Fruit exosomes: a sustainable green cancer therapeutic
Received
26th September 2024
, Accepted 8th November 2024
First published on 20th November 2024
Abstract
Extracellular vesicles, particularly their subpopulation of exosomes, have emerged as a promising avenue for cell-free anti-cancer therapies in the current decade of exosomal research, opening a new chapter in cancer precision medicine. This paradigm shift towards plant-based exosomes holds significant implications for both therapeutic efficacy and sustainability. Plant-derived exosomes offer a non-toxic source for anti-cancer agents, addressing concerns about both patient well-being and the environmental impact of treatment production. This sustainable approach has the potential to make cancer precision therapy more affordable, scalable, and accessible, while simultaneously inspiring scientific minds to explore the vast potential of this “Green Therapy”. This article delves into the potential of fruit-based exosomes in cancer precision therapy, exploring their advantages, challenges, and future perspectives. We discuss the current understanding of fruit exosome biogenesis, leading and isolation techniques, cargo loading mechanisms, their interactions with recipient cellular targets and challenges associated with fruit-based exosomal research. Despite the challenges, the future of fruit-derived exosome-based cancer therapy appears promising. Continued research and interdisciplinary collaborations are crucial to fully unlock the therapeutic potential of fruit-derived exosome-based impactful natural cancer treatment.
 Asmit Das | Asmit Das is a dedicated Research Associate at the Neuron Institute of Applied Research, specializing in exosome research and cancer nano-medicine. He actively contributes to the field as a Member of both the Indian Extracellular Vesicle Society (IEVS) and the Malaysian Extracellular Vesicle Society (MEVS). His research endeavors extend to collaborations with the Center for Global Health Research at Saveetha Medical College and Hospital in Chennai, India. Asmit brings a strong analytical background from his experience in Quality Assurance within the FMCG industry, ensuring rigor and precision in his research. |
 Swarup Sonar | Mr Swarup Sonar is a distinguished member of the Indian Extracellular Vesicles Society (IEVS) and the International Society of Liquid Biopsy (ISLB). Recently, he joined the Malaysian Extracellular Vesicles Society (MEVS), further expanding his professional network. He is involved in research activities with the Center for Global Health Research at Saveetha Medical College and Hospital, Chennai, Tamil Nadu, India. His research interests include exosome-based cancer medicine, biomarkers for early diagnosis and prognosis of cancer, extracellular vesicle-based liquid biopsy, and the targeted application of nano-medicine in cancer research. He has published several articles in these domains. |
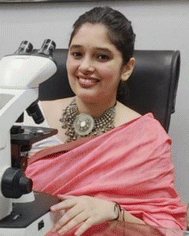 Ketki Kalele | Dr Ketki Kalele is a distinguished oral histopathologist, has dedicated her career to advancing the fields of oral pathology, medical oncology, and cancer research. A dedicated Member of the Indian Extracellular Vesicles Society (IEVS) and an Executive Committee Member of the Malaysian Extracellular Vesicles Society (MEVS), a visionary founder of NIAR, and director of Warkas Research and Innovations Pvt. Ltd, she has also been instrumental in fostering a collaborative environment among healthcare professionals. Her commitment to social welfare and her numerous accolades, including the Women Leadership Award in 2022 and Best Research Paper by the Asia Pacific Dental Association in 2021, underscore her exceptional achievements in the field of medicine. |
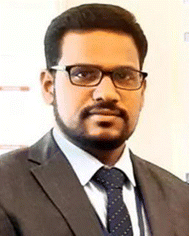 Vetriselvan Subramaniyan | Professor Vetriselvan Subramaniyan is a distinguished academic with over 15 years of experience in higher education. A graduate of Annamalai University, his career spans prestigious institutions globally, including Arba Minch University, MAHSA University, and currently, Sunway University where he holds a professor position. His impactful contributions to the field are evidenced by eight international patents, over 180 publications with a cumulative impact factor exceeding 500, and numerous grants from organizations like the Ministry of Higher Education, Malaysia. Professor Subramaniyan's leadership extends to his role as the Founding President of the Malaysian Extracellular Vesicles Society (http://www.mevsociety.com), a testament to his commitment to advancing this crucial area of research. He also holds memberships in several renowned scientific societies and serves on various editorial boards. |
Sustainability spotlight
Exosomes derived from fruits present a promising avenue for sustainable and environment-friendly cancer treatment and drug delivery. These naturally sourced nanovesicles offer inherent biocompatibility, biodegradability, and low immunogenicity, positioning them as ideal carriers for therapeutic cargo. Notably, fruit-derived exosomes demonstrate significant anti-cancer properties with minimal toxicity compared to their mammalian and synthetic counterparts. Their ability to encapsulate and deliver a variety of anti-cancer agents, including anti-carcinogenic drugs, proteins, and nucleic acids, while selectively targeting cancer cells, underscores their therapeutic potential. This green nanomedicine approach, utilizing fruit-derived exosomes, minimizes the ecological footprint associated with conventional drug delivery systems, paving the way for effective, affordable, and globally accessible cancer treatments.
|
1. Introduction
Cancer remains a significant global health burden, necessitating the development of innovative therapeutic approaches to combat its multifaceted complexities.1,2 Conventional cancer treatments, such as chemotherapy, radiation therapy, and surgical interventions, have inherent limitations, including systemic toxicity, drug resistance, and a lack of tumor-targeted specificity.3–7 The rapidly evolving field of nanomedicine, particularly the use of extracellular vesicles, including exosomes, for precise drug delivery, offers a new era for personalized treatment of chronic diseases, especially cancer.4,8–10 Mammalian-derived exosomes, while promising, face clinical translation hurdles due to potential immune rejection, toxicity risks, and production challenges. Fruit-derived exosomes offer a safer alternative, leveraging structural and functional similarities while mitigating significant risks.11–13 Plant and fruit extracts have long been investigated for their potential anticancer properties, with numerous studies highlighting the ability of their bioactive compounds to inhibit tumor growth and progression.14–16 However, harnessing these beneficial effects for clinical applications has proven challenging.15 Fruit-derived exosomes, derived from abundant, edible sources, ensure scalability and biocompatibility. These exosomes represent a novel strategy for utilizing the anticancer properties of these phytochemicals. These nanovesicles offer a naturally engineered platform for encapsulating and delivering concentrated doses of these bioactive compounds (phytochemicals) to targeted tumor sites, enhancing their bioavailability and therapeutic efficacy.11,17,18 Fruit-derived exosomes simultaneously demonstrate a remarkable ability to encapsulate and deliver a variety of therapeutic cargoes, including chemotherapeutic drugs, small interfering RNAs, and microRNAs, directly to tumor sites. This targeted delivery of therapeutics using fruit-derived exosomes enhances drug efficacy by selectively transporting the cargo to tumor cells, while minimizing off-target effects on healthy tissues and associated adverse reactions. The inherent biocompatibility and tumor-homing capabilities of these naturally-derived nanoparticles allow for safer and more precise drug delivery, addressing a key limitation of conventional cancer treatments. Furthermore, research indicates that fruit-derived exosomes can modulate the tumor microenvironment, influencing the complex interplay between cancer cells and their surroundings to inhibit tumor growth and progression. Fruit-derived exosomes can also be engineered to enhance their targeting capabilities, drug-loading efficiency, and anti-tumor properties.19,20 They show immense potential for improving the clinical translatability of plant-based cancer therapies and improving cancer treatment outcomes.18,21 Despite the promising results, further research is needed to fully understand the mechanisms of action, optimize the production and purification methods, and ensure the safety and efficacy of fruit-derived exosomes in clinical settings. Ongoing clinical trials and regulatory developments will also play a crucial role in the translation of these novel therapeutics from the bench to the bedside (Fig. 1).
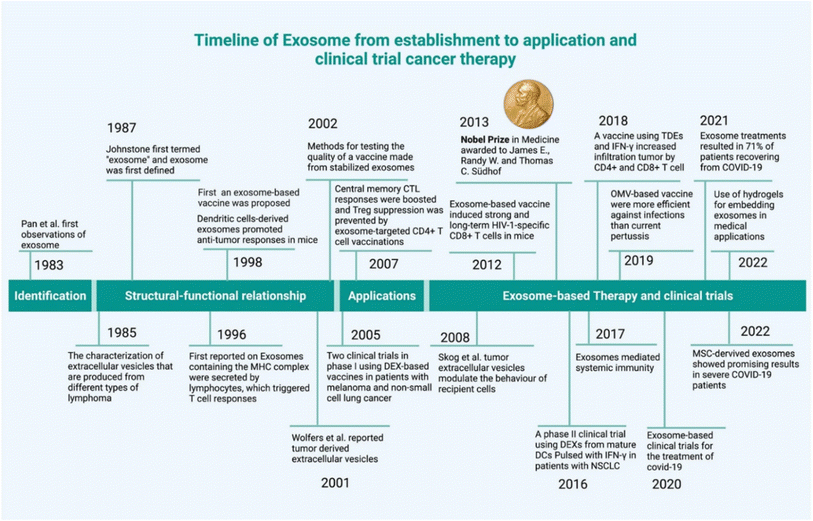 |
| Fig. 1 Exosome-based cancer therapeutic developmental landscape (reproduced with permission under Creative Commons CC BY 4.0 license from ref. 22. Copyright @ 2022 The Authors). | |
This review explores the burgeoning field of fruit-derived exosomes as a novel, green, and sustainable approach to cancer therapy. Fruit exosomes, naturally occurring nanovesicles, offer a unique convergence of plant-derived exosome advantages and potential edible benefits as functional foods. This dual functionality positions them as a revolutionary tool for cancer management, providing both accessible targeted therapeutic intervention and nutritional support. Their biocompatibility, inherent targeting ability, and capacity to encapsulate diverse anticancer payloads highlight their potential to enhance treatment efficacy while minimizing off-targets and systemic toxicity. Their unique ability to modulate the tumor microenvironment further positions them as a promising alternative to conventional therapies. Although the potential for edible incorporation into diets exists, further research is essential to standardize extraction and characterization methods, ensuring consistent therapeutic efficacy and safety.
