DOI:
10.1039/C6RA04719J
(Paper)
RSC Adv., 2016,
6, 42324-42333
Cu–BTC@cotton composite: design and removal of ethion insecticide from water†
Received
22nd February 2016
, Accepted 13th April 2016
First published on 15th April 2016
Abstract
Pesticide removal from wastewater is of significant general benefit to protect of humans from the effect of pollution. Current study offers excellent material for organophosphate insecticide pollution remediation based on metal–organic frameworks (MOFs). A facile method was used to functionalize cotton fabric by assembly with Cu–BTC MOFs. The so-prepared Cu–BTC@cotton composite was characterized by X-ray diffraction, infrared spectroscopy, elemental analysis, and electron microscope. Cu–BTC was successfully bonded with cotton fabrics through interaction between Cu and cellulose functional groups. Adsorption of ethion as organophosphorus insecticide onto Cu–BTC@cotton composite was systematically studied. Binding sites of composite represented in cellulose functional groups and Cu of MOF were both linked with ethion via sulfur. The equilibrium adsorption isotherm proved that the adsorption of ethion insecticide was fitted well to the Langmuir model. The maximum sorption capacity of Cu–BTC@cotton composite reached 182 mg g−1 and the removal percent of ethion exceeded 97%. Furthermore, Cu–BTC@cotton composite is very stable and can be easily recycled using a simple organic solvent. After recycling five times, the adsorption efficiency of Cu–BTC@cotton composite was still very good and surpassing 85%. Therefore, Cu–BTC@cotton is a perfect sorbent to remove insecticides from wastewater with excellent efficiency.
1. Introduction
Metal–organic frameworks (MOFs) are new hybrid materials discovered fifteen years ago; they are metals joined with an organic (called a linker) to form a 1D, 2D or 3D structure.1 Because of the pores in MOFs' structure, MOFs have high surface area and chemical tenability and therefore hold great interest in fields such as adsorption/storage of carbon dioxide,2 hydrogen storage,3 adsorption of vapours,4 separation of chemicals,5 drug delivery/biomedicine,6 polymerization,7 magnetism,8 catalysis,9 and luminescence.10 Recently, MOFs have been used to remove hazardous materials such as organosulfur and dyes' aromatic molecules.11 It was found that MOFs are more effective compared to activated carbon or mesoporous silica materials12 related to their different pore sizes, their chemical stability and owing to their active sites.13
Organophosphate pesticides (OP) are used to control pests in several crops by inhibiting acetyl cholinesterase enzyme activity in pests.14 Several billion US dollars are spent each year for purchasing these pesticides, reflecting the huge amount of pesticide used. As OP is sprayed over crops or soils, it remains in fruits and vegetables and is released into water.15 Science and study focuses primarily and most importantly on the impact this pollution has on human health and life. Thus, many studies have recently been published whose interest is in pesticide removal from wastewater, including different techniques such as photocatalytic degradation by semiconductors, e.g. TiO2, Fe2O3,16 reverse osmosis and nanofiltration membranes,17 oxidation by hydrogen peroxide or ozone,18 coagulation–flocculation19 and adsorption.20 Adsorption is the most commonly used technique for removing pesticides from water20 and it has become a significant method in both scientific study and commercial applications for removing hazardous materials. The most popular adsorbents to minimize pesticide concentrations in water are activated carbon21 and agricultural waste.22 The high cost of activated carbon manufacturing and regeneration, as well as the modification and low efficiency of agricultural waste are huge problems, limiting the use of these materials. Therefore, discovering and creating new materials with high adsorption capacity and low cost is extremely timely.
Owing to their high adsorption capacity, MOFs provide a superior solution in adsorptive removal of various toxic components,23 but natural materials are still promising due to their low commercial value. Cellulose is a perfect natural polymer as it is a biodegradable, biocompatible and renewable material.24 The consistency of cellulose, such as alcoholic and carboxylic coordinate groups, contributes to its increasing sorption affinity towards metal-containing compounds25–32 and subsequently, enhance the applicability of cellulosic material in the removal of pesticides. Our team has identified a key to solve the problem of pesticide removal from water by using an MOF–fabric composite. According to the literature, there are few studies on pesticide removal from water using MOF–fabric composite.33,34 In fact, no study has been reported to regenerate MOF–fabric composite in the absorptive removal of organophosphate pesticides from water to date.
The current study focuses on creating a low-cost effective adsorbent material based on MOF–fabric composite and its use in pesticide removal. The post synthetically modified cotton fabric was carried out by adding different amounts of MOFs. Creation of the composite was characterized by scanning electron microscope, X-ray diffraction and infrared spectroscopy. Investigation of the MOF–fabric composite for organophosphate pesticide (OP) removal from water was systematically studied and its regeneration was tested. Adsorption studies were carried out under various parameters such as contact time, pesticide concentration and composite concentration. Adsorption kinetic data was tested by pseudo-first-order and pseudo-second-order. Equilibrium data were analyzed using Langmuir and Freundlich isotherm models.
2. Experimental
Preparation of Cu–BTC MOF
Cu–BTC MOF was prepared according to new method by our team. Benzene-1,3,5-tricarboxylic acid (BTC) (0.29 g, 1.38 mmol) and copper(II) nitrate trihydrate (0.61 g, 2.53 mmol) were soluble in 50 mL N,N-dimethylformamide; the mixture was heated in open air until the N,N-dimethylformamide solvent evaporated and then the residues were added to cold water (50 mL). The blue powder was isolated by filtration and dried at room temperature.
Preparation of 14C-ethion
14C-Ethion labelled at the carbon atom of the ethyl groups was prepared as reported in the literature.35 14C-ethanol was added to phosphorus pentasulfide and then methylene chloride was added. The specific activity of prepared 14C-ethion was 7.4 MBq g−1 and radiometric purity was 98%.
