DOI:
10.1039/D4PM00191E
(Review Article)
RSC Pharm., 2025,
2, 59-81
Strategies for beating the bitter taste of pharmaceutical formulations towards better therapeutic outcomes
Received
1st July 2024
, Accepted 10th December 2024
First published on 18th December 2024
Abstract
Oral drug delivery remains the most favored method of administration due to its convenience and patient compliance. However, the unpleasant taste of certain medications often leads to poor acceptance, particularly among pediatric and geriatric patients. To address this issue, taste-masking (TM) technologies have emerged as effective solutions for improving the palatability of oral drugs. This review provides an overview of the key features of TM technologies, including the properties of materials used, their mechanisms, and applications in drug delivery. Typically, TM materials work by complexing or encapsulating drug molecules to prevent direct interaction with taste receptors, thus mitigating unpleasant flavors and enhancing the overall sensory experience. The review explores a range of materials—both synthetic and natural—and various TM technologies designed to mask bitter taste. Additionally, it discusses the latest methods for assessing the effectiveness of TM and the current regulatory landscape surrounding the use of these technologies in drug delivery.
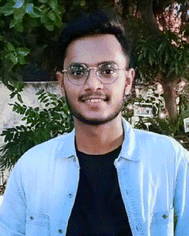 Lohare Rahul Sanjay | Mr Rahul Lohare is an MS student in the Department of Pharmaceutics, National Institute of Pharmaceutical Education and Research, Kolkata, West Bengal, India. His project work is majorly focused on spray dried pharmaceutical materials for solubility improvement, bioavailability improvement, and taste masking. |
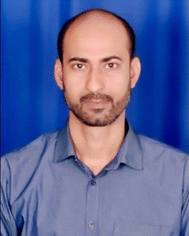 Santanu Kaity | Dr Santanu Kaity is presently working as an assistant professor at the Department of Pharmaceutics, National Institute of Pharmaceutical Education and Research, Kolkata, West Bengal, India. Prior to this, he was associated with the formulation research and development department at Mylan Laboratories Limited, a Viatris company, Hyderabad, India, and at the Pharmaceutical Technology Centre, Centre of Excellence, Cadila Healthcare Limited, Zydus Cadila, Ahmedabad, India. |
1. Introduction
Taste can have several meanings depending on the context, but its primary definition relates to the sense of taste, which is one of the five basic human senses, along with sight, touch, hearing and smell. In this context, “taste” refers to the ability to perceive taste and distinguish between different flavours in food and beverages. It involves the sensory experience of sweet, sour, bitter, salty, and umami (savoury) flavours.1 Specialised taste cells that are grouped together in tiny clusters are called taste buds, which are mostly found on the soft palate and dorsal surface of the tongue and help to detect stimuli.2 These cells are activated by chemical signals, specifically neurotransmitters, which trigger the transmission of taste information to the brain. The brain processes this taste information to create the perception of flavour and generate an appropriate response.3 Chewable tablets, liquids, and suspensions are the most common oral paediatric drugs in the market today. Additionally, orally dissolving films, mini-tablets, and orodispersing/disintegrating tablets are becoming more popular.4 Healthcare professionals face a major problem when it comes to oral delivery of bitter drugs that are prescribed for paediatric patients. A good oral dosage form should have inbuilt bitterness inhibition and reduction ingredients or mechanisms as important aspects to improve patient compliance. The palatability of these formulations has improved through proven techniques for bitterness inhibition and reduction. If reduced bitterness can be achieved to some extent, the creation of oral formulations as alternatives for typically injectable formulations may become more prevalent. If reduced bitterness can be achieved in oral formulations, it could significantly impact the pharmaceutical industry by making oral medications a more viable alternative to injectable formulations. Traditionally, injectables are preferred for drugs with unpleasant tastes or those that require rapid absorption and high bioavailability. However, with advancements in formulation technology that mitigate bitterness, pharmaceutical companies might be encouraged to develop more oral drugs instead of injectables. This shift could be driven by several factors. For one, oral formulations are generally more convenient and less invasive, which could improve patient compliance and reduce healthcare costs associated with injections. In recent years, there has been significant progress in developing compositions that are bitterless, tasteless, or taste-masked. To mask the bitter taste of drug substances, three different approaches are undertaken in general, namely, (a) physical barriers, (b) change taste perception, and (c) change drug solubility.5 To explain more specifically, taste masking can be achieved by the addition of flavouring agents,6 sweeteners and amino acids,7 polymer coating,8 conventional granulation,9 ion-exchange resins,10 spray congealing with lipids,11 inclusion complex formation with cyclodextrins,12 freeze-drying,13 multiple emulsification,14 and also taste masking by gelatin or liposome encapsulation.15 More recently, research has been done with excipients that block the bitter taste receptor and prevent taste perception.16 Thus, any pharmaceutical formulation with a tasty flavour is certainly preferable over a competitor's product, resulting in higher patient compliance and therapeutic benefits as well as increased sales and profits for the company. Numerous formulations with enhanced efficacy and acceptance have been created due to a desire to make these products more palatable. Masking undesirable APIs is desirable if they are included in solid oral formulations, intended to release the API within the oral cavity, for example, orodispersible tablets (ODTs), which in most cases release the medicament in the oral cavity. However, patient compliance may be affected by the bitter taste of many active components; that is why the pharmaceutical industry is investigating new taste-masking technologies to improve the palatability of ODTs. ODTs have been linked to high patient compliance rates, particularly in the case of paediatric, geriatric, and mental patients who lack access to water or who have trouble swallowing conventional tablets.
Taste masked formulations are predominantly developed for paediatric and geriatric populations. Both children and elderly patients often face significant challenges with medication compliance due to the bitter taste of drugs. The unpleasant taste of many therapeutics is a notable barrier, particularly for children, who are highly sensitive to bitter flavours and may strongly refuse to take such medications. Additionally, elderly patients may experience altered taste sensitivity or have difficulty swallowing, further complicating medication adherence. Consequently, taste masking is a critical factor in ensuring patient compliance with therapeutic regimens. Developing novel taste masking technologies is essential to prevent children from experiencing unpleasant tastes and to enhance compliance among paediatric and geriatric patients.
Exact statistics on bitter medicines for children are not readily available, but it is well known that many medications, especially those for serious conditions, have a bitter taste, which can hinder medication adherence in children. In the USA, 1 in 12 children are on psychiatric drugs, many of which are bitter. In Australia, the rates of children on mental health-related medications are 0.2% for those under 5, 4.3% for ages 5–11, and 8.2% for ages 12–17 years. Common bitter compounds include chloroquine (5%), quinine (10%) for malaria, and caffeine (8%) in respiratory medications. Erythromycin (15%) and certain antibiotics like metronidazole (17%) are also noted for their bitterness. Acetaminophen (25%), a widely used pain reliever and fever reducer, and dextromethorphan (20%), a common cough suppressant, are particularly unpleasant in taste. These bitter molecules can significantly affect children's willingness to take their medications, highlighting the need for strategies to mask the bitterness.17
The main objective of this review is to explore the taste masking technologies of bitter drugs in accordance with drug delivery, target use of proper taste masking polymers, taste masking agents, and flavours which are easily compatible with active drugs. This article also reviews various techniques for taste-masked formulation development, strategies for taste masking, instrumental techniques for the measurement of the extent of taste masking, the current regulatory perspective, and the future perspective of taste-masked formulations.
2. Mechanism of taste sensations
Specific ion channels and second messenger systems are needed for the conversion of taste signals from chemical stimuli to electrical impulses for transmission to the brain. The specific type of transduction system used depends on the taste itself.18 As was previously noted, apical ion channels detect salty and sour tastes, while G protein-coupled receptors (GPCRs) detect bitter, sweet, and umami tastes. Various taste sensations in the tongue are shown in Fig. 1, and their mechanism of sensation is shown in Fig. 2.
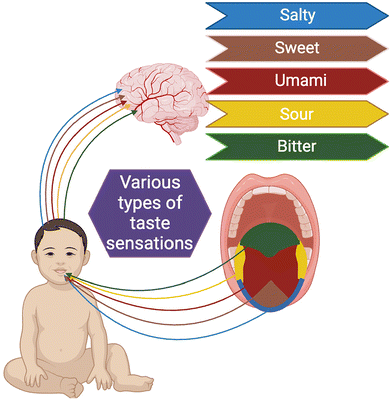 |
| Fig. 1 Schematic representation of the five basic taste sensations on the human tongue. | |
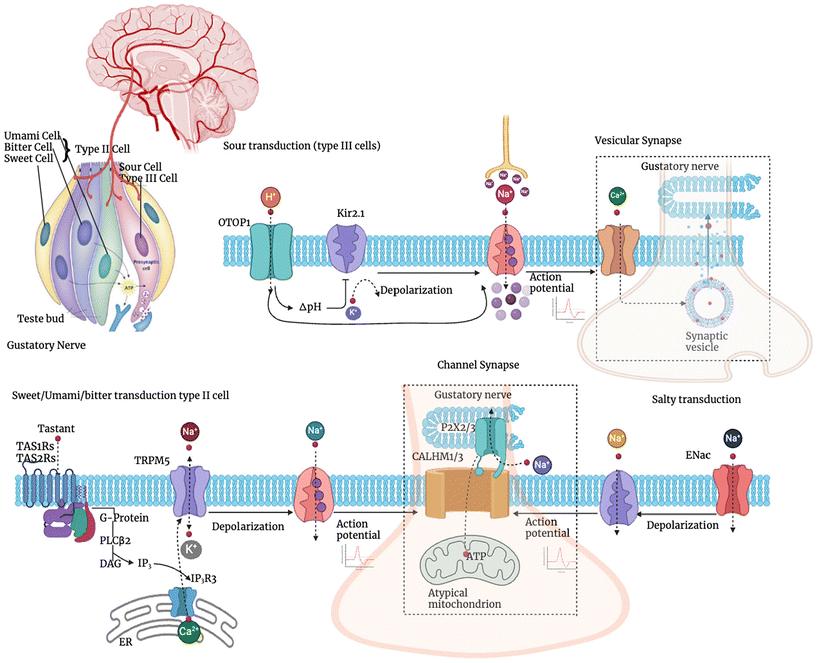 |
| Fig. 2 Signal transduction and taste sensation mechanisms by the human brain.21 | |
2.1. Salty and sour tastes
The taste pores and their gustatory hairs come into contact with the Na+ and H+ ions present in salty and sour molecules, respectively. An intracellular cascade is started when the ions attach to their unique receptors on a taste receptor cell (TRC) inside the taste bud.19 Voltage-gated channels become active as the cell membrane depolarizes. A cell's extracellular calcium influx causes the release of neurotransmitters into the synaptic cleft, which are then transported to the brain via the corresponding cranial nerve.20
2.2. Sweet, bitter, and umami tastes
A GPCR is bound by sugar, alkaloids, or L-amino acids, which activates a common signalling pathway. These GPCRs trigger the phospholipase C (PLC) pathway once they are turned on. Through the activation of inositol triphosphate (IP3) receptors, calcium is released from endoplasmic reticulum storage. With sodium inflow, this calcium release induces membrane depolarisation and the release of neurotransmitters. The connected cranial nerve then transmits taste information to the brain.20
The nucleus tractus solitorius (NTS) of the medulla receives signals from the vagus nerve, the glossopharyngeal nerve, and the chorda tympani branch of the facial nerve. From here, information travels to the postcentral gyrus, where it is submerged by the lateral sulcus, and then to the ventral posteromedial nucleus (VPM) of the thalamus. Gustatory information is also received by the lateral hypothalamus and the central nucleus of the amygdala, which are also involved in the emotional aspect of taste, such as the memory of taste, and contribute to ingestive behaviour.22
Taste buds have their own taste coding. Sweet, bitter, umami, sour, and salt cells are specific taste cells that are responsible for sensing each taste quality. Sweet, bitter, and umami taste cells are grouped together as type II cells because they share the same signalling cascade, but sour taste cells are classified as type III cells. It should be noted that bitter and sour flavours play a key role in enhancing the perception of high salt taste. Traditional vesicular synapses are used for neurotransmission during sour transduction in type III cells, which also involves OTOP1 and Kir2.1 as the H+ sensor and signal amplifier channels, respectively. Although sweet, umami, and bitter type II cells have different types of taste receptors, they all follow the same signalling route in which the activation of TRPM5 channels results in depolarization that causes action potentials to fire. Na+ inflow through ENaC triggers sodium taste transduction by causing supra-threshold depolarization that leads to the production of action potentials. The channel synapse containing CALHM1/3 channels is used by both type II cells and sodium taste cells as the route for action potential-dependent neurotransmitter release. Here, Kir2.1 represents the inward rectifier K+ channel, OTOP1 refers to otopetrin1, phospholipase C2 is represented by PLC2, inositol trisphosphate is abbreviated as IP3, diacylglycerol is expressed as DAG, ER represents endoplasmic reticulum, transient receptor potential melastatin is abbreviated as TRPM, calcium homeostasis modulator is represented as CALHM, and epithelial Na+ channel is represented as ENaC.21
3. Strategies for taste masking
Taste masking is crucial in various industries like pharmaceutical, food and cosmetics to make products more palatable. In the pharmaceutical sector, taste masking is one of the prime important criteria, as for few medicines, bitter taste can influence the treatment outcome also. Particularly in the case of protease inhibitors, the bitter taste can highly impact the therapeutic outcome for acquired immunodeficiency syndrome (AIDS)-affected children. Here in this review, we tried to focus on various taste masking strategies like coating, sweeteners, granulation, microencapsulation, solid dispersion, complexation, pH modifiers, rheological modification and fast dissolving approaches towards developing well-tolerable medications for kids, which can simultaneously improve patient compliance and meet the highest possible therapeutic goals (Fig. 3).