2. Fruit exosome biogenesis
Plants employ various pathways for exosome biogenesis (Fig. 2), including those resembling the exosomal pathway in mammals23–25 (involving endosomal compartments), the Exocyst-Positive Organelle (EXPO) pathway, and a pathway involving the plant vacuole. Each pathway, with its unique mechanisms, contributes to the secretion of bioactive molecules essential for intercellular communication and various physiological processes.23,24,26 The EXPO pathway offers a distinct route for secreting exosomes, particularly in plants.27 Originating from the ER and Golgi, specialized compartments bud off, aided by the exocyst complex and small GTPases (Rab and Ral proteins). These EXPO compartments, enriched with various biomolecules (proteins, lipids, and nucleic acids), travel along the actin cytoskeleton to the plasma membrane. Fusion with the membrane, facilitated by SNARE proteins, releases the nanoparticles into the extracellular space. This unique pathway is crucial for secreting cell wall components and bioactive molecules within the exosomes, and enables intercellular communication, influencing various physiological processes in plants.26–28 The multivesicular body (MVB) pathway, a conserved mechanism across eukaryotes, generates exosome-like nanoparticles through a series of steps within endosomes.29–31 Initially, intraluminal vesicles bud inward from the endosomal membrane, a process orchestrated by the endosomal sorting complex required for transport. This complex recognizes and incorporates ubiquitinated cargo proteins into the forming ILVs. Lipids, particularly phosphatidylinositol 3-phosphate (PI3P), play a crucial role by recruiting endosomal sorting complex required for transport (ESCRT) components to the endosomal membrane.32–34 Intraluminal vesicle (ILV)-containing endosomes mature into multivesicular bodies, which can fuse with the plasma membrane, a process regulated by Rab GTPases, to release ILVs as exosomes. These nanovesicles, rich in bioactive molecules, play crucial roles in intercellular communication and plant defence responses.29,30,35
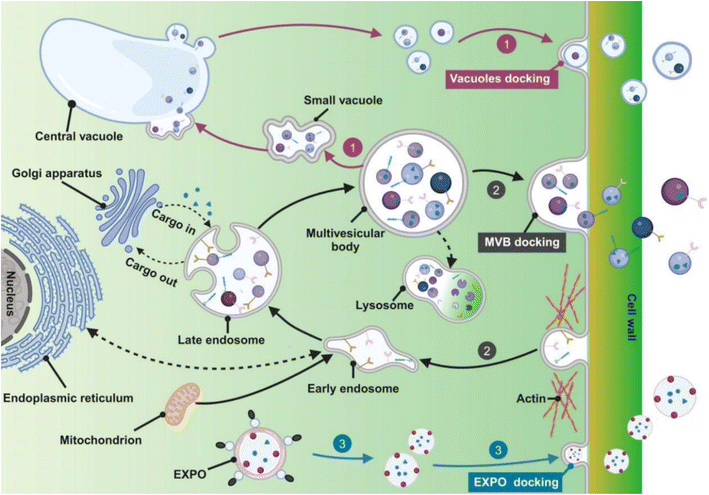 |
| Fig. 2 Fruit exosome biogenesis (1) vacuolar pathway; (2) MVB pathway; (3) EXPO pathway (reproduced with permission under Creative Commons CC BY 4.0 license from ref. 36. Copyright @ 2024 The Authors). | |
Additionally, the vacuolar pathway, another route for fruit exosome biogenesis, involves the budding of vesicles from the vacuolar membrane, a process potentially involving ESCRT machinery and lipids (PI3P and PI(3,5)P2).26,28,29,37 While less understood than other pathways, it plays a crucial role in protein storage, nutrient recycling, and plant defence (using antimicrobial proteins). The fusion of vacuoles with the plasma membrane is regulated by small GTPases (like Rab and Ral proteins) and tethering/fusion factors (including the exocyst complex and SNARE proteins), facilitating exosome release into the extracellular space.28,30,35 While these pathways share some similarities with the biogenesis of mammalian-derived exosomes, they also exhibit unique characteristics due to their plant origin. However, further research is needed to fully elucidate its regulatory mechanisms and biotechnological potential. Differentiation between fruit-derived exosomes and mammalian cell-derived exosomes is presented in Table 1.
Table 1 Comparison of fruit-derived exosomes and mammalian cell-derived exosomes
Characteristics |
Fruit-derived exosomes |
Mammalian cell-derived exosomes |
Reference |
Origin |
Fruits, plant origin |
Mammalian cells (e.g., mesenchymal stem cells, dendritic cells, etc.) |
25 and 36 |
Size (nm) |
30–800 nm |
30–150 nm |
36 and 38–40 |
Zeta potential (mV) |
−70 to approximately neutral |
−34.3 to −6.3 |
18, 40 and 41 |
Cargo |
- Proteins |
- Proteins (highly abundant) |
25, 36 and 42 |
- Nucleic acids |
- Nucleic acids |
- Secondary metabolites (unique to plants) |
- Lipids |
Protein content |
Typically lower protein concentration; proteins are mainly cytosolic with few membrane proteins present |
Higher protein diversity, including membrane proteins and signaling molecules |
18, 25, 40, 43 and 44 |
miRNA content |
Contains various miRNAs, but the diversity and functional roles may be limited compared to mammalian exosomes |
Rich in diverse miRNAs that play significant roles in intercellular communication and regulation |
36, 40, 42 and 43 |
Lipid composition |
Lipid profiles are simpler, primarily consisting of phospholipids and some bioactive lipids; may lack certain lipid species found in mammalian exosomes |
Complex lipid composition, including sphingolipids and cholesterol, which are crucial for membrane stability and function |
25, 36, 43 and 44 |
Immunogenicity |
Lower risk of immunogenic response due to lack of mammalian proteins |
Potential for immunogenic response due to the presence of foreign proteins |
25, 36, 42 and 44 |
Scalability |
Potentially easier and more cost-effective to isolate from abundant plant sources |
Can be challenging to isolate large quantities from mammalian cells |
25, 36, 43 and 44 |
Means of internalization |
Clathrin/caveolae-mediated endocytosis, macropinocytosis, and phagocytosis |
Phagocytosis and endocytosis |
40, 42 and 44 |
Common route of administration |
Oral administration |
Intravenous administration |
25, 36, 40 and 44 |
3. Isolation and characterization fruit exosomes
The isolation and characterization of fruit exosomes necessitate rigorous and meticulous methodologies to fully elucidate and harness their therapeutic capabilities. Involving a delicate balance of separating these nanoscale vesicles from the fruit matrix without compromising their structural integrity or biological activity. Various methods exist for isolating these exosomes, all of which rely on leveraging their distinct physical characteristics.45–47 Differential ultracentrifugation remains a cornerstone technique for exosome isolation. This method exploits the principle of sedimentation, subjecting samples to sequential centrifugation steps at escalating gravitational forces (g). By targeting specific sedimentation coefficients, exosomes are effectively pelleted and separated from a heterogeneous mixture of larger vesicles and cellular debris.47,48 Density gradient centrifugation improves purity by separating exosomes based on their buoyant density (1.13–1.18 g mL−1) within a sucrose or iodixanol gradient.49 Tangential flow filtration (TFF) is a scalable technique designed for processing large volumes, utilizing membranes with defined pore sizes (typically 50–100 nm) to selectively retain exosomes while allowing smaller molecules to pass through.50,51 Ultrafiltration operates on a similar principle but employs centrifugal force to drive samples through the membrane, making it particularly suitable for smaller volumes.46,52 Precipitation-based methods, frequently available as commercial kits, take advantage of the altered solubility of exosomes in the presence of water-excluding polymers like polyethylene glycol (PEG), resulting in their precipitation.46,53 Immunoaffinity capture provides high specificity by utilizing antibodies immobilized on beads or surfaces to selectively bind exosomes that express specific surface markers.54,55 Microfluidic isolation employs miniaturized devices that integrate various separation mechanisms such as filtration, affinity capture, and acoustic trapping for high-throughput and automated isolation.52,56,57 Acoustic fluid handling manipulates exosomes based on their acoustic properties, using sound waves to focus and separate them.58 Magnetophoresis involves magnetic beads coated with antibodies against exosomal markers, facilitating efficient capture and release of exosomes through magnetic fields.59 Deterministic lateral displacement (DLD) exploits the size and deformability of exosomes, separating them based on their trajectory in microfluidic channels with asymmetrically arranged obstacles.60,61 Lastly, field-flow fractionation separates particles in a thin channel under external fields—such as flow or electric fields—based on their differential migration.62–65 Characterizing exosomes involves a variety of techniques aimed at elucidating their biophysical and biochemical properties.66 One widely used method is nanoparticle tracking analysis (NTA), which visualizes and monitors the Brownian motion of individual exosomes, utilizing light scattering to determine their size distribution and concentration.67,68 Dynamic light scattering (DLS) also employs light scattering but focuses on measuring the fluctuations in scattered intensity caused by Brownian motion, providing insights into hydrodynamic size and polydispersity.65,69 Electron microscopy, including both transmission and scanning electron microscopy, offers high-resolution images that reveal the morphology, size, and structural characteristics of exosomes.66 Atomic force microscopy (AFM) provides nanoscale topographical data by scanning a sharp tip across the exosome surface, allowing for detailed examination of size, shape, and surface features.66,70 Western blotting remains a fundamental technique for detecting specific proteins within exosome lysates, thereby confirming the presence of known exosomal markers and target proteins.48,71 Flow cytometry can analyze individual exosomes for size, granularity, and expression of surface markers using fluorescently labeled antibodies; however, this method faces challenges due to the small size of exosomes.53,72 Enzyme-linked immunosorbent assays (ELISA) quantify specific proteins or other molecules in exosome samples through antibody-based detection, offering high sensitivity and specificity.47,66 Raman spectroscopy analyzes the inelastic scattering of light from molecules within exosomes to provide information about their biochemical composition and structure.73 Mass spectrometry serves to identify and quantify proteins, lipids, and metabolites in exosome samples, yielding a comprehensive molecular profile.62,74,75 RNA sequencing examines the RNA content within exosomes, revealing mRNA, miRNA, and other RNA species that provide insights into their functional roles and origins.66 Lipidomics focuses on characterizing the lipid composition of exosomes to enhance understanding of their membrane structure and function.74,76 Lastly, the Integrated Magnetic-Electrochemical Exosome (iMEX) sensor is a rapid and sensitive approach for quantifying exosome surface markers, demonstrating its effectiveness in detecting varying numbers of extracellular vesicles spiked into human plasma.66 In the exosome isolation and profiling process, the combination of nanotechnology and nanomaterials promotes it as a next-generation promising precision medicine development platform.77–80
4. Exosomes and cancer
The tumor microenvironment (TME) consists of a complex network of cellular and non-cellular components that facilitate cancer progression and metastasis.81–83 A defining feature of the TME is the hypoxic condition, characterized by inadequate blood supply and oxygen availability, which enables cancer cells to activate survival pathways mediated by hypoxia-inducible factors (HIFs).8,84–87 This adaptability allows malignant cells to proliferate uncontrollably and divert essential resources for growth.87–89 Consequently, cancer cells disrupt the homeostasis of surrounding normal tissues, creating a microenvironment conducive to malignant transformation and metastasis.84,90 Hypoxia also stimulates the secretion of exosomes from tumor cells, which are nanoscale vesicles that play crucial roles in intercellular communication within the TME.66,91 Tumor-derived exosomes (TEXs) facilitate angiogenesis by transferring pro-angiogenic factors and microRNAs to endothelial cells, enhancing their proliferation and migration.81,92,93 For example, exosomes from hypoxic tumor cells contain high levels of miR-210, promoting angiogenesis by targeting endothelial cell function genes.94,95 TEXs significantly influence immune cell behavior, contributing to immune evasion by shifting the immune response toward an immunosuppressive state.96–98 They can reprogram macrophages from an anti-tumorigenic M1 phenotype to a pro-tumorigenic M2 phenotype,99,100 with exosomal miR-934 facilitating this shift and enhancing metastasis.100 Additionally, TEXs promote the differentiation of monocytes into myeloid-derived suppressor cells (MDSCs), further inhibiting T-cell responses through signaling molecules like prostaglandin E2 (PGE2) and transforming growth factor beta (TGF-β).101 Moreover, TEXs suppress T cell activity via cargo such as TGF-β and programmed death-ligand 1 (PD-L1), which inhibit T cell activation and promote regulatory T cell differentiation.102 Dendritic cells can also be converted into tolerogenic cells by TEXs, diminishing their ability to activate T cells effectively.102 This interplay underscores how tumors evade immune surveillance and promote growth. Exosomes are also implicated in metastasis through processes like the epithelial–mesenchymal transition (EMT), where epithelial cells acquire migratory properties. TEXs transfer bioactive molecules that facilitate the EMT and enhance metastatic potential.103 For instance, exosomal microRNA-106b-5p has been linked to colorectal cancer metastasis by influencing interactions with M2 macrophages.104 Furthermore, exosomal surface proteins such as tetraspanins and integrins play critical roles in organ-specific metastasis by mediating interactions between circulating tumor cells and target microenvironments.105,106 Exosomes also contribute to therapeutic resistance by transferring drug resistance genes and proteins. For example, exosomes from cisplatin-resistant lung cancer can transfer miRNA-100-5p, altering mTOR signaling to enhance survival during chemotherapy.107 In breast cancer, exosomes can sequester human epidermal growth factor receptor 2 (HER2) targeted drugs or reprogram gene expression in recipient cells to reduce treatment susceptibility.108 In summary, exosomes act as both facilitators of cancer progression and potential targets for innovative therapeutic strategies. Their multifaceted roles in the TME highlight their significance in understanding cancer biology and developing new treatments. In the TME, TEX-mediated cancer promoting signature is described in Fig. 3.