Preparation of MOF/cotton composite
Before treatment with metal–organic framework, cotton fabric was activated by oxidation with hydrogen peroxide using an exhaustion technique.31 A 20 × 20 cm specimen of cotton fabric was submerged in a mixture solution containing 2 M H2O2, 4 g L−1 sodium silicate and 10 g L−1 sodium hydroxide using a material to liquor ratio of 1
:
30. The immersion was processed at 90 °C for 60 min. At the end of the process, the fabric was removed and rinsed two times with hot and cold water and then dried at 80 °C ± 5 °C. The carboxyl content of the cotton fabric after oxidation was measured using methylene blue method36 and was 34.8 ± 1.3 mmol kg−1.
The oxidized cotton fabric was soaked in 100 mL of ethanol containing a different quantity of Cu–BTC to produce 5% and 10% Cu–BTC@cotton. The samples were patted dry and then heated to 45 °C for 12 min before vigorously washing with water to remove the un-reacted materials. The fabric was then dried overnight at 45 °C.
Adsorption tests onto Cu–BTC@cotton composite
A stock solution of 14C-ethion (100 ppm) was prepared by dissolving 14C-ethion in deionized water. The optimized condition experiments were carried out by shaking 200 mL 14C-ethion solution with different doses of Cu–BTC@cotton composite (5–25 mg) at different time intervals. Untreated cotton fabric was used at the same experimental conditions for comparison. The 14C-ethion solution was separated from the adsorbent by centrifugation at 5000 rpm for 10 min. The removal percent of ethion was evaluated using eqn (1). |
 | (1) |
where C0 is the initial concentration of ethion and Ct is the concentration at time t. Three independent measurements were carried out for all experiments and the mean was considered.
Kinetic study
The adsorption kinetics of cotton and Cu–BTC@cotton were analyzed by pseudo-first-order37 and pseudo-second-order38 kinetics models using eqn (2) and (3). |
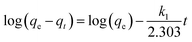 | (2) |
where qe and qt are adsorption capacity (mg g−1) at equilibrium and at time t, respectively, and k1 is the rate constant of pseudo-first order adsorption. |
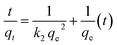 | (3) |
where k2 is the rate constant of pseudo-second-order adsorption.
Sorption isotherms
To understand the process and principle of adsorption systems, adsorption isotherms are an important parameter. Thus, the experimental data were modelled with the Langmuir39 and Freundlich40 paradigms by applying eqn (4) and (5):
Langmuir isotherm:
|
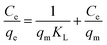 | (4) |
Freundlich isotherm:
|
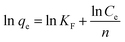 | (5) |
where
KL (L mg
−1) is the Langmuir equilibrium constant,
qm is the maximum adsorption capacity (mg g
−1), and
KF ((mg g
−1) (L m g
−1)
1/n) and
n are Freundlich constants that relate to adsorption capacity and adsorption intensity.
Ethion insecticide determination
The ethion amount remaining in solution was detected using radioactivity assay method. The radioactivity of water samples during different times of uptake was determined using a Packard liquid-scintillation spectrometer (Model TRI-CARB 2300 TR) in vials containing a dioxane-based scintillation cocktail. Radioactivity levels were all corrected for quenching using an internal standard.
Statistical analysis session
The data presented in the current study are mean value for three independent measurements. Standard errors were calculated by Microsoft Excel Program (2007) and included in the tables and figures.
3. Results and discussion
Water is the most essential material for sustaining life on the earth, especially for living organisms, and there is no life without it. Every year, billions of dollars are spent to treat, clean and purify water. However, UNICEF and World Health Organization (WHO) reported in 2012 that around 780 million of people still suffered from a lack of drinking water.41 Therefore, availability of clean water at an affordable price remains a crucial goal for humanity. The current study is interested in designing low-cost and effective adsorbent material (MOF–cotton fabric composite) using a quite simple technique based on an immersion process. MOF–cotton fabrics were used for removal of organophosphate pesticides from water.
Characterization of composite
SEM analysis. The top view of Cu–BTC–cotton was characterized using an electron microscope and the SEM images are presented in Fig. 1. An octahedral shape of homogeneous 3D porous structures of Cu–BTC MOF was observed on a clear rough surface of cotton fabric. The shape of Cu–BTC MOF was similar to that shown in the literature for preparation of MOF.42,43 The copper signal was recorded in an EDX analysis for MOF–cotton composite confirming the presence of Cu–BTC MOF on cotton surface.
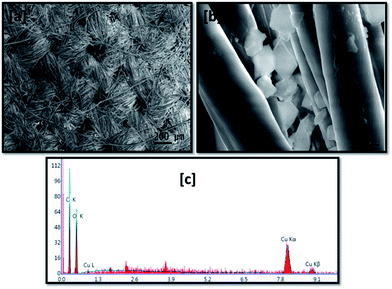 |
| Fig. 1 SEM images of Cu–BTC@cotton at different magnification scales: (a) 200 μm, (b) 5 μm and (c) EDX analysis. | |
X-ray diffraction (XRD) analysis. XRD analyses were applied for Cu–BTC, cotton and Cu–BTC@cotton samples (Fig. 2). The original cotton showed an intense diffraction peak at 2θ = 22.9° assigned to (002) lattice plane of cellulose I. In addition, the diffractions peaks of (101) and (101) lattice planes for cellulose I appeared at 2θ = 15° and 16.6°.31 For Cu–BTC, many peaks at 2θ = 6.7°, 9.4°, 11.6°, 13.4°, 17.4°, 19.1°, 20.1°, 25.9°, 29.4°, 35.3° and 39.2° were recorded, which matched with previous PXRD data for Cu–BTC.42,43 Diffraction peaks of cellulose I and Cu–BTC were both detected for Cu–BTC@cotton at the same diffraction angle. This confirmed that Cu–BTC existed on cotton and the crystal structure of cotton and Cu–BTC was not changed due to their mutual interaction.
