DOI:
10.1039/C6RA05168E
(Paper)
RSC Adv., 2016,
6, 44646-44654
Radiolabeled pyridinyl analogues of dibenzylideneacetone as β-amyloid imaging probes†
Received
27th February 2016
, Accepted 18th April 2016
First published on 20th April 2016
Abstract
In continuation of our investigation of the dibenzylideneacetone scaffold as Aβ imaging probes, a series of derivatives containing pyridine rings with lower lipophilicity was synthesized and evaluated. Some of these probes displayed high affinities to Aβ1–42 aggregates, which ranged from 18.0 to 8.2 nM. The high and specific binding of the radiolabeled tracers was further confirmed by in vitro autoradiography on brain sections from AD patients and transgenic mouse. In biodistribution experiments, two 18F-labeled tracers [18F]17a and [18F]18 displayed high initial brain uptake (5.05 and 6.24% ID g−1, respectively, at 2 min postinjection) and fast clearance from the brain with brain2 min/brain60 min ratios of 6.08 and 8.00. These results demonstrate that the probes [18F]17a and [18F]18 with a dibenzylideneacetone structure containing pyridine rings have great strength in imaging Aβ plaques in the brain.
Introduction
As the world's population ages, Alzheimer's disease (AD), which results in a devastating neurodegenerative disorder, is causing enormous socioeconomic costs and effects.1,2 So far, it has been proved that AD is incurable, and the medications can only provide temporary relief from symptoms and cannot stop or reverse the underlying course of AD. According to the amyloid cascade hypothesis, the deposition of extracellular β-amyloid (Aβ) plaques is a major neuropathological hallmark of AD.3 Therefore, it is of great importance to precisely detect the Aβ plaques in preclinical AD with the help of noninvasive techniques, such as positron emission tomography (PET) or single photon emission computed tomography (SPECT).4,5
Over the past decades, a number of radiolabeled probes have been developed for imaging Aβ plaques. Among them, PET imaging agents, including 11C-labeled tracers like [11C]PIB,6,7 [11C]SB-13,8 [11C]BF-227,9 and 18F-labeled tracers such as [18F]FDDNP,10 [18F]AV-45,11–13 [18F]BAY94-9172
14 and [18F]GE-067,15 have achieved marvellous progress so far. However, shortcomings still exist; for example, the 18F-labeled tracers have high non-specific binding in white matter. Compared to PET, the development of SPECT lags far behind. [123I]IMPY,16,17 as the first SPECT Aβ imaging agent, failed to be translated to clinical use owing to its high lipophilicity, in vivo instability and poor signal-to-noise ratio in SPECT imaging. Therefore, more effort is necessary to design smarter PET or SPECT imaging probes with better in vivo biological properties.
Curcumin is known for its inhibition of the aggregation of Aβ fibrils, formation of oligomers and reduction of amyloids in vivo.18 Cui et al. focused on structural modifications and synthesized a series of dibenzylideneacetones.19–21 Most of the derivatives displayed high affinities to Aβ aggregates, and several probes showed high initial brain uptake, but displayed slow washout rates from the mouse brain, which is caused by the high lipophilicity. Inspired by previous experiences, we attempted to develop more efficient radiolabeled probes targeting Aβ plaques. To improve the hydrophilicity and thus reduce the non-specific binding to the white matter in the brain, we replaced the phenyl rings of the dibenzylideneacetone scaffold with pyridinyl rings. Herein, we designed and synthesized 18F-labeled and 125I-labeled pyridine dibenzylideneacetones derivatives and evaluated them as radiolabeled tracers for Aβ plaques in the brain.
Results and discussion
Chemistry
The key synthesis steps of these pyridine dibenzylideneacetone derivatives are outlined in Scheme 1. The final products 15a–17a, 15b–17b, 15c–17c, 18 and 19 were obtained via the base-catalyzed Claisen condensation reaction from fluoropegylated (FPEG), fluorinated or iodinated aromatic aldehydes in yields of 20–60%. The FPEG (n = 2, 3) modified aldehyde intermediates (6, 7, 13 and 14) were obtained by fluorination of the tosylate esters (3, 4, 10 and 11) using anhydrous tetra-n-butylammonium fluoride in THF. In addition, the fluorinated intermediates (5 and 12) with short ethoxy units (n = 1) were prepared by nucleophilic substitutions from 2-fluoroethanol or 1-bromo-2-fluoroethane. All of these key compounds were fully characterized by spectroscopic methods and verified with 1H/13C NMR and MS (EI), and the purities were determined to be higher than 95% by high-performance liquid chromatography (HPLC) (Table S1 in ESI†).
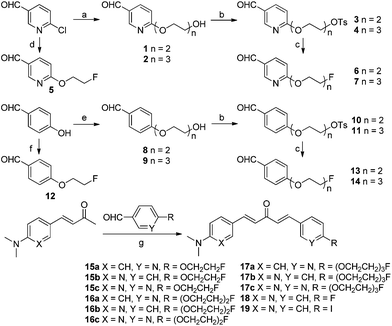 |
| Scheme 1 Reagents and conditions: (a) 2,2′-oxydiethanol or 2,2′-(propane-1,3-diylbis(oxy))diethanol, CH2Cl2, t-BuOK, r.t. (b) TsCl, CH2Cl2, triethylamine, r.t. (c) TBAF (1 M in THF), THF, 80 °C. (d) 2-Fluoroethanol, CH2Cl2, t-BuOK, r.t. (e) 2-(2-Chloroethoxy)ethanol or 2-(3-(2-chloroethoxy)propoxy)ethanol, KOH, EtOH, 80 °C. (f) 1-Bromo-2-fluoroethane, KOH, EtOH, 80 °C. (g) NaOMe (28% in MeOH), MeOH, r.t. | |
Binding studies using Aβ aggregates in solution
In vitro competition binding assay was carried out using [125I]IMPY as the competing radioligand to quantitatively evaluate the binding affinities of these pyridine dibenzylideneacetone derivatives for Aβ1–42 aggregates. IMPY was also screened under the same assay as control. The results are shown in Table 1. In comparison with the results of the dibenzylideneacetone derivatives reported by us previously, the incorporation of a pyridine ring had a generally negative effect on the binding affinity. Much experimental data has demonstrated that the introduction of an N,N-dimethylamino group to the phenyl ring is favorable for the binding affinity because the N,N-dimethylaniline moiety could insert to the hydrophobic binding domain of Aβ fibers through hydrophobic interaction.5,22 When compared with 15a–17a, whose N,N-dimethylamino groups were attached to the phenyl ring, ligands 15b–17b with an N,N-dimethylamino group on the pyridine ring displayed decreased affinity. The affinity was further decreased when both phenyl rings in the dibenzylideneacetone backbone were replaced by pyridine rings (15c–17c). In addition, the length of the FPEG chains has a slight effect on the binding affinity when an N,N-dimethylamino group is attached to the phenyl ring (15a–17a). However, for 15b–17b and 15c–17c, when N,N-dimethylamino groups were attached to the pyridine rings, the affinities of these ligands gradually decreased with the extension of FPEG chains. Finally, one iodinated (19, Ki = 18.0 ± 3.5 nM) and two fluorinated compounds (17a, Ki = 11.3 ± 2.1 nM; 18, Ki = 8.2 ± 0.7 nM), which exhibited comparable affinities to that of IMPY (Ki = 16.9 ± 6.7 nM), were selected for radiolabeling and further biological evaluations.