 |
| Fig. 3 Role of exosomes in cancer progression. (Reproduced with permission under Creative Commons CC BY 4.0 license from ref. 109 copyright @ 2019 Springer publisher.) | |
5. Therapeutics application of fruit-derived exosomes
Exosome-based cancer therapeutic application presents a more advanced approach compared to traditional cell-based therapies.110 Fruit-derived exosomes are gaining traction as potential candidates for precision cancer therapies (Fig. 4).111–113 This section will explore various anti-cancer therapeutic approaches utilizing these exosomes, examining their sources, mechanisms of action, therapeutic payloads, targeted therapies, efficacy in specific cancer types, and relevant research. Lemon-derived exosomes exhibit anti-cancer properties primarily through the induction of apoptosis in cancer cells, activating the TRAIL pathway to promote cell death in various cancer types.114,115 These exosomes contain small RNAs, including microRNAs, and show promise as natural anti-cancer agents against triple-negative breast cancer by inhibiting cell proliferation, migration, and phosphatidylinositol 3-kinase/protein kinase B (PI3K/AKT) and mitogen-activated protein kinase/extracellular signal-regulated kinase (MAPK/ERK) signaling pathways.116 They also target various cancer cell lines, including SW480 (human colorectal adenocarcinoma cell line), LAMA84 (human chronic myeloid leukemia cell line) and A549 (human lung carcinoma cell line) by downregulating anti-apoptotic proteins and upregulating pro-apoptotic factors. Studies have shown that lemon-derived exosomes inhibit proliferation in colorectal cancer cells in vitro and suppress tumour growth in xenograft models of chronic myeloid leukemia.40,114–116 Strawberry-derived exosome-like nanoparticles exhibit anti-cancer properties by preventing oxidative stress-induced damage in human mesenchymal stromal cells, modulating cellular differentiation, and promoting apoptosis in tumor cells through the delivery of bioactive compounds such as vitamin C, anthocyanins, and small RNAs.40,117 These exosomes may primarily target breast cancer cells, where they exert protective effects against oxidative stress and induce apoptosis, as indicated by research showing that they significantly reduce oxidative stress levels in cancer cell lines.117–119 Grapefruit-derived exosomes exhibit anti-cancer properties by inducing cell cycle arrest and apoptosis in cancer cells through the modulation of signaling pathways such as PI3K/AKT. These nanovesicles exhibit anti-tumor activity due to their rich cargo, which includes polyphenols, flavonoids, various miRNAs, and an enrichment of specific metabolites like alpha hydroxy acids (glycolic and citric acids), the amino acids (leucine and isoleucine), and the fatty acids (palmitic acid and doconexent).40,120 They primarily target breast, lung and melanoma cells and impair the proliferation of cancer cells, inhibiting metastasis and promoting apoptosis, as demonstrated by significant anti-cancer effects in vitro and reduced tumor sizes in animal models.114,120 Treatment with apple-derived exosomes not only effectively suppressed tumor growth by increasing the levels of miR-30a-5p, which in turn regulates cell cycle progression, but also proved to be completely safe. This anti-tumor effect was linked to the regulation of specific proteins like E2F transcription factor 7 (E2F7) in gallbladder/esophageal cancer and Glucose-Regulated Protein 78 (GRP78) in renal carcinoma. Additionally, these exosomes demonstrated anti-inflammatory properties by boosting the production of miRNA-146a in M2 macrophages.121–123 Bitter melon-derived exosomes (BMEV) demonstrate both anti-tumor and anti-inflammatory properties against oral squamous cell carcinoma (OSCC). These exosomes trigger cell death through a mechanism involving ROS production and JUN protein upregulation, while surprisingly downregulating the inflammatory nucleotide-binding domain and leucine-rich repeat protein 3 (NLRP3) pathway. This NLRP3 downregulation, potentially mediated by BMEV associated RNAs, enhances the sensitivity of OSCC cells to the chemotherapy drug 5-fluorouracil (5-FU), suggesting a promising synergistic therapeutic strategy.124,125 Lemon exosome-based anti-cancer therapeutic activity is very effective.126
 |
| Fig. 4 Anti-cancer activity of plant-derived EVs (reproduced with permission under Creative Commons CC BY 4.0 license from ref. 18 copyright @ 2022 The Authors). TRAIL-tumor necrosis factor-related apoptosis-inducing ligand, PDVs-plant derived extracellular vesicles, DR5-death receptor 5 is activated when it binds to TRAIL, which initiates apoptosis. | |
Grape-derived exosomes show great promise as oral drug delivery vehicles due to their biocompatibility, biodegradability, and remarkable stability in challenging environments such as the gastrointestinal tract. These nanovesicles, with an average size of 205.1 nm, significantly boosted leucine-rich repeat-containing G-protein-coupled receptor 5 (Lgr5) gene expression in rats, suggesting a potential role in promoting intestinal stem cell activity and regeneration. Since Lgr5 is a marker of intestinal stem cells, implicated in colon cancer development, grape-derived exosomes may offer a novel therapeutic strategy for targeting this disease.125,127 Though still in their infancy, fruit-derived exosomes offer a promising new avenue for cancer precision therapies, potentially providing a biologically safer and more effective treatment approach to combat this devastating disease and improve patient outcomes Table 2.
Table 2 Fruit-derived exosomes-applications in drug delivery
Fruit exosome source |
Therapeutic carried |
Target |
Reference |
Grape |
Fisetin |
MOLT-4 cell |
128
|
Grapefruit |
Doxorubicin, heparin nanoparticles |
Glioma |
129
|
HSP70, variants of BSA |
Colon cancer cells |
130
|
Doxorubicin–(si)RNA co-delivery |
MDR LoVo colon cancer cells |
131
|
HSP70 |
Glioma cells |
44
|
miRNA17 |
Brain tumor |
115
|
Doxorubicin |
Breast cancer |
132
|
Apple, orange, pomegranate |
ath-miR159a |
Caco-2 cells |
133
|
ath-miR162a-3p |
ath-miR166b-3p |
ath-miR396b-5p |
Watermelon |
Therapeutic miRNA mimic (hsa-miR146a-5p) |
Ovarian cancer cells (ID8, A2780, and OVCAR8 models) |
134
|
Bitter melon |
5-Fluorouracil |
Oral squamous cell carcinoma |
124
|
Lemon |
Doxorubicin |
Ovarian cancer |
126
|
6. Toxicity of fruit-derived exosomes
Fruit-derived exosomes have exhibited a promising safety profile, supporting their potential for clinical translation. The inherent absence of zoonotic or human pathogens in fruit-derived exosomes contributes to their favourable biocompatibility, as evidenced by in vitro and in vivo studies across various administration routes.36 Oral administration of fruit-derived exosomes, even at relatively high doses (e.g., grapefruit-derived exosomes at 10 mg kg−1 in mice for 7 days), has not been associated with adverse effects on systemic inflammatory markers like serum interferon-gamma (IFN-γ) levels, liver function (liver enzymes), or hepatocellular injury (AST/ALT levels).36,38 Similarly, other routes of administration, including intraperitoneal36,135 and intranasal,36,136 have not raised significant safety concerns. Intravenous administration of fruit-derived exosomes, particularly those derived from grapefruit, and lemon,36,126 has generally been well-tolerated in preclinical models. However, given the inherent diversity in composition and biophysical properties of fruit-derived exosomes from different fruit sources, comprehensive safety evaluations remain indispensable before considering their intravenous use in clinical settings.