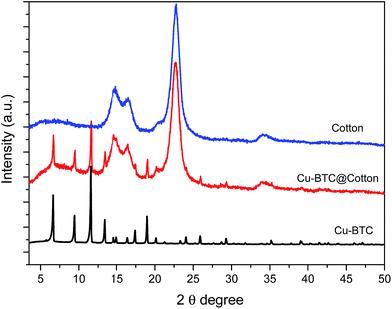 |
| Fig. 2 XRD patterns of Cu–BTC, cotton and Cu–BTC@cotton composite. | |
ATR-FTIR spectra analysis. ATR-FTIR spectra of cotton and ethion–Cu–BTC@cotton are both shown in Fig. 3. The IR spectra of cotton show a broad band at 3404 cm−1 due to the stretching frequency of the OH group, and the band at 2899 cm−1 is related to the asymmetric C–H stretching vibration.31,44
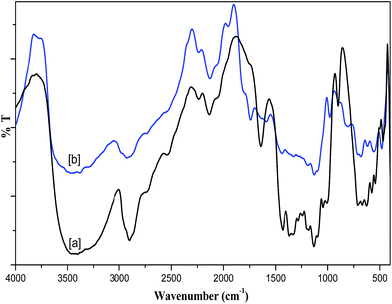 |
| Fig. 3 FT-IR spectra of (a) cotton and (b) Cu–BTC@cotton–ethion. | |
Absorption bands at 1642, 1437 and 1354 cm−1 are attributed to C
O group, H–C–H scissoring, and C–H bending vibration, respectively.31,44 The bands placed in 1191 and 1143 cm−1 are assigned to the asymmetric bridge C–O–C; moreover, a broad peak observed at 1023 cm−1 corresponds to O–C stretching.31,44
After loading of Cu–BTC onto cotton, a new peak appeared at 1582 cm−1, corresponding to asymmetric stretching vibrations of O–C
O group for butane tetra carboxylic acid (BTC). This confirmed that the interaction between Cu–BTC and cotton occurred through the interaction between COOH and OH groups of cotton and Cu.31,36 After ethion adsorption, four new absorption peaks were recorded at 1742, 1043, 810 and 655 cm−1, which were assigned to S–P
S, P–O–C, P
S and C–S stretching, respectively.35,45,46 These peaks are related to ethion structure and therefore affirmed the existence of ethion onto Cu–BTC@cotton after the adsorption process. Intensity of OH and O–C
O peaks were both significantly decreased after ethion adsorption onto Cu–BTC@cotton due to the interaction between cotton and ethion molecules.
The presence of Cu–BTC on cotton was confirmed by IR spectra, XRD patterns and SEM images. The interaction between cotton as cellulosic materials was introduced in the schematic (Fig. 4a). Cellulose as a polysaccharide material contains a great number of interactive function groups comprising primary alcohol, secondary alcohol and carboxyl. These active sites can interact easily with metals or metal-containing materials through cationic exchange and/or coordination making cellulose a good ligand.25–31 Due to its availability to participate in both interactions (cationic exchange and complexation), the carboxyl group is a more effective binding site compared to the hydroxyl groups.31 Therefore, the oxidation process was performed to increase the carboxyl content of cellulose and consequently intensify Cu–BTC content onto cotton.
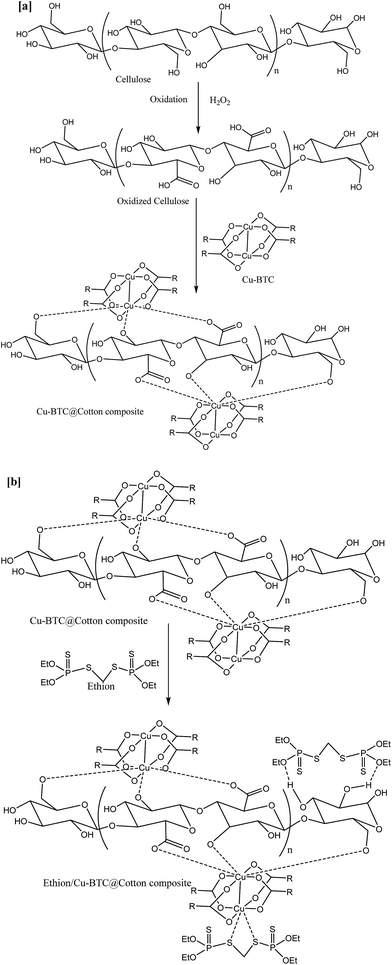 |
| Fig. 4 (a) Scheme for synthesis of Cu–BTC@cotton composite through oxidation of cotton and assembly with Cu–BTC MOFs; (b) schematic proposing the interaction of ethion insecticide with Cu–BTC@cotton composite. | |
As reported in the literature, complexation is the preferable way to chelate copper with cellulose.25,28 In the same way, interaction of Cu–BTC with cellulose mainly occurred via a coordination interaction between Cu and cellulose binding sites, as shown in Fig. 4a.
Application of composite for ethion insecticide removal
Efficient removal of hazardous materials from the environment has become an important issue from a biological and environmental standpoint. From this point, the adsorption systematic study of ethion onto Cu–BTC@cotton composite is much interested application. In this section, adsorption of ethion insecticide on the composite material will be introduced as function of composite mass, ethion concentration and adsorption time. Moreover, adsorption kinetics and regeneration/reuse of composite were both measured.
Mechanism of interaction
According to the porosity structure of the MOF,42,43 Cu–BTC MOF supposed to be highly sorbent material. Sorption efficiency of the so-prepared Cu–BTC@cotton composite might be much greater owing to the available binding sites of cellulose in composite. To perform excellent candidates for adsorption of pesticide from water, adsorption mechanism of ethion as insecticide compound onto Cu–BTC@cotton composite is very important issue. Adsorption of ethion onto Cu–BTC@cotton could be carried out through two main ways: physical adsorption and/or chemical interaction. Pores of Cu–BTC can act as trapping sites for ethion molecules and therefore the physical adsorption onto Cu–BTC@cotton composite can't be ignored. The proposed chemical interactions between ethion and Cu–BTC@cotton composite presented in two different interactions, including Cu–BTC side and cotton side (Fig. 4b). Coordination linkage between sulfur of ethion with copper of Cu–BTC or hydrogen bonding between oxygen of ethion and functional groups of cellulose are both interactions possible to happen. These hypotheses were confirmed by FT-IR spectrum data.