Table 1 Inhibition constants for the binding of [125I]IMPY to Aβ1–42 aggregatesa
Compd |
X |
Y |
R |
Ki (nM) |
Measured in triplicate with values given as the mean ± SD. |
15a |
CH |
N |
OCH2CH2F |
26.6 ± 4.9 |
15b |
N |
CH |
27.3 ± 4.5 |
15c |
N |
N |
60.8 ± 6.7 |
16a |
CH |
N |
(OCH2CH2)2F |
27.3 ± 4.5 |
16b |
N |
CH |
44.2 ± 3.3 |
16c |
N |
N |
50.3 ± 5.2 |
17a |
CH |
N |
(OCH2CH2)3F |
11.3 ± 2.1 |
17b |
N |
CH |
54.8 ± 4.7 |
17c |
N |
N |
163.9 ± 21.4 |
18 |
N |
CH |
F |
8.2 ± 0.7 |
19 |
N |
CH |
I |
18.0 ± 3.5 |
IMPY |
— |
— |
— |
16.9 ± 6.7 |
Radiochemistry
The fluorinated ligands (17a and 18) and iodinated ligand 19 with high binding affinities were selected for radiolabeling and further biological evaluations. The FPEG (n = 3) modified dibenzylideneacetone [18F]17a was prepared from the tosylate precursor 21 with [18F]fluoride/potassium carbonate and Kryptofix 222 in acetonitrile under heating at 100 °C for 5 min with a radiochemical yield of 19.8% (Scheme 2). For [18F]18 with the 18F atom directly attached to the benzene ring, a two-step strategy was applied. The 18F-labeled intermediate [18F]-4-fluorobenzaldehyde was prepared from the trimethyl ammonium iodide precursor. After [18F]fluorination, the crude product was loaded on a Sep-Pak Plus-C18 cartridge to remove inorganic salts and dimethyl sulfoxide (DMSO), and the [18F]-4-fluorobenzaldehyde was eluted by ethanol; finally, (E)-4-(6-(dimethylamino)pyridin-3-yl)but-3-en-2-one and NaOH were added to proceed the Claisen condensation for 20 min at 60 °C. The overall radiochemical yield was calculated to be 12.2% (Scheme 2). The radioiodinated ligand [125I]19 was obtained from the corresponding tributyltinprecursor 22 by an iododestannylation reaction with hydrogen peroxide as the oxidant in a radiochemical yield of 32.2% (Scheme 3). After purification by HPLC, the radiochemical purities of these radiotracers were higher than 95% and their radiochemical identities were verified by comparison of the retention times with that of the nonradioactive ligands (Fig. 1).
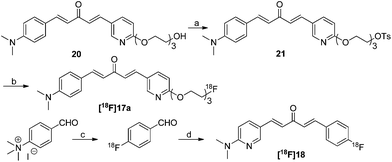 |
| Scheme 2 Reagents and conditions: (a) TsCl, CH2Cl2, triethylamine, r.t. (b) [18F]−, K2CO3, Kryptofix 222, DMSO, 120 °C. (c) [18F]−, K2CO3, Kryptofix 222, DMSO, 150 °C. (d) (E)-4-(6-(Dimethylamino)pyridin-3-yl)but-3-en-2-one, NaOH, EtOH, 60 °C. | |
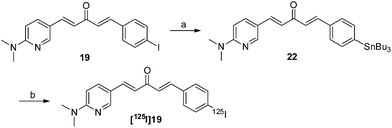 |
| Scheme 3 Reagents and conditions: (a) (Bu3Sn)2, (Ph3P)4Pd, toluene, reflux. (b) (1) [125I]NaI, HCl (1 M), H2O2 (3%); (2) NaHSO3, NaHCO3. | |
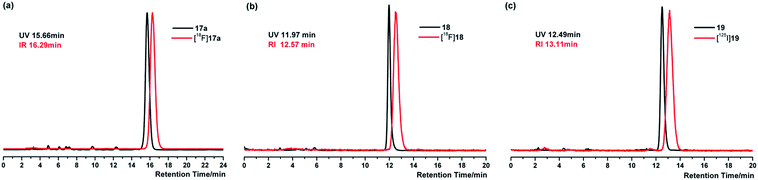 |
| Fig. 1 HPLC profiles of the purified radiotracers of [18F]17a, [18F]18 and [125I]19 (red line) and the nonradioactive ligands (17a, 18 and 19, black line). HPLC conditions: Venusil MP C18 column (Bonna-Agela Technologies, 4.6 mm × 250 mm), CH3CN/H2O = 60/40 for (a), CH3CN/H2O = 65/35 for (b), CH3CN/H2O = 70/30 for (c), 1 mL min−1, UV = 254 nm. | |
Biodistribution studies
Partition coefficient measurements and biodistribution experiments in normal ICR mice (20–22 g, male) were performed to assess the pharmacokinetics of these radiotracers. The log
D values of [18F]17a, [18F]18, and [125I]19 were measured to be 2.36 ± 0.09, 3.14 ± 0.03 and 3.31 ± 0.31, respectively, which was between 2 and 3.5, demonstrating that they have suitable lipophilicity to penetrate the blood–brain barrier (BBB) as brain imaging agents. As shown in Table 2, both of the two 18F-labeled ligands displayed excellent initial BBB penetration with brain uptakes higher than 5% ID g−1 at 2 min post-injection, which was comparable to [18F]AV-45 (6.59 ± 0.02% ID g−1) under the same experimental conditions. Furthermore, [18F]17a and [18F]18 (brain2 min/brain60 min = 6.08, 8.00, respectively) displayed faster washout rates than [18F]AV-45 (brain2 min/brain60 min = 3.89) from the brain, which is highly desirable for Aβ imaging probes. As compared with 18FPEG modified dibenzylideneacetone derivatives reported previously (4.1–5.2% ID g−1 at 2 min, brain2 min/brain60 min = 4.1–4.6),19,21 [18F]17a and [18F]18 displayed improved washout rates from the brain. In common with the 125I-labeleddibenzylideneacetone reported before,19,21 [125I]19 with a pyridine ring also exhibited low initial brain uptake (2.06% ID g−1 at 2 min post-injection), which may result from the high lipophilicity when incorporating the iodine atom into the molecule's structure. In addition, lower uptake in blood and liver of these pyridinyl modified dibenzylideneacetones was observed than that of dibenzylideneacetones reported previously,19,21 suggesting that their lipophilicity was subsequently reduced and may result in better signal-to-noise ratios with lower nonspecific uptake. Low levels of bone uptake (2.50 and 3.87% ID g−1 at 1 h post-injection) indicate that [18F]17a and [18F]18 had low defluorination. In conclusion, the excellent pharmacokinetics of the two 18F-labeled ligands indicated that the introduction of pyridine actually increased the clearance rate from the healthy brain and resulted in lower non-specific binding to white matter, which may contribute to improving the sensitivity of diagnosis (Table S2 in ESI†).
Table 2 Biodistribution of [18F]17a, [18F]18 and [125I]19 in normal ICR micea
Organ |
2 min |
10 min |
30 min |
60 min |
Expressed as % injected dose per gram. Each value represents the mean ± SD for 5–6 mice. |
[18F]17a (log D = 2.36 ± 0.09) |
Blood |
3.17 ± 0.22 |
2.06 ± 0.19 |
1.59 ± 0.18 |
1.35 ± 0.12 |
Brain |
5.05 ± 0.55 |
1.80 ± 0.25 |
0.98 ± 0.17 |
0.83 ± 0.06 |
Bone |
2.04 ± 0.36 |
1.15 ± 0.34 |
1.49 ± 1.02 |
2.50 ± 0.42 |
![[thin space (1/6-em)]](https://www.rsc.org/images/entities/char_2009.gif) |
[18F]18 (log D = 3.14 ± 0.03) |
Blood |
2.57 ± 0.13 |
2.29 ± 0.13 |
2.12 ± 0.45 |
1.28 ± 0.34 |
Brain |
6.24 ± 0.27 |
3.35 ± 0.32 |
1.35 ± 0.05 |
0.78 ± 0.07 |
Bone |
2.66 ± 0.14 |
2.36 ± 0.15 |
3.78 ± 0.51 |
3.87 ± 0.62 |
![[thin space (1/6-em)]](https://www.rsc.org/images/entities/char_2009.gif) |
[125I]19 (log D = 3.31 ± 0.31) |
Blood |
3.71 ± 0.75 |
1.80 ± 0.16 |
1.33 ± 0.21 |
1.02 ± 0.22 |
Brain |
2.06 ± 0.16 |
1.72 ± 0.15 |
1.36 ± 0.18 |
1.04 ± 0.17 |
Thyroid |
0.07 ± 0.004 |
0.04 ± 0.01 |
0.03 ± 0.004 |
0.04 ± 0.01 |
In vitro autoradiography
To confirm the binding affinities of these 18F-labeled derivatives to Aβ plaques, we employed qualitative evaluation with in vitro autoradiography on brain sections of AD patients and transgenic (Tg) model mice further. As shown in Fig. 2 and 3, notable labeling of Aβ plaques on sections of Tg mice and AD patients were observed, while the brain sections of the control mouse and normal people showed no such labeling. The location of accumulated radioactivity for the distribution of these Aβ plaques was consistent with the results of fluorescent staining with thioflavin-S. All these results demonstrated that these two derivatives had high affinities and specificity to Aβ plaques.