7. Clinical trials
Exosomes are gaining notable recognition and global research interest due to their promising therapeutic potential for various diseases including cancer, to address significant clinical challenges precisely and effectively. Numerous pre-clinical trials and laboratory investigations have explored the potential of exosomes, especially those derived from various human cells and plants (including various fruits, vegetables, and other plant parts), as a therapeutic tool for cancer treatment.137,138 Meanwhile, exosome-mediated drug delivery is emerging as a cutting-edge approach in clinical trials, holding tremendous potential for tackling cancer and other global health concerns.139 Clinical trials are crucial for translational medicines in modern oncology practices. In recent times, those have been investigated in the clinical trial stage to examine their potential, efficacy, side effects, and limitations further.140 In the current decade, scientists around the world are studying plant-derived exosomes, particularly those from fruits, as candidates for clinical trials due to their low toxicity, high biocompatibility, and the effectiveness of their molecular cargos especially phytochemicals.38,141 A recent clinical study (clinical trial ID: NCT01668849) investigated the anti-inflammatory properties of grape-derived exosomes, administered in grape powder form, to determine their effectiveness in reducing oral mucositis in head and neck cancer patients undergoing chemo-radiation therapy, including their impact on cytokine production, immune responses to tumor exosomal antigens, and associated metabolic and molecular markers (https://clinicaltrials.gov/). Moreover, exosomes show remarkable advancements in medicine and cancer research, with substantial improvements made in recent years. The clinical journey of exosomes is not without obstacles. Plant-derived exosomes, including those from fruits, hold therapeutic promise, but their clinical application faces significant hurdles. Notably, the translation of exosome-based clinical trials must conform to rigorous good manufacturing practices (GMP), addressing the numerous challenges that arise.142,143 As research continues to uncover the benefits of exosome-based therapies, future research efforts should prioritize conducting comprehensive clinical trials to explore the therapeutic efficacy and drug delivery potency of exosomes, with a specific focus on plant and fruit-derived sources, which are currently underrepresented in clinical investigations.
8. Future perspectives and challenges
Fruit-derived exosomes show great promise in targeted cancer treatment because they are naturally compatible with the body, unlikely to trigger an immune response, and capable of delivering various therapeutic molecules like miRNAs, proteins, and bioactive molecules. Their therapeutic potential is evident in their anti-tumor effects, due to their unique biomolecular cargoes.12,115,144 Fruit-derived exosomes offer a renewable and abundant source for large-scale production, addressing a key limitation associated with mammalian exosomes.12,145,146 A major hurdle is the lack of standardized definitions, nomenclature, and practices for characterizing and classifying these plant-derived vesicles.36,147 Establishing a robust framework with rigorous physicochemical characterization and well-defined biological pathways is crucial for advancing exosome research. Moreover, fruit-derived exosomes with their inherent heterogeneity,148 further compounded by the limitations of current isolation techniques, pose a significant hurdle.11,147 Optimizing extraction protocols to enhance fruit-derived exosome yield while maintaining purity and structural integrity is crucial for advancing preclinical and facilitating clinical translation. A deeper understanding of the molecular mechanisms underlying the anti-tumor effects of fruit-derived exosomes is also crucial.12,115 Identifying the specific miRNAs, proteins, and small molecules responsible for these effects, as well as their target pathways in cancer cells, will be essential for developing more targeted and effective therapies. Exploring potential synergistic interactions between different fruit-derived exosomal components may also uncover novel therapeutic strategies.115,149,150 To facilitate clinical translation, extensive in vivo studies are necessary to evaluate the safety, biodistribution, and therapeutic efficacy of fruit-derived exosomes in relevant animal models of cancer.12,145 Developing strategies to enhance their tumor-homing ability, such as surface modification with targeting ligands, may improve their specificity and therapeutic index. Additionally, investigating their potential as carriers for existing chemotherapeutic agents or in combination with other treatment modalities, such as immunotherapy, could lead to more effective cancer treatment approaches.36,151,152 In summary, while fruit-derived exosomes demonstrate significant potential for precision cancer therapy, addressing challenges related to standardization, yield optimization, mechanistic understanding, and clinical translation is pivotal to fully unlocking their therapeutic promise. Collaborative efforts among researchers, clinicians, and industry partners will be crucial to drive progress in this field and ultimately improve patient outcomes in cancer treatment.
9. Conclusion
Fruit-derived exosomes stand as a testament to the powerful synergy between nature's bounty and cutting-edge medical science. These nanoscale vesicles, derived from fruits, hold immense promise for revolutionizing cancer treatment by ushering in a new era of precision therapy. Their inherent biocompatibility, low immunogenicity, and remarkable ability to navigate biological barriers position them as exceptional candidates for targeted drug delivery, offering a potent weapon in the fight against cancer. While the field is still in its nascent stages, the therapeutic potential of fruit-derived exosomes is undeniable. Early research has illustrated their ability to effectively encapsulate and deliver a diverse array of therapeutic agents, including chemotherapeutics, small interfering RNAs, and microRNAs, directly to cancer cells with remarkable precision. This targeted approach minimizes off-target effects, reducing the often debilitating side effects associated with conventional cancer treatments. However, like any evolving field, challenges remain. Standardizing extraction and isolation methods is crucial to ensure consistent purity, yield, and ultimately, therapeutic efficacy. The lack of standardized protocols currently hinders the ability to compare results across studies and establish a clear consensus on the clinical potential of fruit-derived exosomes. Furthermore, a deeper understanding of fruit-derived exosome biogenesis, cargo loading mechanisms, and their intricate interactions with recipient cells is essential to fully unlock their therapeutic potential. Despite these challenges, the future of fruit exosome-based cancer therapy is ripe with opportunities. Advancements in nanotechnology and molecular engineering hold the key to further enhancing their targeting capabilities, allowing for the development of even more precise delivery systems tailored to specific cancer types and molecular subtypes. This will let us breathe in a future where cancer treatment is not only more effective but also significantly less toxic, improving the quality of life for countless individuals battling this devastating disease. The journey towards realizing the full potential of these nanoscale vesicles in precision cancer therapy requires a collaborative, interdisciplinary approach. By uniting the expertise of plant biologists, nanotechnologists, pharmacologists, and oncologists, we can overcome the current hurdles and pave the way for a future where nature's own delivery vehicles revolutionize cancer treatment. The seeds of progress have been sown, and with continued dedication and innovation, we can cultivate a future where exosomes blossom into a powerful weapon in the fight against cancer.
Data availability
Data sharing is not applicable to this article as no datasets were generated or analyzed during the current study.
Conflicts of interest
The authors of this article declare no conflicts of interest.
References
- R. L. Siegel, K. D. Miller, N. S. Wagle and A. Jemal, Cancer statistics, Ca-Cancer J. Clin., 2023, 73(1), 17–48 CrossRef
.
- F. Bray, M. Laversanne, H. Sung, J. Ferlay, R. L. Siegel, I. Soerjomataram and A. Jemal, Global cancer statistics 2022: GLOBOCAN estimates of incidence and mortality worldwide for 36 cancers in 185 countries, Ca-Cancer J. Clin., 2024, 74(3), 229–263 CrossRef
.
- J. Dai, Y. Su, S. Zhong, L. Cong, B. Liu, J. Yang, Y. Tao, Z. He, C. Chen and Y. Jiang, Exosomes: key players in cancer and potential therapeutic strategy, Signal Transduction Targeted Ther., 2020, 5(1), 145 CrossRef CAS PubMed
.
- N. L. Syn, L. Wang, E. K. Chow, C. T. Lim and B. C. Goh, Exosomes in Cancer Nanomedicine and Immunotherapy: Prospects and Challenges, Trends Biotechnol., 2017, 35(7), 665–676 CrossRef CAS PubMed
.
- M. Pesec and T. Sherertz, Global health from a cancer care perspective, Future Oncol., 2015, 11(15), 2235–2245 CrossRef CAS PubMed
.
- P. Ranganathan, A. Dare, E. M. Harrison, T. P. Kingham, M. Mutebi, G. Parham, R. Sullivan and C. S. Pramesh, Inequities in global cancer surgery: challenges and solutions, J. Surg. Oncol., 2024, 129(1), 150–158 CrossRef PubMed
.
- C. Are, S. Rajaram, M. Are, H. Raj, B. O. Anderson, R. Chaluvarya Swamy, M. Vijayakumar, T. Song, M. Pandey, J. A. Edney and E. L. Cazap, A review of global cancer burden: trends, challenges, strategies, and a role for surgeons, J. Surg. Oncol., 2013, 107(2), 221–226 CrossRef
.
- W. Meng, C. He, Y. Hao, L. Wang, L. Li and G. Zhu, Prospects and challenges of extracellular vesicle-based drug delivery system: considering cell source, Drug Deliv., 2020, 27(1), 585–598 CrossRef CAS
.
- G. Kibria, E. K. Ramos, Y. Wan, D. R. Gius and H. Liu, Exosomes as a Drug Delivery System in Cancer Therapy: Potential and Challenges, Mol. Pharm., 2018, 15(9), 3625–3633 CrossRef CAS
.
- W. Liao, Y. Du, C. Zhang, F. Pan, Y. Yao, T. Zhang and Q. Peng, Exosomes: the next generation of endogenous nanomaterials for advanced drug delivery and therapy, Acta Biomater., 2019, 86, 1–14 CrossRef CAS
.
- S. Madhan, R. Dhar and A. Devi, Plant-derived exosomes: a green approach for cancer drug delivery, J. Mater. Chem. B, 2024, 12(9), 2236–2252 RSC
.
- N. P. Ly, H. S. Han, M. Kim, J. H. Park and K. Y. Choi, Plant-derived nanovesicles: current understanding and applications for cancer therapy, Bioact. Mater., 2022, 22, 365–383 Search PubMed
.
- O. Urzì, S. Raimondo and R. Alessandro, Extracellular Vesicles from Plants: Current Knowledge and Open Questions, Int. J. Mol. Sci., 2021, 22(10), 5366 CrossRef
.
- E. D. Teodor, O. Ungureanu, F. Gatea and G. L. Radu, The Potential of Flavonoids and Tannins from Medicinal Plants as Anticancer Agents, Anti-Cancer Agents Med. Chem., 2020, 20(18), 2216–2227 CrossRef CAS
.
- T. Khan, M. Ali, A. Khan, P. Nisar, S. A. Jan, S. Afridi and Z. K. Shinwari, Anticancer Plants: A Review of the Active Phytochemicals, Applications in Animal Models, and Regulatory Aspects, Biomolecules, 2019, 10(1), 47 CrossRef
.
- A. Ranjan, S. Ramachandran, N. Gupta, I. Kaushik, S. Wright, S. Srivastava, H. Das, S. Srivastava, S. Prasad and S. K. Srivastava, Role of Phytochemicals in Cancer Prevention, Int. J. Mol. Sci., 2019, 20(20), 4981 CrossRef CAS
.
- Y. Zhao, H. Tan, J. Zhang, B. Pan, N. Wang, T. Chen, Y. Shi and Z. Wang, Plant-Derived Vesicles: A New Era for Anti-Cancer Drug Delivery and Cancer Treatment, Int. J. Nanomed., 2023, 18, 6847–6868 CrossRef CAS PubMed
.
- M. Q. Lian, W. H. Chng, J. Liang, H. Q. Yeo, C. K. Lee, M. Belaid, M. Tollemeto, M. G. Wacker, B. Czarny and G. Pastorin, Plant-derived extracellular vesicles: recent advancements and current challenges on their use for biomedical applications, J. Extracell. Vesicles, 2022, 11(12), e12283 CrossRef PubMed
.