Effect of the amount of Cu–BTC@cotton composite
Fig. 5 shows the removal percentage of ethion insecticide as a function of the amount of Cu–BTC@cotton composite. Two composites with different MOF content of 5% and 10% were used in ethion removal and cotton fabric was used for comparison. Regardless of the material used, by increasing the adsorbent amount, the removal percentage of ethion insecticide was increased. Due to the binding sites of cellulose, cotton fabric showed adsorption affinity towards ethion insecticide. This affirmed the assumption mechanism in Fig. 4b regarding hydrogen bonding between ethion–O and OH of cellulose. Using 312 mg of cotton, the maximum capacity of 45.7% ± 0.7% was reached from 20 mg L−1 ethion. At lower doses of ethion, 10% Cu–BTC@cotton displayed greater efficiency in ethion removal than that of 5%. Accordingly, 25.5% ± 1.2% and 53.4% ± 1.7% were removed from ethion using 50 mg of 5% and 10% of Cu–BTC@cotton, respectively. This was due to the higher MOF content in the 10% sample. The maximum removal percent from ethion was achieved (97.4% ± 0.5%) using 112.5 mg and 200 mg of 10% Cu–BTC@cotton and 5% Cu–BTC@cotton, respectively. Further increase in amount of MOF onto cotton did not show a significant improvement in ethion removal. Thus, for the quantitative removal of ethion from 100 mL of 20 mg L−1, the optimal dosage required from 10% and 5% Cu–BTC@cotton was 112.5 mg and 200 mg, respectively.
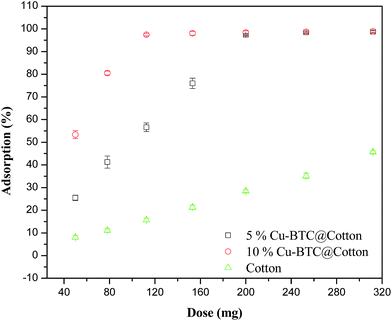 |
| Fig. 5 Effect of the Cu–BTC@cotton dose on ethion insecticide adsorption, 2 mg ethion/100 mL H2O for 180 minutes. | |
From these results two important facts can be concluded as follows: Cu–BTC@cotton composites exhibited extremely high sorption capacity compared to cotton, as cotton treated with 10% of Cu–BTC showed the best results, via the action of binding sites and porous structure, which may have an effect on the sorption of ethion. Augmentation of Cu–BTC on the composite enlarged both active sites and porosity on composite and consequently raised the removal capacity of ethion. These data support and confirm the proposed mechanism between ethion and Cu–BTC@cotton composite.
Effects of time and ethion initial concentration on sorption capacity of Cu–BTC@cotton
As an optimal dose, 112.5 mg from cotton and synthesized composite was used for studying the effect of agitation time (15–180 min) on 20 mg L−1 ethion removal and the results were presented in Fig. 6. For all materials, a plateau shape was observed for ethion removal. The results show that the sorption of ethion was rapid at the initial stages of contact time and then slowed near the equilibrium. This is because of the availability of many active sites for uptake during the initial stage. The adsorption percentage of ethion increased with time and then reached the equilibrium time after 120 min (the maximum time at which no more ethion would be further taken up from the solutions). However, equilibrium was achieved for all adsorbents; the maximum sorption capacity was dependent on the substrate and followed the order 10% Cu–BTC@cotton > 5% Cu–BTC@cotton > cotton. Ethion removal percentage at equilibrium was 97.9% ± 0.3%, 56.6% ± 1.9% and 15.7% ± 0.8% for the same order.
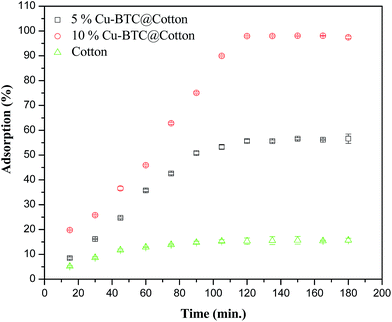 |
| Fig. 6 Effects of time on ethion removal by Cu–BTC@cotton composite. | |
The effect of initial concentration of ethion insecticide (5–35 mg L−1) on adsorption uptake (mg g−1 fabric) is illustrated in Fig. 7. A 112.5 mg of cotton and Cu–BTC@cotton composite was used as adsorbent for 180 minutes contact time. The same plateau shape in the graph was observed, reflecting that ethion uptake from water was increased by increasing the ethion initial concentration up to a certain concentration (optimal concentration = 20 mg L−1). After this ethion concentration, there was no change in ethion uptake on the adsorbent. Sorption of ethion follows the order same as for time effect and the maximum amount was 10 mg g−1, 26.7 mg g−1 and 182 mg g−1 for cotton, 5% Cu–BTC@cotton and 10% Cu–BTC@cotton, respectively. Both time equilibrium and ethion concentration results are in agreement with the proposed mechanism and therefore further confirm the argumentation presented in the interaction of Cu–BTC@cotton with ethion.
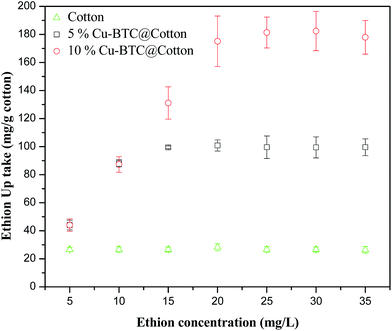 |
| Fig. 7 Effect of the ethion dose on ethion removal by Cu–BTC@cotton composite. | |
Adsorption kinetics and isotherms
For publishing the efficiency of adsorbent and the nature of adsorption process, the kinetics of ethion adsorption on Cu–BTC@cotton composite was studied by two common models, namely, Lagergren's pseudo-first order and pseudo-second order models. The linear plots between log(qe − qt) versus t for pseudo-first order and of t/qt versus t for pseudo-second order models were sketched. The values of rate constants k1 and k2 were calculated from the slope of the corresponding plot. The kinetic parameters (k1 and k2) of ethion adsorption on Cu–BTC@cotton composite are presented in Table 1. By comparing the values of correlation coefficients (R2) for the two models of absorbers, it can be observed that ethion adsorption on composite was well-fitted to the pseudo-second order model. This supports the assumption that the rate-determining step of ethion adsorption on Cu–BTC@cotton composite may be described as a chemical sorption (chemisorptions). In chemisorption, ethion molecule binds with Cu–BTC@cotton composite by forming chemical bonds with the Cu–BTC side and cotton function groups and this is in harmony with the suggested mechanism. The kinetic constant (k2, which is one of the criteria for efficiency of adsorbent) of 10% Cu–BTC@cotton showed the rapid adsorption (more than 10 times) compared to cotton only. The rapid adsorption confirms the role of Cu–BTC in adsorption acceleration due to its highly active sites.