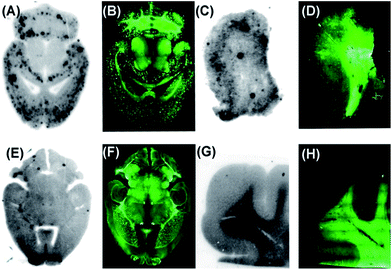 |
| Fig. 2 In vitro autoradiography of [18F]17a in mouse brain sections ((A) Tg, C57BL6, APPswe/PSEN1, 11 months old; (E) wild-type, C57BL6, 11 months old) and human brain sections ((C) AD, 91 years old, male; (G) normal, 74 years old, male). The presence and distribution of plaques in the sections were confirmed with thioflavin-S (B, D, F and H). | |
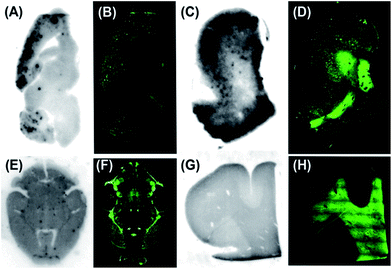 |
| Fig. 3 In vitro autoradiography of [18F]18 in mouse brain sections ((A) Tg, C57BL6, APPswe/PSEN1, 11 months old; (E) wild-type, C57BL6, 11 months old) and human brain sections ((C) AD, 91 years old, male; (G) normal, 74 years old, male). The presence and distribution of plaques in the sections were confirmed with thioflavin-S (B, D, F and H). | |
Ex vivo autoradiography
To further confirm the specific binding of 18F-labeled ligands to Aβ plaques in vivo, [18F]18 with better brain kinetics was selected for ex vivo autoradiographic studies in Tg mice and age-matched wild-type mice. As shown in the autoradiographic image (Fig. 4), distinct labeling of Aβ plaques in the cortex regions of Tg mouse brain slices was observed, while no such labeling could be found in the wild-type mouse brain. The labeling of the plaques was further confirmed by fluorescent staining with thioflavin-S. These results demonstrated that [18F]18 did penetrate the intact BBB and bind to Aβ plaques specifically in vivo.
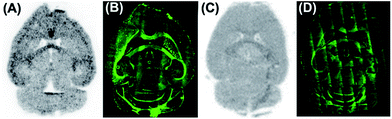 |
| Fig. 4 Ex vivo autoradiography of [18F]18 in Tg mouse ((A) C57BL6, APPswe/PSEN1, male, 24 months old) and an age-matched wild-type mouse ((C) C57BL6, male, 24 months old). The Aβ plaques were confirmed by in vitro containing the same brain sections with thioflavin-S (B and D). | |
Conclusions
In conclusion, we successfully synthesized eleven pyridinyl analogues of dibenzylideneacetone and three of them were selected to label with radionuclides 18F and 125I. In vitro binding studies showed that these complexes displayed high affinities for Aβ aggregates (ranged from 8.2 to 18.0 nM). Biodistribution experiments in normal mice indicated that the two 18F-labeled tracers displayed high initial uptake and fast clearance from the brain, which is suitable for Aβ imaging agents. In in vitro autoradiography on brain sections from AD brain and Tg mouse, the Aβ plaques were significantly labelled by these two 18F-labeled tracers, which confirmed their high affinities and specificity. In ex vivo autoradiography studies, [18F]18 could penetrate the intact BBB and bind to Aβ plaques specifically in vivo. Taken together, the preliminary results suggest that [18F]18 may be a potential PET probe for imaging Aβ plaques in the brain. Furthermore, additional chemical refinements of the dibenzylideneacetone are required to develop more promising Aβ imaging agents for both PET and SPECT in the diagnosis of AD.
Experimental
All of the chemicals used for synthesis were commercial products employed without further purification. [125I]NaI was purchased from PerkinElmer. 1H NMR (400 MHz) and 13C NMR (101 MHz) spectra were obtained on Bruker Avance III NMR spectrometers in CDCl3 solution at room temperature with trimethylsilyl (TMS) as an internal standard. High-resolution mass spectrometry (HRMS) and mass spectra (MS) were obtained using a Surveyor MSQ Plus (ESI) (Waltham, MA, USA) instrument. HPLC was performed on a Shimadzu SCL-20 AVP system equipped with a Bioscan flow count 3200 NaI/PMT γ-radiation scintillation detector and a SPD-20A UV detector (λ = 254 nm). The HPLC separations were achieved on a Venusil MP C18 column (Bonna-Agela Technologies, 5 μm, 10 mm × 250 mm) eluted with a binary gradient system at a flow rate of 4.0 mL min−1. Mobile phase A was water, while mobile phase B was acetonitrile. Fluorescent observation was performed on an inverted fluorescence microscope (Axio Observer Z1, Zeiss) equipped with a GFP filter set (excitation, 505 nm). Normal ICR mice (5 weeks, male) used for biodistribution experiments were obtained from Vital River Laboratory Animal Technology Co. Ltd. Post-mortem brain tissues from an autopsy-confirmed AD patient (91 years old, male), and a control subject (74 years old, male) were obtained from the Chinese Brain Bank Center. Tg mice (APPswe/PSEN1, male, 24 months old) and wild-type mice (C57BL6, male, 24 months old) were purchased from the Institute of Laboratory Animal Science, Chinese Academy of Medical Sciences. All protocols requiring the use of mice were approved by the animal care committee of Beijing Normal University. The purity of all key compounds was proven to be more than 95% by HPLC.
Radiolabeling
The method of dealing with [18F]fluoride was reported previously.23 To obtain [18F]17a, a solution of the tosylate precursor 21 (3.0 mg) in acetonitrile (1 mL) was added to the reaction vessel containing 18F activity. The mixture was heated at 120 °C for 5 min. Deionized water (10 mL) was added, and the mixture was passed through a preconditioned Sep-Pak Plus-C18 cartridge (Bonna-Agela Technologies). The cartridge was washed with 10 mL of deionized water, and the labeled compound was eluted with 1 mL of acetonitrile. The eluent was concentrated under the flow of nitrogen and purified by HPLC (Venusil MP C18 column, 5 μm, 10 × 250 mm, CH3CN/water = 60/40, flow rate = 4 mL min−1). The radiochemical yield was 19.8% (decay corrected). For [18F]18, a solution of 4-formyl-N,N,N-trimethylbenzenaminiumiodide (5.0 mg) in DMSO (1 mL) was added to the reaction vessel containing the 18F activity. The mixture was heated at 150 °C for 5 min. The [18F]4-fluorobenzaldehyde was purified by Sep-Pak Plus-C18 cartridge (Bonna-Agela Technologies) as described above, and was eluted with 1 mL of ethanol. Then (E)-4-(6-(dimethylamino)pyridin-3-yl)but-3-en-2-one (5.0 mg) and NaOH (4.0 mg) were added to the elution, and the mixture was maintained for 20 min at 60 °C. After adjusting pH to 7.0 with acetic acid, the mixture was purified by HPLC (Venusil MP C18 column, 5 μm, 10 × 250 mm, CH3CN/water = 65/35, flow rate = 4 mL min−1). The radiochemical yield was 12.2% (decay corrected).
For [125I]19, a mixture of tributyltin precursor 22 (0.1 mg in 100 μL of ethanol), [125I]NaI (2 μL, PerkinElmer), H2O2 (100 μL, 3%) and HCl (100 μL, 1 M) was maintained at room temperature for 10 min. Then saturated NaHSO3 (50 μL) and NaHCO3 were added successively. After purification by HPLC (Venusil MP C18 column, 5 μm, 4.6 × 250 mm, CH3CN/water = 70/30, flow rate = 1 mL min−1), the radiochemical purity of these ligands was greater than 98% and the overall radiochemical yield was 32.2%.
In vitro binding assay using Aβ aggregates
Peptides Aβ1-42 were purchased from Osaka Peptide Institute. We performed the aggregation by following the procedure described previously.23 A final volume of 1 mL reaction mixture contained 100 μL of Aβ1-42 aggregates (28 nM in the final assay mixture), 100 μL of radioligand ([125I]IMPY, 100
000 cpm/100 μL), 100 μL of different concentrations of inhibitors (10−4 to 10−8.5 M in ethanol), and 700 μL of PBS containing 0.5% BSA. After incubation for 3 h at room temperature, the mixture was filtered through borosilicate glass fiber filters (Whatman GF/B) with an Mp-48T cell harvester (Brandel, Gaithersburg, MD). Filters containing the bound [125I]IMPY were examined in an automatic γ-counter (WALLAC/Wizard1470, PerkinElmer) with 70% efficiency. The half-maximal inhibitory concentration (IC50) was determined by the displacement curves of three independent experiments using GraphPad Prism 5.0. Then the inhibition constant (Ki) was calculated according to the Cheng–Prusoff equation.