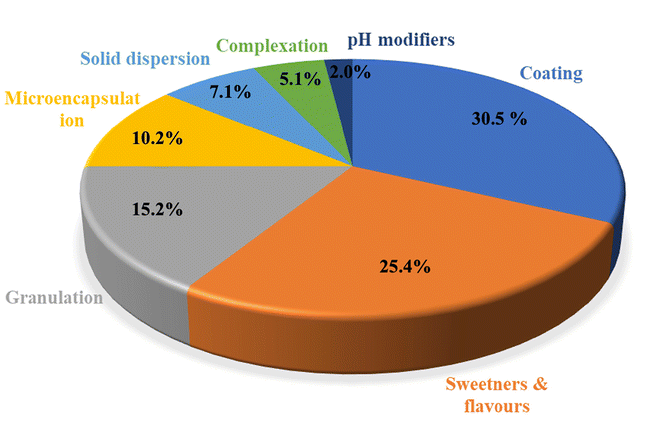 |
| Fig. 3 Currently used taste masking strategies for the development of better formulations. | |
3.1. Taste masking by coating
The most common technique for masking bitter or unpleasant drugs is polymer coating or physical barriers. Active pharmaceutical ingredients coated with a physical barrier successfully give palatable oral formulations, and quick dissolution of the API (drug) can be prevented in the mouth by coating. When the tablet disintegrates, the large, insoluble coated drug particles may remain in the mouth, creating a gritty and sandy feeling in the mouth cavity. This also brings up concerns with traditional tablet formulations since any reduction in palatability is something we want to avoid. Fluidised bed coating is a common approach for coating a core particle in a continuous layer. When using the fluidised bed coating approach, there are typically two types of procedures that can be used—coating with a molten mass or using a polymeric solution. The conventional coating method is commonly used for taste masking. It does need an evaporating solvent from solutions or dispersions to coat the material of interest. This approach offers a wide variety of acceptable polymers that are suitable for masking the taste of bitter drugs or preventing the grittiness of the coated particles. Depending on the granule characteristics of the active ingredients, the polymer content varies from 10 to 40% w/w. A few bitter drug substances which are processed for formulation development using taste masking technology by polymer coating are presented in Table 2. The coating process is categorized based on the solvent system employed, the coating material utilized, and the number of coating layers applied. Hydrophilic polymers, hydrophobic polymers, sweeteners, and lipids are used alone or in combination as coating materials for masking the bitter taste.16
Encasing the bitter-taste drug particles inside a polymer coat lessens the bitterness perception by preventing the drug from dissolving in saliva. Nevertheless, there are drawbacks to this technique as well. These include the potential complexity and expense of the coating process, the requirement for exact control over coating parameters to guarantee uniformity and efficacy, and the potential to alter the drug release profile, which may have an impact on the therapeutic efficacy of the drug and performance of the dosage form.
3.1.1 Types of materials used for taste masking by coating.
Polymer-based taste masking is a common approach used in pharmaceuticals to mask unpleasant taste. For taste masking purpose, various types of polymers are used, namely, natural, semisynthetic and synthetic.
3.1.2 Natural polymers.
The selection of polymers for taste masking of bitter drugs depends on the dosage form, specific drug and formulation requirements. By improving the palatability of drugs while maintaining their therapeutic efficacy, they contribute to enhancing patient compliance and acceptability. Various natural polymers can be included in pharmaceutical formulations to change the bitter taste of different drugs. Natural sources of polysaccharides include algae (alginates), animals (chitosan and chondroitin), plants (pectin, guar gum and mannan) and microbes (dextran and xanthan gum). Many advantageous characteristics of monosaccharide polymers include their high stability, hydrophilicity, lack of toxicity, ability to form gels, biodegradability, and having flexibility for chemical modification by simple techniques.
Various natural substances are employed in the pharmaceutical dosage form to mask the taste of bitter drugs. Gelatin, derived from collagen, forms a tasteless coating around tablets and capsules. Acacia gum, sourced from the acacia tree, provides a glossy coating that can regulate drug release. Alginates, extracted from brown seaweed, are added to liquid formulations for taste masking. Starches like potato, cornstarch, and tapioca are utilized for chewable pills’ coatings.23 Pectin, found in fruits, enhances the taste of chewable tablets or gummies.24 Chitosan, derived from crab shells, aids in both taste masking and drug release enhancement.25 Guar gum, obtained from the guar bean, serves as a binder and taste-masking agent in tablets and granules.26 Agar, derived from seaweed, is used to mask the taste of drugs in oral formulations.27 Xanthan gum, produced by fermenting carbohydrates, acts as a suspending agent and taste-masking agent in liquid formulations.28 Gellan gum, obtained from bacteria, facilitates controlled drug release while masking bitter taste.29,30
3.1.3 Synthetic and semisynthetic polymers.
Polymer selection is crucial for effective taste masking through coating. The performance of the finished dosage form is significantly affected by the physicochemical and biological properties of the polymer, such as its ability to form films, lack of toxicity, and biodegradability.31,32 There are various kinds of synthetic and semisynthetic polymers available, which are frequently used in taste masking, and are majorly categorized as water-soluble and insoluble. By mixing the water-insoluble and water-soluble polymers in a variable ratio, a taste-masking layer can be formed. Water-soluble polymers dissolve, disperse, and swell in water, altering the physical properties of aqueous systems by gelation and thickening. Traditionally, synthetic vinyl polymers and cellulose ethers (methylcellulose and HPMC) are used as water-soluble polymers for masking taste. Polymers and their mixtures that are insoluble at neutral pH are the most effective taste-masking agents. Ethyl cellulose and methacrylic copolymers are frequently used in taste masking. They act as an effective barrier against the transfer of drug molecules to the surface and water molecules to the core because they are insoluble at the neutral pH of saliva.33,34 This produces a taste-masking effect. However, for water-insoluble polymers, organic solvent is not recommended for taste masking. Therefore, various polymers from this class are synthesised in the latex or pseudo-latex form in order to minimise the usage of organic solvents during the coating process. Copolymers of acrylate and methacrylate and copolymers of ethyl acrylate, ethyl cellulose and methyl acrylate occur in the dispersion of the latex or pseudo-latex form.35 Various polymer mixtures like Eudragit® EPO ReadyMix, Aquacoat® ECD, Sepifilm® TMLP, Kollicoat SmartSeal® 30 D and Opadry® AMB are utilized for taste masking of pharmaceuticals.
Examples of major water soluble polymers are methylcellulose – MC (Methocel® and Metolose® SM-4) and hypromellose – HPMC (Pharmacoat®, Sepifilm® LP, Spectracel® and AnyCoat® C); the derivatives of cellulose are included in this group. Cellulose naturally appears as a white, odourless, crystalline powder. Alpha-cellulose, derived as a pulp from fibrous plant sources, is hydrolysed with diluted mineral acid solutions to form it. Different types of cellulose are commercially available in different particle sizes and with different moisture and viscosity levels. Various cellulose ethers can be used in a variety of products.36,37 Some cellulose ethers that are water soluble are methylcellulose (MC), sodium carboxymethyl cellulose (Na-CMC), hydroxypropyl methylcellulose (HPMC), and hydroxyethyl cellulose (HEC). For odour and taste masking applications, MC and HPMC are widely used. Some HPMC-containing film coating products that are commercially available are Spectracel®, Pharmacoat®, AnyCoat® C, and Sepifilm®. The viscosity of the polymer dispersion used improves the effectiveness of taste masking. A list of the most explored synthetic polymers used for the masking of bitter taste is given in Table 1.
Table 1 List of synthetic polymers used for taste masking of pharmaceutical products
Polymer types |
Polymer |
Molecule of interest |
Ref. |
Polymethacrylate |
Eudragit EPO |
Acetaminophen |
38
|
Chlorpheniramine maleate |
39
|
Donepezil HCl |
40
|
Ibuprofen |
41
|
Itopride HCl, prednisone |
42
|
Metoclopramide HCl |
43
|
Ondansetron HCl |
44
|
Quinine sulphate |
45
|
Sumatriptan succinate |
46
|
Trimetazidine HCl |
47
|
Eudragit E100 |
Clarithromycin |
48
|
Ornidazole |
49
|
Ondansetron HCl |
50
|
Ofloxacin |
51
|
Indinavir |
52
|
Sildenafil citrate |
53
|
Naproxen |
54
|
Pirenzepine and oxybutynin HCl |
55
|
Polymethacrylates |
Eudragit L30D-55 |
Ibuprofen and roxithromycin |
56
|
Eudragit L100-55 |
Cefuroxime axetil |
57
|
Cetirizine HCl |
58
|
Verapamil HCl |
58
|
Eudragit L30 D |
Clarithromycin and erythromycin |
59
|
Eudragit S100 |
Roxithromycin |
60
|
Polymethacrylates |
Eudragit RL |
Cefuroxime axetil |
57
|
Eudragit RS |
Enoxacin |
61
|
Eudragit RL 30D |
Cetirizine HCl |
62
|
Kollicoat Smartseal 30 D |
Ornidazole |
61
|
Polyacrylic acid |
Carbopol 934 |
Erythromycin and clarithromycin |
63
|
Carbopol 934P |
Dihydroartemisinin |
64
|
Carbopol 971 |
Enrofloxacin |
65
|
Polyvinyl pyrollidone (PVP) |
PVP K90 + PVAP |
Ibuprofen |
66
|
Kollidon VA 64 |
Acetaminophen |
67
|
Polyvinylacetal diethylaminoacetate |
AEA |
Trimebutine maleate |
68
|
Table 2 Most commonly used coating polymers for taste masking of pharmaceuticals
APIs/drugs |
Materials used |
Technique |
Ref. |
Acetaminophen |
Cellulose acetate butyrate, cellulose acetate, HPC/cellulose acetate, Eudragit EPO |
Film coating |
71
|
Amiprilose HCl |
Sodium alginate and calcium gluconate |
Spray coating |
72
|
Levofloxacin |
Cellulose acetate, Eudragit E100 |
Enteric coating |
73
|
Nicorandil |
D-Mannitol, lactose and croscarmellose sodium |
Granulation and coating |
74
|
Diclofenac |
Ethyl cellulose |
Granular coating |
75
|
Oxybutynin and pirenzepine |
HPC, MCC, Eudragit E100 |
Dispersion coating. |
76
|
Bifemelane HCl |
Sucrose, PEG, glycerin monostearate, Eudragit L-30-D-55 |
Coating and spraying |
7
|
Cetraxate HCl |
Eudragit S-100, Macrogol-6000, corn starch |
Melt granulation and coating |
7
|
Ibuprofen |
Mannitol, propylene glycol, Eudragit L300 and flavour |
Spray coating |
41
|
Tramadol hydrochloride |
Eudragit E 100 |
Mass extraction technique |
77
|
Terfenadine |
Carrageenan, sodium alginate, macrogol-400 |
Mixing |
7
|
Morphine HCl |
Eudragit NE 30D |
Pellet coating |
78
|
Famotidine |
HPMC, HPC, cellulose acetate |
Rotogranulation and coating |
79
|
Enoxacin |
HPMC, EC, HPC, Eudragit RS |
Granulation and coating |
80
|
Sparfloxacin |
HPMC, HMC/EC, EC, titanium dioxide, L-HPC and sucrose fatty acid ester mixture |
Granulation and coating |
7
|
Cefeanel daloxate HCl |
EC, HPMC, PVP, trisodium citrate |
Granulation and coating |
7
|
Triprolidine HCl |
HPMC |
Dispersion coating |
81
|
Ibuprofen |
Methacrylic acid copolymer (Eudragit) |
Air-suspension coating |
82
|
Propantheline bromide |
EC, L-HPC |
Granulation and coating |
7
|
Pinaverium |
Shellac or cellulose |
Film coating |
7
|
Aspirin |
Triacetin and cellulose acetate latex |
Rotogranulation and coating |
83
|
A water-insoluble polymer cellulose ether, i.e., ethyl cellulose (EC) (Aquacoat®, Ethocel® and Surelease®), was synthesized by the reaction of alkali cellulose with ethyl cellulose. There are mainly aqueous and organic dispersions of ethyl cellulose. EC is a powder or granular material which is white to light tan in colour and has a melting point between 240 and 255 °C. It is mostly utilised in oral formulations and is stable against light, oxygen, heat and chemicals. The extent of drug release is varied with the molecular weight of ethyl cellulose. Ethyl cellulose is widely utilised in the microencapsulation process as a modified release polymer in addition to exhibiting taste masking capability. Ethocel® is a commercially available product of ethyl cellulose. It dissolves in a variety of solvents, including natural oils, aliphatic alcohols, and chlorinated solvents. It is practically insoluble in propylene glycol, glycerine and water.69 Eudragit® E PO, Eudragit® E 12.5, Eudragit® E PO ReadyMix, Eudragit® E 100 and Kollicoat SmartSeal® 30 D are the copolymers based on acrylic and methacrylic acids. They are produced by polymerising methacrylic and acrylic acids or their esters. Through the formation of salts, poly(meth)acrylates become soluble in digestive fluids; pH-dependent release of the active component is made feasible by the acidic or alkaline groups in Eudragit® L, S, and E polymers. They are used to control drug solubilization by covering or concealing the drug in direct contact with the fluids present in the mouth cavity by exhibiting taste-masking properties. Eudragits are categorised into cationic, anionic and neutral polymers. To mask a bitter taste and keep moisture out, manufacturers frequently use the cationic Eudragit series E.70
3.2. Taste masking by sweeteners, flavours and amino acids
A straightforward approach for taste masking in formulations like oral liquids, chewable tablets or other paediatric formulations is generally achieved by addition of sweeteners, flavours and amino acids. However, for highly water soluble and highly bitter drugs, this technique is not successful. To increase the efficiency of these techniques, flavours and artificial sweeteners are used with other taste masking techniques. Selection of flavour is very crucial for achieving the desirable extent of taste masking in highly bitter drugs. The details of flavouring agents containing paediatric formulations are given in Table 3. Many drugs administered to the oral cavity, such as mouthwashes and dentifrices, often lead to an unpleasant taste. To counteract this, the addition of isoborneol, borneol, or fenchone can effectively mask the undesirable flavours of certain formulations. This is particularly useful for cough drops and mouthwashes that contain medicinal, bitter-tasting ingredients like eucalyptus oil. Some flavouring agents have a cooling effect that helps individual feel less bitter. Primarily, numbness in the taste buds quickly gives a cooling effect after administration. Although the product's temperature has not changed physically, the brain still perceives it as cool. Janovasky and Wesley proposed a list of recommended flavours for masking a particular taste sensation.84
Table 3 A few marketed formulations with specific flavouring agents
Dosage form |
Dosage form |
Flavouring agent |
Ref. |
Cefuroxime axetil |
Granules for oral suspension |
Tutti frutti flavour |
85
|
Raltegravir |
Granules for oral suspension |
Banana with natural flavour |
86
|
Azithromycin |
Microsphere for oral suspension |
Artificial cherry flavour |
87
|
Abacavir sulfate |
Oral solution |
Artificial strawberry and banana flavour |
87
|
Dolasetron mesylate |
Injection solution (for oral dosing) |
Apple or apple grape juice |
87
|
Amprenavir |
Oral solution |
Artificial grape bubblegum flavour |
87
|
Fluoxetin HCl |
Oral solution |
Mint |
87
|
Ritonavir |
Oral solution |
Creamy caramel flavour |
87
|
It is common practice to use sweeteners, flavours, and amino acids to mask the taste of supplements and medications to make them more palatable. Sweeteners such as sucralose and aspartame can effectively mask bitter taste, increasing the product's acceptability, particularly for younger consumers. However, their use raises questions about potential allergic reactions and long-term health effects. Although flavours like fruit or mint have a pleasant and adaptable masking effect, they can also be overpowering or not to everyone's taste. Amino acids, such as leucine and glycine, can lessen the bitterness and increase the umami flavour, providing a natural substitute with extra nutritional advantages; however, they may change the pH of the product or interact with the active ingredients. Achieving efficient taste masking without sacrificing the product's stability, efficacy, or safety still has numerous unattended gaps which require extensive research.