- L. Y. Yang, C. Q. Li, Y. L. Zhang, M. W. Ma, W. Cheng and G. J. Zhang, Emerging Drug Delivery Vectors: Engineering of Plant-Derived Nanovesicles and Their Applications in Biomedicine, Int. J. Nanomed., 2024, 19, 2591–2610 CrossRef PubMed
.
- X. Chen, S. Ji, Y. Yan, S. Lin, L. He, X. Huang, L. Chang, D. Zheng and Y. Lu, Engineered Plant-Derived Nanovesicles Facilitate Tumor Therapy: Natural Bioactivity Plus Drug Controlled Release Platform, Int. J. Nanomed., 2023, 18, 4779–4804 CrossRef CAS PubMed
.
- L. Cui, G. Perini, V. Palmieri, M. De Spirito and M. Papi, Plant-Derived Extracellular Vesicles as a Novel Frontier in Cancer Therapeutics, Nanomaterials, 2024, 14(16), 1331 CrossRef CAS PubMed
.
- B. M. Hussen, G. S. H. Faraj, M. F. Rasul, H. J. Hidayat, A. Salihi, A. Baniahmad, M. Taheri and S. Ghafouri-Frad, Strategies to overcome the main challenges of the use of exosomes as drug carrier for cancer therapy, Cancer Cell Int., 2022, 22(1), 323 CrossRef PubMed
.
- N. P. Hessvik and A. Llorente, Current knowledge on exosome biogenesis and release, Cell. Mol. Life Sci., 2018, 75(2), 193–208 CrossRef CAS
.
- Q. F. Han, W. J. Li, K. S. Hu, J. Gao, W. L. Zhai, J. H. Yang and S. J. Zhang, Exosome biogenesis: machinery, regulation, and therapeutic implications in cancer, Mol. Cancer, 2022, 21(1), 207 CrossRef PubMed
.
- I. M. Sall and T. A. Flaviu, Plant and mammalian-derived extracellular vesicles: a new therapeutic approach for the future, Front. Bioeng. Biotechnol., 2023, 11, 1215650 CrossRef PubMed
.
- E. Maricchiolo, E. Panfili, A. Pompa, F. De Marchis, M. Bellucci and M. T. Pallotta, Unconventional Pathways of Protein Secretion: Mammals vs. Plants, Front. Cell Dev. Biol., 2022, 10, 895853 CrossRef
.
- J. Wang, Y. Ding, J. Wang, S. Hillmer, Y. Miao, S. W. Lo, X. Wang, D. G. Robinson and L. Jiang, EXPO, an exocyst-positive organelle distinct from multivesicular endosomes and autophagosomes, mediates cytosol to cell wall exocytosis in Arabidopsis and tobacco cells, Plant Cell, 2010, 22(12), 4009–4030 CrossRef CAS PubMed
.
- J. T. Farley, M. K. Eldahshoury and C. de Marcos Lousa, Unconventional Secretion of Plant Extracellular Vesicles and Their Benefits to Human Health: A Mini Review, Front. Cell Dev. Biol., 2022, 10, 883841 CrossRef
.
- Y. Zhang, Y. Liu, H. Liu and W. H. Tang, Exosomes: biogenesis, biologic function and clinical potential, Cell Biosci., 2019, 9, 19 CrossRef
.
- Q. An, A. J. van Bel and R. Hückelhoven, Do plant cells secrete exosomes derived from multivesicular bodies?, Plant Signaling Behav., 2007, 2(1), 4–7 CrossRef
.
- Y. Cui, Y. He, W. Cao, J. Gao and L. Jiang, The Multivesicular Body and Autophagosome Pathways in Plants, Front. Plant Sci., 2018, 9, 1837 CrossRef
.
- L. Christ, C. Raiborg, E. M. Wenzel, C. Campsteijn and H. Stenmark, Cellular Functions and Molecular Mechanisms of the ESCRT Membrane-Scission Machinery, Trends Biochem. Sci., 2017, 42(1), 42–56 CrossRef CAS PubMed
.
- T. Wollert and J. H. Hurley, Molecular mechanism of multivesicular body biogenesis by ESCRT complexes, Nature, 2010, 464(7290), 864–869 CrossRef CAS PubMed
.
- A. L. Schuh and A. Audhya, The ESCRT machinery: from the plasma membrane to endosomes and back again, Crit. Rev. Biochem. Mol. Biol., 2014, 49(3), 242–261 CrossRef CAS
.
- L. Zhang, J. Xing and J. Lin, At the intersection of exocytosis and endocytosis in plants, New Phytol., 2019, 224(4), 1479–1489 CrossRef CAS PubMed
.
- B. Zhao, H. Lin, X. Jiang, W. Li, Y. Gao, M. Li, Y. Yu, N. Chen and J. Gao, Exosome-like nanoparticles derived from fruits, vegetables, and herbs: innovative strategies of therapeutic and drug delivery, Theranostics, 2024, 14(12), 4598–4621 CrossRef CAS PubMed
.
- L. Xiang, E. Etxeberria and W. Van den Ende, Vacuolar protein sorting mechanisms in plants, FEBS J., 2013, 280(4), 979–993 CrossRef CAS
.
- B. Wang, X. Zhuang, Z. B. Deng, H. Jiang, J. Mu, Q. Wang, X. Xiang, H. Guo, L. Zhang, G. Dryden, J. Yan, D. Miller and H. G. Zhang, Targeted drug delivery to intestinal macrophages by bioactive nanovesicles released from grapefruit, Mol. Ther., 2014, 22(3), 522–534 CrossRef CAS
.
- R. Lee, H. J. Ko, K. Kim, Y. Sohn, S. Y. Min, J. A. Kim, D. Na and J. H. Yeon, Anti-melanogenic effects of extracellular vesicles derived from plant leaves and stems in mouse melanoma cells and human healthy skin, J. Extracell. Vesicles, 2019, 9(1), 1703480 CrossRef
.
- Q. Yi, Z. Xu, A. Thakur, K. Zhang, Q. Liang, Y. Liu and Y. Yan, Current understanding of plant-derived exosome-like nanoparticles in regulating the inflammatory response and immune system microenvironment, Pharmacol. Res., 2023, 190, 106733 CrossRef CAS
.
- S. Ju, J. Mu, T. Dokland, X. Zhuang, Q. Wang, H. Jiang, X. Xiang, Z. B. Deng, B. Wang, L. Zhang, M. Roth, R. Welti, J. Mobley, Y. Jun, D. Miller and H. G. Zhang, Grape exosome-like nanoparticles induce intestinal stem cells and protect mice from DSS-induced colitis, Mol. Ther., 2013, 21(7), 1345–1357 CrossRef CAS
.
- H. A. Dad, T. W. Gu, A. Q. Zhu, L. Q. Huang and L. H. Peng, Plant Exosome-like Nanovesicles: Emerging Therapeutics and Drug Delivery Nanoplatforms, Mol. Ther., 2021, 29(1), 13–31 CrossRef CAS
.
- D. Subha, K. Harshnii, K. G. Madhikiruba, M. Nandhini and K. S. Tamilselvi, Plant derived exosome- like nanovesicles: an updated overview, Plant Nano Biol., 2022, 3, 100022 CrossRef
.
- A. Kilasoniya, L. Garaeva, T. Shtam, A. Spitsyna, E. Putevich, B. Moreno-Chamba, J. Salazar-Bermeo, E. Komarova, A. Malek, M. Valero and D. Saura, Potential of Plant Exosome Vesicles from Grapefruit (Citrus × paradisi) and Tomato (Solanum lycopersicum) Juices as Functional Ingredients and Targeted Drug Delivery Vehicles, Antioxidants, 2023, 12(4), 943 CrossRef CAS PubMed
.
- M. Omrani, H. Beyrampour-Basmenj, R. Jahanban-Esfahlan, M. Talebi, M. Raeisi, Z. A. Serej, N. Akbar-Gharalari, S. Khodakarimi, J. Wu and A. Ebrahimi-Kalan, Global trend in exosome isolation and application: an update concept in management of diseases, Mol. Cell. Biochem., 2024, 479(3), 679–691 CrossRef CAS
.
- W. Z. Liu, Z. J. Ma and X. W. Kang, Current status and outlook of advances in exosome isolation, Anal. Bioanal. Chem., 2022, 414(24), 7123–7141 CrossRef CAS PubMed
.
- C. Gardiner, D. Di Vizio, S. Sahoo, C. Théry, K. W. Witwer, M. Wauben and A. F. Hill, Techniques used for the isolation and characterization of extracellular vesicles: results of a worldwide survey, J. Extracell. Vesicles, 2016, 5, 32945 CrossRef PubMed
.
- R. J. Lobb, M. Becker, S. W. Wen, C. S. Wong, A. P. Wiegmans, A. Leimgruber and A. Möller, Optimized exosome isolation protocol for cell culture supernatant and human plasma, J. Extracell. Vesicles, 2015, 4, 27031 CrossRef PubMed
.
- S. Kamerkar, V. S. LeBleu, H. Sugimoto, S. Yang, C. F. Ruivo, S. A. Melo, J. J. Lee and R. Kalluri, Exosomes facilitate therapeutic targeting of oncogenic KRAS in pancreatic cancer, Nature, 2017, 546(7659), 498–503 CrossRef CAS
.
- R. Ghosh, Rapid antibody screening by membrane chromatographic immunoassay technique, J. Chromatogr. B: Anal. Technol. Biomed. Life Sci., 2006, 844(1), 163–167 CrossRef CAS
.
- B. Lebreton, A. Brown and R. van Reis, Application of high-performance tangential flow filtration (HPTFF) to the purification of a human pharmaceutical antibody fragment expressed in Escherichia coli, Biotechnol. Bioeng., 2008, 100(5), 964–974 CrossRef CAS PubMed
.
- L. Ding, X. Yang, Z. Gao, C. Y. Effah, X. Zhang, Y. Wu and L. Qu, A Holistic Review of the State-of-the-Art Microfluidics for Exosome Separation: An Overview of the Current Status, Existing Obstacles, and Future Outlook, Small, 2021, 17(29), e2007174 CrossRef PubMed
.
- Y. Tian, M. Gong, Y. Hu, H. Liu, W. Zhang, M. Zhang, X. Hu, D. Aubert, S. Zhu, L. Wu and X. Yan, Quality and efficiency assessment of six extracellular vesicle isolation methods by nano-flow cytometry, J. Extracell. Vesicles, 2019, 9(1), 1697028 CrossRef
.