Determination of the partition coefficient
The determination of partition coefficients of [18F]17a, [18F]18 and [125I]19 was performed by following the procedures previously reported.23 A solution of the radiotracer (50 μCi in ethanol) was added to premixed suspensions containing 3.0 g of n-octanol and 3.0 g of PBS (0.05 M, pH 7.4) in a test tube, which was vortexed for 5 min at room temperature and then centrifuged for 5 min at 3000 rpm. Two weighted samples from the n-octanol (50 μL) and PBS (500 μL) layers were measured by automatic γ-counter. The partition coefficient was expressed as the logarithm of the ratio of the count per gram from n-octanol versus PBS. Samples from the n-octanol layer were repartitioned until consistent partition coefficient values were obtained. The measurement was done in triplicate and repeated three times.
Biodistribution studies
The biodistribution experiments were performed in normal male mice (ICR, 5 weeks, male). A saline solution containing the HPLC purified radiolabeled tracer (100 μL, 10% ethanol, 2–7 μCi) was injected directly into the tail vein. The mice (n = 5 for each time point) were sacrificed by decapitation exactly at 2, 10, 30 and 60 min after injection. Samples of blood and organs of interest were collected and weighed, and radioactivity was measured with an automatic γ counter (WALLAC/Wizard1470, PerkinElmer). The results were expressed in terms of the percentage of the injected dose per gram (% ID g−1) of blood or organs.
In vitro autoradiography studies
Paraffin-embedded brain sections were deparaffinized in xylene for 5 min, then washed with 100% ethanol and 40% ethanol/H2O. After that, the brain sections were incubated with 18F-labeled tracers (6 μCi per 100 μL) at room temperature for 40 min, then washed with 40% EtOH. After drying, the sections were exposed to a phosphorus plate (type: SR, PerkinElmer) for 1–3 h. In vitro autoradiographic images were obtained using a Cyclone storage phosphor system (Packard). After autoradiographic examination, the same sections were stained by thioflavin-S to confirm the presence of Aβ plaques.
Ex vivo autoradiography studies
A saline solution of [18F]18 (600 μCi per 400 μL) was injected directly into the tail vein of a Tg mouse (C57BL6, APPswe/PSEN1, male, 24 months old) and a wild-type mouse (C57BL6, male, 24 months old). The mice were sacrificed by decapitation at 20 min after intravenous injection. The brains were immediately removed and frozen at −20 °C. Sections of 20 μm were cut and exposed to a phosphorus plate for 3–4 h. In vitro autoradiographic images were obtained using a Cyclone storage phosphor system (PerkinElmer, USA). After autoradiographic examination, the same sections were stained by thioflavin-S to confirm the presence of Aβ plaques.
Chemical synthesis
6-(2-(2-Hydroxyethoxy)ethoxy)nicotinaldehyde (1). A solution of 2,2′-oxydiethanol (1.06 g, 10 mmol) and t-BuOK (1.12 g, 10 mmol) in CH2Cl2 (10 mL) was stirred for 30 min at room temperature, then 6-chloronicotinaldehyde (1.42 g, 10 mmol) was added to the mixture slowly. The resulting mixture was stirred at room temperature for an additional 3 h, then the solvent was evaporated under reduced pressure. After 50 mL of water was added, the mixture was extracted by CH2Cl2 (3 × 10 mL). Combined organic layers were dried over anhydrous MgSO4, filtered and concentrated under vacuum. The residue was purified by silica gel chromatography (petroleum ether/AcOEt = 1/1, v/v), and 1 was obtained as a light yellow waxy solid in a yield of 19.2%. 1H NMR (400 MHz, CDCl3) δ 9.95 (s, 1H), 8.61 (d, J = 2.2 Hz, 1H), 8.07 (dd, J = 8.6, 2.3 Hz, 1H), 6.89 (d, J = 8.7 Hz, 1H), 4.62–4.59 (m, 2H), 3.90–3.87 (m, 2H), 3.78–3.75 (m, 2H), 3.68–3.65 (m, 2H). MS (ESI): m/z calcd for C10H13NO4, 211.1; found 212.3 (M + H+).
6-(2-(2-(2-Hydroxyethoxy)ethoxy)ethoxy)nicotinaldehyde (2). The procedure described above for the preparation of 1 was used and 2 was obtained as a light yellow waxy solid in a yield of 43.0%. 1H NMR (400 MHz, CDCl3) δ 9.95 (s, 1H), 8.61 (dd, J = 2.8, 0.8 Hz, 1H), 8.06 (dd, J = 8.8, 2.4 Hz, 1H), 6.89 (td, J = 1.6, 0.8 Hz, 1H), 4.61–4.59 (m, 2H), 3.77–3.70 (m, 6H), 3.70–3.61 (m, 2H). MS (ESI): m/z calcd for C12H17NO5, 255.1; found 256.3 (M + H+).
2-(2-((5-Formylpyridin-2-yl)oxy)ethoxy)ethyl 4-methylbenzenesulfonate (3). A solution of 1 (2.11 g, 10 mmol) and tosyl chloride (2.85 g, 15 mmol) in CH2Cl2 (5 mL) was stirred in an ice bath. Then triethylamine (10 mL) was added to the mixture in drops. The resulting mixture was stirred at room temperature for 5 h, then the solvent was evaporated under reduced pressure. After 50 mL of water was added, the mixture was extracted by CH2Cl2 (3 × 10 mL). Combined organic layers were dried over anhydrous MgSO4, filtered and concentrated under vacuum. The residue was purified by silica gel chromatography (petroleum ether/AcOEt = 1/1, v/v), and 3 was obtained as a light yellow oil in a yield of 62.4%. 1H NMR (400 MHz, CDCl3) δ 9.96 (s, 1H), 8.61 (d, J = 2.2 Hz, 1H), 8.08 (dd, J = 8.7, 2.3 Hz, 1H), 7.80 (d, J = 8.2 Hz, 2H), 7.32 (d, J = 8.2 Hz, 2H), 6.88 (d, J = 8.7 Hz, 1H), 4.52 (t, J = 4.6 Hz, 2H), 4.19 (t, J = 4.6 Hz, 2H), 3.81 (t, J = 4.7 Hz, 2H), 3.75 (t, J = 4.6 Hz, 2H), 2.43 (s, 3H). MS (ESI): m/z calcd for C17H19NO6S, 365.1; found 366.1 (M + H+).
2-(2-(2-((5-Formylpyridin-2-yl)oxy)ethoxy)ethoxy)ethyl 4-methylbenzenesulfonate (4). The procedure described above for the preparation of 3 was used and 4 was obtained as a light yellow oil in a yield of 87.3%. 1H NMR (400 MHz, CDCl3) δ 9.96 (s, 1H), 8.63 (d, J = 2.2 Hz, 1H), 8.11 (dd, J = 8.7, 2.3 Hz, 1H), 7.80 (d, J = 8.3 Hz, 2H), 7.34 (d, J = 8.0 Hz, 2H), 6.93 (d, J = 8.7 Hz, 1H), 4.64–4.57 (m, 2H), 4.15 (dd, J = 9.5, 4.6 Hz, 2H), 3.85 (dt, J = 12.2, 5.4 Hz, 2H), 3.69 (dd, J = 8.9, 4.2 Hz, 2H), 3.68–3.63 (m, 2H), 3.64–3.58 (m, 2H), 2.44 (s, 3H). MS (ESI): m/z calcd for C19H23NO7S, 409.1; found 410.1 (M + H+).
6-(2-Fluoroethoxy)nicotinaldehyde (5). The procedure described above for the preparation of 1 was used and 5 was obtained as a light yellow solid from 2-fluoroethanol in a yield of 27.8%. 1H NMR (400 MHz, CDCl3) δ 9.97 (s, 1H), 8.62 (d, J = 2.2 Hz, 1H), 8.10 (dd, J = 8.7, 2.3 Hz, 1H), 6.93 (d, J = 8.6 Hz, 1H), 4.78 (dt, J = 47.1, 3.8 Hz, 3H), 4.66 (dd, J = 4.2, 2.6 Hz, 1H). MS (ESI): m/z calcd for C8H8FNO2, 169.1; found 170.3 (M + H+).
6-(2-(2-Fluoroethoxy)ethoxy)nicotinaldehyde (6). A solution of 3 (3.65 g, 10 mmol) and anhydrous TBAF (10.5 mL, 1 M in THF) in 10 mL of dry tetrahydrofuran (THF) was refluxed for 4 h at 80 °C. After removal of the THF, the residue was purified by silica gel chromatography (petroleum ether/AcOEt = 2/1, v/v), and 6 was obtained as a light yellow waxy solid in a yield of 47.0%. 1H NMR (400 MHz, CDCl3) δ 9.96 (s, 1H), 8.61 (d, J = 2.3 Hz, 1H), 8.08 (dd, J = 8.7, 2.3 Hz, 1H), 6.90 (d, J = 8.7 Hz, 1H), 4.66 (t, J = 4.1 Hz, 1H), 4.61 (dd, J = 5.4, 4.0 Hz, 2H), 4.54 (t, J = 4.1 Hz, 1H), 3.92 (t, J = 4.7 Hz, 2H), 3.88–3.83 (m, J = 4.2 Hz, 1H), 3.78 (t, J = 4.2 Hz, 1H). MS (ESI): m/z calcd for C10H12FNO3, 213.1; found 214.3 (M + H+).