Table 1 Kinetic parameters for ethion insecticide adsorption
Sample |
Pseudo-first order model |
Pseudo-second order model |
K1 (min−1) |
R2 |
K2 × 10−3 (g mg−1 min−1) |
R2 |
Cotton |
0.029 ± 0.002 |
0.786 |
1.24 ± 0.02 |
0.999 |
5% Cu–BTC@cotton |
0.041 ± 0.005 |
0.911 |
10.54 ± 0.04 |
0.998 |
10% Cu–BTC@cotton |
0.048 ± 0.006 |
0.934 |
16.12 ± 0.03 |
0.992 |
Two adsorption isotherms (Langmuir and Freundlich) are examined for ethion adsorption on the Cu–BTC@cotton composite. The related parameters and correlation coefficients (R2) of the two models are tabulated in Table 2. For Langmuir isotherm, the maximum sorption capacity (qm) and equilibrium constant (KL) were both observed to have significantly increased from 3.21 mg g−1 and 2.75 L mg−1 for cotton to 22.72 mg g−1 and 12.75 L mg−1 for 10% Cu–BTC@cotton. This is logically due to an increasing of the binding sites, which was achieved by increasing the amount of Cu–BTC onto cotton and consequently the amount of adsorbed ethion grew. In the case of the Freundlich isotherm, sorption capacity (KF) was considerably enlarged from 2.38 mg g−1 (L m g−1)1/n to 13.49 mg g−1 (L m g−1)1/n using cotton and 10% Cu–BTC@cotton composite, respectively. The increase in KF is a result of increasing the binding sites through increasing the amount of Cu–BTC onto cotton. The value of n for the Freundlich isotherm, which indicates adsorbate–adsorbate interaction, was observed to have been reduced from 14.49 to 3.63. This reflects that the interaction between ethion molecules decreased by increasing binding sites, which encouraged ethion molecules to bind with the composite rather than to interact with themselves.
Table 2 Parameters of Langmuir and Freundlich isothermal adsorption equations of ethion insecticide
Sample |
Langmuir parameters |
Freundlich parameters |
qm |
KL |
R2 |
n |
KF |
R2 |
Cotton |
3.21 |
2.75 |
0.897 |
14.49 |
2.38 |
0.631 |
5% Cu–BTC@cotton |
10.41 |
8.72 |
0.999 |
11.76 |
8.47 |
0.667 |
10% Cu–BTC@cotton |
22.72 |
12.75 |
0.998 |
3.63 |
13.49 |
0.705 |
From the R2 and the fitting lines, it can be observed that the Langmuir model (type I in the IEUPAC system) exhibits a better fit than the Freundlich model. As the Langmuir profile assumes that the surface of adsorbent is homogenous and forms a monolayer adsorption,47–50 the competence of the Langmuir type profile can be ascribed to two reasons. The first is the homogenous distribution of active adsorption sites on the surface of Cu–BTC@cotton composite. However, the main reason is the achievement of monolayer sorption of ethion as a maximum capacity was observed and measured previously. Monolayer sorption is a result of binding between active sites of Cu–BTC@cotton composite and ethion. As presented in the mechanism (Fig. 4b) and confirmed from FTIR, the binding between composite and ethion was carried out through two interactions modes, including the Cu–BTC side and cotton side. In both chemical interactions, linkage between ethion and composite is limited by composite active sites, including the Cu– and functional groups of cellulose, and consequently forms only one layer. Therefore, the proposed mechanism and sorption isotherm behavior are consistent with one another, confirming the monolayer sorption, i.e., each one site is occupied with only one ethion molecule.49,50
Regeneration and reuse of Cu–BTC@cotton
Reuse is one of the important parameters through which sorbent materials can be evaluated. Desorption of ethion from Cu–BTC@cotton composite is an influential factor for studying the regeneration process of composite and subsequent reuse of composite once again. The fact that ethion insecticide is well dissolved in acetonitrile as an organic solvent allowed us to perform desorption experiments of ethion using acetonitrile. By contacting ethion–Cu–BTC@cotton composite with acetonitrile solution, more than 95% of ethion was removed from Cu–BTC@cotton composite and leached out to the acetonitrile medium.
This indicates that Cu–BTC@cotton is successfully regenerated using simple organic solvent. The regenerated composite was conducted to the adsorption process again towards ethion insecticide to show the efficiency of the reuse process. Repetitive regeneration/reuse process represented in consecutive desorption/adsorption was tested to five cycles and the results are shown in Fig. 8. After five cycles, the adsorption efficiency of the Cu–BTC@cotton composite towards ethion was still good and ethion removal percent was over 85%. These results prove that the so-prepared Cu–BTC@cotton is a stable and highly effective adsorbent and can be successfully used for removal of organophosphorus insecticides from wastewater.
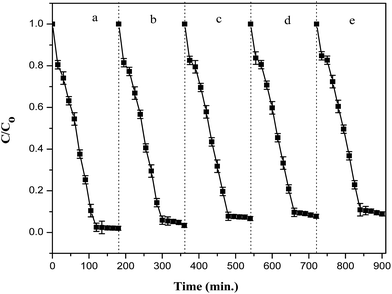 |
| Fig. 8 Regeneration and reuse of 10% Cu–BTC@cotton composites; (a) first cycle, (b) second cycle, (c) third cycle, (d) fourth cycle, (e) fifth cycle. | |
Leaching test for copper
However, removal of pollutants from wastewater is an important fundamental issue, but preventing pollutant release to wastewater is just as important. By the same concept, prevention of the release of copper as an inorganic pollutant to wastewater is as exceedingly important as is removal of ethion insecticides as organic pollutants from wastewater. The adequacy of the proposed sorbent solves the problem of pollutant removal, without leaving a remnant of another pollutant behind. Cu–BTC@cotton composite contains copper and then copper may be released to the surroundings (ethion solution) during the adsorption process of ethion insecticide. Thus, the copper content in solution was analyzed using inductively coupled plasma optical emission spectroscopy after ethion removal via Cu–BTC@cotton composite. For all samples, the concentration of the leached copper in the solution was found to be less than 0.002% (wt/v). This shows the higher affinity of Cu to bind with insoluble cellulose (cotton) than its leaching into the surrounding solution, which is explained by the strong interaction between Cu in the framework and the binding sites of cellulose. The copper release amount is much lower than the maximum contaminant level for copper (0.5 mg L−1) in wastewater according to Austrian legal regulations.51 Therefore, use of the so-prepared Cu–BTC@cotton in removal of organophosphorus insecticides from wastewater is highly effective and safe towards copper release into wastewater, increasing its utility over the present adsorbent materials.