3.2.1 Types of flavours.
Flavours are divided into two main categories: natural and synthetic. Natural flavours encompass a variety of sources, including spirits like lemon and orange extracts, raspberry juices, blackcurrant syrups, and aromatic elements like anise and cinnamon waters. Extracts such as liquorice, tinctures like ginger, and aromatic oils like peppermint and lemon are also derived from natural sources. On the other hand, synthetic flavours are artificially created and commonly found in powdered form, alcoholic solutions, or aqueous solutions. These synthetic options offer consistency and versatility in flavour profiles across a broad selection of products. Table 4 presents a list of both natural and synthetic flavouring agents and sweeteners used for taste masking.
Table 4 Different drug substance specific natural/synthetic sweeteners and flavours used for bitter taste masking
Drug substance |
Sweeteners/flavouring agents |
Evaluation method/study population |
Ref. |
Natural taste masking agents
|
Azithromycin or clarithromycin |
Mixture of dry syrup and acidic jellies |
Taste sensor |
88
|
Buprenorphine/naloxone |
Menthol and sucralose |
Healthy volunteers |
89
|
Cefuroxime axetil |
Stearic acid and sucrose |
Dissolution, human volunteers |
90
|
Bactramin C and polymyxin B sulfate |
Food additive BMI-60 |
Adult and child volunteers |
91
|
Dexamethasone |
Thaumatin and sucralose |
Breast cancer patients |
92
|
Donepezil hydrochloride |
Ammonium glycyrrhizinate |
Human volunteers |
93
|
Epinephrine |
Citric acid |
Electrical tongue |
94
|
Propranolol hydrochloride |
Simple syrup |
Children |
95
|
Midazolam |
Strawberry syrup |
Children |
96
|
Midazolam |
Sucrose and cyclodextrin |
Solubility and dissolution |
97
|
Probiotics and microbial food supplements |
Tropical juices |
Trained panel |
98
|
Quinine, caffeine and salicine |
Flavanones from Yerba santa |
Panelists |
99
|
Quinine hydrochloride |
Sucrose |
Taste sensor |
100
|
Ginseng |
Dark chocolate |
Panelists |
101
|
Quinine |
Cocoa or chocolate-flavoured |
Adult volunteers |
102
|
Diclofenac |
Mint, liquorice flavour and sucralose |
Electrical tongue |
103
|
Synthetic taste masking agents
|
Chloroquine phosphate |
Aspartam |
Human panel |
104
|
Ibuprofen |
Dissolution test |
105
|
Ondansetron |
Human volunteers |
106
|
Ranitidine HCl |
Children and adults |
107
|
Metformin |
Acesulfame-isomalt |
Self-made questionnaire |
108
|
Quinine sulfate |
Acesulfame potassium |
Electrical tongue |
109
|
Famotidine menthol (flavours) |
Menthol (flavour), aspartame (sweetener) |
Healthy volunteers |
110
|
Polyphenols of olive leaf extract |
Sucrose and sodium cyclamate |
Trained sensory panel |
111
|
Hydrocortisone |
Neotame |
Young healthy adults |
112
|
According to a study of the tastes of all humans, sweet is highly agreeable to our species. For this purpose, several types of sweeteners are used. The widespread natural and artificial sweeteners utilised in medications, together with their comparative sweetness levels and pertinent comments, are presented in Table 5. The values presented as relative sweetness levels indicate how sweet each substance is compared to sucrose (table sugar), which serves as the standard with a sweetness level of 1. These levels are determined through sensory evaluation tests involving human taste panels. Participants taste solutions of each sweetener at different concentrations and compare them to a standard sucrose solution. The sweetness level is quantified by how much of the sweetener is needed to achieve the same perceived sweetness as the standard sucrose solution. For example, a relative sweetness of 200 for aspartame means that aspartame is 200 times sweeter than sucrose, meaning much less aspartame is required to achieve the same sweetness level. The determination involves both controlled laboratory settings and statistical analysis to ensure accuracy and reliability in the perceived sweetness comparison. This method helps in identifying and quantifying the potency of various sweeteners, enabling their appropriate application in food and beverage formulations.
Table 5 Sweeteners commonly used along with their relative sweetness levels113
Sweeteners |
Comparative sweetness level |
Comment |
Sucrose |
1 (standard) |
Most commonly used |
Mannitol |
0.60 |
Negative heat of solution |
Lactose |
0.16 |
High amount is required |
Cyclamate |
40 |
Banned |
Glycerrhizine |
50 |
Moderately expensive |
Acesulfame potassium |
137–200 |
Bitter in high concentration |
Aspartame |
200 |
Less stable in solution |
Sucralose |
600 |
Synergistic sweetening effect |
Saccharine |
500 |
Bitter in high concentration |
Aspartame is a common sweetener used for the reduction of bitterness. For bitterness reduction of 25% acetaminophen, a very small concentration (0.8%) is required. The US-FDA banned cyclamate in 1970 due to its carcinogenic activity. Neohesperidin dihydrochalcone serves as an artificial flavour enhancer and suppresses bitterness.
3.3. Taste masking by microencapsulation
Microencapsulation is crucial across industries like pharmaceutical, offering multiple advantages towards efficient drug delivery. Among these, coacervation-phase separation, fluidized bed, solvent evaporation, and spray drying, as well as spray congealing, stand out for their efficacy in taste masking. These techniques involve encapsulating active ingredients within a protective shell, ensuring taste neutrality or controlled release. Additionally, methods such as air suspension coating, pan coating, multi-orifice-centrifugal processes, and interfacial polymerization also contribute to diverse microencapsulation applications, each offering unique advantages tailored to specific product requirements. Microencapsulation involves enveloping tiny solid or liquid particles with polymeric materials or films, effectively preventing oxidation, volatilization, and masking of undesirable drug tastes.114 The technique relies primarily on evaporation or solvent extraction principles, although variations such as spray drying and coacervation-phase separation are also employed for microencapsulation.115
Selecting the right polymer for microencapsulation is a complex task. The chosen coating polymer should hinder the release of the active ingredient in the mouth while facilitating release in the stomach or small intestine for absorption. An ideal polymer for taste masking should be insoluble in saliva with a pH value of 6.8, but should readily dissolve at a pH value of 1.2. Eudragit E, a commonly employed taste-masking polymer in microencapsulation, comprises butyl methacrylate, methyl methacrylate, and dimethylaminoethyl methacrylate. Characterized as a cationic polymer with a glass transition temperature of 48 °C, Eudragit E forms a permeable, insoluble, and swellable film at pH 5 or above, yet easily dissolves via salt formation at acidic pH levels below 5. This property allows Eudragit E to prevent drug release in saliva with a pH range of 6.8 to 7.4 while promptly dissolving in gastric fluid with a pH range of 1.0 to 1.5, making it a suitable choice for microencapsulation applications.116 A few examples of taste-masked drug products developed by the microencapsulation technique are presented in Table 6.
Table 6 Example of taste masked drugs by the microencapsulation technique
Drug |
Taste masking ingredient |
Microencapsulation technique |
Ref. |
Atomoxetine HCl |
Methacrylate copolymer |
Fluidised bed |
117
|
Diclofenac |
Eudragit E |
Fluidized bed |
118
|
Berberine hydrochloride |
Eudragit E |
Fluidized bed |
119
|
Naproxen sodium |
Eudragit E |
Fluidized bed |
54
|
Lafutidine |
Ethyl cellulose and hypermellose |
Fluidized bed |
9
|
Prednisolone |
Eudragit E |
Spray drying |
120
|
Amlodipine and famotidine |
Ethylcellulose |
Spray drying |
121
|
Sildenafil citrate |
Eudragit E |
Spray drying |
53
|
Acetaminophen |
Eudragit E |
Spray congealing |
122
|
Chlorpheniramine maleate |
Chitosan/alginate |
Ionotropic gelatin |
123
|
Ciprofloxacin |
Methacrylic acid divinyl benzene copolymer |
Interfacial polymerisation |
124
|
Drotaverine hydrochloride |
Polyvinyl pyrrolidone |
Solvent evaporation |
125
|
Ondansetron hydrochloride |
Eudragit E |
Solvent evaporation |
126
|
Taste masking through microencapsulation covers bitter or unpleasant-tasting medications in a tasteless or pleasant-tasting coating, which has various benefits, including increased patient compliance, especially for paediatric and geriatric populations. By controlling the release rate and shielding the active ingredient from deterioration, this method improves the stability and bioavailability of the medication. Its drawbacks include higher production costs, possible manufacturing process complexity, and the possibility of changed drug release profiles in the event that encapsulation is not properly controlled. Regulatory obstacles and the requirement for comprehensive testing to guarantee the security and effectiveness of the encapsulated product may also arise.
3.4. Nanotechnology for taste masking
Nanotechnology has made remarkable strides in the field of taste masking, offering innovative solutions that were once beyond reach. Traditional methods of taste masking often involve using large quantities of excipients or sweeteners to cover up unpleasant flavours, which can alter the intended properties of a product. Nanotechnology, however, utilizes nanoparticles and nanostructures to provide a more sophisticated approach. By manipulating particles at the nanoscale, researchers can create highly effective taste-masking agents that operate on a molecular level. For instance, nanoparticles can encapsulate bitter or undesirable compounds, preventing them from interacting with taste receptors on the tongue. This encapsulation ensures that the unpleasant flavours are released only under specific conditions, such as during digestion, thus leaving the taste profile of the product intact. Moreover, nanotechnology enables the development of targeted release systems, where the taste-masking agent is designed to dissolve or degrade only at certain pH levels or temperatures, further enhancing the control over flavour perception. These advancements not only improve the palatability of pharmaceuticals and nutraceuticals but also have applications in the food and beverage industries, where they can create products with a more appealing taste without compromising on efficacy or safety. As research continues to evolve, the integration of the possibilities of product formulation paves the way for a new era of flavour management.127 The details of various nanotechnology-based taste masking technologies for pharmaceuticals are described here.
3.4.1. Liposomal carriers for taste masking.
The development of liposomal formulations has been very promising in overcoming the bitterness of poorly palatable drugs. For instance, mefloquine is an extremely bitter antimalarial drug that was encapsulated in liposomes to improve its acceptability for liquid pediatric dosage forms. Liposomal entrapment efficiently masked the bitterness of the drug, and the encapsulation was achieved by a solvent-assisted loading method that utilized an ammonium sulfate gradient. Liposomes were prepared using 1,2-distearoyl-sn-glycero-3-phosphocholine (DSPC) and cholesterol as lipid components.128 The study also looked into the preparation of liposomes that carry loratadine and how they can help mask taste, achieve better solubilization, and improve its absorption in the body. Prepared using a phospholipid/cholesterol composition and thin-film hydration process, the study showed that liposomes acted as barriers and largely improved the bitterness of orodispersible loratadine and may work with better solubilization and absorption in the human body.129 Additionally, caffeine, one of the most bitter drugs known, was loaded into chitosan-coated nanoliposomes, known as chitosomes. Chitosomes were prepared using a combination of lecithin, cholesterol, and polysorbate 80. After coating with chitosan on the outer layer, they are hydrated by the thin-film hydration method. The taste-masking efficiency was excellent compared to plain caffeine preparation, while the stability was superior to plain liposomes.
3.4.2. Polymeric nanoparticles for taste masking.
A novel taste-masked oral powder formulation of ibuprofen has been developed utilizing a polymeric nanoparticle approach via spray drying. It contains Eudragit L 100 (methacrylic acid–methyl methacrylate copolymer (1
:
1)) as the pH-sensitive polymer that will not dissolve at acidic pH, while the formulation includes PVP K30 and β-cyclodextrin. The prime aim here was to make the formulation as palatable and soluble as possible for the drug. The study emphasized that the ratio of the components like β-cyclodextrin and PVP K30 along with the methacrylic acid–methyl methacrylate copolymer relative to ibuprofen needs to be optimized to yield an effective formulation.130 Saquinavir-filled nanoparticles were formulated for developing a pediatric oral formulation as a taste mask and stabilizer. Nanoparticles were prepared using interfacial polymerization incorporating a methacrylic acid–methyl methacrylate copolymer 1
:
2 (Eudragit RS 100), pullulan, and triglycerides produced from capric and caprylic acids. Nanoparticles were successful in reducing the bitterness of the compound, making it more appropriate for pediatric administration.131
3.4.3. Solid lipid nanoparticles for taste masking.
In a recent study, Zhang et al. prepared reverse solid lipid nanoparticles of atomoxetine hydrochloride for masking the bitterness of the active. The formulation process began with the preparation of plain liposomes, which were then mixed in equal proportions with a solution containing atomoxetine hydrochloride. It was then freeze-dried in a powder composed of the drug and phospholipid, followed by mixing in a medium chain triglyceride to produce an aqueous-clear suspension. It effectively masked the bitterness of the drug.132 Chao Li et al. prepared enteric-coated SLNs for enrofloxacin to increase its palatability, stability, and oral bioavailability. The SLNs were prepared using hot homogenization, followed by ultrasonic emulsification techniques with octadecanoic acid as the lipid matrix. Polyvinyl alcohol was used as an emulsifier and polyacrylic resin was used for enteric coating in this study. The research showed that these enteric-coated SLNs efficiently masked the bitter taste of enrofloxacin while increasing its stability and bioavailability.133 Dandagi et al. prepared SLNs loaded with quinine sulfate to mask its very bitter taste. The lipid carrier used was glyceryl monostearate, while the surfactants were polysorbate 80, poloxamer 407, and poloxamer 188. The results showed that SLNs are highly effective in taste masking and made quinine sulfate tolerable for administration purposes.134
3.4.4. Taste masking by nanostructured lipid carriers.
Akhoond Zardini et al. prepared nanostructured lipid carriers (NLCs) in order to improve the solubility and hide the bitterness of lycopene. Ultrasonication in combination with a high-shear homogenization technique was used for NLC preparation. Glyceryl monostearate and glyceryl distearate served as solid lipid precursors, whereas caprylic/capric triglyceride served as a liquid lipid precursor. Co-surfactants that are used in this study for stabilization purposes include Tween 80 and lecithin. The lycopene-loaded NLCs, when added to orange juice, successfully masked the characteristic bitterness of lycopene and improved its solubility, rendering it organoleptically acceptable.135
