Rh(III)-catalyzed C–H activation, 1,5-H shift, intramolecular allene insertion and protonolysis to afford thiepine sulfones has been realized. As a result of extremely readily accessible starting materials and the convenient operation, the protocol presented here should be an appealing strategy in organic synthesis. Further studies on the synthetic application are currently ongoing.
Acknowledgements
We are grateful to the National Natural Science Foundation of China (Project No. 20972134) and Zhejiang Provincial Natural Science Foundation of China (Project No. LY14B020008) for financial support.
Notes and references
- For selected reviews on C–H activation, see:
(a) P. L. Arnold, M. W. McMullon, J. Rieb and F. E. Kuhn, Angew. Chem., Int. Ed., 2015, 54, 82 CrossRef CAS PubMed;
(b) L. Ackermann, Acc. Chem. Res., 2014, 47, 281 CrossRef CAS PubMed;
(c) J. Wencel-Delord and F. Glorius, Nat. Chem., 2013, 5, 369 CrossRef CAS PubMed;
(d) N. Kuhl, M. N. Hopkinson, J. Wencel-Delord and F. Glorius, Angew. Chem., Int. Ed., 2012, 51, 10236 CrossRef CAS PubMed;
(e) S. R. Neufeldt and M. S. Sanford, Acc. Chem. Res., 2012, 45, 936 CrossRef CAS PubMed;
(f) J. Yamaguchi, A. D. Yamaguchi and K. Itami, Angew. Chem., Int. Ed., 2012, 51, 8960 CrossRef CAS PubMed;
(g) K. M. Engle, T. Mei, M. Wasa and J. Yu, Acc. Chem. Res., 2012, 45, 788 CrossRef CAS PubMed;
(h) M. C. White, Science, 2012, 335, 807 CrossRef CAS PubMed;
(i) C. S. Yeung and V. M. Dong, Chem. Rev., 2011, 111, 1215 CrossRef CAS PubMed;
(j) L. Ackermann, Chem. Rev., 2011, 111, 1315 CrossRef CAS PubMed.
- For selected reviews on chelation-assisted C(sp2)–H activation, see:
(a) B. Li and P. H. Dixneuf, Chem. Soc. Rev., 2013, 42, 5744 RSC;
(b) G. Song, F. Wang and X. Li, Chem. Soc. Rev., 2012, 41, 3651 RSC;
(c) J. Wencel-Delord, T. Drçge, F. Liu and F. Glorius, Chem. Soc. Rev., 2011, 40, 4740 RSC;
(d) S. Conejero, M. Paneque, M. L. Poveda, L. L. Santos and E. Carmona, Acc. Chem. Res., 2010, 43, 572 CrossRef CAS PubMed.
- For selected references on ortho-C–H activation, see:
(a) K. Nobushige, K. Hirano, T. Satoh and M. Miura, Org. Lett., 2014, 16, 1188 CrossRef CAS PubMed;
(b) B. Wang, C. Shen, J. Yao, H. Yin and Y. Zhang, Org. Lett., 2014, 16, 46 CrossRef CAS PubMed;
(c) T. Wesch, A. Berthelot-Bréhier, F. R. Leroux and F. Colobert, Org. Lett., 2013, 15, 2490 CrossRef CAS PubMed;
(d) T. Wesch, R. L. Frédéric and F. Colobert, Adv. Synth. Catal., 2013, 355, 2139 CrossRef CAS PubMed;
(e) A. García-Rubia, M. Á. Fernández-Ibáñez, R. G. Arrayás and J. C. Carretero, Chem. – Eur. J., 2011, 17, 3567 CrossRef PubMed.
- Cui's group proposed recently an eight-membered rhodacycle intermediate, see:
(a) S. Cui, Y. Zhang and Q. Wu, Chem. Sci., 2013, 4, 3421 RSC;
(b) S. Cui, Y. Zhang, D. Wang and Q. Wu, Chem. Sci., 2013, 4, 391 Search PubMed.
-
(a) Z. Shi, C. Grohmann and F. Glorius, Angew. Chem., Int. Ed., 2013, 52, 5393 CrossRef CAS PubMed;
(b) P. Duan, X. Lan, Y. Chen, S. Qian, J. J. Li, L. Lu, Y. Lu, B. Chen, M. Hong and J. Zhao, Chem. Commun., 2014, 50, 12135 RSC.
-
(a) L. Liu, D. Chen and H. Zhou, Adv. Synth. Catal., 2015, 357, 389 CrossRef CAS PubMed;
(b) G. Zhao, Q. Zhang and H. Zhou, J. Org. Chem., 2014, 79, 10867 CrossRef CAS PubMed;
(c) G. Zhao, Q. Zhang and H. Zhou, Adv. Synth. Catal., 2013, 355, 3492 CrossRef CAS PubMed;
(d) H. Zhou, L. Liu and S. Xu, J. Org. Chem., 2012, 77, 9418 CrossRef CAS PubMed;
(e) H. Zhou, Y. Xing, J. Yao and Y. Lu, J. Org. Chem., 2011, 76, 4582 CrossRef CAS PubMed;
(f) H. Zhou, Y. Xie, L. Ren and R. Su, Org. Lett., 2010, 12, 356 CrossRef CAS PubMed;
(g) H. Zhou, D. Zhu, Y. Xie, H. Huang and K. Wang, J. Org. Chem., 2010, 75, 2706 CrossRef CAS PubMed;
(h) H. Zhou, Y. Xing, J. Yao and J. Chen, Org. Lett., 2010, 12, 3674 CrossRef CAS PubMed.
- CCDC 1050464.
- S. De Sarkar, W. Liu, S. I. Kozhushkov and L. Ackermanna, Adv. Synth. Catal., 2014, 356, 1461 CrossRef CAS PubMed.
-
(a) J. Cao, X. Yang, X. Hua, Y. Deng and G. Lai, Org. Lett., 2011, 13, 478 CrossRef CAS PubMed;
(b) K. Mori, T. Kawasaki, S. Sueoka and T. Akiyama, Org. Lett., 2010, 12, 1732 CrossRef CAS PubMed;
(c) S. Murarka, I. Deb, C. Zhang and D. Seidel, J. Am. Chem. Soc., 2009, 131, 13226 CrossRef CAS PubMed;
(d) P. A. Vadola and D. Sames, J. Am. Chem. Soc., 2009, 131, 16525 CrossRef CAS PubMed;
(e) F. Sha and X. Huang, Angew. Chem., Int. Ed., 2009, 48, 3458 CrossRef CAS PubMed;
(f) J. Barluenga, M. Fananas-Mastral, F. Aznar and C. Valdes, Angew. Chem., Int. Ed., 2008, 47, 6594 CrossRef CAS PubMed.
-
(a) K. M. Brummond, H. Chen, B. Mitasev and A. D. Casarez, Org. Lett., 2004, 6, 2161 CrossRef CAS PubMed;
(b) S. Ma and E. Negishi, J. Org. Chem., 1994, 59, 4730 CrossRef CAS.
Footnote |
† Electronic supplementary information (ESI) available. CCDC 1050464. For ESI and crystallographic data in CIF or other electronic format see DOI: 10.1039/c5qo00089k |
|
This journal is © the Partner Organisations 2015 |