DOI:
10.1039/D3SD00317E
(Critical Review)
Sens. Diagn., 2024,
3, 1119-1134
Point-of-care diagnostic devices for periodontitis – current trends and urgent need
Received
1st December 2023
, Accepted 16th May 2024
First published on 3rd June 2024
Abstract
Point of care (POC) diagnostic devices provide a method for rapid accurate identification of disease through analysis of biologically relevant substances. This review focuses on the utility of POC testing for early detection of periodontitis, a critical factor in treating the disease. Accessing the oral cavity for biological sampling is less invasive when compared to other internal test sites, and oral fluids contain biomarkers indicative of periodontitis. The ease of access makes the mouth an excellent target location for the development of POC devices. In this review, accepted standards in industry by which these devices must adhere, provided by the World Health Organization such as REASSURED and CLIA, are discussed. An overview is provided for many periodontal biomarkers currently being investigated as a means of predicting periodontal disease and its progression. POC devices currently being investigated for the identification and monitoring of periodontal disease such as paper-based and lab-on-a-chip based devices are outlined. Limitations of current POC devices on the market are provided and future directions in leveraging biomarkers as an adjunctive method for oral diagnosis along with AI-based analysis systems are discussed. Here, we present the ESSENCE sensor platform, which combines a porous non-planar electrode with enhanced shear flow to achieve unprecedented sensitivity and selectivity. The combination of the ESENCE chip with an automated platform allows us to meet the WHO's ASSURED criteria. This platform promises to be an exciting POC candidate for early detection of periodontitis.
1. Introduction
1.1 Cost and insurance hurdles
Oral health is vital to people of all ages, including children, adults, old age people, and Medicare beneficiaries with disabilities, and is connected to overall health.4 But often, oral health needs to be recognized, and maintenance is challenging due to many factors, including cost and insurance hurdles.6 Medicare's national health insurance program does not cover dental care and routine dental checkups. Almost 65%, nearly 37 million people, do not have dental coverage through Medicare. Consequently, the patient does not visit the dentist (almost 49%). Limited dental insurance, like Medicare beneficiaries using dental services (nearly 19%), leads to a high amount of out-of-pocket costs ($1000) for oral health care.7 Such a high cost makes dentist visits and dental procedures unaffordable. There are options for dental care advance plans like Medicare Advantage, private dental, Medicaid, and employer-sponsored plans. However, such plans are often provided just to the working age, only cover regular dentist visits, and are often subjected to annual caps.8 One must pay out-of-pocket for complicated dental issues. Adults aged more than 60 years require more attention for oral health for an incident; 14% or more adults have untreated caries, and about 68% have periodontitis.9 Most adults over 65 who do not have natural teeth are prone to difficulties such as chewing and swallowing, leading to health complications.9 Untreated caries, periodontitis, and edentulism are subject to high-cost emergency room visits, forcing them to spend more. Hence, attention is required to deal with such challenges and find a way to make dental care more accessible and affordable. The Centers for Medicare and Medicaid Services (CMS) is reviewing a plan to cover oral health, illnesses, and injuries with Medicare.10 Other considerations include a premium-based model like voluntary dental benefits, similar to the Part D drug benefit prescription.11 There is a need to propose better solutions for dental health plans since poor oral care results in complicated health risks. Another approach to reduce cost and make oral diagnosis available to a large population of different age groups would be technological development, accessibility, and affordability. Advances in POC diagnosis systems have recently gained immense attention due to advantages like chairside operation, easy operation, and quick analysis protocols.12
1.2 Summary of oral POC diagnostic device classes
Point of care (POC) diagnostic devices represent a method of clinical monitoring and testing that allows for quick and accurate identification of the medical malady in the office or while the patient is close at hand. POC diagnostic devices can rapidly identify biologically relevant substances like blood glucose levels,13 liver enzymes,14 blood in the stool,15 pregnancy,16 and the presence of drugs in the blood or urine amongst others.17 Though many varieties of POC devices exist, diagnosis of infectious diseases is of great global interest. The World Health Organization's criteria for an effective POC test for such cases are summarized by the acronym ASSURED; the device must be affordable, sensitive, specific, user-friendly, rapid and robust, equipment-free, and deliverable to end users.18,19 This has been updated to account for real-time health monitoring with a revision to the ASSURED criteria, now termed REASSURED, to include real-time connectivity and ease of specimen collection to the initial standards.19 The oral cavity is more easily accessible to health care providers in a less invasive manner than the GI tract, vascular system, or other body parts. Rapid and accurate diagnosis of oral pathologies and their pathogenic basis is beneficial to ensure that the most appropriate treatment is used and the illness can be treated quickly. Fluids accessible and assessable in the mouth, like saliva, mouth rinse, peri-implantitis fluid (PISF), and gingival crevicular fluid (GCF), allow noninvasive testing for notable biomarkers relevant to both specific oral diseases like periodontitis and systemic issues.20
1.2.1. Consumer-targeted POC devices.
Many off-the-shelf POC devices are available over the counter. These products yield easy-to-understand results, allowing patients to monitor their health personally or know when to seek professional care. Perhaps the most common instance of an at-home POC device is the pregnancy test, which reacts to hCG in the urine. Rapid tests for COVID-19 use a similar antibody-based detection system for at-home identification of the illness.
1.2.2. Clinician targeted POC devices.
Various rapid diagnostic tests are performed in clinical or hospital settings. These POC tests meet the WHO ASSURED criteria but are not available for use by the general public. Examples include the rapid antigen streptococcal screen (Strep A test), which uses samples collected by swabbing the tonsils to quickly detect the presence of the causative bacterium.
1.2.3. Insurance targeted POC devices.
Classical diagnostic imaging and testing in the dental and craniofacial space can be costly. For this reason, a cheaper and more efficient alternative may be required as a preliminary screen to gain insurance approval for a secondary and more expensive technique. For example, an in-office X-ray machine may be used first to indicate the need for a more expensive cone beam CT scan.
1.3 Biosensors in the oral cavity
A subset of POC devices used in medicine are biosensors, which generate a measurable signal in proportion to a detected biological reaction or chemical concentration. Every biosensor consists of both static and dynamic elements, which are optimized for increased performance. A biosensor must be selective for the desired analyte, create a reproducible and precise output, have biologically appropriate sensitivity for the minimum and maximum amount of analyte that can be detected, and demonstrate the accuracy of the measured signal; there must be linearity of the measured response.21 There has been considerable advancement in the development of biological sensors over the last decade, especially the rise of electrochemical sensors, as they tend to be cheaper to miniaturize than other spectroscopic or chromatographic detection methods.22 The combination of next-generation sequencing, salivaomics, and biosensors enables the identification of non-cultivable or difficult-to-culture microbes that were previously undetectable using traditional cultivation methods from oral fluids. A critical property most relevant in the case of dental and craniofacial biosensors is the stability of the device. Biosensors are susceptible to ambient disturbances in the area surrounding the device, which can change the precision and accuracy of the system. Long incubation periods or continuous monitoring increases the likelihood of a stability disturbance.21 In the oral cavity, the changes caused by breathing, speaking, mastication, and the introduction of exogenous materials like food, drink, or removable orthodontic systems can cause disturbances that must be accounted for.
Despite this, the National Institute of Dental and Craniofacial Research (NIDCR) is especially interested in funding research into the use of biosensors in the oral cavity as the mouth can be conveniently accessed during office visits.23 The mouth offers a variety of appropriate biosensor attachment surfaces. Hard tissues like the teeth or bone, firm tissues like the gingiva or hard palate, or soft tissues like the cheeks are all possible placements for short or long-term in situ biosensors.24 Additionally, as the mouth is the entry point for both the GI tract and the lungs, biosensors placed within it will be in a location that serves as an entry point for bacteria and viruses, medications, toxins, and nutritional substances, information about which could be used in the diagnosis and management of a variety of health conditions. Some of the methods in development for oral diagnosis by point-of-care devices are seen in Fig. 1.