- G. K. Patel, M. A. Khan, H. Zubair, S. K. Srivastava, M. Khushman, S. Singh and A. P. Singh, Comparative analysis of exosome isolation methods using culture supernatant for optimum yield, purity and downstream applications, Sci. Rep., 2019, 9(1), 5335 CrossRef PubMed
.
- M. Zhang, K. Jin, L. Gao, Z. Zhang, F. Li, F. Zhou and L. Zhang, Methods and Technologies for Exosome Isolation and Characterization, Small Methods, 2018, 2(9), 1800021 CrossRef
.
- S. Lin, Z. Yu, D. Chen, Z. Wang, J. Miao, Q. Li, D. Zhang, J. Song and D. Cui, Progress in Microfluidics-Based Exosome Separation and Detection Technologies for Diagnostic Applications, Small, 2020, 16(9), e1903916 CrossRef PubMed
.
- B. Lin, Y. Lei, J. Wang, L. Zhu, Y. Wu, H. Zhang, L. Wu, P. Zhang and C. Yang, Microfluidic-Based Exosome Analysis for Liquid Biopsy, Small Methods, 2021, 5(3), e2001131 CrossRef
.
- Z. Wang, F. Li, J. Rufo, C. Chen, S. Yang, L. Li, J. Zhang, J. Cheng, Y. Kim, M. Wu, E. Abemayor, M. Tu, D. Chia, R. Spruce, N. Batis, H. Mehanna, D. T. W. Wong and T. J. Huang, Acoustofluidic Salivary Exosome Isolation: A Liquid Biopsy Compatible Approach for Human Papillomavirus-Associated Oropharyngeal Cancer Detection, J. Mol. Diagn., 2020, 22(1), 50–59 CrossRef CAS
.
- K. Zhang, Y. Yue, S. Wu, W. Liu, J. Shi and Z. Zhang, Rapid Capture and Nondestructive Release of Extracellular Vesicles Using Aptamer-Based Magnetic Isolation, ACS Sens., 2019, 4(5), 1245–1251 CrossRef CAS
.
- S. M. Santana, M. A. Antonyak, R. A. Cerione and B. J. Kirby, Microfluidic isolation of cancer-cell-derived microvesicles from hetergeneous extracellular shed vesicle populations, Biomed. Microdevices, 2014, 16(6), 869–877 CrossRef CAS
.
- B. H. Wunsch, J. T. Smith, S. M. Gifford, C. Wang, M. Brink, R. L. Bruce, R. H. Austin, G. Stolovitzky and Y. Astier, Nanoscale lateral displacement arrays for the separation of exosomes and colloids down to 20 nm, Nat. Nanotechnol., 2016, 11(11), 936–940 CrossRef CAS PubMed
.
- D. Kang, S. Oh, S. M. Ahn, B. H. Lee and M. H. Moon, Proteomic analysis of exosomes from human neural stem cells by flow field-flow fractionation and nanoflow liquid chromatography-tandem mass spectrometry, J. Proteome Res., 2008, 7(8), 3475–3480 CrossRef CAS PubMed
.
- H. Zhang and D. Lyden, Asymmetric-flow field-flow fractionation technology for exomere and small extracellular vesicle separation and characterization, Nat. Protoc., 2019, 14(4), 1027–1053 CrossRef CAS
.
- H. Zhang, D. Freitas, H. S. Kim, K. Fabijanic, Z. Li, H. Chen, M. T. Mark, H. Molina, A. B. Martin, L. Bojmar, J. Fang, S. Rampersaud, A. Hoshino, I. Matei, C. M. Kenific, M. Nakajima, A. P. Mutvei, P. Sansone, W. Buehring, H. Wang, J. P. Jimenez, L. Cohen-Gould, N. Paknejad, M. Brendel, K. Manova-Todorova, A. Magalhães, J. A. Ferreira, H. Osório, A. M. Silva, A. Massey, J. R. Cubillos-Ruiz, G. Galletti, P. Giannakakou, A. M. Cuervo, J. Blenis, R. Schwartz, M. S. Brady, H. Peinado, J. Bromberg, H. Matsui, C. A. Reis and D. Lyden, Identification of distinct nanoparticles and subsets of extracellular vesicles by asymmetric flow field-flow fractionation, Nat. Cell Biol., 2018, 20(3), 332–343 CrossRef CAS
.
- S. Sitar, A. Kejžar, D. Pahovnik, K. Kogej, M. Tušek-Žnidarič, M. Lenassi and E. Žagar, Size characterization and quantification of exosomes by asymmetrical-flow field-flow fractionation, Anal. Chem., 2015, 87(18), 9225–9233 CrossRef CAS PubMed
.
- H. Shao, H. Im, C. M. Castro, X. Breakefield, R. Weissleder and H. Lee, New Technologies for Analysis of Extracellular Vesicles, Chem. Rev., 2018, 118(4), 1917–1950 CrossRef CAS
.
- V. Sokolova, A. K. Ludwig, S. Hornung, O. Rotan, P. A. Horn, M. Epple and B. Giebel, Characterisation of exosomes derived from human cells by nanoparticle tracking analysis and scanning electron microscopy, Colloids Surf., B, 2011, 87(1), 146–150 CrossRef CAS PubMed
.
- R. A. Dragovic, C. Gardiner, A. S. Brooks, D. S. Tannetta, D. J. Ferguson, P. Hole, B. Carr, C. W. Redman, A. L. Harris, P. J. Dobson, P. Harrison and I. L. Sargent, Sizing and phenotyping of cellular vesicles using nanoparticle tracking analysis, Nanomedicine, 2011, 7(6), 780–788 CrossRef CAS PubMed
.
- A. S. Lawrie, A. Albanyan, R. A. Cardigan, I. J. Mackie and P. Harrison, Microparticle sizing by dynamic light scattering in fresh-frozen plasma, Vox Sang., 2009, 96(3), 206–212 CrossRef CAS
.
- Y. Yuana, T. H. Oosterkamp, S. Bahatyrova, B. Ashcroft, P. Garcia Rodriguez, R. M. Bertina and S. Osanto, Atomic force microscopy: a novel approach to the detection of nanosized blood microparticles, J. Thromb. Haemostasis, 2010, 8(2), 315–323 CrossRef CAS
.
- T. Katsuda, N. Kosaka and T. Ochiya, The roles of extracellular vesicles in cancer biology: toward the development of novel cancer biomarkers, Proteomics, 2014, 14(4–5), 412–425 CrossRef CAS PubMed
.
- N. Arraud, C. Gounou, D. Turpin and A. R. Brisson, Fluorescence triggering: a general strategy for enumerating and phenotyping extracellular vesicles by flow cytometry, Cytometry, Part A, 2016, 89(2), 184–195 CrossRef CAS
.
- I. Tatischeff, E. Larquet, J. M. Falcón-Pérez, P. Y. Turpin and S. G. Kruglik, Fast characterisation of cell-derived extracellular vesicles by nanoparticles tracking analysis, cryo-electron microscopy, and Raman tweezers microspectroscopy, J. Extracell. Vesicles, 2012, 1, 19179 CrossRef
.
- S. Kreimer, A. M. Belov, I. Ghiran, S. K. Murthy, D. A. Frank and A. R. Ivanov, Mass-spectrometry-based molecular characterization of extracellular vesicles: lipidomics and proteomics, J. Proteome Res., 2015, 14(6), 2367–2384 CrossRef CAS PubMed
.
- G. Pocsfalvi, C. Stanly, A. Vilasi, I. Fiume, G. Capasso, L. Turiák, E. I. Buzas and K. Vékey, Mass spectrometry of extracellular vesicles, Mass Spectrom. Rev., 2016, 35(1), 3–21 CrossRef CAS PubMed
.
- D. S. Choi, D. K. Kim, Y. K. Kim and Y. S. Gho, Proteomics, transcriptomics and lipidomics of exosomes and ectosomes, Proteomics, 2013, 13(10–11), 1554–1571 CrossRef CAS PubMed
.
- K. Clack, N. Soda, S. Kasetsirikul, R. G. Mahmudunnabi, N. T. Nguyen and M. J. A. Shiddiky, Toward Personalized Nanomedicine: The Critical Evaluation of Micro and Nanodevices for Cancer Biomarker Analysis in Liquid Biopsy, Small, 2023, 19(15), e2205856 CrossRef PubMed
.
- F. Z. Farhana, M. Umer, A. Saeed, A. S. Pannu, M. Shahbazi, A. Jabur, H. J. Nam, K. Ostrikov, P. Sonar, S. H. Firoz and M. J. Shiddiky, Isolation and detection of exosomes using Fe2O3 nanoparticles, ACS Appl. Nano Mater., 2021, 4(2), 1175–1186 CrossRef CAS
.
- K. Boriachek, M. N. Islam, A. Möller, C. Salomon, N. T. Nguyen, M. S. A. Hossain, Y. Yamauchi and M. J. A. Shiddiky, Biological Functions and Current Advances in Isolation and Detection Strategies for Exosome Nanovesicles, Small, 2018, 14(6), 1702153 CrossRef
.
- M. K. Masud, J. Na, M. Younus, M. S. A. Hossain, Y. Bando, M. J. A. Shiddiky and Y. Yamauchi, Superparamagnetic nanoarchitectures for disease-specific biomarker detection, Chem. Soc. Rev., 2019, 48(24), 5717–5751 RSC
.
- S. Maheshwari, A. K. Singh, R. K. Arya, D. Pandey, A. Singh and D. Datta, Exosomes: Emerging Players of Intercellular Communication in Tumor Microenvironment, Discoveries, 2014, 2(3), e26 CrossRef PubMed
.
- J. Maia, S. Caja, M. C. Strano Moraes, N. Couto and B. Costa-Silva, Exosome-Based Cell-Cell Communication in the Tumor Microenvironment, Front. Cell Dev. Biol., 2018, 6, 18 CrossRef PubMed
.
- D. Hanahan and R. A. Weinberg, Hallmarks of cancer: the next generation, Cell, 2011, 144(5), 646–674 CrossRef CAS
.
- V. Petrova, M. Annicchiarico-Petruzzelli, G. Melino and I. Amelio, The hypoxic tumour microenvironment, Oncogenesis, 2018, 7(1), 10 CrossRef
.
- Z. Chen, F. Han, Y. Du, H. Shi and W. Zhou, Hypoxic microenvironment in cancer: molecular mechanisms and therapeutic interventions, Signal Transduction Targeted Ther., 2023, 8(1), 70 CrossRef
.
- G. L. Semenza, Hypoxia-inducible factors: mediators of cancer progression and targets for cancer therapy, Trends Pharmacol. Sci., 2012, 33(4), 207–214 CrossRef CAS PubMed
.
- P. Vaupel, The role of hypoxia-induced factors in tumor progression, Oncologist, 2004, 9(suppl. 5), 10–17 CrossRef CAS PubMed
.