3.4.5. Polymeric micelles and taste masking.
Li et al. investigated the potential of amphiphilic block copolymers as micelle-forming agents for taste masking in aqueous solutions. The study tested the ability of four water-soluble bitter drugs—berberine hydrochloride, quinine sulfate, gentopicroside, and matrine—to be masked by amphiphilic block copolymers. The specific copolymers used were mPEG2000-PCL2000, mPEG2000-PLLA2000, PLLA2000-PEG2000-PLLA2000, and mPEG2000-PLGA50/502000. The results demonstrated that these copolymers could effectively encapsulate the bitter drugs, reducing their bitter taste in aqueous solutions.136
3.4.6. Taste masking by reverse micelle nanoparticles.
Huang et al. developed a taste masked azithromycin formulation by using freeze-drying method-based reverse micelles. In this approach, phospholipids and azithromycin were mixed in medium-chain triglycerides and then lyophilized to obtain the dried powder. The drug was encapsulated within lecithin micelles in the medium-chain triglyceride matrix, creating a physical barrier for the API and isolating the drug from taste receptors. The study inferred that the reverse micelles effectively masked the bitter taste of azithromycin, making it more palatable.137
3.4.7. Taste masking by nanosponge technology.
Omar et al. fabricated nanosystems (NSs) of griseofulvin using cyclodextrin to improve its bioavailability and also to improve the taste of the developed formulation. Three different plain β-cyclodextrin nanosystems were prepared using a reaction based on cross-linking, where β-cyclodextrin was cross-linked with diphenyl carbonate (DPC) in the presence of ultrasonication. This approach aimed to enhance the solubility, taste masking, and bioavailability of griseofulvin.138
3.4.8. Taste masking by nanohybrid technology.
An inorganic clay, montmorillonite (MMT) intercalated sildenafil (SDN), as a nanohybrid system was developed for taste masking. After intercalation, the nanohybrid system of SDN-MMT was coated with polyvinylacetal diethylaminoacetate (AEA), a basic polymer. The AEA-coated SDN-MMT nanohybrid exhibited slower dissolution at neutral pH when compared with a marketed sildenafil formulation, Viagra® tablet. This reduced dissolution resulted in minimum exposure of the drug to taste receptors in the mouth, thereby improving the taste experience in the buccal cavity.139
3.5. Taste masking by complexation (inclusion complexation)
To mitigate the perception of bitterness, the complexing agent can achieve its goal by either diminishing the oral solubility of the drugs post-consumption or reducing the exposure of taste buds to the drug particles.16 Low-dosage drugs are the most suitable for this strategy. Due to its ability to create inclusion complexes with a range of molecules without covalent bonds, cyclodextrin is the most commonly used complexing agent for inclusion-type complexes. It is a cyclic oligosaccharide that is sweet, non-toxic, and made from starch.140,141 A list of formulations in which complexation-based taste masking technology was applied for better formulation development is presented in Table 7.
Table 7 Taste masking of bitter drugs by complexation
Drug |
Complexing agent |
Dosage form |
Ref. |
Carbepentane citrate |
Cyclodextrin |
Oral liquid |
7
|
Benexate hydrochloride |
Cyclodextrin |
Granules |
7
|
Ibuprofen |
Hydroxy propyl-β-cyclodextrin |
Solution |
7
|
Chloroquine phosphate |
Tannic acid |
Syrup |
142
|
Gymnema sylvestre
|
Chitosan |
Oral liquid |
7
|
Dimenhydrinate |
Eudragit S-100 |
Chewable tablet |
143
|
Benzethonium chloride |
β-Cyclodextrin |
Mouthwash |
140
|
Dioscin |
β-Cyclodextrin |
Solid oral |
144
|
Benexate |
β-Cyclodextrin |
Powder |
140
|
Hexitidine |
HP-β-cyclodextrin |
Aqueous solution |
140
|
Acetaminophen |
β-Cyclodextrin |
Pellet |
145
|
Pinaverium bromide |
β-Cyclodextrin |
Tablet |
145
|
Libexine HCl |
β-Cyclodextrin |
Oral solid |
145
|
Metronidazole benzoate |
γ-Ccyclodextrin |
Aqueous solution |
146
|
There are a number of benefits and drawbacks to taste masking by complexation, especially when it is achieved through inclusion complexation. Its ability to successfully cover up the bitter taste of medications without appreciably changing their pharmacological characteristics is one of its main advantages. This method isolates the bitter or unpleasant taste from the taste buds by trapping the drug molecules inside the cavity of cyclodextrins or other appropriate complexing agents. This can improve adherence to treatment, particularly in the case of younger and older patients who are frequently sensitive to bitter drugs. Additionally, inclusion complexes can increase some medications’ solubility and stability, which may increase their bioavailability. There are a few disadvantages of this strategy, though. The size and compatibility of the drug molecule with the complexing agent, which may not be appropriate for all drugs, can restrict the formation of inclusion complexes. Furthermore, the procedure might necessitate complex manufacturing methods and be expensive. Additionally, there is a chance that the drug's release rate from the complex may be impacted, which could have an effect on the medication's effectiveness.
3.6. Taste masking by granulation
Granulation stands out as a cost-effective, rapid, and easily scalable method for taste masking. In this approach, bitter-taste drugs are combined with flavours, waxes, and polymers, serving as granulating agents. During the granulation process, taste masking can be achieved using liquid and low melting point waxes, such as glyceryl behenate, glycerol palmitostearate, and hydrogenated castor oil. The formulation of taste-masked oral solid or liquid dosage forms involves processing mixtures of bitter drugs, hydrophobic polymers, sweeteners, lipids, or waxes through dry, wet or melt granulation techniques. Granulation effectively reduces the exposure of the bitter component to the tongue upon oral consumption, yet the extent of bitterness masking may vary. Nevertheless, taste-masked granules incorporating saliva-insoluble polymers can be formulated into various tablet forms, including rapidly dissolving tablets and chewable tablets.147 To mask the taste of calcium-containing substances like calcium carbonate, melt granulation is used. The granules produced by melt granulation have a calcium-containing substance with a sugar alcohol as a binding agent and have a pleasant taste and mouth feel. To enhance the effectiveness of taste masking, sugar alcohols, flavours, and other ingredients are also added to the mixture. Various examples of taste masking of bitter drugs by using the granulation technique are presented in Table 8.
Table 8 Taste masking of the bitter drugs by using a granulating agent148
Drug |
Granulating agent |
Category |
Alprazolam |
Eudragit E 100 |
Anxiolytic |
Clopidogrel sulphate |
Sugar alcohol, castor oil |
Antiplatelet |
Calcium compound |
Sugar alcohol |
Mineral supplement |
Dextromethorphan |
Cyclodextrin |
Antitussive |
Erythromycin |
Alginic acid |
Macrolide |
Granisetron HCl |
Glycerol palmitostearate or glyceryl stearate |
Antiemetic, anti-nauseant |
Ibuprofen |
Microcrystalline cellulose (MCC) |
Anti-inflammatory |
Levofloxacin |
Sugar alcohol, castor oil |
Fluoroquinolone antibiotic |
Macrolide antibiotic |
Polycarbophil |
Macrolide |
Ondansetron |
Polacrillin potassium |
Anti-nauseant, antiemetic |
Penicillin and macrolide |
Wax or hydrogel |
Antibiotics |
Pristinamycin and telithromycin |
Beeswax or glyceryl stearate |
Macrolides |
Vitamins |
Polyglycerol ester of polyvalent fatty acid |
Diet supplement |
Granulation or coating the active pharmaceutical ingredient (API) inside granules improves palatability by delaying the patient's perception of an unpleasant taste. This approach has the potential to improve the stability and controlled release of the API, and it also improves patient compliance, particularly in children and those having high sensitivity towards odd tastes. Nevertheless, drawbacks include higher production costs and complexity, possible difficulties maintaining consistency and uniformity in taste masking, and the potential for partial taste exposure due to granules’ residual release of the API in the mouth.
3.7. Taste masking by ion exchange resin complexation
Ion-exchange resins (IERs) are characterized by high molecular weight polymers featuring both anionic and cationic functional groups. These cross-linked polyelectrolytes are water-insoluble and possesses numerous binding sites, thus exhibiting a high capacity for drug loading. Cation and anion exchange resins represent the two primary classifications of ion exchange resins based on the charge carried by their functional groups. Within each category, they are further categorized as strong or weak, depending on their affinity for counter ions. Anionic resins primarily exchange chloride ions, whereas cation exchange resins typically exchange sodium, potassium, or aluminium salts. For the drug loading on resins, both column and batch methods are used.144 IERs, through weak ionic bonding with oppositely charged drug molecules, form insoluble resonates. After ingestion, in the digestive system, resin exchanges the drug with a counter ion and the drug is eluted and absorbed. IERs like amberlite are used in taste masking of dextromethorphan and made into fast-acting and oral films. To produce the best taste masking agent, the right and careful selection of IER is necessary. Along with the resin's biocompatibility and biodegradability, the concentration of the exchangeable group, adequate functional group, and swelling ratio determine the choice. IERs are mainly classified into four groups: strong acid cation resins (including sulphuric acid species like indion 244, amberlite IRP-69, amberlite IR-120, and tulsion T-344) can be used to mask the taste of basic drugs, because they work across the full pH range; weak acid cation exchange resins like Amberlite IRP-64, Tussion T-335 and Indion 204 with carboxylic acid moieties are still functional above pH 6.0; strong base anion exchange resins like Amberlite IRA-400 and Indion 454 with quaternary amine ionic sites are used to mask the taste of acidic drugs and they are functional across the full pH range; and tertiary amine substituted weak base anion exchange resins like Amberlite IRC-718 are effective well below pH 7.0.149 Various examples of taste masking by ion exchange resins are given in Table 9.
Table 9 Taste masking of bitter drugs using ion exchange resins
Drug |
Category |
Commercial resin used |
Ref. |
Azithromycin, metronidazole, Quinine sulphate, erdosteine, Paracetamol, |
Macrolide, antiprotozoal, antimalaria, mucolytics, analgesics |
Dowex 2, Amberlite IR 4B |
150
|
Buflomedil |
Vasoactive agent |
Amberlite IRP 69 |
151
|
Clarithromycin |
Macrolide antibiotic |
Carbomer 934 |
144
|
Chlorpheniramine maleate |
Antihistamines |
Indion CRP 254, Indion CRP 244 |
152
|
Clopidogrel sulphate |
Antiplatelet drug |
Water soluble cation exchange resin with sulfonic acid groups |
153
|
Ciprofloxacin |
Fluoroquinolones |
Lewatit CNP, Indion 234 |
154
|
Chloroquine phosphate |
Antimalarial |
Indion cation exchange resin |
155
|
Dextromethorphan hydrobromide |
Antitussive |
Carbomer 934, Amberlite® IRP-69 |
156
|
Donepezil chloride |
Anti-Alzheimer's drugs |
PVP and anionic polymer |
157
|
Erythromycin |
Macrolide antibiotic |
Carbomer 934 |
158
|
Ephedrine hydrochloride |
Sympathomimetic drug |
Indion CRP 244/254 |
148
|
Erythromycin stearate |
Macrolide antibiotic |
Dowex 50, Amberlite IR 120, Indion 244 |
148
|
Orbifloxacin |
Fluoroquinolone antibiotic |
Amberlite IRP 64/69 |
148
|
Paroxetine hydrochloride |
Antidepressant |
Amberlite IRP 88 |
148
|
Remacemide hydrochloride |
Anti-Parkinson's drug |
Amberlite IRP 64 |
148
|
Ranitidine hydrochloride |
Antihistamines |
Amberlite IRP 69/88 |
148
|
Spiramycin, roxithromycin, dimenhydrinate, norfloxacin, ofloxacin, levocetrizine |
Macrolide antibiotic, antihistamine, antibiotic, Antihistamine |
Kyron-T-104, Purolite C 102 DR, Amberlite IRP 50, Doshin P 544(R), Indion 204 |
159
|
Ion exchange resin complexation for taste masking has a number of benefits and drawbacks. One important benefit is that it binds the active pharmaceutical ingredients (APIs) to the resin, preventing them from interacting with taste buds and effectively masking the taste of bitter drugs. This approach is flexible and can be applied to a variety of medications, improving patient adherence, particularly in the case of younger and older patients. Furthermore, controlled release of the API can be achieved with ion exchange resins, enhancing therapeutic efficacy. Nevertheless, there are drawbacks that could affect the medication's overall efficacy, such as the possibility of drug–resin complexes having reduced bioavailability and slower drug release rates. In addition, there may be difficulties with the stability and compatibility of the drug–resin complexes with other formulation elements, as well as a complex and expensive manufacturing process.
3.8. Taste masking by prodrugs, salts or derivatives
Prodrugs are frequently used to mask the taste of bitter substances by decreasing the solubility of the drug in saliva and/or decreasing the affinity of the drug for receptors. The bitter taste commonly associated with opioid analgesics and antagonists is mitigated by employing the buccal distribution technique, which enhances bioavailability compared to oral administration. Notably, several prodrugs of naltrexone, naloxone, nalbuphine, oxymorphone, butorphanol and levallorphan exhibit a lack of bitter taste due to esterification of the 3-phenolic hydroxyl group. This modification renders these compounds more palatable and improves patient compliance. It was concluded that changes in taste were not due to variations in water solubility and that the phenolic functional group is important when interacting with taste receptors. As a result, buccal administration of the synthetic prodrugs of these substances increased bioavailability in comparison with oral administration without the typical bitter taste. Karaman and co-workers developed prodrugs to disguise the unpleasant taste of several medications such as atenolol, pseudoephedrine, dopamine, cephalexin, amoxicillin, and cefaclor. The linker's role in these prodrugs is to prevent the free amine group from forming in the parent compound while maintaining the release of the drug in a controlled manner.160–162
Converting a water-soluble drug into a less water-soluble salt form is another taste-masking technique. The formation of salt makes drugs tasteless and also decreases the solubility of the drug and reduces its solubility in saliva. Chloramphenicol's strong bitterness is a significant drawback when used in paediatric formulations. Chloramphenicol palmitate (CP), a sparingly soluble ester form, is essentially tasteless due to its limited aqueous solubility. Pancreatic lipase readily hydrolyses the type of chloramphenicol palmitate that is used in drugs to form active chloramphenicol.163 Another approach was used for masking the bitterness of propiverine HCl; it was desalinated to create a propiverine-free base, which was then formed into fine granules. The free base fine granules and tablets of propiverine HCl dissolve at about the same rate and have nearly the same bioavailability as the original drug.164
Prodrugs, salts, or derivatives have a number of benefits and drawbacks when it comes to taste masking. Prodrugs are pharmacologically inert derivatives that the body transforms into active medications and these have the potential to greatly lessen unpleasant tastes and improve patient compliance, particularly in the elderly and paediatric populations. Salts have the ability to change a drug's stability and solubility, which may enhance its flavour and palatability in general. Without compromising the medication's ability to work as intended, derivatives can change the chemical structure to reduce bitterness. These approaches do have drawbacks, though, as prodrugs need metabolic conversion, which varies from person to person and may result in uneven drug efficacy. Salt formation may have an impact on how well the medication is absorbed or cause new side effects. Derivative creation may require intricate chemical synthesis, which raises production costs and duration.