Stability of Cu–BTC and Cu–BTC@cotton in contact with water
The stability of Cu–BTC MOF and Cu–BTC@cotton were tested against water by adding 200 mg of prepared Cu–BTC or 2 cm × 2 cm Cu–BTC@cotton in a vial containing 10 mL water at room temperature. The samples were removed from the solution by centrifuge and filtration at different times (1, 2, 3, 4, and 5 hours; this range was chosen due to the working period of pesticide adsorption). The samples were dried in an oven at 75 °C overnight. Cu–BTC and Cu–BTC@cotton samples were characterized by PXRD to determine any structural changes (Fig. S1 & S2†). The results showed an insignificant change in diffraction patterns over the period of 5 h. This means both of Cu–BTC and Cu–BTC@cotton are stable toward water. Our results are consistent with the previous studies on small periods of contact with water.52–55
4. Conclusions
According to its binding sites and their pore surfaces, MOFs are promising materials for adsorption-related applications. Cu–BTC as MOF material were selected for application onto cotton fabrics using a quite simple technique. Good binding of Cu–BTC with cotton was carried out through chemical interaction between Cu and active sites of cellulose. Different spectroscopic tools confirmed that interaction and characterized the Cu–BTC@cotton composite. Cu–BTC@cotton composite was been successfully developed as a novel adsorbent for the efficient removal of ethion as OP insecticide from an aqueous solution. Feasibility studies of ethion adsorption onto composite suggest that binding sites of composite represented in reactive groups and Cu of cellulose and MOF, respectively, bound ethion via sulfur. Ethion adsorption profile was fitted to the Langmuir model better than the Freundlich model. To remove more than 97% from 20 mg L−1 ethion, a 112.5 mg was an optimal concentration of Cu–BTC@cotton composite and exhibited maximum sorption capacity of 182 mg g−1. After recycling (desorption/adsorption) 5 times, the adsorption efficiency of composite was quite good (>85%). These results confirmed that Cu–BTC@cotton composite is an excellent material for ethion insecticide removal from water, which will create new research challenges to use MOFs@textile composite for pollution remediation from wastewater.
Acknowledgements
The authors acknowledge Prof. Dr H. Kamel, Radioisotopes Department, Atomic Energy Authority, Cairo, Egypt, for his help in the radioactivity measurements.
References
- J. Tan and B. Civalleri, Metal–Organic Frameworks and Hybrid Materials: From Fundamentals to Applications, CrystEngComm, 2015, 17, 197–198 RSC.
- K. Sumida, D. L. Rogow, J. A. Mason, T. M. McDonald, E. D. Bloch, Z. R. Herm, T. Bae and J. R. Long, Carbon Dioxide Capture in Metal–Organic Frameworks, Chem. Rev., 2012, 112, 724–781 CrossRef CAS PubMed.
- L. J. Murray, M. Dincă and J. R. Long, Hydrogen storage in metal–organic frameworks, Chem. Soc. Rev., 2009, 38, 1294–1314 RSC.
- S. Bourrelly, B. Moulin, A. Rivera, G. Maurin, S. Devautour-Vinot, C. Serre, T. Devic, P. Horcajada, A. Vimont, G. Clet, M. Daturi, J. Lavalley, S. Loera-Serna, R. Denoyel, P. L. Llewellyn and G. Férey, Explanation of the Adsorption of Polar Vapors in the Highly Flexible Metal Organic Framework MIL-53(Cr), J. Am. Chem. Soc., 2010, 132, 9488–9498 CrossRef CAS PubMed.
- J. Li, J. Sculley and H. Zhou, Metal–organic frameworks for separations, Chem. Rev., 2012, 112, 869–932 CrossRef CAS PubMed.
- P. Horcajada, T. Chalati, C. Serre, B. Gillet, C. Sebrie, T. Baati, J. F. Eubank, D. Heurtaux, P. Clayette, C. Kreuz, J. Chang, Y. K. Hwang, V. Marsaud, P. Bories, L. Cynober, S. Gil, G. Férey, P. Couvreur and R. Gref, Porous metal–organic-framework nanoscale carriers as a potential platform for drug delivery and imaging, Nat. Mater., 2010, 9, 172–178 CrossRef CAS PubMed.
- K. Xie, Q. Fu, Y. He, J. Kim, S. J. Goh, E. Nam, G. G. Qiao and P. A. Webley, Synthesis of well dispersed polymer grafted metal–organic framework nanoparticles, Chem. Commun., 2015, 51, 15566–15569 RSC.
- M. Kurmoo, Magnetic metal–organic frameworks, Chem. Soc. Rev., 2009, 38, 1353–1379 RSC.
- J. Liu, L. Chen, H. Cui, J. Zhang, L. Zhang and C. Su, Applications of metal–organic frameworks in heterogeneous supramolecular catalysis, Chem. Soc. Rev., 2014, 43, 6011–6061 RSC.
- Z. Hu, B. J. Deibert and J. Li, Luminescent metal–organic frameworks for chemical sensing and explosive detection, Chem. Soc. Rev., 2014, 43, 5815–5840 RSC.
- A. J. Howarth, Y. Liu, J. T. Hupp and O. K. Farha, Metal–organic frameworks for applications in remediation of oxyanion/cation-contaminated water, CrystEngComm, 2015, 17, 7245–7253 RSC.