6-(2-(2-(2-Fluoroethoxy)ethoxy)ethoxy)nicotinaldehyde (7). The procedure described above for the preparation of 6 was used and 7 was obtained as a brown oil in a yield of 57.2%. 1H NMR (400 MHz, CDCl3) δ 9.96 (s, 1H), 8.61 (d, J = 2.2 Hz, 1H), 8.08 (dd, J = 8.7, 2.3 Hz, 1H), 6.90 (d, J = 8.7 Hz, 1H), 4.63 (t, J = 4.1 Hz, 1H), 4.61 (t, J = 4.2 Hz, 2H), 4.51 (t, J = 4.1 Hz, 1H), 3.89 (t, J = 4.8 Hz, 2H), 3.79 (t, J = 4.2 Hz, 1H), 3.77–3.68 (m, 5H). MS (ESI): m/z calcd for C12H16FNO4, 257.1; found 258.1 (M + H+).
4-(2-(2-Hydroxyethoxy)ethoxy)benzaldehyde (8). A solution of 4-hydroxybenzaldehyde (2.44 g, 20 mmol) and KOH (4.22 g, 25 mmol) in ethanol (30 mL) was refluxed for 30 min at 80 °C. The solution of 2-(2-chloroethoxy) (1.25 g, 20 mmol) in ethanol (20 mL) was dropped in the mixture. After refluxing for another 1 h at 80 °C, the solvent was evaporated under reduced pressure. After 50 mL of water was added, the mixture was extracted by CH2Cl2 (3 × 10 mL). Combined organic layers were dried over anhydrous MgSO4, filtered and concentrated under vacuum. The residue was purified by silica gel chromatography (petroleum ether/AcOEt = 2/1, v/v) and 8 was obtained as a light yellow waxy oil in a yield of 56.0%. 1H NMR (400 MHz, CDCl3) δ 9.84 (s, 1H), 7.79 (d, J = 8.7 Hz, 2H), 6.99 (d, J = 8.7 Hz, 2H), 4.31–3.96 (m, 2H), 3.97–3.78 (m, 2H), 3.80–3.67 (m, 2H), 3.69–3.57 (m, 2H). MS (ESI): m/z calcd for C11H14O4, 210.1; found 211.2 (M + H+).
4-(2-(2-(2-Hydroxyethoxy)ethoxy)ethoxy)benzaldehyde (9). The procedure described above for the preparation of 8 was used and 9 was obtained as a light yellow oil in a yield of 48.3%. 1H NMR (400 MHz, CDCl3) δ 9.86 (s, 1H), 7.81 (dd, J = 8.7, 2.1 Hz, 2H), 7.01 (dd, J = 8.6, 2.1 Hz, 2H), 4.21–4.20 (m, 2H), 3.89–3.87 (m, 2H), 3.73–3.72 (m, 6H), 3.61–3.59 (m, 2H). MS (ESI): m/z calcd for C13H18O5, 254.1; found 255.3 (M + H+).
2-(2-(4-Formylphenoxy)ethoxy)ethyl 4-methylbenzenesulfonate (10). The procedure described above for the preparation of 3 was used and 10 was obtained as a light yellow waxy solid in a yield of 93.8%. 1H NMR (400 MHz, CDCl3) δ 9.88 (s, 1H), 7.81 (dd, J = 16.3, 8.5 Hz, 4H), 7.30 (d, J = 8.2 Hz, 2H), 7.00 (d, J = 8.7 Hz, 2H), 4.19 (t, J = 4.6 Hz, 2H), 4.14 (t, J = 4.4 Hz, 2H), 3.82 (t, J = 4.6 Hz, 2H), 3.77 (t, J = 4.7 Hz, 2H), 2.41 (s, 3H). MS (ESI): m/z calcd for C18H20O6S, 364.1; found 365.1 (M + H+).
2-(2-(2-(4-Formylphenoxy)ethoxy)ethoxy)ethyl 4-methylbenzenesulfonate (11). The procedure described above for the preparation of 3 was used and 11 was obtained as a light yellow oil in a yield of 59.4%. 1H NMR (400 MHz, CDCl3) δ 9.89 (s, 1H), 7.81 (dd, J = 14.4, 8.4 Hz, 4H), 7.33 (d, J = 8.1 Hz, 2H), 7.02 (d, J = 8.7 Hz, 2H), 4.20 (dd, J = 9.3, 4.5 Hz, 2H), 4.18–4.14 (m, 2H), 3.87 (t, J = 4.6 Hz, 2H), 3.73–3.65 (m, 4H), 3.63 (dd, J = 5.8, 3.0 Hz, 2H), 2.43 (s, 3H). MS (ESI): m/z calcd for C20H24O7S, 408.1; found 409.1 (M + H+).
4-(2-Fluoroethoxy)benzaldehyde (12). The procedure described above for the preparation of 8 was used and 12 was obtained as a light yellow solid from 1-chloro-2-fluoroethane in a yield of 87.8%. 1H NMR (400 MHz, CDCl3) δ 9.90 (s, 1H), 7.86 (d, J = 8.7 Hz, 2H), 7.04 (d, J = 8.6 Hz, 2H), 4.88–4.71 (m, 2H), 4.37–4.24 (m, 2H). MS (ESI): m/z calcd for C9H9FO2, 168.1; found 169.3 (M + H+).
4-(2-(2-Fluoroethoxy)ethoxy)benzaldehyde (13). The procedure described above for the preparation of 6 was used and 13 was obtained as a light yellow waxy solid in a yield of 71.8%. 1H NMR (400 MHz, CDCl3) δ 9.89 (s, 1H), 7.84 (d, J = 8.6 Hz, 2H), 7.03 (d, J = 8.7 Hz, 2H), 4.61 (dt, J = 47.6, 4.0 Hz, 2H), 4.24 (t, J = 4.7 Hz, 2H), 3.96–3.91 (m, 2H), 3.87 (t, J = 4.1 Hz, 1H), 3.80 (t, J = 4.1 Hz, 1H). MS (ESI): m/z calcd for C11H13FO3, 212.1; found 213.3 (M + H+).
4-(2-(2-(2-Fluoroethoxy)ethoxy)ethoxy)benzaldehyde (14). The procedure described above for the preparation of 6 was used and 14 was obtained as a light yellow waxy solid in a yield of 88.9%. 1H NMR (400 MHz, CDCl3) δ 9.87 (s, 1H), 7.84 (d, J = 8.7 Hz, 2H), 7.03 (d, J = 8.7 Hz, 2H), 4.57 (dt, J = 47.7, 4.1 Hz, 2H), 4.23 (t, J = 4.6 Hz, 2H), 3.91 (t, J = 4.8 Hz, 2H), 3.80 (t, J = 4.2 Hz, 1H), 3.78–3.70 (m, 5H). MS (ESI): m/z calcd for C13H17FO4, 256.1; found 257.2 (M + H+).
General procedure for the synthesis of dibenzylideneacetones derivatives (15a–17a, 15b–17b, 15c–17c, 18–20)
To a solution of the corresponding alkenone (10 mmol) and substituted aromatic aldehydes (10 mmol) in 10 mL of methanol was added 0.5 mL of NaOMe (28% in methanol). The reaction mixture was stirred for about 6 h at room temperature. The precipitate was collected by filtration, and recrystallized from ethanol or purified by silica gel chromatography to afford the final products.
(1E,4E)-1-(4-(Dimethylamino)phenyl)-5-(6-(2-fluoroethoxy)pyridin-3-yl)penta-1,4-dien-3-one (15a). Orange solid, yield 40.2%. 1H NMR (400 MHz, CDCl3) δ 8.32 (s, 1H), 7.90 (dd, J = 8.8, 2.3 Hz, 1H), 7.69 (dd, J = 23.2, 15.8 Hz, 2H), 7.53 (d, J = 8.6 Hz, 2H), 7.01 (d, J = 15.9 Hz, 1H), 6.88 (s, 1H), 6.85 (d, J = 5.5 Hz, 1H), 6.70 (d, J = 8.7 Hz, 2H), 4.77 (dt, J = 47.4, 3.7 Hz, 3H), 4.66 (t, J = 4.2 Hz, 1H), 4.59 (t, J = 4.2 Hz, 1H), 3.05 (s, 6H). 13C NMR (101 MHz, CDCl3) δ 188.33, 164.21, 152.05, 148.36, 144.48, 138.12, 136.76, 130.41, 125.10, 125.04, 120.75, 111.88, 111.85, 81.90 (d, J = 168.8 Hz), 65.25 (d, J = 19.8 Hz), 40.18. HRMS (ESI): m/z calcd for C20H21FN2O2, 341.1665; found 341.1666 (M+).