3.9. Taste masking by solid dispersion
The technique of distributing or dispersing a drug throughout a dispersion medium is known as solid dispersion. Normally, the medium is molten or a polymer solution. Either the molten mixture can be solidified or the solvent can be evaporated to create the drug-containing granules. There are various techniques for the preparation of solid dispersions. Spray congealing is a technique that is typically used to modify the structure of materials to produce masked pellets with sizes ranging from 0.25 to 2.0 mm and free-flowing powders from liquids. The drug is allowed to melt, dissolve or disperse in a heated melt of additional additives throughout this process. To produce spherical congealed pellets, it is then sprayed into an air chamber where the temperature is below the melting point of the formulation ingredients. Beeswax and water-insoluble polymers like ethylcellulose are generally used for this purpose. Solid bonds created from the congealed melts keep the particles together. Most spray congealing procedures do not involve solvent evaporation; therefore, the particles are typically robust and non-porous, holding together when agitated. The properties of the final spray congealed product depend on the properties of the material used. Poorly soluble drugs can be made more soluble by specific interactions with hydrophilic polymers, and vice versa with reliable interactions between the drug and hydrophobic polymers. Francese and co-workers (1995) reported a drug–polymer matrix composition to achieve the taste masking of dimenhydrinate. Shellac, cellulose acetate phthalate, and zein are examples of copolymers whose esters and carboxylic acid groups may interact physically and chemically with the dimenhydrinate's amine or amido group.165
There are a number of benefits and drawbacks of taste masking by solid dispersions. By encasing drugs in a polymer matrix that keeps the drug from dissolving in the mouth and thereby lessening its taste, solid dispersions can effectively mask the unpleasant taste of drugs. Patient compliance can be increased with this strategy, particularly in the case of younger and older patients. Furthermore, poorly water-soluble drugs’ solubility and bioavailability can be improved by solid dispersion technology. Nevertheless, there are disadvantages as well, like the difficulty of the manufacturing process, possible problems with the dispersion's stability over time, and the potential for insufficient taste masking if the medication is released too soon. Furthermore, choosing the right carriers and processing parameters can be difficult and necessitate thorough formulation.166
3.10. Rheological modification
Decreasing the diffusion of bitter compounds from the saliva to the taste buds can be accomplished by increasing the viscosity with rheological modifiers like carbohydrates or gum. To minimize the strong bitter taste of acetaminophen solution, it can be made with MCC (microcrystalline cellulose) (0.6–1%) and xanthan gum (0.1–0.2%). Phenobarbital and acetaminophen syrup have a bitter taste masked by a polyhydric alcohol like polypropylene glycol or polyethylene glycol with gelatin and polyvinyl pyrrolidone. Mirtazapine, an antidepressant, is manufactured as an aqueous suspension utilizing maltitol as a thickener and methionine as a stabiliser. Maltitol masks the drug's undesirable taste, while inhibiting its unfavourable local anaesthetic action. It is stable in the acidic pH range of 2 to 3. The astringent taste of tannic acid is concealed by the presence of flavouring agents and gelatin by inducing the jelling effect upon cooling.167
3.11. Taste masking by fast-dissolving approaches
Orally disintegrating tablets (ODTs), often called fast-dissolving dosage forms (FDDFs), are a recent and effective technical advancement for masking bitter taste. ODTs are preferred over the conventional tablet forms as they disintegrate and dissolve readily in the saliva without water. ODTs, as new dosage forms, have several distinctive characteristics that set them apart from more conventional ones. ODT formulations’ primary advantage is combining the benefits of both liquid and conventional tablet dosage forms. Children and the elderly can consume ODTs more easily because they do not have to take the medication with water. The development of a suitable ODT requires careful consideration of taste masking. Sweeteners and flavours are currently used as taste masking agents in some fast-dissolving/disintegrating tablet formulations. However, these are insufficient tools for masking the taste of a variety of bitter drugs. The process of compaction is another significant challenge when dealing with coated drug particles. ODT technology's low compaction force is useful for masking the bitter taste of medicine since it reduces the chance of breaking of the barrier coat during compression. Adsorption onto or complexation with carriers and spray coating of drug particles are the two main techniques for masking taste. In addition, ODTs use modern methods including spray drying, freeze-drying, moulding, spinning, sublimation, effervescence and sintering. Certain excipients can also be added like sugars, calcium salt and disintegrants, in addition to compression techniques that are widely used. Below is a brief description of a few of the FDDF technologies.
A FDDF tablet called Zydis® (Cardinal Health) is manufactured by lyophilizing or freeze-drying the drug in a matrix that typically contains flavours, gelatin and sweeteners to improve the dosage forms’ taste. A frozen drug suspension or solution that contains excipients forms structures upon solvent removal. In 2 to 3 seconds, it dissolves on the tongue. Additionally, it includes complexation with ion exchange resins or microencapsulation using specialized polymers to mask the bitterness of the drugs.160 Another fast-disintegrating/dissolving dose form is OraSolv® (Cima Labs). A very slight effervescence helps the OraSolv technology deliver in the saliva. The tablet matrix dissolves in less than a minute, and the tablet matrix dissolves, leaving the coated drug powder behind. The OraSolv formulation uses a dual approach to taste masking; in addition to sweeteners or flavours, this technology also uses drug powder coating and effervescence to disguise the drug unpleasant taste. A second-generation fast-disintegrating/dissolving tablet formulation is called DuraSolv® (Cima Labs). It is manufactured in a process similar to OraSolv®, but higher compaction pressures were used during tableting; it has a substantially higher mechanical strength. Many FDDF formulations are available to mask the taste of bitter drugs, including Ceform™ (Biovail), FlashTab® (Ethypharm), EasyTec™ (Antares Pharma) and Oraquick® (KV Pharmaceuticals).159 Various examples of taste masking by commercially available fast dissolving dosage forms are given in Table 10.
Table 10 A few commercially available fast dissolving dosage forms with taste masking technology
Drug |
Product FDDF |
Indications |
Company name |
Ref. |
Acetaminophen caffeine |
Excedrin® QuickTabs™ |
Pain reliever |
Ethypharm – BMS |
168
|
Acetaminophen |
Tempra® FirsTabs |
Paediatric analgesics |
CIMA – Med Johnson |
169
|
Acetaminophen |
Tylenol® |
Headache |
McNeil PPC |
170
|
Acetaminophen, dextromethorphan, pseudoephedrine |
Triaminic® SoftChews® |
Throat pain and cough |
CIMA – Novartis Consumer Health |
171
|
Diphenhydramine citrate |
Benadryl® Fastmelt™ |
Antihistamine |
Yamanouchi – Pfizer |
— |
Famotidine |
Pepcid RPD® |
Heartburn |
Merck & Co., Inc. |
172
|
Hyoscyamine sulfate |
NuLev™ |
Irritable bowel syndrome |
CIMA – Schwarz Pharma |
173
|
Loratadine |
Dimetapp® |
Antihistamine |
Wyeth |
174
|
Loratadine |
Claritin® RediTabs® |
Antihistamine |
R.P. Scherer – Schering Plough |
175
|
Mirtazapine |
Remeron® SolTabs™ |
Depression |
CIMA – Organon |
176
|
Ondansetron HCl |
Zofran® |
Nausea and vomiting |
R.P. Scherer – Glaxo SmithKline |
177
|
Olanzapine |
Zyprexa® Zydis® |
Schizophrenia |
R.P. Scherer – Eli Lilly |
178
|
Rizatriptan benzoate |
Maxalt® MLT |
Migraine |
R.P. Scherer – Merck |
179
|
Zolmitriptan |
Zomig® ZMT and Rapimelt |
Migraine |
CIMA – Astra Zeneca |
180
|
4. Factors affecting the taste masking approaches
There are various factors affecting the selection for taste masking approaches like the dose of the API (active pharmaceutical ingredient), extent of bitter taste, drug solubility, drug particle size and size distribution, dosage form and ionic properties of the drug.
4.1 Dose of APIs
The dose of a drug may determine the most effective formulation approach for achieving taste masking. In paediatric formulations, the dose is often small enough to permit the use of flavouring additives to mask the taste of the drug. For instance, sweeteners were employed to create a palatable oral formulation of low-dose paediatric aspirin. However, due to the significant quantity of acetaminophen involved, the same strategy proved ineffective in mitigating the taste issue. In such cases, a coating with sweeteners is preferred to ensure taste masking and an appropriate final dosage form size.16
4.2 Drug particle size and size distribution
The efficiency of the taste-masking technique would depend on the properties of the drug particles. Poor taste masking efficiency and uneven dissolution of coated particles are caused by core materials with irregular shapes and small particle sizes. Taste masking through coating can be ineffective due to issues such as abrasions, the formation of dusty particles, and inconsistent coating thickness. Such coating defects can be minimized or completely eliminated by using a multilayer coating with an inner spacing layer that differentiates the drug from a taste-masking layer. Gatifloxacin and dextromethorphan taste-masked granules were manufactured using a layered coating with an inner spacing layer and an outer taste-masking layer.
4.3 Extent of bitter taste
Even a small amount of exposure is enough to detect an awful taste in medicines with an aggressively unpleasant flavour. In one example, due to ibuprofen's dominating taste, sweeteners were unable to mask the taste of its oral formulation. For aggressively bitter drugs, coating is a more efficient approach; even coating defects occur that reduces efficiency of the technique. Similar to this, liquid oral suspensions cannot mask their taste by microencapsulation of extremely bitter APIs like azithromycin. Viscosity modifiers are used to improve taste masking efficiency. Viscosity enhancers in oral suspensions can mask the unpleasant taste arising from drug leakage from coated drugs or microcapsules. The taste masking of bitter drugs like etoricoxib, celecoxib and quinine and of antibiotics like ofloxacin, ciprofloxacin, levofloxacin, cefuroxime axetil, clarithromycin and erythromycin is difficult by using conventional taste masking techniques like sweeteners, amino acids, and flavouring agents.181
4.4 Drug solubility
The physicochemical characteristics of the drugs are crucial when choosing a taste-masking technology. For example, sodium bicarbonate (alkalizing agent) was added to a fast disintegrating ondansetron composition to decrease the water solubility and the resulting gustatory perception because ondansetron has a considerably poor water solubility at higher pH levels. Efficiently masking the bitter taste associated with a poorly soluble form of ranitidine can be achieved through the lipid coating of the drugs. If the product is produced using an aqueous medium, conventional lipid coating of the drug component for ranitidine water-soluble forms (such as ranitidine hydrochloride) may not sufficiently achieve the desired degree of taste masking. In order to effectively mask the taste, ranitidine hydrochloride was first added to the inner core of a polymeric binder, a lipid, or wax that has a higher melting point than the outer coating.148
4.5 Dosage forms
According to estimations, children and about 50% of aged people have trouble swallowing tablets. These issues have been addressed with liquid oral dosage forms and chewable tablets. However, a few drugs are challenging to formulate in these dosage forms due to their unpleasant taste. For formulations meant to be ingested whole without chewing, capsules, coated tablets, and slowly disintegrating hard tablets stand as the favoured taste-masking technologies. Alternatively, taste masking in liquid oral formulations can be achieved through various methods including particle coating, sweeteners, granulation, and microencapsulation. Chewable tablets also benefit from these technologies, complemented by viscosity enhancers and pH modifiers.148
4.6 Ionic properties of the drug
The suitability of drug candidates for ion exchange resin polymers, along with the selection of appropriate polymers, hinges on the ionic properties of the pharmaceuticals. Specifically, cationic polymers are well-suited excipients for anionic drugs such as sildenafil, while anionic polymers are preferable for cationic drugs like donepezil hydrochloride.182
5. Evaluation tests
In the pharmaceutical industry, evaluating taste masking substances is crucial, particularly for orally delivered drugs that could have unpleasant tastes. A flavour masking agent's potency can be evaluated using a variety of tests and methods. Some specific tests and methods frequently employed for assessing taste-masked substances are given in Fig. 4.
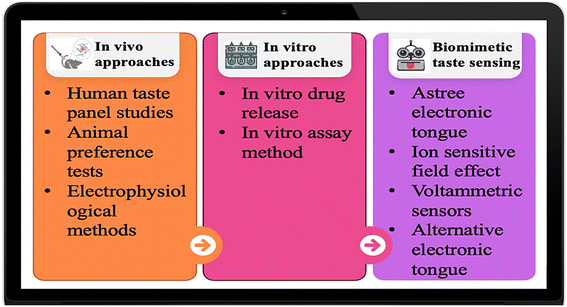 |
| Fig. 4 Types of evaluation tests for the determination of taste masking efficiency.183 | |
5.1 Electronic tongue
When combined with data from human taste assessments, electronic tongue or taste sensors are tools that are trained to quickly screen the flavour qualities of formulations. Application of the electronic tongue requires test chemicals to be sufficiently soluble in water. Co-solvents like ethanol can be employed to make test substances more soluble and to expand the scope of an instrument's application. These devices share a common structure of three main parts: a sensory array, signal-emitting and receiving hardware, and pattern recognition software. Recent years have seen the development of three different types of devices known as electronic tongue, or taste sensors, based on potential, impedance spectroscopy, and voltammetry. Iiyama and co-workers introduced the potential-based gustatory sensors initially. It is made up of several lipid types and polyvinylchloride (PVC) membranes that convert taste quality information, such as salty and bitterness, into electrical signals.184 Another type of prospective taste sensors was described by Vlasov and co-workers, which was built by numerous non-specific sensors based on chalcogenide glasses as transducers.185 The second electronic tongue, which was based on impedance spectroscopy, was initially reported by Riul and co-workers, who used impedance spectroscopy to analyse sensors made of supramolecular thin films of conducting polymers and lipid-like material. Winquist and co-workers created the third type, which was based on the voltammeter idea. It comprises a number of metallic electrodes (Ag/AgCl reference electrode and stainless steel counter electrode, and platinum, gold, palladium, iridium, rhenium, and rhodium) that function as working electrodes. Electronic tongues can analyze and compare the flavour profiles of food products, ensuring consistency in taste. For example, they are used in the beverage industry to maintain the same taste in different batches of wine or coffee. Electronic tongues are used to detect biomarkers in body fluids, such as saliva which can indicate the presence of diseases like diabetes or infections. During the drug development process, electronic tongues help in formulating drugs that are both effective and have a pleasant taste, improving patient compliance.186
Method development and process validation are essential for the effective use of an electronic tongue. Method development involves establishing analytical procedures to ensure that the electronic tongue can accurately and reliably identify and quantify different taste profiles. This includes selecting appropriate sensors, calibrating the device, and optimizing operational parameters. Process validation is crucial to confirm that the electronic tongue consistently produces accurate results under varied conditions, ensuring reproducibility and robustness. This involves rigorous testing and documentation to demonstrate the reliability and accuracy of the system in detecting specific analytes in diverse sample matrices, thereby ensuring that the technology meets regulatory and quality standards.187
5.2. Instrumentation
A sample table, an amplifier, a computer for data storage, various sensor types attached to an arm, and an electronic taste-sensing system are its major components. A simple explanation of the electrochemical taste-sensing mechanism is provided in Fig. 5.