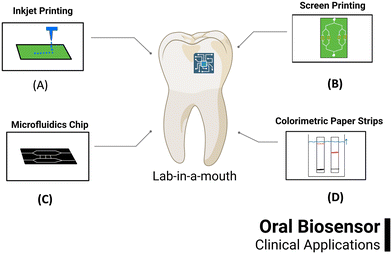 |
| Fig. 1 Emerging methods for oral diagnosis by POC: (A) inkjet printing,1 (B) screen printing,2 (C) microfluidic chip3 and (D) colorimetric paper strips.5 | |
2. Requirements
2.1 CLIA requirements for POC devices (ASSURED compliant)
In addition to the ASSURED criteria mentioned previously, novel POC devices for implementation in the oral cavity must be CLIA-compliant except under specific circumstances. The Clinical Laboratory Improvement Amendments of 1988 (CLIA) regulations are federal standards that must be met in the testing of human specimens (tissue, saliva, blood, etc.) for diagnosis, prevention, or treatment of disease.25 CLIA standards protect human patients from potentially unsafe or unreliable testing. For example, faulty cholesterol tests resulting in high cholesterol being reported as normal can devastate an unsuspecting patient.26 Laboratories can apply for CLIA certification by filling out form CMS-116 online and sending it to their local state agency for the state where the laboratory is located. Interested programs should also check with their state agency for other state-specific requirements to ensure compliance.27
There are also circumstances where a laboratory may be exempt from CLIA testing. Specific tests are CLIA waived because they are determined “to be so simple that there is little risk of error”. These tests include glucose, urine, pregnancy, cholesterol, and fecal occult blood tests.25
2.2 Clinical care requirements for POC devices
Early detection of periodontal disease by clinicians is imperative for effective treatment and prevention of disease progression. A predictive test for periodontitis is needed while still in the treatable stages versus a diagnostic tool to supplement current methods. The conventional clinical index for periodontitis includes bleeding on probing (BOP), probing depth (PD), clinical attachment loss (CAL), and bone loss by X-rays. These clinical markers indicate accumulated damage and severity of periodontal disease. However, periodontal biomarkers are being investigated as an additional method for detecting or predicting periodontal disease activity.28,29 Currently, the only biomarker that has shown utility as a predictive marker of early periodontitis is macrophage inflammatory protein-1 alpha (mip1a).30–33 An increase in macrophage inflammatory protein-1 alpha and monocyte chemoattractant protein-1 with increasing severity of periodontal disease makes effective biomarkers to determine the disease stage.31,33 Additionally, inflammatory markers such as interleukin-1β, interleukin-6, and tumor necrosis factor-α mediate inflammation of gums and tissue injury in periodontitis, making them effective, if not specific, biomarkers for identification of the inflammation associated with periodontal disease.34
For example, gingival crevicular fluid (GCF) or saliva can aid clinicians in determining periodontitis or the severity of periodontitis. For example, matrix metalloproteinases, namely MMP-8 and MMP-9, are significantly elevated in the GCF of periodontitis patients.35–37 They are markers of collagen degradation, a classic symptom of periodontal disease.35 However, it has been shown that it is the active form of MMP-8 (aMMP-8) that is a relevant biomarker for periodontitis and periimplantitis rather than the total or latent proMMP-8.37 The active form of MMP-9, or aMMP-9, is also a useful biomarker for the identification of periodontitis in addition to the latent MMP-9 (IMMP-9).38 Human odontogenic ameloblast-associated protein, found in gingival crevicular fluid, indicates detachment of the junctional epithelium from the tooth surface in periodontitis, making it a possible biomarker for clinical use.39 Cathepsin G is increased in adult periodontitis GCF when compared with healthy controls. This increase reflects the disease process in nearby inflamed gingiva and improved response to microbiota or dental plaque in periodontitis lesions.40 In addition, calprotectin levels in gingival crevicular fluid and plasma are elevated in patients with periodontitis compared to healthy controls.41,42
Neutrophil elastase (NE), usually localized within the cytoplasm of neutrophils, is increased in the saliva of periodontitis patients and can also be used as a biomarker for disease progression.43 The mechanism by which NE is released and its role in disease is currently unclear; future studies on this mechanism may further elucidate the role of NE as a biomarker for periodontitis. Several Gram-negative bacteria are often implicated in periodontal disease, which can provide evidence of disease. Depending on the patient, the relevant species can include P. gingivalis, T. denticola, P. intermedia, and A. actinomycetemcomitans.44–47 Specifically, the gingipain proteases of P. gingivalis were shown to be a promising biomarker for periodontal disease.48 Other biomarkers include the presence of pathogen-associated molecular patterns (PAMPs) like lipopolysaccharides (LPSs) that are present in all Gram-negative bacteria.49,50 With further testing and development of identification methods, clinicians can use these biomarkers for early detection of periodontal disease, augmenting current treatment and prevention options.
3. State-of-the-art oral diagnostics
3.1 Clinical practice
Periodontitis is considered one of the most chronic oral diseases. The onset of periodontitis leads to deep pockets between the tooth surface and the gingival epithelium. In clinical practice, probing pocket depth (PPD) is the most widely used method for assessing corono-apical extension and pocket depth.51 Williams and CPITN probes traditionally used in clinical settings have complications affecting results, including probing pressure and angulation, the probe material, shape and size, and reference deposition.52 Advancements in state-of-the-art technology like the Vivacre TPS probe offer advantages like computer-linked pressure-sensitive electronic or manual probes to measure the pocket depth, tooth mobility, and attachment level with precise control and high reproducibility.53
Radiography is another standard method used in clinical practice, essential to display the change of the architecture and the position of alveoli in a periodontal examination. Traditionally, radiography can reveal bone destruction but fails to articulate the degree of destruction for detecting bone demineralization. Additionally, the superposition of images can also distort the accuracy of the results. Panoramic radiography is advantageous for forming a complete planar image of the dentition. However, it fails to provide a complete 3D structure or visualize subtle alveolar changes.54 Cone-beam computed tomography systems (CBCT), called dental CT, have advanced traditional diagnostic imaging methods. CBCT is used to examine the depth of alveolar bone for implants with a relatively low radiation dose, and it reflects the actual size of the alveolar osseous without overlap or distortion.55 Despite advances in clinical practices for oral diagnosis, there is a need for new technologies to provide data on the real-time progress of diseases. Table 1 provides details of clinical methods with advantages and disadvantages.
Table 1 Current clinical methods used by clinicians for analysis and diagnosis of periodontal disease
Clinical methods |
Clinical relevance |
Advantages |
Disadvantages |
Ref |
Periodontal probing |
Degree of gingival bleeding, the extent of soft-tissue destruction by pocket probing depth analysis, attachment level |
Easy to perform by the dentist in the hospital or clinical setting |
Difficult to operate on-site for analysis |
56–59
|
Radiography |
Type and extent of alveolar bone loss and bone-tissue destruction analysis |
One of the best methods in a clinical setting |
It is high cost and requires a technician |
54 and 60 |
Inspection |
Gingival index changes analyzing the color, shape, and degree of recession |
It does not require complicated instruments |
Prone to human error in analysis |
61 and 62 |
Percussion |
Determines the status of the periodontal ligament |
It does not require complicated instruments |
Indirect test |
63–65
|
Palpation |
Tooth mobility changes and texture of the gingiva |
It does not require complicated instruments |
Requires technical training to perform the analysis |
66 and 67 |
3.2 Research and development
The NIDCR invests in salivary diagnostic research because saliva contains many of the same biomarkers as blood while being more accessible to collect. This allows for quick, low-cost, and non-invasive testing that can be repeated easily to monitor health conditions over time. Salivary diagnostic research has the potential to identify biomarkers associated with oral cancers, anti-HIV antibodies, and many more.68
Quick detection of biomarkers associated with periodontitis is important, as early symptoms of the disease may be easily missed or overlooked by the patient. Early diagnosis is valuable as treatment after detection can prevent periodontitis from becoming so severe that vital supporting tooth structures are permanently lost. Monitoring the progression of periodontal disease over time is also essential for dentists to stay up to date with the patient's health. Many of the tests required for this level of disease monitoring require either specialized and bulky equipment or that the patient samples must be sent away for testing, meaning results take longer to acquire. Many existing methods used in clinical studies for detecting these critical salivary biomarkers rely on ELISA or immunofluorescence assays, which are not possible to perform chairside while also using expensive equipment and large volumes of reagents.69 Current point of care (POC) systems being investigated to identify and monitor periodontal disease are outlined in the following section and Table 2.
Table 2 Current point-of-care (POC) systems being investigated for use in the identification and monitoring of periodontal disease subdivided by category, including point-of-care tests (POCTs), paper-based POCT, and lab-on-a-chip (LOC)