(1E,4E)-1-(6-(Dimethylamino)pyridin-3-yl)-5-(4-(2-fluoroethoxy)phenyl)penta-1,4-dien-3-one (15b). Yellow solid, yield 28.7%. 1H NMR (400 MHz, CDCl3) δ 8.38 (s, 1H), 7.78 (s, 1H), 7.69 (d, J = 15.9 Hz, 2H), 7.58 (d, J = 8.7 Hz, 2H), 7.02–6.93 (m, 3H), 6.88 (d, J = 15.5 Hz, 1H), 6.60 (s, 1H), 4.79 (dt, J = 39.4, 4.0 Hz, 2H), 4.27 (dt, J = 27.7, 4.2 Hz, 2H), 3.21 (s, 6H). 13C NMR (101 MHz, CDCl3) δ 188.47, 160.19, 159.63, 150.69, 142.05, 140.75, 135.29, 130.05, 128.32, 123.78, 121.60, 118.69, 114.98, 106.05, 81.74 (d, J = 171.7 Hz), 67.16 (d, J = 20.6 Hz), 38.18. HRMS (ESI): m/z calcd for C20H21FN2O2, 341.1665; found 341.1664 (M+).
(1E,4E)-1-(6-(Dimethylamino)pyridin-3-yl)-5-(6-(2-fluoroethoxy)pyridin-3-yl)penta-1,4-dien-3-one (15c). Yellow solid, yield 59.4%. 1H NMR (400 MHz, CDCl3) δ 8.34 (dd, J = 18.4, 2.2 Hz, 2H), 7.90 (dd, J = 8.7, 2.4 Hz, 1H), 7.77 (d, J = 9.0 Hz, 1H), 7.67 (d, J = 15.9 Hz, 2H), 6.99 (d, J = 15.9 Hz, 1H), 6.88 (d, J = 2.4 Hz, 1H), 6.84 (d, J = 9.6 Hz, 1H), 6.58 (d, J = 8.9 Hz, 1H), 4.77 (dt, J = 47.4, 4.0 Hz, 2H), 4.66 (t, J = 4.3 Hz, 1H), 4.59 (t, J = 3.4 Hz, 1H), 3.19 (s, 6H). 13C NMR (101 MHz, CDCl3) δ 188.02, 164.28, 159.87, 151.09, 148.44, 141.43, 138.55, 136.74, 135.16, 124.88, 124.80, 121.23, 118.51, 111.85, 105.96, 81.35 (d, J = 170.4 Hz), 65.25 (d, J = 20.0 Hz), 38.11. HRMS (ESI): m/z calcd for C19H20FN3O2, 342.1618; found 342.1617 (M+).
(1E,4E)-1-(4-(Dimethylamino)phenyl)-5-(6-(2-(2-fluoroethoxy)ethoxy)pyridin-3-yl)penta-1,4-dien-3-one (16a). Red solid, yield 30.6%. 1H NMR (400 MHz, CDCl3) δ 8.33 (s, 1H), 7.88 (dd, J = 8.7, 2.4 Hz, 1H), 7.69 (t, J = 15.7 Hz, 2H), 7.58 (d, J = 8.7 Hz, 2H), 6.99 (d, J = 15.9 Hz, 2H), 6.92 (d, J = 15.8 Hz, 2H), 6.85 (dd, J = 8.7, 3.0 Hz, 1H), 4.66 (t, J = 4.2 Hz, 1H), 4.55 (dd, J = 8.7, 4.2 Hz, 3H), 3.91 (t, J = 4.6 Hz, 2H), 3.85 (t, J = 4.2 Hz, 1H), 3.78 (t, J = 4.2 Hz, 1H), 3.09 (s, 6H). 13C NMR (101 MHz, CDCl3) δ 188.31, 164.60, 151.87, 148.80, 148.45, 144.29, 139.72, 138.28, 136.56, 130.36, 124.35, 120.88, 112.00, 111.85, 82.65 (d, J = 169.7 Hz), 70.43 (d, J = 20.8 Hz), 69.81, 65.51, 40.25. HRMS (ESI): m/z calcd for C22H25FN2O3, 385.1927; found 385.1933 (M+).
(1E,4E)-1-(6-(Dimethylamino)pyridin-3-yl)-5-(4-(2-(2-fluoroethoxy)ethoxy)phenyl)penta-1,4-dien-3-one (16b). Orange solid, yield 33.6%. 1H NMR (400 MHz, CDCl3) δ 8.35 (d, J = 2.3 Hz, 1H), 7.75 (dd, J = 9.0, 2.4 Hz, 1H), 7.70 (d, J = 6.3 Hz, 1H), 7.66 (d, J = 6.2 Hz, 1H), 7.57 (d, J = 8.7 Hz, 2H), 6.97 (d, J = 9.2 Hz, 2H), 6.94 (d, J = 2.1 Hz, 1H), 6.86 (d, J = 15.8 Hz, 1H), 6.55 (d, J = 9.0 Hz, 1H), 4.61 (dt, J = 49.6, 4.1 Hz, 2H), 4.19 (dd, J = 10.3, 5.4 Hz, 2H), 3.91 (dd, J = 8.6, 4.0 Hz, 2H), 3.89–3.86 (m, 1H), 3.81–3.78 (m, 1H), 3.17 (s, 6H). 13C NMR (101 MHz, CDCl3) δ 188.52, 160.56, 159.74, 150.82, 142.18, 140.77, 135.18, 130.00, 127.97, 123.57, 121.54, 118.68, 114.99, 105.97, 83.16 (d, J = 169.7 Hz), 70.63 (d, J = 19.7 Hz), 69.80, 67.54, 38.13. HRMS (ESI): m/z calcd for C22H25FN2O3, 385.1927; found 385.1926 (M+).
(1E,4E)-1-(6-(Dimethylamino)pyridin-3-yl)-5-(6-(2-(2-fluoroethoxy)ethoxy)pyridin-3-yl)penta-1,4-dien-3-one (16c). Yellow solid, yield 42.0%. 1H NMR (400 MHz, CDCl3) δ 8.36 (d, J = 2.0 Hz, 1H), 8.32 (d, J = 2.3 Hz, 1H), 7.88 (dd, J = 8.7, 2.4 Hz, 1H), 7.77 (d, J = 9.0 Hz, 1H), 7.67 (d, J = 15.9 Hz, 2H), 6.98 (d, J = 15.9 Hz, 1H), 6.86 (d, J = 6.0 Hz, 1H), 6.85 (s, 1H), 6.58 (d, J = 9.0 Hz, 1H), 4.66 (t, J = 4.1 Hz, 1H), 4.55 (dt, J = 6.9, 2.7 Hz, 3H), 3.91 (t, J = 4.7 Hz, 2H), 3.85 (t, J = 4.2 Hz, 1H), 3.78 (t, J = 4.2 Hz, 1H), 3.19 (s, 6H). 13C NMR (101 MHz, CDCl3) δ 188.04, 164.70, 159.75, 150.89, 148.58, 141.27, 138.73, 136.57, 135.25, 124.62, 124.59, 121.32, 118.56, 111.88, 106.03, 83.14 (d, J = 169.9 Hz), 70.43 (d, J = 19.8 Hz), 69.79, 65.54, 38.15. HRMS (ESI): m/z calcd for C21H24FN3O3, 386.1880; found 386.1884 (M+).