- B. Muz, P. de la Puente, F. Azab and A. K. Azab, The role of hypoxia in cancer progression, angiogenesis, metastasis, and resistance to therapy, Hypoxia, 2015, 3, 83–92 CrossRef PubMed
.
- J. Zhou, T. Schmid, S. Schnitzer and B. Brüne, Tumor hypoxia and cancer progression, Cancer Lett., 2006, 237(1), 10–21 CrossRef CAS
.
- A. C. Chiang and J. Massagué, Molecular basis of metastasis, N. Engl. J. Med., 2008, 359(26), 2814–2823 CrossRef CAS PubMed
.
- J. E. Park, H. S. Tan, A. Datta, R. C. Lai, H. Zhang, W. Meng, S. K. Lim and S. K. Sze, Hypoxic tumor cell modulates its microenvironment to enhance angiogenic and metastatic potential by secretion of proteins and exosomes, Mol. Cell. Proteomics, 2010, 9(6), 1085–1099 CrossRef CAS
.
- C. Roma-Rodrigues, A. R. Fernandes and P. V. Baptista, Exosome in tumour microenvironment: overview of the crosstalk between normal and cancer cells, BioMed Res. Int., 2014, 2014, 179486 Search PubMed
.
- C. Aslan, S. Maralbashi, F. Salari, H. Kahroba, F. Sigaroodi, T. Kazemi and P. Kharaziha, TEXs: Implication in angiogenesis and antiangiogenesis cancer therapy, J. Cell. Physiol., 2019, 234(10), 16885–16903 CrossRef CAS PubMed
.
- P. Fasanaro, Y. D'Alessandra, V. Di Stefano, R. Melchionna, S. Romani, G. Pompilio, M. C. Capogrossi and F. Martelli, MicroRNA-210 modulates endothelial cell response to hypoxia and inhibits the receptor tyrosine kinase ligand Ephrin-A3, J. Biol. Chem., 2008, 283(23), 15878–15883 CrossRef CAS
.
- S. Landskroner-Eiger, I. Moneke and W. C. Sessa, miRNAs as modulators of angiogenesis, Cold Spring Harbor Perspect. Med., 2013, 3(2), a006643 Search PubMed
.
- X. Shao, S. Hua, T. Feng, D. K. W. Ocansey and L. Yin, Hypoxia-Regulated TEXs and Tumor Progression: A Focus on Immune Evasion, Int. J. Mol. Sci., 2022, 23(19), 11789 CrossRef CAS PubMed
.
- W. Guo, T. Qiao, B. Dong, T. Li, Q. Liu and X. Xu, The Effect of Hypoxia-Induced Exosomes on Anti-Tumor Immunity and Its Implication for Immunotherapy, Front. Immunol., 2022, 13, 915985 CrossRef CAS PubMed
.
- T. L. Whiteside, TEXs and Their Role in Tumor-Induced Immune Suppression, Vaccines, 2016, 4(4), 35 CrossRef
.
- S. M. Morrissey, F. Zhang, C. Ding, D. E. Montoya-Durango, X. Hu, C. Yang, Z. Wang, F. Yuan, M. Fox, H. G. Zhang, H. Guo, D. Tieri, M. Kong, C. T. Watson, R. A. Mitchell, X. Zhang, K. M. McMasters, J. Huang and J. Yan, TEXs drive immunosuppressive macrophages in a pre-metastatic niche through glycolytic dominant metabolic reprogramming, Cell Metab., 2021, 33(10), 2040–2058 CrossRef CAS PubMed
.
- R. Sengupta, I. S. Topiwala, A. M. Shakthi, R. Dhar and A. Devi, Immune Cell-Derived Exosomes: A Cell-Free Cutting-Edge Tumor Immunotherapy, ACS Appl. Bio Mater., 2024 DOI:10.1021/acsabm.4c00660
.
- X. Tian, H. Shen, Z. Li, T. Wang and S. Wang, Tumor-derived exosomes, myeloid-derived suppressor cells, and tumor microenvironment, J. Hematol. Oncol., 2019, 12(1), 84 CrossRef PubMed
.
- R. Hosseini, L. Asef-Kabiri, H. Yousefi, H. Sarvnaz, M. Salehi, M. E. Akbari and N. Eskandari, The roles of tumor-derived exosomes in altered differentiation, maturation and function of dendritic cells, Mol. Cancer, 2021, 20(1), 83 CrossRef CAS
.
- J. Jiang, J. Li, X. Zhou, X. Zhao, B. Huang and Y. Qin, Exosomes Regulate the Epithelial-Mesenchymal Transition in Cancer, Front. Oncol., 2022, 12, 864980 CrossRef CAS
.
- C. Yang, R. Dou, C. Wei, K. Liu, D. Shi, C. Zhang, Q. Liu, S. Wang and B. Xiong, Tumor-derived exosomal microRNA-106b-5p activates EMT-cancer cell and M2-subtype TAM interaction to facilitate CRC metastasis, Mol. Ther., 2021, 29(6), 2088–2107 CrossRef CAS PubMed
.
- A. Das, P. Saha, K. Kalele and S. Sonar, Clinical signature of exosomal tetraspanin proteins in cancer, Clin. Transl. Discovery, 2024, 4, e341 CrossRef CAS
.
- A. Hoshino, B. Costa-Silva, T. L. Shen, G. Rodrigues, A. Hashimoto, M. Tesic Mark, H. Molina, S. Kohsaka, A. Di Giannatale, S. Ceder, S. Singh, C. Williams, N. Soplop, K. Uryu, L. Pharmer, T. King, L. Bojmar, A. E. Davies, Y. Ararso, T. Zhang, H. Zhang, J. Hernandez, J. M. Weiss, V. D. Dumont-Cole, K. Kramer, L. H. Wexler, A. Narendran, G. K. Schwartz, J. H. Healey, P. Sandstrom, K. J. Labori, E. H. Kure, P. M. Grandgenett, M. A. Hollingsworth, M. de Sousa, S. Kaur, M. Jain, K. Mallya, S. K. Batra, W. R. Jarnagin, M. S. Brady, O. Fodstad, V. Muller, K. Pantel, A. J. Minn, M. J. Bissell, B. A. Garcia, Y. Kang, V. K. Rajasekhar, C. M. Ghajar, I. Matei, H. Peinado, J. Bromberg and D. Lyden, Tumour exosome integrins determine organotropic metastasis, Nature, 2015, 527(7578), 329–335 CrossRef CAS
.
- X. Qin, S. Yu, L. Zhou, M. Shi, Y. Hu, X. Xu, B. Shen, S. Liu, D. Yan and J. Feng, Cisplatin-resistant lung cancer cell-derived exosomes increase cisplatin resistance of recipient cells in exosomal miR-100-5p-dependent manner, Int. J. Nanomed., 2017, 12, 3721–3733 CrossRef CAS
.
- X. Dong, X. Bai, J. Ni, H. Zhang, W. Duan, P. Graham and Y. Li, Exosomes and breast cancer drug resistance, Cell Death Dis., 2020, 11(11), 987 CrossRef CAS PubMed
.
- Y. Guo, X. Ji, J. Liu, D. Fan, Q. Zhou, C. Chen, W. Wang, G. Wang, H. Wang, W. Yuan, Z. Ji and Z. Sun, Effects of exosomes on pre-metastatic niche formation in tumors, Mol. Cancer, 2019, 18(1), 39 CrossRef
.
- R. Dhar and A. Devi, Engineered cell versus modified
exosomes in cancer therapy, Clin. Transl. Discovery, 2024, 4, e320 CrossRef CAS
.
- Q. Chen, M. Zu, H. Gong, Y. Ma, J. Sun, S. Ran, X. Shi, J. Zhang and B. Xiao, Tea leaf-derived exosome-like nanotherapeutics retard breast tumor growth by pro-apoptosis and microbiota modulation, J. Nanobiotechnol., 2023, 21(1), 6 CrossRef CAS
.
- R. T. Morales and J. Ko, Future of Digital Assays to Resolve Clinical Heterogeneity of Single Extracellular Vesicles, ACS Nano, 2022, 16(8), 11619–11645 CrossRef CAS
.
- D. Mirgh, S. Sonar, S. Ghosh, M. D. Adhikari, V. Subramaniyan, S. Gorai and K. Anand, Landscape of exosomes to modified exosomes: a state of the art in cancer therapy, RSC Adv., 2024, 14(42), 30807–30829 RSC
.
- S. Raimondo, F. Naselli, S. Fontana, F. Monteleone, A. Lo Dico, L. Saieva, G. Zito, A. Flugy, M. Manno, M. A. Di Bella, G. De Leo and R. Alessandro, Citrus limon-derived nanovesicles inhibit cancer cell proliferation and suppress CML xenograft growth by inducing TRAIL-mediated cell death, Oncotarget, 2015, 6(23), 19514–19527 CrossRef PubMed
.
- Z. L. Tan, J. F. Li, H. M. Luo, Y. Y. Liu and Y. Jin, Plant extracellular vesicles: a novel bioactive nanoparticle for tumor therapy, Front. Pharmacol, 2022, 13, 1006299 CrossRef CAS
.
- L. Cui, G. Perini, A. Augello, V. Palmieri, M. De Spirito and M. Papi, Plant-derived extracellular nanovesicles: a promising biomedical approach for effective targeting of triple negative breast cancer cells, Front. Bioeng. Biotechnol., 2024, 12, 1390708 CrossRef PubMed
.
- F. Perut, L. Roncuzzi, S. Avnet, A. Massa, N. Zini, S. Sabbadini, F. Giampieri, B. Mezzetti and N. Baldini, Strawberry-Derived Exosome-Like Nanoparticles Prevent Oxidative Stress in Human Mesenchymal Stromal Cells, Biomolecules, 2021, 11(1), 87 CrossRef CAS
.
- G. E. Forcados, D. B. James, A. B. Sallau, A. Muhammad and P. Mabeta, Oxidative Stress and Carcinogenesis: Potential of Phytochemicals in Breast Cancer Therapy, Nutr. Cancer, 2017, 69(3), 365–374 CrossRef CAS
.
- H. Gurer-Orhan, E. Ince, D. Konyar, L. Saso and S. Suzen, The Role of Oxidative Stress Modulators in Breast Cancer, Curr. Med. Chem., 2018, 25(33), 4084–4101 CrossRef CAS PubMed
.
- C. Stanly, M. Alfieri, A. Ambrosone, A. Leone, I. Fiume and G. Pocsfalvi, Grapefruit-Derived Micro and Nanovesicles Show Distinct Metabolome Profiles and Anticancer Activities in the A375 Human Melanoma Cell Line, Cells, 2020, 9(12), 2722 CrossRef CAS PubMed
.