Classification |
Sample |
Detection principle |
Biomarker/target analyte |
Limit of detection |
Ref. |
POCT |
Saliva |
Surface acoustic waves |
MMP-8 |
62.5 ng ml−1 |
80
|
Saliva |
Surface plasmon resonance (SPR) |
MMP-9 |
8 pg mL−1 |
81
|
Commercial saliva & gingival crevicular fluid (GCF) |
Colorimetric |
Human neutrophil elastase & cathepsin-G |
1 pg mL−1 |
82
|
Saliva |
Fluorescence resonance energy transfer (FRET) and SPR |
Odontogenic ameloblast-associated protein |
1.63 nM |
83
|
|
|
|
|
84
|
Saliva & GCF |
Visual detection |
Gingipain protease |
49 CFU mL−1 |
48
|
(Porphyromonas gingivali) |
Saliva |
Immunoassay |
IL-1β |
28 pg ml−1 |
85
|
IL-6 |
5.5 pg ml−1 |
|
MIP -1α |
5 pg ml−1 |
|
MMP-8 |
165.9 ng ml−1 |
|
Saliva |
Electrochemical |
Human odontogenic ameloblast-associated protein (ODAM) |
1 nM |
79
|
|
Saliva |
Surface plasmon resonance (SPR) |
MMP-8 |
225 pM |
86
|
Paper-based POCT |
Subgingival plaque |
Immunochromatography |
40 k-outer membrane protein of P. gingivalis |
5 copies per mL |
87
|
Saliva |
Immunochromatography |
Monoclonal antibodies of P. gingivalis |
105 cells per ml |
88
|
GCF |
Immunochromatography |
MMP-8 |
1 mg L−1 |
89
|
Saliva |
Colorimetric |
Nitrite |
10 μM L−1 |
90
|
Saliva |
Immunochromatography |
MMP-8 |
25 ng ml−1 |
91
|
MMP-9 |
100 ng ml−1 |
Saliva |
Proteolytic activity |
Cathepsin-G |
100 fg ml−1 |
92
|
(Visual detection) |
Neutrophil elastase |
1 pg ml−1 |
Saliva |
Proteolytic activity |
Gingipains of P. gingivalis |
49 CFU per ml |
93
|
(Visual detection) |
|
Mouth rinse |
Immunochromatography |
aMMP-8 |
25 ng ml−1 |
94
|
|
GCF |
Disk-like lateral flow immunoassay |
MMP-8 |
5.455 ng ml−1 |
28
|
IL-1β |
0.054 ng ml−1 |
TNF-α |
4.439 ng ml−1 |
|
Gingival crevicular fluid |
Isothermal recombinase polymerase amplification + lateral flow strips |
P. gingivalis
|
9.27 CFU per reaction |
75
|
LOC |
GCF |
Current continuous flow polymerase chain reaction (CF-PCR) |
Treponema denticola
|
125 CFU per μl |
77
|
GCF |
Sandwich enzyme-linked immunosorbent assay |
Calprotectin |
1.56 ng ml−1 |
95
|
Bacterial culture |
PCR |
P. intermedia
|
2000 copies per reaction |
96
|
|
A. actinomycetemcomitans
|
10 copies pe reaction |
|
P. gingivalis
|
10 copies per reaction |
|
T. denticola
|
20 000 copies per reaction |
Electrochemical sensing |
Saliva |
Electrochemical |
Salivary peroxidase |
0.5 ng ml−1 |
97
|
Artificial saliva |
Electrochemical |
Antimicrobial peptides (magainin I) |
102 CFU per mL |
84
|
(Streptococcus sanguinis) |
Saliva |
Electrochemical |
IL-1β |
0.014 ng ml−1 |
78
|
|
MMP-8 |
0.13 ng ml−1 |
|
Saliva |
Electrochemical |
Human odontogenic ameloblast-associated protein (ODAM) |
1 nM |
79
|
3.2.1. POCT – point of care testing.
A variety of point-of-care tests (POCTs) for use in the diagnosis and monitoring of periodontitis have been explored using several detection principles, including surface plasmon resonance (SPR), surface acoustic waves, colorimetric methods, and fluorescence resonance energy transfer (FRET). Other devices include the use of novel molecularly imprinted polymers (MIPs) for on-site detection of oral diseases.70 Wearable mouthguards have played a crucial role in the diagnosis and monitoring of oral health. They enable the assessment of various biochemical parameters, such as monitoring saliva turbidity to assess oral hygiene and conducting saliva glucose monitoring, among other functions, providing real-time data.71 Some of the most recent advances in the area use electrochemical sensors to make portable and rapid devices for the chairside detection of biomarkers associated with periodontal disease. Using these electrochemical sensors allows for higher sensitivity and selectivity in initial diagnosis, aiding in the reduction of false positives and negatives and allowing for more accurate and precise POCT systems for the detection of periodontal disease progression. In addition, the use of paper-based POCT systems allows for clinicians to receive diagnostic results easily and accurately when compared to current diagnostic methods.
3.2.1.1. Paper-based POCT – paper-based point of care testing.
Many paper-based POCTs are being developed as the paper-based test strip requires even less equipment when used chairside.72 Detection methods are more limited when the POCT is paper-based, typically relying on immunochromatography or colorimetric readouts, which are detected visually, making them less quantifiable than electrochemical results. Although the results from paper-based POCT systems may have less sensitivity than electrochemical POCT or other methods when used chairside, they give dentists and physicians a reliable, cost-effective, simple, and time-efficient approach to the diagnosis and disease tracking of periodontitis.73 A workflow for conventional paper-based POCT used in detecting and measuring periodontitis biomarkers is seen in Fig. 2 based on the work done by He et al.28
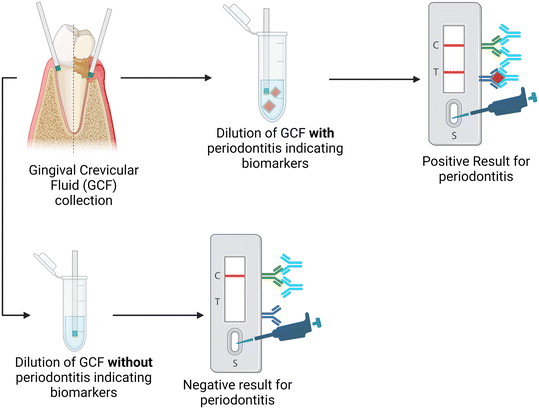 |
| Fig. 2 Schematic for the process of the qualitative paper-based lateral flow immunoassay for diagnosis of periodontal disease based on the presence of multiple biomarkers. | |
The work by He et al. used a paper-based POCT to detect periodontitis by developing a disk-like lateral flow immunoassay to simultaneously detect three different biomarkers.28 The luminescence probe was able to detect MMP-8, IL-1β, and TNF-α using gingival crevicular fluid in a 30-minute timeframe. The results from this paper-based device also showed high correlation rates compared to standard clinical results. This indicates that the paper-based POCT device shows promise as an accurate and timely way to diagnose and check the progression of periodontitis based on multiple biomarkers.28 Using GCF rather than saliva helps to indicate the condition of specific teeth instead of the entire mouth.74
Paper-based lateral flow strips (LFSs) can also detect the presence of specific bacteria associated with periodontal disease. Ge et al. used isothermal recombinase polymerase amplification (RPA) for nucleic acid amplification paired with a nanoparticle-based lateral flow strip to allow visualization of the results. Porphyromonas gingivalis, a bacterial species most closely associated with severe periodontal disease, was detected using species-specific 16S rRNA as the RPA target. Gingival crevicular fluid was used as the analyte, and the LFA test accurately determined the presence of P. gingivalis, having results consistent with the more involved and expensive PCR assay used conventionally.75
3.2.1.2. LOC – lab-on-a-chip.
With many laboratory testing methods requiring extensive and expensive equipment to analyze biological samples, there is a push to miniaturize testing infrastructure and create lab-on-a-chip (LOC) technologies. LOC systems reduce the amount of analyte needed and aim to have high throughput, with rapid analysis time, while being portable and automated.
In diagnosing and monitoring the state of periodontal disease, identifying oral pathogens responsible for the destruction of periodontal tissue may be beneficial.76 Identifying the pathogens by culturing the patient samples is typically time-consuming, and a clinical office must be equipped for such a process. Identifying the samples using PCR is a more realistic alternative. Still, the equipment needed for every step in the continuous flow polymerase chain reaction (CF-PCR) process would only be found in laboratories. In addition, many versions of PCR used today have issues concerning long run times, machine size, false positives, etc. These issues make PCR a process typically performed in laboratories, preventing its use in POC systems. Li et al. successfully created a LOC capable of CF-PCR for identifying key periodontal pathogens: Porphyromonas gingivalis, Treponema denticola, and Tannerella forsythia. To generate a LOC for PCR, this group incorporated automatic sample injection, which removes the need for a more costly syringe pump. This device also achieved rapid DNA amplification and detected the PCR product in sequence without the need for external technology for final confirmation of the presence of the pathogenic bacteria. This LOC all-in-one system lowered the cost of such systems, a significant improvement necessary for implementing such a system in the field today.77
3.2.1.3. Electrochemical sensing methods.
Currently, the electrochemical sensing methods for periodontal disease markers are large and costly and cannot be performed chairside and in a cost-efficient manner. Zhang et al. have created a small device for the electrochemical sensing of multiple periodontal disease biomarkers. In addition, it has been found that miniaturization of the device made by Joe et al. would be valuable to the POCT market. A POCT workflow and mechanism for detecting and measuring periodontitis biomarkers based on the devices made by Zhang et al. and Joe et al. are seen in Fig. 3.78,79
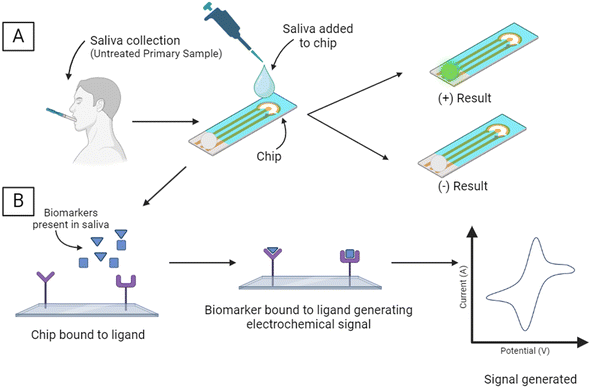 |
| Fig. 3 Schematic for the electrochemical biomarker sensing process for the detection of common periodontal biomarkers. [A] Saliva is collected from the patient and added to the device/chip which will yield either a positive or negative result depending on the presence of the biomarker. [B] An electrical signal is generated by the binding of the biomarker to the ligand. Quantification of the biomarkers can be interpreted using the intensity of the generated signal. | |
Zhang et al. have developed a dual-channel electrochemical immunosensor capable of detecting and measuring the presence of both IL-1β and MMP-8 in saliva as a POCT to aid in the rapid and accurate diagnosis of periodontitis.78 The simultaneous determination of the levels of these biomarkers synchronously allows for higher accuracy in diagnosing the current stage of periodontitis.78 Tested against both spiked artificial saliva and clinical samples, the device has high sensitivity and a wide linear range using IrOx/Ti3C2Tx nanocomposites. The use of an electrochemical immunosensor allows for the detection process to be more cost-effective, timely, and more accessible to perform than currently used techniques such as immunofluorescence and enzyme-linked immunosorbent assays. Both techniques, while accurate, are costly and require large machinery, which is not practical for chairside diagnosis and monitoring of periodontal disease. The electrochemical sensor produced by Zhang et al. shows promise in detecting the presence of the biomarkers mentioned above while being practical for chairside use.78
Another electrochemical sensor for the detection of periodontal diseases was developed by Joe et al.79 This POCT was first validated by the detection of known protein biomarkers for other diseases (cardiovascular diseases and type 2 diabetes), and it was then modified for use in an aptamer-based sandwich-type electrochemical biosensor capable of detecting human odontogenic ameloblast-associated protein (ODAM) in saliva. The binding of the target biomarker to the aptamer creates a redox reaction in the bottom plate of the electrochemical sensor which then attracts a secondary aptamer, producing the resulting positive signal. Miniaturization of the detection equipment is vital in ensuring that the device is usable at the chairside and replacing larger equipment that takes up valuable clinical benchtop space.79
4. Gaps in the field
4.1 Microbiological profile
The oral microbiome consists of around 1000 species of microorganisms, including bacteria (Phyla – Actinobacteria, Firmicutes, Proteobacteria, Fusobacteria, and Spirochetes), fungi (Candida, Saccharomyces, Aspergillus, Cladosporium, Fusarium and Cryptococcus) and protozoa (Entamoeba gingivalis and Trichomonas tenax).98 The oral microbiome also accommodates protein signals, the extracellular matrix, enzymatic products, and molecular communications, creating mucosal barriers in the mouth to resist pathogenic invaders.99 Viruses, mainly bacteriophages, are found to be associated with the oral microbiome, acting as commensals and pathogens. The bacteriophages release lytic enzymes, altering the composition of the oral microbiome.100 The presence of Aggregatibacter actinomycetemcomitans was found to be associated with localized aggressive periodontitis.101 Extending to the COVID-19 pandemic, viruses could be present in the salivary glands, resulting in asymptomatic carriers.102 The oral surface cavity consists of teeth, keratinized epithelium, and nonkeratinized epithelium, making up to 20%, 50%, and 30% of the total surface area, and the mouth cavity consists of the tongue, inner cheeks, and hard and soft palates. Each surface has microbiota and microenvironments, including saliva and gingival cervices.103 Disruption of relative proportions of the oral microbiome and the host cells can lead to dysbiosis, which is periodontal disease. Factors causing dysbiosis include diseases such as diabetes, genetic differences, innate/adaptive immune factors, oral hygiene, diet, smoking, the activity of salivary proteins, smoking, and antibiotics/antimicrobial agents.104 Some of the key pathogens associated with periodontitis are classified in Table 3 with different color schemes based on the study by Socransky and Haffajee.105,106 According to the color scheme, the purple, yellow, and green colors are associated with the bacteria corresponding to periodontal health, and bacteria in orange, red, and other groups are associated with suspected periodontopathogens.105
Table 3 Socransky color code complexes105
Bacteria species |
Color complex |
Pathogenesis |
Actinomyces
|
Purple |
Healthy |
Veillonella
|
Streptococcus: gordonii, intermedius, mitis, sanguis
|
Yellow |
Healthy |
Capnocytophaga, E. corrodens
|
Green |
Healthy |
Campylobacter rectus
|
Orange |
Pathogenic |
Fusobacterium nucleatum
|
P. micros
|
P. intermedia
|
T. forsythia
|
Red |
Pathogenic |
P. gingivalis
|
T. denticola
|
A. actinomycetemcomitans
|
Not grouped |
Pathogenic |
Selenomonas
|
Non-oral bacteria such as Gram-negative enteric rods, enterococci, and staphylococci are non-resident to the oral microbiome and lead to pathogenesis in mostly non-healthy patients.107 The route of introduction could be from the mouth by water, food, chewing items, and many more. Non-oral microbes such as Acinetobacter baumannii and Pseudomonas aeruginosa are reported as the most pathogenic species causing symptoms leading to periodontitis like periodontal attachment loss.108,109 Since the oral cavity habitats have diverse microenvironments comprising highly aerated aerobic microbes and sheltered anaerobic microbes, they represent one of the most complex microbial communities in the human body and are an essential site for diagnosis. With the advance in molecular and point-of-care techniques, oral sampling and analysis are key to diagnosis.110 Mitsakakis et al. developed a centrifugal microfluidic-based fully automated chair-side POC tool for diagnosing antibiotic-resistant species for oral infections.111 Other non-culturing tools explored for the rapid diagnosis of oral microbiome include droplet microfluidics.112 However, despite culturing and non-culturing techniques developed for the detection of the oral microbiome, there is high potential in the development of technology establishing rapid chairside detection tactics.