(1E,4E)-1-(4-(Dimethylamino)phenyl)-5-(6-(2-(2-(2-fluoroethoxy)ethoxy)ethoxy)pyridin-3-yl)penta-1,4-dien-3-one (17a). Red solid, yield 26.0%. 1H NMR (400 MHz, CDCl3) δ 8.30 (s, 1H), 7.87 (dd, J = 8.7, 2.3 Hz, 1H), 7.69 (dd, J = 19.5, 15.8 Hz, 2H), 7.55 (d, J = 8.7 Hz, 2H), 6.99 (d, J = 15.9 Hz, 1H), 6.89 (d, J = 15.8 Hz, 2H), 6.83 (d, J = 8.6 Hz, 2H), 4.63 (t, J = 4.2 Hz, 1H), 4.54 (t, J = 4.6 Hz, 2H), 4.51 (t, J = 4.2 Hz, 1H), 3.88 (t, J = 4.8 Hz, 2H), 3.79 (t, J = 4.2 Hz, 1H), 3.77–3.69 (m, 5H), 3.07 (s, 6H). 13C NMR (101 MHz, CDCl3) δ 188.31, 164.65, 152.02, 148.45, 144.36, 138.24, 136.52, 130.36, 124.86, 124.64, 122.41, 120.71, 111.85, 111.82, 83.13 (d, J = 169.5 Hz), 70.81, 70.70, 70.43 (d, J = 19.8 Hz), 69.64, 65.55, 40.10. HRMS (ESI): m/z calcd for C24H29FN2O4, 429.2190; found 429.2183 (M+).
(1E,4E)-1-(6-(Dimethylamino)pyridin-3-yl)-5-(4-(2-(2-(2-fluoroethoxy)ethoxy)ethoxy)phenyl)penta-1,4-dien-3-one (17b). Yellow solid, yield 56.2%. 1H NMR (400 MHz, CDCl3) δ 8.37 (s, 1H), 7.77 (d, J = 8.6 Hz, 1H), 7.67 (dd, J = 15.8, 12.9 Hz, 2H), 7.56 (d, J = 8.7 Hz, 2H), 6.97 (dd, J = 16.4, 9.2 Hz, 3H), 6.87 (d, J = 15.8 Hz, 1H), 6.58 (d, J = 6.7 Hz, 1H), 4.63 (dd, J = 5.1, 4.2 Hz, 1H), 4.51 (t, J = 4.2 Hz, 1H), 4.19 (t, J = 4.6 Hz, 2H), 3.89 (t, J = 4.9 Hz, 2H), 3.80 (t, J = 4.2 Hz, 1H), 3.78–3.74 (m, 2H), 3.74–3.70 (m, 3H), 3.19 (s, 6H). 13C NMR (101 MHz, CDCl3) δ 188.52, 160.65, 159.72, 150.80, 142.22, 140.74, 135.19, 129.99, 127.88, 123.52, 121.55, 118.69, 115.00, 105.97, 82.64 (d, J = 169.6 Hz), 70.86, 70.45 (d, J = 19.8 Hz), 69.68, 67.53, 38.13. HRMS (ESI): m/z calcd for C24H29FN2O4, 429.2190; found 429.2191 (M+).
(1E,4E)-1-(6-(Dimethylamino)pyridin-3-yl)-5-(6-(2-(2-(2-fluoroethoxy)ethoxy)ethoxy)pyridin-3-yl)penta-1,4-dien-3-one (17c). Orange oil, yield 20.4%. 1H NMR (400 MHz, CDCl3) δ 8.36 (d, J = 2.1 Hz, 1H), 8.32 (d, J = 2.3 Hz, 1H), 7.87 (dd, J = 8.7, 2.4 Hz, 1H), 7.76 (dd, J = 9.0, 2.2 Hz, 1H), 7.67 (dd, J = 15.8, 1.8 Hz, 2H), 6.98 (d, J = 15.9 Hz, 1H), 6.86 (d, J = 9.8 Hz, 1H), 6.83 (d, J = 2.6 Hz, 1H), 6.57 (d, J = 9.0 Hz, 1H), 4.63 (t, J = 4.2 Hz, 1H), 4.53 (t, J = 4.6 Hz, 2H), 4.51 (t, J = 4.2 Hz, 1H), 3.88 (t, J = 4.2 Hz, 2H), 3.79 (t, J = 4.6 Hz, 1H), 3.76–3.68 (m, J = 9.3, 5.1 Hz, 5H), 3.19 (s, 6H). 13C NMR (101 MHz, CDCl3) δ 188.07, 164.74, 159.82, 151.01, 148.57, 141.32, 138.74, 136.52, 135.17, 124.56, 124.50, 121.24, 118.52, 111.86, 105.97, 83.12 (d, J = 169.7 Hz), 70.81, 70.70, 70.42 (d, J = 19.8 Hz), 69.63, 67.57, 38.10. HRMS (ESI): m/z calcd for C23H28FN3O4, 430.2142; found 430.2144 (M+).
(1E,4E)-1-(6-(Dimethylamino)pyridin-3-yl)-5-(4-fluorophenyl)penta-1,4-dien-3-one (18). Yellow solid, yield 52.2%. 1H NMR (400 MHz, CDCl3) δ 8.36 (d, J = 2.0 Hz, 1H), 7.76 (dd, J = 9.0, 1.9 Hz, 1H), 7.68 (dd, J = 15.8, 2.2 Hz, 2H), 7.61 (dd, J = 8.6, 5.4 Hz, 2H), 7.10 (t, J = 8.6 Hz, 2H), 7.01 (d, J = 15.8 Hz, 1H), 6.86 (d, J = 15.8 Hz, 1H), 6.57 (d, J = 9.0 Hz, 1H). 13C NMR (101 MHz, CDCl3) δ 188.28, 163.88 (d, J = 252.2 Hz), 159.78, 150.95, 141.33, 141.09, 135.22, 131.27, 130.15, 125.26, 121.33, 118.53, 115.55 (d, J = 22.0 Hz), 106.01, 38.14. HRMS (ESI): m/z calcd for C18H17FN2O, 297.1403; found 297.1402 (M+).
(1E,4E)-1-(6-(Dimethylamino)pyridin-3-yl)-5-(4-iodophenyl)penta-1,4-dien-3-one (19). Orange solid, yield 58.1%. 1H NMR (400 MHz, CDCl3) δ 8.35 (d, J = 2.4 Hz, 1H), 7.74 (d, J = 8.4 Hz, 3H), 7.68 (d, J = 15.8 Hz, 1H), 7.62 (d, J = 15.9 Hz, 1H), 7.33 (d, J = 8.3 Hz, 2H), 7.06 (d, J = 15.9 Hz, 1H), 6.82 (d, J = 12.2 Hz, 1H), 6.53 (dd, J = 9.0 Hz, 1H), 3.16 (s, 6H). 13C NMR (101 MHz, CDCl3) δ 188.16, 159.70, 150.85, 141.47, 141.10, 138.10, 135.30, 134.52, 129.73, 126.11, 121.32, 118.51, 106.07, 96.41, 38.17. HRMS (ESI): m/z calcd for C18H17IN2O, 405.0464; found 405.0465 (M+).
(1E,4E)-1-(4-(Dimethylamino)phenyl)-5-(6-(2-(2-(2-hydroxyethoxy)ethoxy)ethoxy)pyridin-3-yl)penta-1,4-dien-3-one (20). Light yellow oil, yield 70.5%. 1H NMR (400 MHz, CDCl3) δ 8.31 (d, J = 2.3 Hz, 1H), 7.87 (dd, J = 8.7, 2.4 Hz, 1H), 7.72 (dd, J = 5.5, 2.2 Hz, 1H), 7.70 (d, J = 4.7 Hz, 1H), 7.65 (d, J = 15.9 Hz, 1H), 7.55–7.50 (m, 2H), 7.03–6.96 (m, 1H), 6.86 (d, J = 8.6 Hz, 1H), 6.83 (d, J = 1.4 Hz, 1H), 6.70 (d, J = 8.9 Hz, 2H), 4.57–4.50 (m, 2H), 3.91–3.84 (m, 2H), 3.77–3.68 (m, 6H), 3.63 (dd, J = 5.3, 3.7 Hz, 2H), 3.05 (s, 6H). MS (ESI): m/z calcd for C24H30N2O5, 426.2; found 426.8 (M+).
2-(2-(2-((5-((1E,4E)-5-(4-(Dimethylamino)phenyl)-3-oxopenta-1,4-dien-1-yl)pyridin-2-yl)oxy)ethoxy)ethoxy)ethyl 4-methylbenzenesulfonate (21). The procedure described above for the preparation of 2 was used and 21 was obtained as a red oil, yield 63.2%. 1H NMR (400 MHz, CDCl3) δ 8.31 (d, J = 2.0 Hz, 1H), 7.87 (dd, J = 8.7, 2.3 Hz, 1H), 7.80 (d, J = 8.3 Hz, 2H), 7.72 (d, J = 15.8 Hz, 1H), 7.66 (d, J = 15.9 Hz, 1H), 7.54 (d, J = 8.8 Hz, 2H), 7.33 (d, J = 8.1 Hz, 2H), 7.00 (d, J = 15.9 Hz, 1H), 6.88 (d, J = 15.7 Hz, 1H), 6.83 (d, J = 8.7 Hz, 1H), 6.81–6.73 (m, 2H), 4.62–4.46 (m, 2H), 4.19–4.13 (m, 2H), 3.91–3.78 (m, 2H), 3.75–3.67 (m, 2H), 3.67–3.57 (m, 4H), 3.06 (s, 6H), 2.44 (s, 3H). MS (ESI): m/z calcd for C31H36N2O7S, 580.2; found 580.7 (M+).