- B. Qi, Y. Wang, Z. J. Chen, X. N. Li, Y. Qi, Y. Yang, G. H. Cui, H. Z. Guo, W. H. Li and S. Zhao, Down-regulation of miR-30a-3p/5p promotes esophageal squamous cell carcinoma cell proliferation by activating the Wnt signaling pathway, World J. Gastroenterol., 2017, 23(45), 7965–7977 CrossRef CAS
.
- C. Wang, L. Cai, J. Liu, G. Wang, H. Li, X. Wang, W. Xu, M. Ren, L. Feng, P. Liu and C. Zhang, MicroRNA-30a-5p Inhibits the Growth of Renal Cell Carcinoma by Modulating GRP78 Expression, Cell. Physiol. Biochem., 2017, 43(6), 2405–2419 CrossRef CAS PubMed
.
- M. Trentini, F. Zanotti, E. Tiengo, F. Camponogara, M. Degasperi, D. Licastro, L. Lovatti and B. Zavan, An Apple a Day Keeps the Doctor Away: Potential Role of miRNA 146 on Macrophages Treated with Exosomes Derived from Apples, Biomedicines, 2022, 10(2), 415 CrossRef CAS PubMed
.
- M. Yang, Q. Luo, X. Chen and F. Chen, Bitter melon derived extracellular vesicles enhance the therapeutic effects and reduce the drug resistance of 5-fluorouracil on oral squamous cell carcinoma, J. Nanobiotechnol., 2021, 19(1), 259 CrossRef CAS
.
- Y. Zhao, H. Tan, J. Zhang, B. Pan, N. Wang, T. Chen, Y. Shi and Z. Wang, Plant-Derived Vesicles: A New Era for Anti-Cancer Drug Delivery and Cancer Treatment, Int. J. Nanomed., 2023, 18, 6847–6868 CrossRef CAS PubMed
.
- Q. Xiao, W. Zhao, C. Wu, X. Wang, J. Chen, X. Shi, S. Sha, J. Li, X. Liang, Y. Yang, H. Guo, Y. Wang and J. B. Fan, Lemon-Derived Extracellular Vesicles Nanodrugs Enable to Efficiently Overcome Cancer Multidrug Resistance by Endocytosis-Triggered Energy Dissipation and Energy Production Reduction, Adv. Sci., 2022, 9(20), e2105274 CrossRef PubMed
.
- M. Rahimi Ghiasi, E. Rahimi, Z. Amirkhani and R. Salehi, Leucine-rich Repeat-containing G-protein Coupled Receptor 5 Gene Overexpression of the Rat Small Intestinal Progenitor Cells in Response to Orally Administered Grape Exosome-like Nanovesicles, Adv. Biomed. Res., 2018, 7, 125 CrossRef PubMed
.
- P. Sarvarian, P. Samadi, E. Gholipour, M. Khodadadi, R. Pourakbari, P. Akbarzadelale and K. Shamsasenjan, Fisetin-loaded grape-derived nanoparticles improve anticancer efficacy in MOLT-4 cells, Biochem. Biophys. Res. Commun., 2023, 658, 69–79 CrossRef CAS
.
- W. Niu, Q. Xiao, X. Wang, J. Zhu, J. Li, X. Liang, Y. Peng, C. Wu, R. Lu, Y. Pan, J. Luo, X. Zhong, H. He, Z. Rong, J. B. Fan and Y. Wang, A Biomimetic Drug Delivery System by Integrating Grapefruit Extracellular Vesicles and Doxorubicin-Loaded Heparin-Based Nanoparticles for Glioma Therapy, Nano Lett., 2021, 21(3), 1484–1492 CrossRef CAS
.
- L. Garaeva, R. Kamyshinsky, Y. Kil, E. Varfolomeeva, N. Verlov, E. Komarova, Y. Garmay, S. Landa, V. Burdakov, A. Myasnikov, I. A. Vinnikov, B. Margulis, I. Guzhova, A. Kagansky, A. L. Konevega and T. Shtam, Delivery of functional exogenous proteins by plant-derived vesicles to human cells in vitro, Sci. Rep., 2021, 11(1), 6489 CrossRef CAS
.
- W. Yan, M. Tao, B. Jiang, M. Yao, Y. Jun, W. Dai, Z. Tang, Y. Gao, L. Zhang, X. Chen and Q. L. Wang, Overcoming Drug Resistance in Colon Cancer by Aptamer-Mediated Targeted Co-Delivery of Drug and siRNA Using Grapefruit-Derived Nanovectors, Cell. Physiol. Biochem., 2018, 50(1), 79–91 CrossRef CAS PubMed
.
- Z. Tang, Y. Jun, Y. Lv, Y. Li, Z. Zhang, M. Tao, X. Chen, J. He, L. Zhang and Q. L. Wang, Aptamer-conjugated and doxorubicin-loaded grapefruit-derived nanovectors for targeted therapy against HER2+ breast cancer, J. Drug Targeting, 2020, 28(2), 186–194 CrossRef CAS PubMed
.
- M. C. López de Las Hazas, J. Tomé-Carneiro, L. Del Pozo-Acebo, A. Del Saz-Lara, L. A. Chapado, L. Balaguer, E. Rojo, J. C. Espín, C. Crespo, D. A. Moreno, C. García-Viguera, J. M. Ordovás, F. Visioli and A. Dávalos, Therapeutic potential of plant-derived extracellular vesicles as nanocarriers for exogenous miRNAs, Pharmacol. Res., 2023, 198, 106999 CrossRef
.
- S. Corvigno, Y. Liu, E. Bayraktar, E. Stur, N. N. Bayram, A. L. Ahumada, S. Nagaraju, C. Rodriguez-Aguayo, H. Chen, T. C. Vu, Y. Wen, H. Liang, L. Zhao, S. Lee, G. Lopez-Berestein and A. K. Sood, Enhanced plant-derived vesicles for nucleotide delivery for cancer therapy, npj Precis. Oncol., 2024, 8(1), 86 CrossRef CAS
.
- R. Di Raimo, D. Mizzoni, M. Spada, V. Dolo, S. Fais and M. Logozzi, Oral Treatment with Plant-Derived Exosomes Restores Redox Balance in H2O2-Treated Mice, Antioxidants, 2023, 12(6), 1169 CrossRef CAS PubMed
.
- X. Zhuang, Y. Teng, A. Samykutty, J. Mu, Z. Deng, L. Zhang, P. Cao, Y. Rong, J. Yan, D. Miller and H. G. Zhang, Grapefruit-derived Nanovectors Delivering Therapeutic miR17 Through an Intranasal Route Inhibit Brain Tumor Progression, Mol. Ther., 2016, 24(1), 96–105 CrossRef CAS PubMed
.
- S. Sonar and K. Anand, Plant-derived exosomes: a green nanomedicine for cancer, Clin. Transl. Discovery, 2024, 4, e333 CrossRef CAS
.
- A. Ramachandran, R. Dhar and A. Devi, Stem Cell-Derived Exosomes: An Advanced Horizon to Cancer Regenerative Medicine, ACS Appl. Bio Mater., 2024, 7(4), 2128–2139 CrossRef CAS PubMed
.
- E. J. Bunggulawa, W. Wang, T. Yin, N. Wang, C. Durkan, Y. Wang and G. Wang, Recent advancements in the use of exosomes as drug delivery systems, J. Nanobiotechnol., 2018, 16(1), 81 CrossRef CAS
.
- S. Sonar, Clinical trial status of exosomes-based cancer theranostics, Clin. Transl. Discovery, 2024, 4, e327 CrossRef CAS
.
- S. Nyahatkar and K. Kalele, Plant exosomes: a natural medicine for oral cancer, Clin. Transl. Discovery, 2024, 4, e269 CrossRef
.
- Y. S. Chen, E. Y. Lin, T. W. Chiou and H. J. Harn, Exosomes in clinical trial and their production in compliance with good manufacturing practice, Ciji Yixue Zazhi, 2019, 32(2), 113–120 Search PubMed
.
- J. Rezaie, M. Feghhi and T. Etemadi, A review on exosomes application in clinical trials: perspective, questions, and challenges, Cell Commun. Signaling, 2022, 20(1), 145 CrossRef
.
- S. Di Gioia, M. N. Hossain and M. Conese, Biological properties and therapeutic effects of plant-derived nanovesicles, Open Med., 2020, 15(1), 1096–1122 CrossRef CAS
.
- N. Mu, J. Li, L. Zeng, J. You, R. Li, A. Qin, X. Liu, F. Yan and Z. Zhou, Plant-Derived Exosome-Like Nanovesicles: Current Progress and Prospects, Int. J. Nanomed., 2023, 18, 4987–5009 CrossRef CAS
.
- M. Logozzi, R. Di Raimo, D. Mizzoni and S. Fais, The Potentiality of Plant-Derived Nanovesicles in Human Health-A Comparison with Human Exosomes and Artificial Nanoparticles, Int. J. Mol. Sci., 2022, 23(9), 4919 CrossRef CAS PubMed
.
- S. Q. Kim and K. H. Kim, Emergence of Edible Plant-Derived Nanovesicles as Functional Food Components and Nanocarriers for Therapeutics Delivery: Potentials in Human Health and Disease, Cells, 2022, 11(14), 2232 CrossRef CAS
.
- R. Agnihotram, R. Dhar, D. Dhar, K. Purushothaman, A. K. Narasimhan and A. Devi, Fusion of Exosomes and Nanotechnology: Cutting-Edge Cancer Theranostics, ACS Appl. Nano Mater., 2024, 7(8), 8489–8506 CrossRef CAS
.
- M. D. Langellotto, G. Rassu, C. Serri, S. Demartis, P. Giunchedi and E. Gavini, Plant-derived extracellular vesicles: a synergetic combination of a drug delivery system and a source of natural bioactive compounds, Drug Delivery Transl. Res., 2024 DOI:10.1007/s13346-024-01698-4
.
- S. Rome, Biological properties of plant-derived extracellular vesicles, Food Funct., 2019, 10(2), 529–538 RSC
.
- M. Cao, N. Diao, X. Cai, X. Chen, Y. Xiao, C. Guo, D. Chen and X. Zhang, Plant exosome nanovesicles (PENs): green delivery platforms, Mater. Horiz., 2023, 10(10), 3879–3894 RSC
.
- M. Cong, S. Tan, S. Li, L. Gao, L. Huang, H. G. Zhang and H. Qiao, Technology insight: plant-derived vesicles-How far from the clinical biotherapeutics and therapeutic drug carriers?, Adv. Drug Delivery Rev., 2022, 182, 114108 CrossRef CAS
.
|
This journal is © The Royal Society of Chemistry 2025 |
Click here to see how this site uses Cookies. View our privacy policy here.