4.2 Cost effectiveness
The adoption of point-of-care (POC) technology in the diagnosis of periodontal disease may depend on its cost effectiveness. Existing POC tests for periodontitis like Periosafe, an aMMP-8 point-of-care diagnostic test, have shown improved efficacy in diagnosis of subclinical periodontal disease compared to the conventional bleeding-on-probing assessments.113 For the patient, the greatest costs associated with periodontitis occur in the later stages of the disease.114 Early identification and treatment result in more predictable treatment outcomes for patients.115 A proactive approach, meaning early identification of periodontitis, will result in more savings in the long run.116 However, the cost benefits of early identification by POC technology are only realized when the identification is followed by treatment to prevent the expensive later stages of the disease from occurring.114 Ultimately, diagnosis alone may not translate into cost effectiveness for patients. For example, analysis of the cost effectiveness of AI screening tools in medicine have shown that while diagnosis by AI may be accurate, its use may not result in cheaper care for the patient.117
4.3 AI advances
Artificial intelligence (AI) could be defined as machines reducing human efforts and performing human tasks. Machine learning (ML) is a subpart of AI that learns different statistical patterns and predicts unseen data. Both AI and ML have been explored for dental theranostics. Some of the significant advantages of AI include (1) heterogeneous data being generated in dentistry, and AI allows the integration of all the data domains, for example, clinical data, dental history, and biomolecular data, allowing interaction and integration of all the data sets in one place.118 (2) Image analysis is routinely carried out in dentistry. AI could drive the analysis of such images by reducing variability in the subjective individual examination, making the entire process error-free and effective, and lowering the entire theranostics costs.119 (3) AI allows experimentation with the data sets, which is impossible to perform in real-life settings.120 (4) AI could be utilized for record keeping, leveraging speech, voice, and text recognition elements, reducing space and time for data records.121 (5) Patients could directly use AI as a promising platform to make the entire theranostic process personalized by collecting data from wearable devices or POC devices. (6) AI could overcome on–off-medicine, where patients barely visit doctors in a real-life setting due to many factors like high out-of-pocket costs, which could be minimized by continuous non-invasive monitoring.122 Periodontitis constitutes a significant cause of tooth loss which is called periodontally compromised teeth (PCT).123 Despite advancements in the diagnosis and treatment of PCT, real-time prediction of the stage of PCT is not yet possible.124 Lee et al. used AI and deep learning to evaluate the prediction and diagnosis of PCT. The deep learning approach accurately determined the need for tooth extraction at 73.4–82.8%.125 Though AI has numerous advantages, some disadvantages include the possibility of bias and data security breaches, transparency of the AI algorithm, and sometimes higher costs of the AI software.126 A mixed approach is needed in the future, where AI could be integrated from multiple data sets from dentistry to make it accessible to a large population.
5. Portable platform
5.1 ASSURE criteria
POC devices in the market suffer from two significant limitations: 1) false-negative test due to the low number of species of interest present in the sample matrix degrading sensitivity. 2) The sample matrix may contain other molecules similar in functionality to the target molecules, contaminating the sample and affecting selectivity. Additionally, in the case of POC diagnostic devices, the design must facilitate rapid analysis without using expensive or bulky equipment. Sensitivity could be increased by additional, costly steps such as amplifying the analyte, concentrating the analyte, and using exogenous labels in combination with optical or electrochemical systems to amplify the detection signals. Selectivity could be mitigated by additional steps such as extensive purification steps like passing through different resins and isolating the species of interest for diagnosis. Multiplexing is challenging in POC; the current method requires various sensors/protocols to detect other classes of molecules. The current state-of-the-art methods like ELISA (enzyme-linked immunosorbent assay) and paper-based assays are plugged with bulky and expensive instruments or high-cost additional optical image analysis systems, making them relatively costly. Thus, the state-of-the-art sensors often do not meet the “ASSURED” criteria for POC diagnostics (Table 4).
Table 4 The WHO criteria for POC devices and the ESSENCE device
WHO criteria18,19 |
ESSENCE meeting the WHO criteria127 |
A |
Affordable |
Channels from double-sided tape, low sample volume ∼80 μl, cheap electrode material |
S |
Sensitive, no false negatives |
Ionic flux confined to nanodomains -> removal of parasitic electrochemical signals |
S |
Specific, no false positives |
Shear-enhanced flow -> removal of non-specific adsorption, biofouling |
U |
User-friendly |
No user inputs, automated fluidics, automated signal collection and analysis |
R |
Rapid |
Detection in 35 minutes |
E |
Equipment-free |
Portable, cheap spectroscopic station |
D |
Delivered |
Regenerative and robust sensor |
5.2 ESSENCE platform
ESSENCE is an electrochemical sensing method that uses a shear-enhanced, flow-through nanoporous capacitive electrode microfluidic analysis system developed by Sagnik et al. The ESSENCE chip consists of a flow-through sampling channel sandwiched between two interdigitated microelectrodes (10 μm wide × 500 μm long) (Fig. 4).
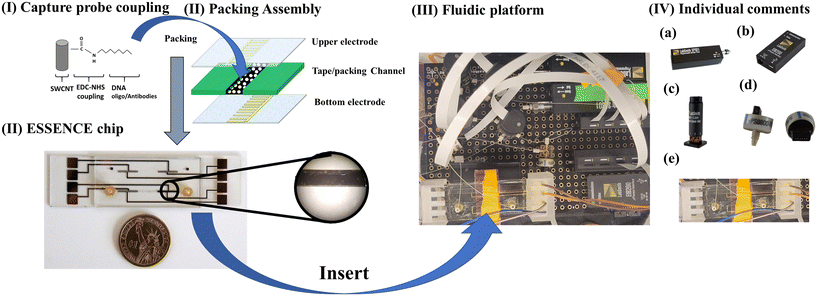 |
| Fig. 4 The procedural flowchart encompassing key steps: (I) surface modification of single-walled carbon nanotubes (SWCNTs) via an EDC-NHS coupling reaction with an ssDNA probe. These modified SWCNTs are combined with 1X PBS as the packing solution and assembled into the ESSENCE microfluidic chip. (II) A closer look at the ESSENCE chip structure reveals the packed solution electrode on the right-hand side. The microfluidic channel traverses between two electrodes, with the left-hand side electrode as a counter electrode devoid of any packed SWCNT material. A microscopy image highlights the uniform packing on the ESSENCE chip after the packing solution has evaporated from the channel. (III) Integration of the ESSENCE chip into the fluidic platform for automated control. (IV) The components of the fluidic platform can be itemized as follows: (a) a syringe pump that furnishes pressure-driven force within the system, (b) controlling units connected to a laptop for system management, (c) a valve system responsible for regulating sample and buffer solutions, as well as overseeing the overall cleaning process, (d) pressure sensors that provide crucial information about the fluidic conditions, and (e) an installation socket designed to accommodate the ESSENCE chip. | |
The channel is filled with a carbon-based transducer material (CBTM) equipped with a molecular capture agent (antibody, DNA aptamer) specific to the bio-target. When a fluid with the target of interest flows through the cell, the microelectrode signal is attenuated. The CBTM acts as a transducer in the electrochemical cell when a binding event occurs at the CBTM surface. The bio-target is detected using electrochemical impedance spectroscopy (EIS). ESSENCE meets the ASSURED criteria set by the WHO (Table 4).