(1E,4E)-1-(6-(Dimethylamino)pyridin-3-yl)-5-(4-(tributylstannyl)phenyl)penta-1,4-dien-3-one (22). A mixture of 19 (80 mg, 0.20 mmol), (Bu3Sn)2 (348.0 mg, 0.60 mmol), and (Ph3P)4Pd (23 mg, 0.02 mmol) in toluene (10 mL) was stirred under reflux for 10 h. The solvent was removed, and the residue was purified by silica gel chromatography to give 23.3 mg of 22 as a orange solid, yield 20.5%. 1H NMR (400 MHz, CDCl3) δ 8.41 (s, 1H), 7.83 (d, J = 7.1 Hz, 1H), 7.71 (d, J = 15.9 Hz, 1H), 7.64 (d, J = 15.8 Hz, 1H), 7.58–7.49 (m, 4H), 7.08 (d, J = 15.9 Hz, 1H), 6.92 (d, J = 15.9 Hz, 1H), 6.73–6.58 (m, 1H), 3.25 (s, 6H), 1.60–1.49 (m, 5H), 1.40–1.27 (m, 7H), 1.11–1.04 (m, 5H), 0.93–0.82 (m, 10H). MS (ESI): m/z calcd for C30H44N2OSn, 568.2; found 569.4 (M + H+).
Acknowledgements
This study was supported by the National Natural Science Foundation of China (no. 21571022 and 21201019), and the National Science and Technology Major Projects for Major New Drugs Innovation and Development (no. 2014ZX09507007-002). We thank Dr Jin Liu (College of Life Science, Beijing Normal University) for assistance in the in vitro neuropathological staining.
Notes and references
- R. Brookmeyer, E. Johnson, K. Ziegler-Graham and H. M. Arrighi, Alzheimer's Dementia, 2007, 3, 186–191 CrossRef PubMed.
- J. Hardy and D. J. Selkoe, Science, 2002, 297, 353–356 CrossRef CAS PubMed.
- D. J. Selkoe, J. Am. Med. Assoc., 2000, 283, 1615–1617 CrossRef CAS PubMed.
- C. A. Mathis, Y. Wang and W. E. Klunk, Curr. Pharm. Des., 2004, 10, 1469–1492 CrossRef CAS PubMed.
- L. Cai, R. B. Innis and V. W. Pike, Curr. Med. Chem., 2007, 14, 19–52 CrossRef CAS PubMed.
- C. A. Mathis, Y. Wang, D. P. Holt, G.-F. Huang, M. L. Debnath and W. E. Klunk, J. Med. Chem., 2003, 46, 2740–2754 CrossRef CAS PubMed.
- W. E. Klunk, H. Engler, A. Nordberg, Y. Wang, G. Blomqvist, D. P. Holt, M. Bergström, I. Savitcheva, G.-F. Huang, S. Estrada, B. Ausén, M. L. Debnath, J. Barletta, J. C. Price, J. Sandell, B. J. Lopresti, A. Wall, P. Koivisto, G. Antoni, C. A. Mathis and B. Långström, Ann. Neurol., 2004, 55, 306–319 CrossRef CAS PubMed.
- A. Kadir, A. Marutle, D. Gonzalez, M. Schöll, O. Almkvist, M. Mousavi, T. Mustafiz, T. Darreh-Shori, I. Nennesmo and A. Nordberg, Brain, 2010, 134, 301–317 CrossRef PubMed.
- N. Okamura, Y. Shiga, S. Furumoto, M. Tashiro, Y. Tsuboi, K. Furukawa, K. Yanai, R. Iwata, H. Arai, Y. Kudo, Y. Itoyama and K. Doh-ura, Eur. J. Nucl. Med. Mol. Imaging, 2010, 37, 934–941 CrossRef CAS PubMed.
- E. D. Agdeppa, V. Kepe, J. Liu, G. W. Small, S.-C. Huang, A. Petrič, N. Satyamurthy and J. R. Barrio, Molecular Imaging and Biology, 2003, 5, 404–417 CrossRef PubMed.
- V. Camus, P. Payoux, L. Barré, B. Desgranges, T. Voisin, C. Tauber, R. La Joie, M. Tafani, C. Hommet, G. Chételat, K. Mondon, V. de La Sayette, J. P. Cottier, E. Beaufils, M. J. Ribeiro, V. Gissot, E. Vierron, J. Vercouillie, B. Vellas, F. Eustache and D. Guilloteau, Eur. J. Nucl. Med. Mol. Imaging, 2012, 39, 621–631 CrossRef CAS PubMed.
- H. F. Kung, S. R. Choi, W. Qu, W. Zhang and D. Skovronsky, J. Med. Chem., 2010, 53, 933–941 CrossRef CAS PubMed.
- D. F. Wong, P. B. Rosenberg, Y. Zhou, A. Kumar, V. Raymont, H. T. Ravert, R. F. Dannals, A. Nandi, J. R. Brašić, W. Ye, J. Hilton, C. Lyketsos, H. F. Kung, A. D. Joshi, D. M. Skovronsky and M. J. Pontecorvo, J. Nucl. Med., 2010, 51, 913–920 CrossRef CAS PubMed.
- C. C. Rowe, U. Ackerman, W. Browne, R. Mulligan, K. L. Pike, G. O'Keefe, H. Tochon-Danguy, G. Chan, S. U. Berlangieri, G. Jones, K. L. Dickinson-Rowe, H. P. Kung, W. Zhang, M. P. Kung, D. Skovronsky, T. Dyrks, G. Holl, S. Krause, M. Friebe, L. Lehman, S. Lindemann, L. M. Dinkelborg, C. L. Masters and V. L. Villemagne, Lancet Neurol., 2008, 7, 129–135 CrossRef CAS PubMed.
- M. Koole, D. M. Lewis, C. Buckley, N. Nelissen, M. Vandenbulcke, D. J. Brooks, R. Vandenberghe and K. Van Laere, J. Nucl. Med., 2009, 50, 818–822 CrossRef CAS PubMed.
- M.-P. Kung, C. Hou, Z.-P. Zhuang, B. Zhang, D. Skovronsky, J. Q. Trojanowski, V. M. Y. Lee and H. F. Kung, Brain Res., 2002, 956, 202–210 CrossRef CAS PubMed.
- A. B. Newberg, N. A. Wintering, K. Plössl, J. Hochold, M. G. Stabin, M. Watson, D. Skovronsky, C. M. Clark, M.-P. Kung and H. F. Kung, J. Nucl. Med., 2006, 47, 748–754 CAS.
- F. Yang, G. P. Lim, A. N. Begum, O. J. Ubeda, M. R. Simmons, S. S. Ambegaokar, P. P. Chen, R. Kayed, C. G. Glabe, S. A. Frautschy and G. M. Cole, J. Biol. Chem., 2005, 280, 5892–5901 CrossRef CAS PubMed.
- M. Cui, M. Ono, H. Kimura, B. Liu and H. Saji, J. Med. Chem., 2011, 54, 2225–2240 CrossRef CAS PubMed.
- Y. Yang, M. Cui, B. Jin, X. Wang, Z. Li, P. Yu, J. Jia, H. Fu, H. Jia and B. Liu, Eur. J. Med. Chem., 2013, 64, 90–98 CrossRef CAS PubMed.
- Z. Li, M. Cui, J. Zhang, J. Dai, X. Zhang, P. Chen, H. Jia and B. Liu, Eur. J. Med. Chem., 2014, 84, 628–638 CrossRef CAS PubMed.
- M. Cui, M. Ono, H. Kimura, H. Kawashima, B. L. Liu and H. Saji, Nucl. Med. Biol., 2011, 38, 313–320 CrossRef CAS PubMed.
- M. Cui, X. Wang, P. Yu, J. Zhang, Z. Li, X. Zhang, Y. Yang, M. Ono, H. Jia, H. Saji and B. Liu, J. Med. Chem., 2012, 55, 9283–9296 CrossRef CAS PubMed.
Footnote |
† Electronic supplementary information (ESI) available: Additional tables and figures, 1H NMR, 13C NMR, MS and HRMS spectra. See DOI: 10.1039/c6ra05168e |
|
This journal is © The Royal Society of Chemistry 2016 |