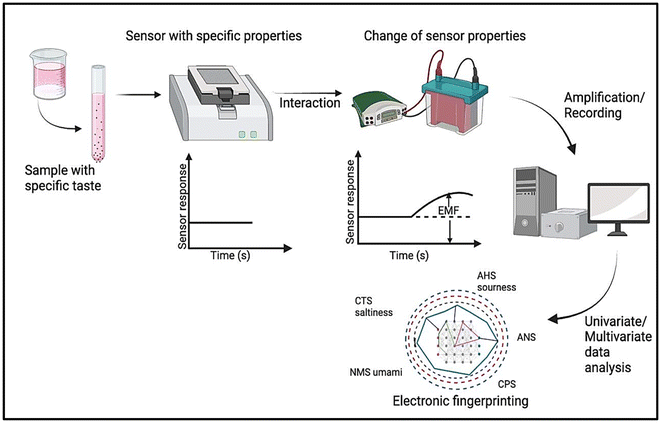 |
| Fig. 5 Schematic representation of an electronic taste conveying system for identifying the degree of taste masking.188 | |
This technology mimics what happens when molecules with particular tastes interact with taste receptors on the tongue. Taste buds are represented by sensors that engage with these chemicals at their surface and cause potential modifications. These signals are compared to action potentials that correspond to brain network activity at the physiological level, which are recorded by computers. The collected data can also be assessed using a matrix of sensor responses that has previously been created and can be compared to human memory or associations with known flavour patterns. Potentiometry is the principle that is used the most. Here, the taste sensing principles of major existing technologies are discussed in more detail.
5.3. Electrochemical concept
The concept of synthesising the human response to external stimuli was first proposed in the year 1943.188 In order to create an “electronic brain” and artificial intelligence based on neural computing, this concept has been expanded. An “electronic nose” (1982) used for gas analysis was the first analytical tool based on these notions.189 A promising tool for both quantitative and qualitative analysis of multi-component matrices is the “electronic tongue,” which was first introduced in 1995.190 According to the literature definition, “the electronic tongue is an analytical instrument comprising of an array of nonspecific, low-selective chemical sensors with high stability and cross-sensitivity to different species in solution, and an appropriate method of pattern recognition (PARC) and/or multivariate calibration for data processing”.191
The application of a variety of nonspecific or low-selective sensors to provide signals during sample analysis is the general idea of the electronic tongue utilised for analysis. The concept behind adopting low-selective sensors is based on an analogy to the biological structure of mammalian taste systems. There are millions of non-specific receptors that respond to various chemicals in the tongue region. On the tongue of mammals, there are many dozens of taste receptors. The brain receives the taste signals from the receptors and processes them via a network of neurons. As a result, the sensed object's image is produced.
6. Regulatory perspective
With the adoption of pediatric regulations in the USA and EU, the demand for age-appropriate medicines for children has significantly increased. The economic impact of poor medication adherence is immense, with inadequate adherence leading to an estimated 125
000 deaths annually in the U.S. alone. According to the European Medical Association (EMA) in 2006, the taste, smell, and texture of medications play crucial roles in their accessibility and acceptability by patients. In 2015, the EMA emphasized concerns regarding the modification of pediatric doses, by incorporating them into soft foods or altering them before administration. They highlighted potential impacts on quality, efficacy, and safety, underscoring the importance of proper dosage administration.192 Regional regulations on taste masking may differ; however, the following general guidelines and considerations are usually followed, i.e., safety and efficacy, formulation, patient compliance and palatability, stability and shelf life, bioavailability, quality control, paediatric use, labelling and packaging, clinical studies, and regulatory approval. Palatability and swallowability are important factors in determining a patient's acceptance of a medication as they impact adherence. One way to describe a drug product's characteristics is its palatability. That improves its flavour, texture, aftertaste, fragrance and acceptability and is an important component in deciding whether patients will accept oral dose formulations. It is possible that swallowability characterized the patient's ability to swallow the medication without choking or gauging. Taste perception develops from a young age and changes as a person ages. Usually, the objective should be drug formulations that most patients would find acceptable and that have a neutral taste. Pharmaceutical companies should work closely with regulatory agencies and consult the latest guidelines to ensure compliance when developing taste-masked drug products. The safety as well as efficacy of the medications are the main concerns of regulatory bodies like the European Medicines Agency (EMA) and the U.S. Food and Drug Administration (FDA).193 When considering drug applications, regulatory bodies may consider the effect of taste masking on patient compliance. The drug's bioavailability should not be adversely affected by taste masking. Regulatory bodies may ask for studies or data to show that the taste-masking formulation does not affect drug absorption or pharmacokinetics. Throughout the drug's shelf life, the taste-masking composition should remain stable. Stability data must be provided to show that the taste masking does not compromise the product's integrity. Validating analytical methods is necessary to confirm taste masking efficacy. Taste-masked drug labelling and packaging provide clear instructions to patients and healthcare providers on administration and dosage. Regulatory bodies frequently require paediatric taste studies to ensure that taste masking is effective and acceptable to the target age group. As part of the drug approval procedure, pharmaceutical companies are required to apply for regulatory approval of taste-masked formulations. A new drug application (NDA) or equivalent regulatory submission is often required for this.
7. Future perspective
The pharmaceutical industry is actively developing new medications for children, promising to introduce vital innovations and close the current gaps in the availability of suitable formulations for young patients. This endeavour is set to enhance the future landscape of paediatric healthcare by addressing the burning issues to improve oral pediatric dosage forms. Optimizing these formulations to be easy to swallow, palatable, and available in suitable dosage units will significantly enhance clinical care for children and benefit many adults as well. A key challenge in this area is the bitter taste of many pediatric medications, which necessitates effective strategies to improve palatability. Current methodologies, such as the use of sweeteners, flavourings, and sophisticated formulation techniques, are being employed to address bitterness. However, future efforts should focus on pioneering new taste-masking technologies and exploring alternative delivery systems like dissolvable films and chewable tablets. Moreover, understanding pediatric dosage form preferences according to specific age and developmental stages, along with validating and cross-validating taste preferences with adult panels, is crucial. Investigating the genetic determinants of taste perception in children can also lead to formulation innovations that prioritize safety, efficacy, and palatability. To achieve these goals, fostering collaborations between researchers and pharmaceutical developers, investing in patient-centred research to comprehensively grasp children's taste preferences, and advocating for regulatory guidelines that emphasize the importance of taste in paediatric medication adherence are essential. Ultimately, enhancing the palatability of paediatric medications will optimize compliance and therapeutic outcomes to grasp the treatment goals for pediatric population.
8. Conclusion
Adherence to medication is significantly affected by how it tastes. Bitter-tasting drugs can be particularly challenging for patients, leading to poor compliance, and even can influence the treatment goal. Improving the acceptability of such bitter tasting medicines by taste masking technology is promising towards improving therapeutic outcomes. Several approaches discussed in this article have been extensively explored on a case basis to enhance the adequacy of such pharmaceutical products; however, there is still a large scope for research in this segment of drug formulation development. The sophisticated approaches described herein are specially beneficial for target patient populations like pediatric and geriatric patients who may have difficulty in swallowing or tolerating unpleasant tastes. For effective taste masking, it is essential to understand the functionality and safety of these materials used for taste masking. Research should focus on how long the taste-masking effect lasts, and ensure that it does not interfere with taste perception for too long. Further investigation should be carried out to invoke guarantee that the taste masking technology or the material used for taste masking does not interfere with the efficacy or bioavailability of the active substance. Continued research and development in this area are necessary to improve the acceptability and effectiveness of medications, particularly for children.
Author contributions
Lohare Rahul Sanjay: writing – original draft and visualization. Makka Krupali Ashokbhai: writing – original draft and visualization. Shubham Ghatole: writing – original draft and visualization. Subhadeep Roy: investigation and review and editing. Kardile Punam Kashinath: investigation and review and editing. Santanu Kaity: conceptualization, writing – review and editing, and supervision.
Data availability
No primary research results, software or codes have been included and no new data were generated or analysed as part of this review.
Conflicts of interest
No conflict of interest to declare.
Acknowledgements
The authors are thankful to the Department of Pharmaceuticals, Ministry of Chemicals and Fertilizers, Government of India for their generous financial support to the institute. The authors are thankful to the National Institute of Pharmaceutical Education and Research (NIPER), Kolkata for providing necessary facilities and support for writing this review. Pictorial representations were drawn using the licensed version of BioRender® software.
References
- D. A. Yarmolinsky, C. S. Zuker and N. J. P. Ryba, Cell, 2009, 139, 234–244 CrossRef CAS PubMed.
- C. M. Mistretta and A. Kumari, Annu. Rev. Physiol., 2017, 79, 335 CrossRef CAS.
- J. D. Fernstrom, S. D. Munger, A. Sclafani, I. E. de Araujo, A. Roberts and S. Molinary, J. Nutr., 2012, 142, 1134S–1141S CrossRef CAS PubMed.
- D. Psimadas, P. Georgoulias, V. Valotassiou and G. Loudos, J. Pharm. Sci., 2012, 101, 2271–2280 CrossRef CAS PubMed.
- T. Simšič, O. Planinšek and A. Baumgartner, Acta Pharm., 2024, 74, 177–199 CrossRef PubMed.
- D. Andrews, S. Salunke, A. Cram, J. Bennett, R. S. Ives, A. W. Basit and C. Tuleu, Eur. J. Pharm. Biopharm., 2021, 158, 35–51 CrossRef CAS PubMed.
- H. Sohi, Y. Sultana and R. K. Khar, Drug Dev. Ind. Pharm., 2004, 30, 429–448 CrossRef CAS PubMed.
- B. Al-kasmi, M. B. Alsirawan, M. Bashimam and H. El-zein, J. Controlled Release, 2017, 260, 134–141 CrossRef CAS PubMed.
- T. Nishiyama, T. Ogata and T. Ozeki, J. Drug Delivery Sci. Technol., 2016, 32, 38–42 CrossRef CAS.
- P. Panraksa, K. Boonsermsukcharoen, K. M. Hwang, E. S. Park and P. Jantrawut, Processes, 2019, 7, 779 CrossRef CAS.
- S. Banerjee, U. Joshi, A. Singh and V. A. Saharan, Chem. Phys. Lipids, 2021, 235, 105031 Search PubMed.
- T. Liu, X. Wan, Z. Luo, C. Liu, P. Quan, D. Cun and L. Fang, Asian J. Pharm. Sci., 2019, 14, 183–192 CrossRef PubMed.
- J. J. Ma, X. Y. Mao, Q. Wang, S. Yang, D. Zhang, S. W. Chen and Y. H. Li, LWT – Food Sci. Technol., 2014, 56, 296–302 CrossRef CAS.
- K. Verma, S. P. Pandey and P. Mishra, Asian J. Pharm., 2018, 12, 1501 Search PubMed.
- E. J. Han, E. Elbegbayar, Y. Baek, J. S. Lee and H. G. Lee, Int. J. Biol. Macromol., 2023, 250, 126259 CrossRef CAS PubMed.
- Z. Ayenew, V. Puri, L. Kumar and A. Bansal, Recent Pat. Drug Delivery Formulation, 2009, 3, 26–39 CrossRef CAS PubMed.
- J. A. Mennella, K. M. Roberts, P. S. Mathew and D. R. Reed, BMC Pediatr., 2015, 15, 1–6 CrossRef PubMed.
- T. A. Gilbertson, Curr. Opin. Neurobiol., 1993, 3, 532–539 CrossRef CAS PubMed.
- E. R. D. C. Neta, S. D. Johanningsmeier and R. F. McFeeters, J. Food Sci., 2007, 72, 33–38 Search PubMed.
- K. F. Medler, Biochim. Biophys. Acta, Mol. Cell Res., 2015, 1853, 2025–2032 CrossRef CAS PubMed.
- A. Taruno, K. Nomura, T. Kusakizako, Z. Ma, O. Nureki and J. K. Foskett, Pflugers Arch., 2021, 473, 3–13 CrossRef CAS PubMed.
- C. S. Li, Y. K. Cho and D. V. Smith, J. Neurophysiol., 2005, 93, 1183–1196 CrossRef PubMed.
- S. Mishra and T. Rai, Food Hydrocolloids, 2006, 20, 557–566 CrossRef CAS.
- K. C. Rani, N. I. E. Jayani, F. Feneke and S. Melanda, IOP Conf. Ser.: Earth Environ. Sci., 2021, 913, 012082 CrossRef.
- K. Priya Dharshini, H. Fang, D. Ramya Devi, J. X. Yang, R. H. Luo, Y. T. Zheng, M. Brzeziński and B. N. Vedha Hari, Carbohydr. Polym., 2021, 256, 117440 CrossRef CAS PubMed.
- Uses of guar gum powder in pharmaceutical formulations, [Online], accessed April 19, 2024.
- S. Lomartire and A. M. M. Gonçalves, Mar. Drugs, 2022, 20, 141 CrossRef CAS PubMed.
- Y. Li, D. Zhang, J. Ash, X. Jia, A. Leone and A. Templeton, J. Pharm. Sci., 2019, 108, 3609–3615 CrossRef CAS PubMed.
- M. C. Gohel, S. A. Nagori, R. K. Parikh, S. N. Shah and M. Dabhi, Indian J. Pharm. Sci., 2009, 71, 120 CrossRef CAS PubMed.