Most peer-reviewed scientific research on detecting periodontitis focuses on fundamental studies, such as biochemical or surface metrology modifications,128,129 aimed at enhancing or synthesizing highly selective and sensitive probe materials. These studies provide several strategies to improve electrochemical signals. On the other hand, ESSENCE offers several notable advantages to support the current generation of electrochemical biosensor studies. The platform primarily focuses on enhancing adaptation technologies, leveraging physical advantages to enhance electrochemical signals. This is achieved by transitioning from the traditional planar electrode interface to a 3-dimensional electrode structure. This modification allows the system to be easily adapted to many current probe studies, offering several benefits through this simple yet crucial tweak. First, its electrode nanoporosity formed by probe materials effectively generates significant shear forces akin to hydrogen bond strength, greatly enhancing selectivity by reducing non-specific adsorption. Secondly, the interdigitated, nanoporous microelectrode design leads to a high signal-to-noise ratio (SNR) achieved through heightened sensitivity and specificity. The nanostructured electrodes confine the ionic flux to nanodomains, resulting in greater sensitivity and an amplified signal, thanks to nanoconfinement effects. Thirdly, the nanoporous electrode architecture promotes the convective transport of the target analyte to the sensing element, overcoming diffusion limitations and reducing assay times. Furthermore, the enhanced convection-mediated transport minimizes signal artifacts, such as parasitic double-layer capacitance, thereby facilitating rapid, high-resolution characterization of binding signals with significantly reduced noise. Lastly, the modularity of ESSENCE enables precise control of shear force through the flow rate, allowing for independent sensitivity and device selectivity adjustment. This decoupling approach helps mitigate issues like biofouling and false positives, which are common challenges in existing technologies, thereby increasing overall efficiency. The flexibility of ESSENCE allows for the utilization of various analytes (e.g., blood, urine, serum) with any capture moiety (aptamer, antibody, oligo) targeting a wide range of molecules (prion, mRNA, DNA, or antigen) for diagnosing oral diseases.
In the case of DNA detection, by only using the most straightforward EDAC reaction on a functionalized carbon nanotube surface, the protocol can be reduced to below 15 minutes, including the priming, washing, and rinsing sub-processes, and still reaches fM LOD. These processes utilize microfluidic technologies to manipulate the fluidic algorithm to increase sensitivity and selectivity for an electrochemical sensing process. LabVIEW/python codes control all the procedures on the ESSENCE platform. These include analyte loading, post-washing, EIS analyzer measuring, and buffer washing procedures. Thus, the ESSENCE platform is a fully automatic “one-stop” sensor platform. It significantly reduces the unreliability of human error and removes the requirements of technician training, making it user-friendly.
6. Conclusions and future directions
Future developments in POC-based oral diagnostics will pave the way to new technologies like microfluidics, paper-based analytical devices, the ESSENCE platform, and many more. However, reaching practitioners and convincing them to use new technologies is still challenging compared to their daily clinical practices. Salivary-based biomarkers are highly used for specific and sensitive detection of periodontitis and other oral diseases. However, since there is a need for universal methods for sample collection, like saliva, this leads to variations in the analysis. This must be fixed before incorporating current POC devices in clinical settings. Biomarkers can be used as an adjunctive method for oral diagnosis along with standard clinical practices. Additionally, biomarker-based tests could be combined with an AI-based analysis system to interpret the results efficiently, reducing human-based error.
Data availability
All data created or analyzed in this study are available on request from the corresponding author.
Conflicts of interest
There are no conflicts to declare.
Acknowledgements
V. A. K. acknowledges support from the NIH NIDCR R01DE031812, NIH NIAMS R21AR079708, NIH NIAMS R01AR080895, NIH NIDCR R01DE029321, NIH NCATS UL1TR003017, and the Undergraduate Research and Innovation program at NJIT.
References
-
R. K. Sokhi, N. Monga, K. K. A. Almerco, D. Shastri, M. Bajracharya and U. Pyakurel, et al., Commonly Used 3D Printing Technologies in Oral Health Science, 3D Printing in Oral Health Science: Applications and Future Directions, Springer, 2022. pp. 47–67 Search PubMed.
-
P. Gulati, K. Pasbola, P. Pandey, R. Sharma, A. Thakkar and P. R. Solanki, Electrochemical immunosensors fabrication for oral cancer detection in patient samples based on carbon based screen printed electrode, SPAST Abstracts, 2021, 1(01) Search PubMed.
- A. E. Herr, A. V. Hatch, W. V. Giannobile, D. J. Throckmorton, H. M. Tran and J. S. Brennan,
et al., Integrated microfluidic platform for oral diagnostics, Ann. N. Y. Acad. Sci., 2007, 1098(1), 362–374 CrossRef CAS PubMed.
- J. L. Fellows, K. A. Atchison, J. Chaffin, E. M. Chávez and N. Tinanoff, Oral Health in America: Implications for dental practice, J. Am. Dent. Assoc., JADA, 2022, 153(7), 601–609 CrossRef PubMed.
- L. Helldén, P. B. Robertson, D. Rosania and J. Hock, Evaluation of a colorimetric assay as a measure of gingival inflammation, J. Periodontol., 1980, 51(5), 279–282 CrossRef PubMed.
- K. L. Sisson, Theoretical explanations for social inequalities in oral health, Community Dent Oral Epidemiol., 2007, 35(2), 81–88 CrossRef PubMed.
- H. Luo, M. E. Moss, R. Basu and F. T. Grant, Rural–Urban Differences in Use of Dental Services and Procedures Among Medicare Beneficiaries in 2018, Public Health Rep., 2022, 00333549221128336 Search PubMed.
- P. F. Cooper and R. J. Manski, Enrollment trends in employer-sponsored health plans that include dental benefits, J. Am. Dent. Assoc., JADA, 2021, 152(5), 377–384 CrossRef PubMed.
- R. López, P. C. Smith, G. Göstemeyer and F. Schwendicke, Ageing, dental caries and periodontal diseases, J. Clin. Periodontol., 2017, 44, S145–S152 CrossRef PubMed.
- J. J. Crall, Oral health policy development since the Surgeon General's Report on Oral Health, Acad. Pediatr., 2009, 9(6), 476–482 CrossRef PubMed.
- B. Tiner, J. D. Johnson, P. J. Schwartz, R. S. Clark, V. L. Nannini and D. M. Shafer,
et al., Oral health benefits in Medicare and Medicaid, J. Oral Maxillofac. Surg., 2021, 79(12), 2386–2388 CrossRef CAS PubMed.
- K. Muthu and M. Narayanan, Role of Saliva in Point of Care Technology, Oral & Maxillofacial Pathology Journal, 2023, 14(1), 81–84 Search PubMed.
- N. K. Tran, C. LaValley, B. Bagley and J. Rodrigo, Point of care blood glucose devices in the hospital setting, Crit. Rev. Clin. Lab. Sci., 2023, 60(4), 290–299 CrossRef CAS PubMed.
- X. Liu, X. Mei, J. Yang and Y. Li, Hydrogel-involved colorimetric platforms based on layered double oxide nanozymes for point-of-care detection of liver-related biomarkers, ACS Appl. Mater. Interfaces, 2022, 14(5), 6985–6993 CrossRef CAS PubMed.
- W. Hsin-Yao, L. Ting-Wei, S. Y.-H. Chiu, L. Wan-Ying, H. Song-Bin and J. C.-H. Hsieh,
et al., Novel Toilet Paper–Based Point-Of-Care Test for the Rapid Detection of Fecal Occult Blood: Instrument Validation Study, J. Med. Internet Res., 2020, 22(8), e20261 CrossRef PubMed.
- M. Rahbar, S. Zou, M. Baharfar and G. Liu, A customized microfluidic paper-based platform for colorimetric immunosensing: demonstrated via hCG assay for pregnancy test, Biosensors, 2021, 11(12), 474 CrossRef CAS PubMed.
- M. Dagar, S. Yadav, V. Sai, J. Satija and H. Bhatia, Emerging trends in point-of-care sensors for illicit drugs analysis, Talanta, 2022, 238, 123048 CrossRef CAS PubMed.
- M. Naseri, Z. M. Ziora, G. P. Simon and W. Batchelor, ASSURED-compliant point-of-care diagnostics for the detection of human viral infections, Rev. Med. Virol., 2022, 32(2), e2263 CrossRef CAS.
- J. A. Otoo and T. S. Schlappi, REASSURED Multiplex Diagnostics: A Critical Review and Forecast, Biosensors, 2022, 12(2), 124 CrossRef CAS PubMed , PubMed PMID: 35200384. Pubmed Central PMCID: PMC8869588. Epub 20220216. eng.
- S. Alassiri, P. Parnanen, N. Rathnayake, G. Johannsen, A. M. Heikkinen and R. Lazzara,
et al., The Ability of Quantitative, Specific, and Sensitive Point-of-Care/Chair-Side Oral Fluid Immunotests for aMMP-8 to Detect Periodontal and Peri-Implant Diseases, Dis. Markers, 2018, 2018, 1306396 Search PubMed , PubMed PMID: 30154936. Pubmed Central PMCID: PMC6098860. Epub 20180805. eng.
- N. Bhalla, P. Jolly, N. Formisano and P. Estrela, Introduction to biosensors, Essays Biochem., 2016, 60(1), 1–8 CrossRef PubMed , PubMed PMID: 27365030. Pubmed Central PMCID: PMC4986445. eng.
- C. Zhu, G. Yang, H. Li, D. Du and Y. Lin, Electrochemical Sensors and Biosensors Based on Nanomaterials and Nanostructures, Anal. Chem., 2015, 87(1), 230–249 CrossRef CAS PubMed.