- E. Haque, J. W. Jun and S. H. Jhung, Adsorptive removal of methyl orange and methylene blue from aqueous solution with a metal–organic framework material, iron terephthalate (MOF-235), J. Hazard. Mater., 2011, 185, 507–511 CrossRef CAS PubMed.
- A. J. Fletcher, K. M. Thomas and M. J. Rosseinsky, Flexibility in metal–organic framework materials: impact on sorption properties, J. Solid State Chem., 2005, 178, 2491–2510 CrossRef CAS.
- C. N. Pope, Organophosphorus pesticides: do they all have the same mechanism of toxicity, J. Toxicol. Environ. Health, Part B, 1999, 2(2), 161–181 CAS.
- D. Sharma, A. Nagpal, Y. B. Pakade and J. K. Katnoria, Analytical methods for estimation of organophosphorus pesticide residues in fruits and vegetables, Talanta, 2010, 82, 1077–1089 CrossRef CAS PubMed.
- S. Devipriya and S. Yesodharan, Photocatalytic degradation of pesticide contaminants in water, Sol. Energy Mater. Sol. Cells, 2005, 86, 309–348 CrossRef CAS.
- K. V. Plakas and A. J. Karabelas, Removal of pesticides from water by NF and RO membranes, Desalination, 2012, 287, 255–265 CrossRef CAS.
- Z. Jia, Y. Li, S. Lu, H. Peng, J. Ge and S. Chen, Treatment of organophosphate-contaminated wastewater by acidic hydrolysis and precipitation, J. Hazard. Mater., 2006, 129, 234–238 CrossRef CAS PubMed.
- M. I. Maldonado, S. Malato, L. A. Perez-Estrada, W. Gernjak, I. Oller, X. Domenech and J. Peral, Partial degradation of five pesticides and an industrial pollutant by ozonation in a pilot-plant scale reactor, J. Hazard. Mater., 2006, 38, 363–369 CrossRef PubMed.
- T. Ahmad, M. Rafatullah, A. Ghazali, O. Sulaiman, R. Hashim and A. Ahmad, Removal of pesticides from water and wastewater by different adsorbents, J. Environ. Sci. Health, Part C: Environ. Carcinog. Ecotoxicol. Rev., 2010, 28, 231–271 CrossRef CAS PubMed.
- R. Leyva-Ramos, L. Fuentes-Rubio, R. M. Guerrero-Coronado and J. Mendoza-Barron, Adsorption of trivalent chromium from aqueous solutions onto activated carbon, J. Chem. Technol. Biotechnol., 1995, 62, 64–67 CrossRef CAS.
- G. Z. Memon, M. I. Bhanger, M. Akhtar, F. N. Talpur and J. R. Memon, Adsorption of methyl parathion pesticide from water using watermelon peels as a low cost adsorbent, Chem. Eng. J., 2008, 138, 616–621 CrossRef CAS.
- Z. Hasan and S. H. Jhung, Removal of hazardous organics from water using metal–organic frameworks (MOFs): plausible mechanisms for selective adsorptions, J. Hazard. Mater., 2015, 283, 329–339 CrossRef CAS PubMed.
- J. George and S. N. Sabapathi, Cellulose nanocrystals: synthesis, functional properties, and applications, Nanotechnol., Sci. Appl., 2015, 8, 45–54 CrossRef PubMed.
- H. E. Emam, A. P. Manian, B. Široká and T. Bechtold, Copper inclusion in cellulose using sodium D-gluconate complexes, Carbohydr. Polym., 2012, 90, 1345–1352 CrossRef CAS PubMed.
- H. E. Emam, A. P. Manian, B. Široká, H. Duelli, B. Redl, A. Pipal and T. Bechtold, Treatments to Impart Antimicrobial Activity to Clothing and Household Cellulosic-Textiles – Why “Nano”-silver?, J. Cleaner Prod., 2013, 39, 17–23 CrossRef CAS.
- T. Bechtold, A. P. Manian, H. B. Öztürk, U. Paul, B. Široká, J. Širokỳ, H. Soliman, L. T. T. Vo and H. Vu-Manh, Ion-interactions as driving force in polysaccharide assembly, Carbohydr. Polym., 2013, 93, 316–323 CrossRef CAS PubMed.
- H. E. Emam, A. P. Manian, B. Široká, H. Duelli, P. Merschak, B. Redl and T. Bechtold, Copper(I) oxide surface modified cellulose fibers—Synthesis, characterization and antimicrobial properties, Surf. Coat. Technol., 2014, 254, 344–351 CrossRef CAS.
- H. E. Emam, S. Mowafi, H. M. Mashaly and M. Rehan, Production of antibacterial colored viscose fibers using in situ prepared spherical Ag nanoparticles, Carbohydr. Polym., 2014, 110, 148–155 CrossRef CAS PubMed.
- H. E. Emam, N. H. Saleh, K. S. Nagy and M. K. Zahran, Functionalization of medical cotton by direct incorporation of silver nanoparticles, Int. J. Biol. Macromol., 2015, 78, 249–256 CrossRef CAS PubMed.
- H. E. Emam and T. Bechtold, Cotton fabrics with UV blocking properties through metal salts deposition, Appl. Surf. Sci., 2015, 357, 1878–1889 CrossRef CAS.
- H. E. Emam, N. H. Saleh, K. S. Nagy and M. K. Zahran, Instantly AgNPs deposition through facile solventless technique for poly-functional cotton fabrics, Int. J. Biol. Macromol., 2016, 84, 308–318 CrossRef CAS PubMed.
- E. López-Maya, C. Montoro, L. M. Rodríguez-Albelo, S. D. A. Cervantes, A. A. Lozano-Pérez, J. L. Cenís, E. Barea and J. A. R. Navarro, Textile/Metal–Organic-Framework Composites as Self-Detoxifying Filters for Chemical-Warfare Agents, Angew. Chem., Int. Ed., 2015, 54, 6790–6794 CrossRef PubMed.