- S. Rajeswari, World J. Pharm. Pharm. Sci., 2017, 6, 472–494 CAS.
- D. Chaimov, L. Baruch, S. Krishtul, I. Meivar-levy, S. Ferber and M. Machluf, J. Controlled Release, 2017, 257, 91–101 CrossRef CAS PubMed.
- B. Karolewicz, Saudi Pharm. J., 2016, 24, 525–536 CrossRef PubMed.
- K. Halake, M. Birajdar, B. S. Kim, H. Bae, C. C. Lee, Y. J. Kim, S. Kim, H. J. Kim, S. Ahn, S. Y. An and J. Lee, J. Ind. Eng. Chem., 2014, 20, 3913–3918 Search PubMed.
- J. Mužíková, Š. Hávová, P. Ondrejček, A. Komersová and V. Lochař, J. Drug Delivery Sci. Technol., 2014, 24, 100–104 Search PubMed.
- G. Thoorens, F. Krier, B. Leclercq, B. Carlin and B. Evrard, Int. J. Pharm., 2014, 473, 64–72 CrossRef CAS PubMed.
- S. Kamel, N. Ali, K. Jahangir, S. M. Shah and A. A. El-Gendy, eXPRESS Polym. Lett., 2008, 2, 758–778 Search PubMed.
- T. L. Rogers and D. Wallick, Drug Dev. Ind. Pharm., 2011, 37, 1259–1271 Search PubMed.
- K. Shiino, Y. Iwao, A. Miyagishima and S. Itai, Int. J. Pharm., 2010, 395, 71–77 Search PubMed.
- H. Lou, M. Liu, W. Qu, Z. Hu, E. Brunson, J. Johnson and H. Almoazen, Drug Dev. Ind. Pharm., 2014, 40, 910–918 Search PubMed.
- Y. D. Yan, J. S. Woo, J. H. Kang, C. S. Yong and H. G. Choi, Biol. Pharm. Bull., 2010, 33, 1364–1370 Search PubMed.
- A. Gryczke, S. Schminke, M. Maniruzzaman, J. Beck and D. Douroumis, Colloids Surf. B Biointerfaces, 2011, 86, 275–284 CrossRef CAS PubMed.
- S. Shah, S. Madan and S. S. Agrawal, Daru, J. Pharm. Sci., 2012, 20, 24 CrossRef CAS PubMed.
- S. A. Randale, C. S. Dabhi, A. R. Tekade, V. S. Belgamwar, S. G. Gattani and S. J. Surana, Chem. Pharm. Bull., 2010, 58, 443–448 CrossRef CAS PubMed.
- S. Khan, P. Kataria, P. Nakhat and P. Yeole, AAPS PharmSciTech, 2007, 8, E127–E133 Search PubMed.
- P. C. Kayumba, N. Huyghebaert, C. Cordella, J. D. Ntawukuliryayo, C. Vervaet and J. P. Remon, Eur. J. Pharm. Biopharm., 2007, 66, 460–465 Search PubMed.
- R. Sheshala, N. Khan and Y. Darwis, Chem. Pharm. Bull., 2011, 59, 920–928 CrossRef CAS PubMed.
- A. A. Barokar, R. D. Wagh, D. T. Baviskar and T. J. Shaikh, Res. J. Pharm. Technol., 2011, 4, 1098–1102 Search PubMed.
- T. Uchida, A. Tanigake, Y. Miyanaga, K. Matsuyama, M. Kunitomo, Y. Kobayashi, H. Ikezaki and A. Taniguchi, J. Pharm. Pharmacol., 2010, 55, 1479–1485 Search PubMed.
- Shishu, Kamalpreet and V. R. Kapoor, Indian J. Pharm. Sci., 2010, 72, 211 Search PubMed.
- D. Bora, P. Borude and K. Bhise, AAPS PharmSciTech, 2008, 9, 1159 Search PubMed.
- K. Malik, G. Arora and I. Singh, Sci. Pharm., 2011, 79, 653 Search PubMed.
- D. A. Chiappetta, Á. M. Carcaboso, C. Bregni, M. Rubio, G. Bramuglia and A. Sosnik, AAPS PharmSciTech, 2009, 10, 1–6 CrossRef CAS PubMed.
- E. J. Yi, J. Y. Kim, Y. S. Rhee, S. H. Kim, H. J. Lee, C. W. Park and E. S. Park, Int. J. Pharm., 2014, 466, 286–295 CrossRef CAS PubMed.
- U. Stange, C. Führling and H. Gieseler, Pharm. Dev. Technol., 2014, 19, 137–147 CrossRef CAS PubMed.
- T. Ishikawa, Y. Watanabe, N. Utoguchi and M. Matsumoto, Chem. Pharm. Bull., 1999, 47, 1451–1454 CrossRef CAS PubMed.
- M. Jańczyk, A. Kutyła, K. Sollohub, H. Wosicka, K. Cal and P. Ciosek, Bioelectrochemistry, 2010, 80, 94–98 CrossRef PubMed.
- M. L. Lorenzo-Lamosa, M. Cuña, J. L. Vila-Jato, D. Torres and M. J. Alonso, J. Microencapsulation, 1997, 14, 607–616 Search PubMed.
- M. Maniruzzaman, M. Bonnefille, A. Aranyos, M. J. Snowden and D. Douroumis, J. Pharm. Pharmacol., 2014, 66, 323–337 CrossRef CAS PubMed.
- X. Zhang, Y. Wang, J. Wang, Y. Wang and S. Li, Chem. Pharm. Bull., 2007, 55, 1261–1263 Search PubMed.
- Y. Gao, F. de Cui, Y. Guan, L. Yang, Y. Sheng Wang and L. N. Zhang, Int. J. Pharm., 2006, 318, 62–69 CrossRef CAS PubMed.
- M. Ueda, Y. Nakamura, H. Makita and Y. Kawashima, J. Microencapsulation, 1993, 10, 461–473 Search PubMed.
- D. D. Douroumis, A. Gryczke and S. Schminke, AAPS PharmSciTech, 2011, 12, 141–151 CrossRef PubMed.
- M. ying, F. Lu, S. Borodkin, L. Woodward, P. Li, C. Diesner, L. Hernandez and M. Vadnere, Pharm. Res., 1991, 8, 706–712 CrossRef PubMed.
- Y. Shahzad, S. Nisar, H. Shah, S. Atique, M. T. Ansari, F. Bashir and T. Hussain, Braz. J. Pharm. Sci., 2011, 47, 323–330 CrossRef CAS.
- M. K. Chun and H. K. Choi, Arch. Pharmacal Res., 2004, 27, 670–675 Search PubMed.
- V. Kumar, T. Yang and Y. Yang, Pharm. Dev. Technol., 2001, 6, 71–81 CrossRef CAS PubMed.
- M. Maniruzzaman, J. S. Boateng, M. Bonnefille, A. Aranyos, J. C. Mitchell and D. Douroumis, Eur. J. Pharm. Biopharm., 2012, 80, 433–442 CrossRef CAS PubMed.
- Y. Hashimoto, M. Tanaka, H. Kishimoto, H. Shiozawa, K. Hasegawa, K. Matsuyama and T. Uchida, J. Pharm. Pharmacol., 2002, 54, 1323–1328 CrossRef CAS PubMed.
- G. S. Rekhi and S. S. Jambhekar, Drug Dev. Ind. Pharm., 1995, 21, 61–77 CrossRef CAS.
- D. W. Seong, J. S. Yeo and S. H. Hwang, J. Ind. Eng. Chem., 2016, 36, 251–254 Search PubMed.
- N. Pearnchob, J. Siepmann and R. Bodmeier, Drug Dev. Ind. Pharm., 2003, 29, 925–938 CrossRef CAS PubMed.
- H. H. Tønnesen and J. Karlsen, Drug Dev. Ind. Pharm., 2002, 28, 621–630 CrossRef PubMed.
-
Y. U. Danny and E. Roche, Taste Masked Pharmaceutical Liquid Formulations, WO0103698A1, 2001 Search PubMed.
- Y. Jin, H. Ohkuma, H. Wang, H. Natsume, K. Sugibayashi and Y. Morimoto, Yakugaku Zasshi, 2002, 122, 989–994 Search PubMed.
- M. Guhmann, M. Preis, F. Gerber, N. Pöllinger, J. Breitkreutz and W. Weitschies, Int. J. Pharm., 2012, 438, 81–90 CrossRef CAS PubMed.
- T. Ishikawa, Y. Watanabe, N. Utoguchi and M. Matsumoto, Chem. Pharm. Bull., 1999, 47, 1451–1454 CrossRef CAS PubMed.
- C. N. Patra, R. Priya, S. Swain, G. Kumar Jena, K. C. Panigrahi and D. Ghose, Futur. J. Pharm. Sci., 2017, 3, 33–45 Search PubMed.
- KR101424653B1, Ph-dependent controlled release pharmaceutical opioid composition with resistance against the influence of ethanol Google Patents. (accessed April 14, 2024).
- CA2079934C, Taste mask coatings for preparing chewable pharmaceutical tablets Google Patents. (accessed April 14, 2024).
- M. Ueda, Y. Nakamura, H. Makita and Y. Kawashima, J. Microencapsulation, 1993, 10, 461–473 CrossRef CAS PubMed.
- EP0298768B1, Flavoured film-coated tablet Google Patents, (accessed April 14, 2024).
- M. Jańczyk, A. Kutyła, K. Sollohub, H. Wosicka, K. Cal and P. Ciosek, Bioelectrochemistry, 2010, 80, 94–98 CrossRef PubMed.
- N. S. Pradnya, B. J. Shital, M. V. Komal and D. D. Ajinkya, World J. Pharm. Res., 2020, 9, 562–597 Search PubMed.
-
L. Lachman, H. A. Lieberman and J. L. Kanig, The Theory and Practice of Industrial Pharmacy, Varghese Pub., House Bombay, Bombay SE, 3rd edn, 1987 Search PubMed.
- E. Bertoni, M. Di Maio, V. Molini and R. Nisticò, J. Dev. Econ., 2019, 141, 102249 CrossRef.
- A. Mills, L. Myers, C. Raudenbush, D. A. Vossen, H. Teppler, Y. R. Miteva, S. Seeley, B. Homony and W. L. Straus, Pediatr. Infect. Dis. J., 2022, 41, 51–56 CrossRef PubMed.
- M. Trofimiuk, K. Wasilewska and K. Winnicka, Int. J. Mol. Sci., 2019, 20, 3200 CrossRef CAS PubMed.
- E. Tsuji, T. Uchida, A. Fukui, R. Fujii and H. Sunada, Chem. Pharm. Bull., 2006, 54, 310–314 CrossRef CAS PubMed.
- A. Fischer, M. Jönsson and P. Hjelmström, Drug Dev. Ind. Pharm., 2015, 41, 79–84 CrossRef CAS PubMed.
- A. R. Menjoge and M. G. Kulkarni, Biomacromolecules, 2007, 8, 532–542 Search PubMed.
- M. Saito, M. Hoshi, A. Igarashi, H. Ogata and K. Edo, Biol. Pharm. Bull., 1999, 22(9), 997–998 CrossRef CAS PubMed.
- M. Nishigaki, K. Kawahara, M. Nawa, M. Futamura, M. Nishimura and K. Matsuura, Int. J. Pharm., 2012, 424, 12–17 CrossRef CAS PubMed.
- K. B. Liew, Y. T. F. Tan and K. K. Peh, Drug Dev. Ind. Pharm., 2015, 41, 583–593 CrossRef CAS PubMed.
- O. Rachid, F. E. R. Simons, M. Rawas-Qalaji and K. J. Simons, AAPS PharmSciTech, 2010, 11, 550–557 CrossRef CAS PubMed.
- S. Klovrzová, L. Zahálka, L. Matysová, P. Horák and Z. Sklubalová, Ceska Slov. Farm., 2013, 62, 35–39 Search PubMed.
- R. Kaartama, E. Turunen, K. Toljamo, H. Kokki, M. Lehtonen and V. P. Ranta, Eur. J. Pharm. Biopharm., 2012, 81, 178–183 CrossRef CAS PubMed.
- U. A. Pandit, P. J. Collier, S. Malviya, T. Voepel-Lewis, D. Wagner and M. J. Siewert, Can J Anesthesia, 2001, 191–195 CrossRef CAS PubMed.
- T. Luckow, V. Sheehan, G. Fitzgerald and C. Delahunty, Appetite, 2006, 47, 315–323 CrossRef CAS PubMed.
- J. P. Ley, G. Krammer, G. Reinders, I. L. Gatfield and H. J. Bertram, J. Agric. Food Chem., 2005, 53, 6061–6066 CrossRef CAS PubMed.
- S. Takagi, K. Toko, K. Wada, H. Yamada and K. Toyoshima, J. Pharm. Sci., 1998, 87, 552–555 CrossRef CAS PubMed.
- H. S. Chung and S. Y. Lee, J. Food Sci., 2012, 77, S202–S210 CrossRef CAS PubMed.
- A. W. Reid and C. H. Becker, J. Am. Pharm. Assoc., Sci. Ed., 1956, 45, 151–152 CrossRef CAS PubMed.
- F. Cilurzo, I. E. Cupone, P. Minghetti, S. Buratti, C. G. M. Gennari and L. Montanari, Drug Dev. Ind. Pharm., 2011, 37, 252–259 CrossRef CAS PubMed.
- M. F. Yanze, C. Duru, M. Jacob, J. M. Bastide and M. Lankeuh, Trop. Med. Int. Health, 2001, 6, 196–201 CrossRef CAS PubMed.
- A. Fini, V. Bergamante, G. C. Ceschel, C. Ronchi and C. A. F. de Moraes, Eur. J. Pharm. Biopharm., 2008, 69, 335–341 CrossRef CAS PubMed.
- R. Sheshala, N. Khan, M. Chitneni and Y. Darwis, Arch. Pharmacal Res., 2011, 34, 1945–1956 CrossRef CAS PubMed.
- V. Z. Ameen, B. F. Pobiner, G. C. Giguere and E. G. Carter, Pediatr. Drugs, 2006, 8, 265–270 CrossRef PubMed.
- S. A. Mostafavi, J. Varshosaz and S. Arabian, Adv. Biomed. Res., 2014, 3, 92 CrossRef PubMed.
- J. Y. Zheng and M. P. Keeney, Int. J. Pharm., 2006, 310, 118–124 CrossRef CAS PubMed.
- T. Sugiura, S. Uchida and N. Namiki, Chem. Pharm. Bull., 2012, 60, 315–319 CrossRef CAS PubMed.