- Biosensors in the Oral Cavity https://www.nidcr.nih.gov: National Institute of Dental and Craniofacial Research, 2018 [updated July 2018]. Available from: https://www.nidcr.nih.gov/grants-funding/funding-priorities/future-research-initiatives/biosensors-in-the-oral-cavity.
- M. S. Mannoor, H. Tao, J. D. Clayton, A. Sengupta, D. L. Kaplan and R. R. Naik,
et al., Graphene-based wireless bacteria detection on tooth enamel, Nat. Commun., 2012, 3(1), 763 CrossRef PubMed.
- Questions and Answers for On Site Visits of CLIA Certificate Of Waiver Laboratories https://cms.gov: Centers for Medicare & Medicaid Services; [cited 2023]. Available from: https://www.cms.gov/Regulations-and-Guidance/Legislation/CLIA/downloads/cliaback.pdf.
- D. Surabattula, C. M. Harvey, F. Aghazadeh, J. Rood and A. Darisipudi, Usability of home cholesterol test kits and how their results impact patients' decisions, Int. J. Ind. Ergon., 2009, 39(1), 167–173 CrossRef.
- How to obtain a CLIA Certificate https://cms.gov: Centers for Medicare & Medicaid Services, [cited 2023]. Available from: https://www.cms.gov/regulations-and-guidance/legislation/clia/downloads/howobtaincliacertificate.pdf.
- W. He, M. You, Z. Li, L. Cao, F. Xu and F. Li,
et al., Upconversion nanoparticles-based lateral flow immunoassay for point-of-care diagnosis of periodontitis, Sens. Actuators, B, 2021, 334, 129673 CrossRef CAS.
- M. S. Tonetti, H. Greenwell and K. S. Kornman, Staging and grading of periodontitis: Framework and proposal of a new classification and case definition, J. Periodontol., 2018, 89(S1), S159–S172 CrossRef PubMed.
- M. Al-Sabbagh, A. Alladah, Y. Lin, R. J. Kryscio, M. V. Thomas and J. L. Ebersole,
et al., Bone remodeling-associated salivary biomarker MIP-1α distinguishes periodontal disease from health, J. Periodontal Res., 2012, 47(3), 389–395 CrossRef CAS PubMed.
- D. H. Fine, K. Markowitz, K. Fairlie, D. Tischio-Bereski, J. Ferrandiz and D. Godboley,
et al., Macrophage inflammatory protein-1α shows predictive value as a risk marker for subjects and sites vulnerable to bone loss in a longitudinal model of aggressive periodontitis, PLoS One, 2014, 9(6), e98541 CrossRef PubMed , PubMed PMID: 24901458. Pubmed Central PMCID: PMC4047026. Epub 20140605. eng.
- D. H. Fine, K. Markowitz, D. Furgang, K. Fairlie, J. Ferrandiz and C. Nasri,
et al., Macrophage inflammatory protein-1alpha: a salivary biomarker of bone loss in a longitudinal cohort study of children at risk for aggressive periodontal disease?, J. Periodontol., 2009, 80(1), 106–113 CrossRef CAS PubMed , PubMed PMID: 19228096. eng.
- K. J. Nisha, A. Suresh, A. Anilkumar and S. Padmanabhan, MIP-1α and MCP-1 as salivary biomarkers in periodontal disease. Saudi, Dent. J., 2018, 30(4), 292–298 CAS , PubMed PMID: 30202165. Pubmed Central PMCID: PMC6128325. Epub 20180706. eng.
- R. Cheng, Z. Wu, M. Li, M. Shao and T. Hu, Interleukin-1β is a potential therapeutic target for periodontitis: a narrative review. International, J. Oral Sci., 2020, 12(1), 2 CAS.
- A. Chandran, R. Bhandary, N. Shenoy and U. A. Shetty, Analysis of collagen fibers in human gingival tissues using picrosirius red stain under polarized microscope, J. Indian Soc. Periodontol., 2021, 25(2), 106–111 CrossRef PubMed , PubMed PMID: 33888941. Pubmed Central PMCID: PMC8041084. Epub 20210301. eng.
- A. M. Marcaccini, C. A. Meschiari, L. R. Zuardi, T. S. De Sousa, M. Taba Jr. and J. M. Teofilo,
et al., Gingival crevicular fluid levels of MMP-8, MMP-9, TIMP-2, and MPO decrease after periodontal therapy, J. Clin. Periodontol., 2010, 37(2), 180–190 CrossRef CAS PubMed.
- I. T. Räisänen, N. Aji, D. Sakellari, A. Grigoriadis, I. Rantala and T. Pätilä,
et al., Active Matrix Metalloproteinase-8 (aMMP-8) Versus Total MMP-8 in Periodontal and Peri-Implant Disease Point-of-Care Diagnostics, Biomedicines, 2023, 11(11), 2885 CrossRef PubMed , PubMed PMID: 38001886. Pubmed Central PMCID: PMC10669684. Epub 20231025. eng.
- P. Ghosh, T. S. Muthuraj, P. Bandyopadhyay, S. Swarnakar, P. Sarkar and A. Varatharajan, Expression of matrix metalloproteinase-9 in gingival tissue biopsy in patients with slowly/ moderately and rapidly progressing periodontitis: An observational study, J. Indian Soc. Periodontol., 2021, 5(5), 386–392 Search PubMed , PubMed PMID: 34667380. Pubmed Central PMCID: PMC8452177. Epub 20210830. eng.
- H. K. Lee, S. J. Kim, Y. H. Kim, Y. Ko, S. Ji and J. C. Park, Odontogenic ameloblast-associated protein (ODAM) in gingival crevicular fluid for site-specific diagnostic value of periodontitis: a pilot study, BMC Oral Health, 2018, 18(1), 148 CrossRef PubMed , PubMed PMID: 30143043. Pubmed Central PMCID: PMC6109327. Epub 20180824. eng.
- T. Tervahartiala, Y. T. Konttinen, T. In, R. Häyrinen-Immonen, Y. Ding and T. Sorsa, Cathepsin G in gingival tissue and crevicular fluid in adult periodontitis, J. Clin. Periodontol., 1996, 23(2), 68–75 CrossRef CAS PubMed , PubMed PMID: 8849841. eng.
- H. Gao, J. Xu, L. He, H. Meng and J. Hou, Calprotectin levels in gingival crevicular fluid and serum of patients with chronic periodontitis and type 2 diabetes mellitus before and after initial periodontal therapy, J. Periodontal Res., 2021, 56(1), 121–130 CrossRef CAS PubMed.
- L. Wei, M. Liu and H. Xiong, Role of Calprotectin as a Biomarker in Periodontal Disease, Mediators Inflammation, 2019, 2019, 3515026 Search PubMed , PubMed PMID: 31530995. Pubmed Central PMCID: PMC6721252. Epub 20190821. eng.
- T. Hiyoshi, H. Domon, T. Maekawa, K. Nagai, H. Tamura and N. Takahashi,
et al., Aggregatibacter actinomycetemcomitans induces detachment and death of human gingival epithelial cells and fibroblasts via elastase release following leukotoxin-dependent neutrophil lysis, Microbiol. Immunol., 2019, 63(3–4), 100–110 CrossRef CAS PubMed , PubMed PMID: 30817027. Epub 20190327. eng.
- K. Y. How, K. P. Song and K. G. Chan, Porphyromonas gingivalis: An Overview of Periodontopathic Pathogen below the Gum Line, Front. microbiol., 2016, 7, 53 Search PubMed , PubMed PMID: 26903954. Pubmed Central PMCID: PMC4746253. Epub 20160209. eng.
- M. N. Sela, Role of Treponema denticola in periodontal diseases, Crit. Rev. Oral Biol. Med., 2001, 12(5), 399–413 CrossRef CAS PubMed , PubMed PMID: 12002822. eng.
- M. Karched, R. G. Bhardwaj, M. Qudeimat, A. Al-Khabbaz and A. Ellepola, Proteomic analysis of the periodontal pathogen Prevotella intermedia secretomes in biofilm and planktonic lifestyles, Sci. Rep., 2022, 12(1), 5636 CrossRef CAS PubMed.
- J. Slots, H. S. Reynolds and R. J. Genco, Actinobacillus actinomycetemcomitans in human periodontal disease: a cross-sectional microbiological investigation, Infect. Immun., 1980, 29(3), 1013–1020 CrossRef CAS PubMed , PubMed PMID: 6968718. Pubmed Central PMCID: PMC551232. eng.
- S. Alhogail, G. A. Suaifan, S. Bizzarro, W. E. Kaman, F. J. Bikker and K. Weber,
et al., On site visual detection of Porphyromonas gingivalis related periodontitis by using a magnetic-nanobead based assay for gingipains protease biomarkers, Microchim. Acta, 2018, 185, 1–8 CrossRef CAS PubMed.
- M. Taba, J. Kinney, A. S. Kim and W. V. Giannobile, Diagnostic biomarkers for oral and periodontal diseases, Dental Clinics, 2005, 49(3), 551–571 Search PubMed.
- H. Shazam, F. Shaikh, M. M. Majeed, S. Khan and S. Khan, Role Of Salivary Biomarkers In Diagnosis Of Periodontitis–A Literature Review, Khyber Med. Univ. J., 2020, 12(4), 326–330 Search PubMed.
- X. Zhang, Z. Hu, X. Zhu, W. Li and J. Chen, Treating periodontitis-a systematic review and meta-analysis comparing ultrasonic and manual subgingival scaling at different probing pocket depths, BMC Oral Health, 2020, 20(1), 1–16 CrossRef CAS PubMed.
- A. Mahapatra, K. Patnaik and S. C. Raj, Probe design: Probing the untold story, Journal of Dental Research and Review, 2021, 8(2), 141–141 Search PubMed.