- L. E. Lange and S. K. Obendorf, Functionalization of cotton fiber by partial etherification and self-assembly of polyoxometalate encapsulated in Cu3(BTC)2 metal–organic framework, ACS Appl. Mater. Interfaces, 2015, 7, 3974–3980 CAS.
- H. Abdel-Gawad, R. M. Abdelhameed, A. M. Elmesalamy and B. Hegazi, Distribution and elimination of 14C-ethion insecticide in chamomile flowers and oil, Phosphorus Sulfur Silicon Relat. Elem., 2011, 186(10), 2122–2134 CrossRef CAS.
- H. E. Emam and M. K. El-Bisi, Merely Ag nanoparticles using different cellulose fibers as removable reductant, Cellulose, 2014, 21, 4219–4230 CrossRef CAS.
- S. Lagergren, Zur theorie der sogenannten adsorption geloster stoffe, K. Sven. Vetenskapsakad. Handl., 1898, 24, 1–39 Search PubMed.
- Y. S. Ho and G. McKay, Kinetic model for lead(II) sorption on the peat, Adsorpt. Sci. Technol., 1998, 16, 243–255 CAS.
- I. Langmuir, The constitution and fundamental properties of solids and liquids, J. Am. Chem. Soc., 1916, 38, 2221–2295 CrossRef CAS.
- H. M. F. Freundlich, Uber die adsorption in losungen, Z. Phys. Chem., 1906, 57, 385–470 CAS.
- Progress on Drinking Water and Sanitation, UNICEF and WHO, update, ISBN: 978 9280646320, 2012 Search PubMed.
- S. S.-Y. Chui, S. M.-F. Lo, J. P. H. Charmant, A. G. Orpen and I. D. Williams, A chemically functionalizable nanoporous material [Cu3(TMA)2(H2O)3]n, Science, 1999, 283, 1148–1150 CrossRef CAS PubMed.
- B. Xiao, P. S. Wheatley, X. B. Zhao, A. J. Fletcher, S. Fox, A. G. Rossi, I. L. Megson, S. Bordiga, L. Regli, K. M. Thomas and R. E. Morris, High-capacity hydrogen and nitric oxide adsorption and storage in a metal–organic framework, J. Am. Chem. Soc., 2007, 129, 1203–1209 CrossRef CAS PubMed.
- S. Barkhordari, M. Yadollahi and H. Namazi, pH sensitive nanocomposite hydrogel beads based on carboxymethyl cellulose/layered double hydroxide as drug delivery systems, J. Polym. Res., 2014, 21, 1–9 CAS.
- H. Abdel-Gawad, H. Taha, R. M. Abdel-Hameed and B. Hegazi, 14C-Ethion residues in soybean seeds: metabolic pathway, effect of processing, bioavailability, toxicity and protective action of artichoke leaf powder towards rats, Toxicol. Environ. Chem., 2013, 95, 288–303 CrossRef CAS.
- H. Abdel-Gawad, R. M. Abdel-Hameed and A. Witczak, Residues of 14C-ethion along the extraction and refining process of maize oil, and the bioavailability of bound residues in the cake for experimental animals, Bull. Environ. Contam. Toxicol., 2013, 91, 240–245 CrossRef CAS PubMed.
- C. H. Giles, D. Smith and A. Huitson, A general treatment and classification of the solute: part I. theoretical, J. Colloid Interface Sci., 1974, 47, 755–765 CrossRef CAS.
- C. H. Giles, A. P. D'silva and I. A. Easton, A general treatment and classification of the solute adsorption isotherm: part II experimental interpretation, J. Colloid Interface Sci., 1974, 47, 766–778 CrossRef CAS.
- K. S. W. Sing, D. H. Everett, R. A. W. Haul, L. Moscou, R. A. Pierotti, J. Rouquerol and T. Siemieniewska, Reporting physisorption data for gas/solid systems, Pure Appl. Chem., 1985, 57, 603–619 CrossRef CAS.
- C. H. Bolster and G. M. Hornberger, On the use of linearized langmuir equations, Soil Sci. Soc. Am. J., 2007, 72, 1797–1848 Search PubMed.
- “Bundesminister für Land- und Forstwirtschaft”, Verordnung des Bundesministers für 460 Land- und Forstwirtschaft über die allgemeine Begrenzung von Abwasseremissionen in 461 Fließgewässer und öffentliche Kanalisationen (AAEV), 19. April 1996, 58. Stück, 462 Bundesministerium für Land- und Forstwirtschaft, Bundesgesetzblatt für die Republik 463 Österreich, Wien, Austria 1996, 186, p. 996.
- F. Gul-E-Noor, B. Jee, A. Poppl, M. Hartmann, D. Himsl and M. Bertmer, Effects of varying water adsorption on a Cu3(BTC)2 metal–organic framework (MOF) as studied by 1H and13C solid-state NMR spectroscopy, Phys. Chem. Chem. Phys., 2011, 13, 7783–7788 RSC.
- J. B. DeCoste, G. W. Peterson, B. J. Schindler, K. L. Killops, M. A. Browe and J. J. Mahle, The effect of water adsorption on the structure of the carboxylate containing metal–organic frameworks Cu–BTC, Mg-MOF-74, and UiO-66, J. Mater. Chem. A, 2013, 1, 11922–11932 CAS.
- C. Prestipino, L. Regli, J. G. Vitillo, F. Bonino, A. Damin, C. Lamberti, A. Zecchina, P. L. Solari, K. O. Kongshaug and S. Bordiga, Local Structure of Framework Cu(II) in HKUST-1 Metal–organic Framework: Spectroscopic Characterization upon Activation and Interaction with Adsorbates, Chem. Mater., 2006, 18, 1337–1346 CrossRef CAS.
- J. B. Decoste, G. W. Peterson, M. W. Smith, C. A. Stone and C. R. Willis, Enhanced Stability of Cu–BTC MOF via Perfluorohexane Plasma Enhanced Chemical Vapor Deposition, J. Am. Chem. Soc., 2012, 134, 1486–1489 CrossRef CAS PubMed.
Footnote |
† Electronic supplementary information (ESI) available. See DOI: 10.1039/c6ra04719j |
|
This journal is © The Royal Society of Chemistry 2016 |