- P. Kranz, N. Braun, N. Schulze and B. Kunz, J. Food Sci., 2010, 75, S308–S311 CrossRef CAS PubMed.
- M. Orlu-Gul, G. Fisco, D. Parmar, H. Gill and C. Tuleu, Drug Dev. Ind. Pharm., 2013, 39, 1028–1036 CrossRef CAS PubMed.
- K. Parker, M. Salas and V. C. Nwosu, Biotechnol. Mol.
Biol. Rev., 2010, 5(5), 71–78 CAS.
-
W. E. Scott and D. G. Mcconnell, U.S. Patent, 1981, 19, 5–8 Search PubMed.
- S. Freitas, H. P. Merkle and B. Gander, J. Controlled Release, 2005, 102, 313–332 CrossRef CAS PubMed.
- S. Thakral, N. K. Thakral and D. K. Majumdar, Expert Opin. Drug Delivery, 2013, 10, 131–149 CrossRef CAS PubMed.
- Atomoxetine HCl methacrylate copolymer fluidised bed – Google search, (accessed April 14, 2024).
- M. Guhmann, M. Preis, F. Gerber, N. Pöllinger, J. Breitkreutz and W. Weitschies, Drug Dev. Ind. Pharm., 2015, 41, 540–551 CrossRef CAS.
- X. Hu, Y. Li, E. Zhang, X. Wang, M. Xing, Q. Wang, J. Lei and H. Huang, AAPS PharmSciTech, 2013, 14, 29–37 CrossRef CAS PubMed.
- W. Brniak, E. Maślak and R. Jachowicz, Eur. J. Pharm. Sci., 2015, 75, 81–90 CrossRef CAS PubMed.
- H. Nakamura, S. Uchida, T. Sugiura and N. Namiki, Int. J. Pharm., 2015, 493, 305–312 CrossRef CAS PubMed.
- Z. Guo, X. Yin, C. Liu, L. Wu, W. Zhu, Q. Shao, P. York, L. Patterson and J. Zhang, Int. J. Pharm., 2016, 499, 47–57 CrossRef CAS PubMed.
- M. Jelvehgari, L. Barghi and F. Barghi, Jundishapur J. Nat. Pharm. Prod., 2014, 9, 39–48 CrossRef PubMed.
- A. M. Rajesh, S. A. Bhatt, G. V. Ramana, H. Brahmbhatt, K. Gajjar, C. B. Tripathi, P. S. Anand and K. M. Popat, Int. J. Pharm. Pharm. Sci., 2014, 6, 352–359 CAS.
- A. K. Tiwari, H. Shah, A. Rajpoot and M. Singhal, J. Chem. Pharm. Res., 2011, 3, 333–341 CAS.
- V. Kharb, V. A. Saharan, K. Dev, H. Jadhav and S. Purohit, Pharm. Dev. Technol., 2014, 19, 839–852 CrossRef CAS PubMed.
- V. Vishvakarma, M. Kaur, M. Nagpal and S. Arora, Curr. Drug Res. Rev., 2023, 15, 1–14 Search PubMed.
- W. L. Tang, W. H. Tang, W. C. Chen, C. Diako, C. F. Ross and S. D. Li, Mol. Pharm., 2017, 14, 1969–1979 CrossRef CAS PubMed.
- Y. Zhu, X. You, K. Huang, F. Raza, X. Lu, Y. Chen, A. Dhinakar, Y. Zhang, Y. Kang and J. Wu,
et al.
, Nanotechnology, 2018, 29, 304001 CrossRef PubMed.
- J. Naik, R. Rajput and M. K. Singh, BioNanoScience, 2021, 11, 21–31 CrossRef.
- K. Krieser, J. Emanuelli, R. M. Daudt, S. Bilatto, J. B. Willig, S. S. Guterres, A. R. Pohlmann, A. Buffon, D. S. Correa and I. C. Külkamp-Guerreiro, Mater. Sci. Eng., C, 2020, 117, 111315 CrossRef CAS PubMed.
- Y. Zhang, L. Shen, T. Wang, H. Li, R. Huang, Z. Zhang, Y. Wang and D. Quan, J. Pharm. Pharmacol., 2020, 72, 776–786 CrossRef CAS PubMed.
- C. Li, K. Zhou, D. Chen, W. Xu, Y. Tao, Y. Pan, K. Meng, M. A. B. Shabbir, Q. Liu and L. Huang,
et al.
, Int. J. Nanomed., 2019, 14, 1619–1631 CrossRef CAS PubMed.
- P. M. Dandagi, S. P. Rath, A. P. Gadad and V. S. Mastiholimath, Indian J. Pharm. Educ. Res., 2014, 48, 93–99 CrossRef.
- A. A. Zardini, M. Mohebbi, R. Farhoosh and S. Bolurian, J. Food Sci. Technol., 2018, 55, 287–298 CrossRef PubMed.
- P. Li, Y. Tian, X. M. Ke, Q. C. Tan, X. Han, H. Y. Ma, J. Pei, J. Z. Lin, R. C. Xu and L. Han,
et al.
, Mol. Pharm., 2020, 17, 1586–1595 CrossRef CAS PubMed.
- R. Huang, Y. Zhang, T. Wang, L. Shen, Z. Zhang, Y. Wang and D. Quan, Asian J. Pharm. Sci., 2018, 13, 343–352 CrossRef PubMed.
- S. M. Omar, F. Ibrahim and A. Ismail, Saudi Pharm. J., 2020, 28, 349–361 CrossRef CAS PubMed.
- J. H. Lee, G. Choi, Y. J. Oh, J. W. Park, Y. B. Choy, M. C. Park, Y. J. Yoon, H. J. Lee, H. C. Chang and J. H. Choy, Int. J. Nanomed., 2012, 7, 1635–1649 CAS.
- J. Szejtli and L. Szente, Eur. J. Pharm. Biopharm., 2005, 61, 115–125 CrossRef CAS PubMed.
- K. B. Lipkowitz, Chem. Rev., 1998, 98, 1829–1873 CrossRef CAS PubMed.
- S. V. Fulzele, S. B. Jaiswal and S. B. Joshi, Indian J. Pharm. Sci., 2001, 63, 45–48 Search PubMed.
-
G. M. Venkatesh, US Pat., US9884014B2, 2018 Search PubMed.
- J. Wadhwa and S. Puri, Int. J. Biopharm. Toxicol. Res., 2011, 1, 3–11 Search PubMed.
-
V. Weiszfeiler and J. Szejtli, in Proceedings of the Fourth International Symposium on Cyclodextrins, 1988, pp. 359–366 Search PubMed.
- Q. Huang, B. Li, S. Yang, P. Ma and Z. Wang, Sci. World J., 2013, 2013, 306476 CrossRef PubMed.
- H. A. Pawar and P. R. Joshi, J. Pharm., 2014, 2014, 789676 Search PubMed.
- D. Nagpal, Int. J. Res. Pharm. Biomed. Sci., 2012, 105–112 Search PubMed.
- S. K. Bajpai, M. Bajpai and S. Saxena, Ion Exch. Solvent Extr., 2007, 18, 103–150 CAS.
- J. Abraham and F. Mathew, Int. J. Pharm. Pharm. Sci., 2014, 6, 12–19 Search PubMed.
-
M. L. Eichman, Drug-resin complexes stabilized by chelating agents, US Pat., 5980882A, 1999 Search PubMed.
- C. P. Yewale, M. N. Rathi, G. G. Kore, G. V. Jadhav and M. P. Wagh, Pharm. Dev. Technol., 2013, 18, 367–376 Search PubMed.
- M. W. Park, S. H. Jeong, S. H. Her, P. J. Kim, J. S. Cho, C. J. Kim, W. S. Chung, K. B. Seung, H. W. Yim and K. Chang, Cardiovasc. Drugs Ther., 2013, 27, 441–449 CrossRef CAS PubMed.
- S. Pisal, R. Zainnuddin, P. Nalawade, K. Mahadik and S. Kadam, AAPS PharmSciTech, 2004, 5, 84 CrossRef PubMed.
- R. Agarwal, R. Mittal and A. Singh, Drug Dev. Ind. Pharm., 2000, 26, 773–776 CrossRef CAS PubMed.
- W. Samprasit, P. Opanasopit, P. Akkaramongkolporn, T. Ngawhirunpat, K. Wongsermsin and S. Panomsuk, Pharm. Dev. Technol., 2012, 17, 315–320 CrossRef CAS PubMed.
- WO2005065645A2 – Donepezil formulations – Google Patents (accessed April 14, 2024).
- V. M. Platon, B. Dragoi and L. Marin, Pharmaceutics, 2022, 14, 2180 CrossRef CAS PubMed.
- G. S. Labib, Drug Des., Dev. Ther., 2015, 9, 5135–5146 CrossRef CAS PubMed.
- H. Hejaz, R. Karaman and M. Khamis, J. Mol. Model., 2012, 18, 103–114 CrossRef CAS PubMed.
- R. Karaman, Chem. Biol. Drug Des., 2011, 78, 853–863 CrossRef CAS PubMed.
- R. Karaman, K. K. Dajani, A. Qtait and M. Khamis, Chem. Biol. Drug Des., 2012, 79, 819–834 CrossRef CAS PubMed.
- H. Andersgaard, P. Finholt, R. Gjermundsen and T. Hoyland, Acta Pharm. Suec., 1974, 11, 239–248 CAS.
- T. Ogata, A. Koide, M. Kinoshita and T. Ozeki, Chem. Pharm. Bull., 2012, 60, 976–984 CrossRef CAS PubMed.
-
F. Francese, M. Maneschi and D. Oldani, WO1995015155A1, 1995.
- S. B. Thoke, A. Gayke, R. Dengale, P. Patil and Y. Sharma, Int. J. Pharm. Sci., 2012, 4, 1895–1907 CAS.
- H. Seager, J. Pharm. Pharmacol., 2011, 50, 375–382 CrossRef PubMed.
- Excedrin quick tab spearmint uses, side effects & warnings, https://www.drugs.com/mtm/excedrin-quick-tab-spearmint.html (accessed April 15, 2024).
- Tempra (Acetaminophen) RxMed: diseases and preparations’ description, https://www.rxmed.com/b.main/b2.pharmaceutical/b2.1.monographs/cps-monographs/cps-(general-monographs-t)/TEMPRA.html (accessed April 15, 2024).
- Headache & Pain Relief TYLENOL®, https://www.tylenol.com/(accessed April 15, 2024).
- Triaminic Softchews oral: uses, side effects, interactions, pictures, warnings & dosing – WebMD, https://www.webmd.com/drugs/2/drug-89162-2096/triaminic-softchews-oral/guaifenesin-pseudoephedrine-oral/details (accessed April 15, 2024).
- Heartburn relief products with active ingredient famotidine | PEPCID®, https://www.pepcid.com/(accessed April 15, 2024).
- NuLev Oral: uses, side effects, interactions, pictures, warnings & dosing - WebMD, https://www.webmd.com/drugs/2/drug-20600/nulev-oral/details (accessed April 15, 2024).
- Great tasting cold relief for kids | Dimetapp®, https://www.dimetapp.com/(accessed April 15, 2024).
- Claritin RediTabs® 24 hour -relieve allergy symptoms, https://www.claritin.com/products/claritin/reditabs-24hr (accessed April 15, 2024).
- Remeron SolTab (Mirtazapine): side effects, uses, dosage, interactions, warnings, https://www.rxlist.com/remeron-soltab-drug.htm (accessed April 15, 2024).
- Zofran uses, dosage & side effects – drugs.com, https://www.drugs.com/zofran.html (accessed April 15, 2024).
- Zyprexa Zydis Oral: uses, side effects, interactions, pictures, warnings & dosing – WebMD, https://www.webmd.com/drugs/2/drug-19876/zyprexa-zydis-oral/details (accessed April 15, 2024).
- Maxalt-MLT Oral: uses, side effects, interactions, pictures, warnings & dosing – WebMD, https://www.webmd.com/drugs/2/drug-8441/maxalt-mlt-oral/details (accessed April 15, 2024).
- Zomig, Zomig Rapimelt (zolmitriptan) dosing, indications, interactions, adverse effects, and more, https://reference.medscape.com/drug/zomig-zmt-zolmitriptan-343036 (accessed April 15, 2024).
- S. Srivastava, A. C. Rana and B. Rajni, Int. Res. J. Pharm., 2012, 3, 92–97 CAS.
- V. Ghadlinge, J. Pharm. Res., 2009, 1049–1054 Search PubMed.
- S. M. Sarika and G. Vilas, Indo Am. J. Pharm. Res., 2014, 4, 204–211 Search PubMed.
- S. Iiyama, S. Ezaki, K. Toko, T. Matsuno and K. Yamafuji, Sens. Actuators, B, 1995, 24, 75–79 CrossRef CAS.
- Y. G. Vlasov, A. V. Legin and A. M. Rudnitskaya, Sens. Actuators, B, 1997, 44, 532–537 CrossRef CAS.
- E. A. Baldwin, J. Bai, A. Plotto and S. Dea, Sensors, 2011, 11, 4744 CrossRef PubMed.
-
L. G. Dias, S. G. Meirinho, A. C. A. Veloso, L. R. Rodrigues and A. M. Peres, Bioinspired Materials for Medical Applications, 2017, pp. 371–402 Search PubMed.
- Y. Vlasov, A. Legin, A. Rudnitskaya, C. Di Natale and A. D'Amico, Pure Appl. Chem., 2005, 77, 1965–1983 CrossRef CAS.
- K. Persaud and G. Dodd, Nature, 1982, 299, 352–355 CrossRef CAS PubMed.
- A. Legin, A. Rudnitskaya, Y. Vlasov, C. Di Natale, E. Mazzone and A. D'Amico, Sens. Actuators, B, 2000, 65, 232–234 CrossRef CAS.
- Y. Vlasov and A. Legin, Fresenius’ J. Anal. Chem., 1998, 361, 255–260 CrossRef CAS.
- Taste Masking 101: optimize taste and improve patient outcomes, American Pharmaceutical Review, https://www.americanpharmaceuticalreview.com/610948-Taste-Masking-101-Optimize-Taste-and-Improve-Patient-Outcomes/(accessed June 5, 2024).
- E11(R1) Addendum: clinical investigation of medicinal products in the pediatric population, FDA Guidance Document, https://www.fda.gov/regulatory-information/search-fda-guidance-documents/e11r1-addendum-clinical-investigation-medicinal-products-pediatric-population (accessed April 18, 2024).
|
This journal is © The Royal Society of Chemistry 2025 |
Click here to see how this site uses Cookies. View our privacy policy here.