- M. C. U. Garcia, M. B. G. Ramos and B. P. Tumang, Accuracy and Reproducibility of UNC-15 and Gram Probe# 2 in Measuring Probing Pocket Depths, Acta Med. Philipp., 2022, 56(8), 24–30 Search PubMed.
- V. Machado, L. Proença, M. Morgado, J. J. Mendes and J. Botelho, Accuracy of panoramic radiograph for diagnosing periodontitis comparing to clinical examination, J. Clin. Med., 2020, 9(7), 2313 CrossRef PubMed.
- R. Schulze and N. Drage, Cone-beam computed tomography and its applications in dental and maxillofacial radiology, Clin. Radiol., 2020, 75(9), 647–657 CrossRef CAS PubMed.
- K. N. A. A. Shayeb, W. Turner and D. G. Gillam, Periodontal probing: a review, Prim. Dent. J., 2014, 3(3), 25–29 CrossRef PubMed.
- B. Holtfreter, D. Alte, C. Schwahn, M. Desvarieux and T. Kocher, Effects of different manual periodontal probes on periodontal measurements, J. Clin. Periodontol., 2012, 39(11), 1032–1041 CrossRef PubMed.
- L. Mayfield, G. Bratthall and R. AttStröm, Periodontal probe precision using 4 different periodontal probes, J. Clin. Periodontol., 1996, 23(2), 76–82 CrossRef CAS PubMed.
-
P. J. Robinson and R. M. Vitek, Periodontal Examination, Dental Clinics of North America, 1980, vol. 24(4), pp. 597–612 Search PubMed.
- E. F. Corbet, D. K. L. Ho and S. M. L. Lai, Radiographs in periodontal disease diagnosis and management, Aust. Dent. J., 2009, 54(s1), S27–S43 Search PubMed.
-
N. S. Gasner and R. S. Schure, Periodontal disease, StatPearls, StatPearls Publishing, 2022 Search PubMed.
- J. Botelho, V. Machado, L. Proença and J. J. Mendes, The 2018 periodontitis case definition improves accuracy performance of full-mouth partial diagnostic protocols, Sci. Rep., 2020, 10(1), 7093 CrossRef CAS PubMed.
- S. R. Panzarini, D. Pedrini, W. R. Poi, C. K. Sonoda, D. A. Brandini and J. C. M. Castro, Dental trauma involving root fracture and periodontal ligament injury: a 10-year retrospective study, Braz. Oral Res., 2008, 22, 229–234 CrossRef PubMed.
- P. Jain, Clinical diagnosis in endodontics, Clin. Dent. Rev., 2019, 3(1), 10 CrossRef.
- I. J. Márton and C. Kiss, Protective and destructive immune reactions in apical periodontitis, Oral Microbiol. Immunol., 2000, 15(3), 139–150 CrossRef PubMed.
- R. A. Abou Samra, R. M. El Backly, H. M. Aly, S. R. Nouh and S. M. Moussa, Revascularization in mature permanent teeth with necrotic pulp and apical periodontitis: case series, Alex. Dent. J., 2018, 43(2), 7–12 CrossRef.
- S. Hourdin, D. Glez, G. Gagnot, O. Sorel and S. Jeanne, A screening method for periodontal disease, J. Dentofac. Anom. Orthod., 2013, 16(1), 104 CrossRef , Epub 2013/01/23.
-
Salivary Diagnostics https://www.nidcr.nih.gov, National Institute of Dental and Craniofacial Research, 2018 [updated August 2018], Available from: https://www.nidcr.nih.gov/grants-funding/funded-research/research-investments-advances/salivary-diagnostics Search PubMed.
- K. A. Umeizudike, H. Lähteenmäki, I. T. Räisänen, J. J. Taylor, P. M. Preshaw and S. M. Bissett,
et al., Ability of matrix metalloproteinase-8 biosensor, IFMA, and ELISA immunoassays to differentiate between periodontal health, gingivitis, and periodontitis, J. Periodontal Res., 2022, 57(3), 558–567 CrossRef CAS PubMed.
- R. Park, S. Jeon, J. Jeong, S.-Y. Park, D.-W. Han and S. W. Hong, Recent Advances of Point-of-Care Devices Integrated with Molecularly Imprinted Polymers-Based Biosensors: From Biomolecule Sensing Design to Intraoral Fluid Testing, Biosensors, 2022, 12(3), 136 CrossRef CAS PubMed.
- K. Ichikawa, K. Iitani, G. Kawase, K. Toma, T. Arakawa and D. V. Dao,
et al., Mouthguard-Type Wearable Sensor for Monitoring Salivary Turbidity to Assess Oral Hygiene, Sensors, 2024, 24(5), 1436 CrossRef CAS PubMed.
- Y. Gong, J. Hu, J. R. Choi, M. You, Y. Zheng and B. Xu,
et al., Improved LFIAs for highly sensitive detection of BNP at point-of-care, Int. J. Nanomed., 2017, 12, 4455–4466 CrossRef CAS PubMed , Pubmed Central PMCID: 28670119.
- W. He, M. You, W. Wan, F. Xu, F. Li and A. Li, Point-of-Care Periodontitis Testing: Biomarkers, Current Technologies, and Perspectives, Trends Biotechnol., 2018, 36(11), 1127–1144 CrossRef CAS PubMed , PubMed PMID: 30041883. Epub 20180702. eng.
- S. P. Barros, R. Williams, S. Offenbacher and T. Morelli, Gingival crevicular fluid as a source of biomarkers for periodontitis, Periodontol., 2000, 70(1), 53–64 CrossRef PubMed.
- D. Ge, F. Wang, Y. Hu, B. Wang, X. Gao and Z. Chen, Fast, Simple, and Highly Specific Molecular Detection of Porphyromonas gingivalis Using Isothermal Amplification and Lateral Flow Strip Methods, Front. Cell. Infect. Microbiol., 2022, 12, 895261 CrossRef CAS PubMed , English.
- M. Taba Jr., J. Kinney, A. S. Kim and W. V. Giannobil000e, Diagnostic biomarkers for oral and periodontal diseases, Dent. Clin. North Am., 2005, 49(3), 551–571 CrossRef PubMed , vi. PubMed PMID: 15978241. Pubmed Central PMCID: PMC2580776. eng.
- Z. Li, R. Ju, S. Sekine, D. Zhang, S. Zhuang and Y. Yamaguchi, All-in-one microfluidic device for on-site diagnosis of pathogens based on an integrated continuous flow PCR and electrophoresis biochip, Lab Chip, 2019, 19(16), 2663–2668 RSC.
- W. Zhang, J. Du, K. Wang, Y. Li, C. Chen and L. Yang,
et al., Integrated dual-channel electrochemical immunosensor for early diagnosis and monitoring of periodontitis by detecting multiple biomarkers in saliva, Anal. Chim. Acta, 2023, 1247, 340878 CrossRef CAS PubMed.
- C. Joe, B. H. Lee, S. H. Kim, Y. Ko and M. B. Gu, Aptamer duo-based portable electrochemical biosensors for early diagnosis of periodontal disease, Biosens. Bioelectron., 2022, 199, 113884 CrossRef CAS PubMed.
- J. J. Taylor, K. M. Jaedicke, R. C. van de Merwe, S. M. Bissett, N. Landsdowne and K. M. Whall,
et al., A Prototype Antibody-based Biosensor for Measurement of Salivary MMP-8 in Periodontitis using Surface Acoustic Wave Technology, Sci. Rep., 2019, 9(1), 11034 CrossRef PubMed , PubMed PMID: 31363141. Pubmed Central PMCID: PMC6667706. Epub 20190730. eng.
- S. Mohseni, T. T. Moghadam, B. Dabirmanesh, S. Jabbari and K. Khajeh, Development of a label-free SPR sensor for detection of matrixmetalloproteinase-9 by antibody immobilization on carboxymethyldextran chip, Biosens. Bioelectron., 2016, 81, 510–516 CrossRef CAS PubMed , PubMed PMID: 27016912. Epub 20160319. eng.
- S. Wignarajah, G. A. Suaifan, S. Bizzarro, F. J. Bikker, W. E. Kaman and M. Zourob, Colorimetric Assay for the Detection of Typical Biomarkers for Periodontitis Using a Magnetic Nanoparticle Biosensor, Anal. Chem., 2015, 87(24), 12161–12168 CrossRef CAS PubMed , PubMed PMID: 26631371. Epub 20151203. eng.
- B. H. Lee, S. H. Kim, Y. Ko, J. C. Park, S. Ji and M. B. Gu, The sensitive detection of ODAM by using sandwich-type biosensors with a cognate pair of aptamers for the early diagnosis of periodontal disease, Biosens. Bioelectron., 2019, 126, 122–128 CrossRef CAS PubMed , PubMed PMID: 30396019. Epub 20181023. eng.
- M. Hoyos-Nogués, S. Brosel-Oliu, N. Abramova, F.-X. Muñoz, A. Bratov and C. Mas-Moruno,
et al., Impedimetric antimicrobial peptide-based sensor for the early detection of periodontopathogenic bacteria, Biosens. Bioelectron., 2016, 86, 377–385 CrossRef PubMed.
- J. Ebersole, R. Nagarajan, D. Akers and C. Miller, Targeted salivary biomarkers for discrimination of periodontal health and disease(s), Front. Cell. Infect. Microbiol., 2015, 5, 62 Search PubMed , English.
- L. Guida, D. Bencivenga, M. Annunziata, F. Arcadio, A. Borriello and F. Della Ragione,
et al., An optical fiber-based point-of-care test for periodontal MMP-8 detection: A proof of concept, J. Dent., 2023, 134, 104553 CrossRef PubMed.
- K. Imamura, S. Takayama, A. Saito, E. Inoue, Y. Nakayama and Y. Ogata,
et al., Evaluation of a novel immunochromatographic device for rapid and accurate clinical detection of Porphyromonas gingivalis in subgingival plaque, J. Microbiol. Methods, 2015, 117, 4–10 CrossRef CAS PubMed.
- N. M. O'Brien-Simpson, K. Burgess, J. C. Lenzo, G. C. Brammar, I. B. Darby and E. C. Reynolds, Rapid Chair-Side Test for Detection of Porphyromonas gingivalis, J. Dent. Res., 2017, 96(6), 618–625 CrossRef PubMed , PubMed PMID: 28182517.
- P. Mäntylä, M. Stenman, D. F. Kinane, S. Tikanoja, H. Luoto and T. Salo,
et al., Gingival crevicular fluid collagenase-2 (MMP-8) test stick for chair-side monitoring of periodontitis, J. Periodontal Res., 2003, 38(4), 436–439 CrossRef PubMed.
- S. A. Bhakta, R. Borba, M. Taba, C. D. Garcia and E. Carrilho, Determination of nitrite in saliva using microfluidic paper-based analytical devices, Anal. Chim. Acta, 2014, 809, 117–122 CrossRef CAS PubMed.
- E. H. Yee, S. Lathwal, P. P. Shah and H. D. Sikes, Detection of Biomarkers of Periodontal Disease in Human Saliva Using Stabilized, Vertical Flow Immunoassays, ACS Sens., 2017, 2(11), 1589–1593 CrossRef CAS PubMed.
- S. Wignarajah, G. A. R. Y. Suaifan, S. Bizzarro, F. J. Bikker, W. E. Kaman and M. Zourob, Colorimetric Assay for the Detection of Typical Biomarkers for Periodontitis Using a Magnetic Nanoparticle Biosensor, Anal. Chem., 2015, 87(24), 12161–12168 CrossRef CAS PubMed.
- S. Alhogail, G. A. R. Y. Suaifan, S. Bizzarro, W. E. Kaman, F. J. Bikker, K. Weber, D. Cialla-May, J. Popp and M. Zourob, On site visual detection of Porphyromonas gingivalis related periodontitis by using a magnetic-nanobead based assay for gingipains protease biomarkers, Microchim. Acta, 2018, 185(2), 149 CrossRef PubMed.
- A. M. Heikkinen, S. O. Nwhator, N. Rathnayake, P. Mäntylä, P. Vatanen and T. Sorsa, Pilot study on oral health status as assessed by an active matrix metalloproteinase-8 chairside mouthrinse test in adolescents, J. Periodontol., 2016, 87(1), 36–40 CrossRef CAS PubMed.
- J.-i. Kido, K. Abe, S. Yatsushiro, M. Bando, Y. Hiroshima and T. Nagata,
et al., Determination of calprotectin in gingival crevicular fluid by immunoassay on a microchip, Clin. Biochem., 2012, 45(15), 1239–1244 CrossRef CAS PubMed.
- C. Gaertig, K. Niemann, J. Berthold, L. Giel, N. Leitschuh and C. Boehm,
et al., Development of a point-of-care-device for fast detection of periodontal pathogens, BMC Oral Health, 2015, 15(1), 165 CrossRef PubMed.
- D. Ivnitski, R. Sitdikov and N. Ivnitski, Non-invasive electrochemical hand-held biosensor as diagnostic indicator of dental diseases, Electrochem. Commun., 2003, 5(3), 225–229 CrossRef CAS.
- A. G. Morrison, S. Sarkar, S. Umar, S. T. Lee and S. M. Thomas, The Contribution of the Human Oral Microbiome to Oral Disease: A Review, Microorganisms, 2023, 11(2), 318 CrossRef CAS PubMed.
- X. Peng, L. Cheng, Y. You, C. Tang, B. Ren and Y. Li,
et al., Oral microbiota in human systematic diseases. International, J. Oral Sci., 2022, 14(1), 14 CAS.
-
M. Parras-Moltó and A. López-Bueno, Methods for Enrichment and Sequencing of Oral Viral Assemblages: Saliva, Oral Mucosa, and Dental Plaque Viromes, inThe Human Virome: Methods and Protocols, ed. Moya A and Pérez Brocal V, Springer New York, New York, NY, 2018, pp. 143–161 Search PubMed.
- P. Gholizadeh, A. Pormohammad, H. Eslami, B. Shokouhi, V. Fakhrzadeh and H. S. Kafil, Oral pathogenesis of Aggregatibacter actinomycetemcomitans, Microb. Pathog., 2017, 113, 303–311 CrossRef CAS PubMed.
- J. P. Haran, E. Bradley, A. L. Zeamer, L. Cincotta, M.-C. Salive and P. Dutta,
et al., Inflammation-type dysbiosis of the oral microbiome associates with the duration of COVID-19 symptoms and long COVID, JCI Insight, 2021, 6(20), 1–14 CrossRef PubMed.
- L. Collins and C. Dawes, The surface area of the adult human mouth and thickness of the salivary film covering the teeth and oral mucosa, J. Dent. Res., 1987, 66(8), 1300–1302 CrossRef CAS PubMed.
- M. Kilian, I. L. C. Chapple, M. Hannig, P. D. Marsh, V. Meuric and A. M. L. Pedersen,
et al., The oral microbiome – an update for oral healthcare professionals, Br. Dent. J., 2016, 221(10), 657–666 CrossRef CAS PubMed.
- S. S. Socransky, C. Smith and A. D. Haffajee, Subgingival microbial profiles in refractory periodontal disease, J. Clin. Periodontol., 2002, 29(3), 260–268 CrossRef PubMed.
- S. Socransky, A. Haffajee, M. Cugini, C. Smith and R. Kent Jr., Microbial complexes in subgingival plaque, J. Clin. Periodontol., 1998, 25(2), 134–144 CrossRef CAS PubMed.
- N. Zaatout, Presence of non-oral bacteria in the oral cavity, Arch. Microbiol., 2021, 203(6), 2747–2760 CrossRef CAS PubMed.
- S. N. Gaber, E. E. M. Hemeda, H.-A. S. Elsayeh, W. Y. A. Wahed, M. A. Khalil and E. G. Ibrahim, Propolis extract: A possible antiseptic oral care against multidrug-resistant non-fermenting bacteria isolated from non-ventilator hospital-acquired pneumonia, J. Pure Appl. Microbiol., 2020, 14(1), 123–131 CrossRef CAS.
- A. S. Alagl, M. Madi, S. Bedi, F. Al Onaizan and Z. S. Al-Aql, The effect of Er, Cr: YSGG and diode laser applications on dental implant surfaces contaminated with Acinetobacter baumannii and Pseudomonas aeruginosa, Materials, 2019, 12(13), 2073 CrossRef CAS PubMed.
- E. D. Valinetz and G. A. Cangelosi, A Look Inside: Oral Sampling for Detection of Non-oral Infectious Diseases, J. Clin. Microbiol., 2021, 59(10), 1–10 CrossRef PubMed.
- K. Mitsakakis, F. Stumpf, O. Strohmeier, V. Klein, D. Mark and F. Von Stetten,
et al., Chair/bedside diagnosis of oral and respiratory tract infections, and identification of antibiotic resistances for personalised monitoring and treatment, Stud. Health Technol. Inform., 2016, 224, 61–66 Search PubMed.
- E. U. Uzoukwu, V. Phandanouvong-Lozano, H. Usman, C. S. Sfeir and T. H. Niepa, Droplet-based microsystems as novel assessment tools for oral microbial dynamics, Biotechnol. Adv., 2022, 55, 107903 CrossRef CAS PubMed.
- I. T. Räisänen, T. Sorsa, G.-J. van der Schoor, T. Tervahartiala, P. van der Schoor and D.-R. Gieselmann,
et al., Active Matrix Metalloproteinase-8 Point-of-Care (PoC)/Chairside Mouthrinse Test vs. Bleeding on Probing in Diagnosing Subclinical Periodontitis in Adolescents, Diagnostics, 2019, 9(1), 34, DOI:10.3390/diagnostics9010034.
- W. V. Giannobile, T. Beikler, J. S. Kinney, C. A. Ramseier, T. Morelli and D. T. Wong, Saliva as a diagnostic tool for periodontal disease: current state and future directions, Periodontol., 2000, 2009(50), 52–64 Search PubMed , PubMed PMID: 19388953. Pubmed Central PMCID: PMC5695225. eng.
- K. S. Kornman, Contemporary approaches for identifying individual risk for periodontitis, Periodontol., 2000, 78(1), 12–29 CrossRef PubMed.
- U. Braegger, Cost-benefit, cost-effectiveness and cost-utility analyses of periodontitis prevention, J. Clin. Periodontol., 2005, 32(Suppl 6), 301–313 CrossRef PubMed , PubMed PMID: 16128845. eng.
- J. Gomez Rossi, N. Rojas-Perilla, J. Krois and F. Schwendicke, Cost-effectiveness of Artificial Intelligence as a Decision-Support System Applied to the Detection and Grading of Melanoma, Dental Caries, and Diabetic Retinopathy, JAMA Netw. Open, 2022, 5(3), e220269 CrossRef PubMed.
- S. B. Khanagar, A. Al-Ehaideb, P. C. Maganur, S. Vishwanathaiah, S. Patil and H. A. Baeshen,
et al., Developments, application, and performance of artificial intelligence in dentistry–A systematic review, J. Dent. Sci., 2021, 16(1), 508–522 CrossRef PubMed.
- S. Deshmukh, Artificial intelligence in dentistry, J. Int. Clin. Dent. Res. Organ., 2018, 10(2), 47 CrossRef.
- V. Bindushree, R. Sameen, V. Vasudevan, T. Shrihari, D. Devaraju and N. S. Mathew, Artificial intelligence: In modern dentistry, J. Dent. Res. Rev., 2020, 7(1), 27–31 Search PubMed.
- S. T. Israni and A. Verghese, Humanizing artificial intelligence, JAMA, J. Am. Med. Assoc., 2019, 321(1), 29–30 CrossRef PubMed.
-
L. Engelmann, Into the Deep-AI and Total Pathology: Deep Medicine: How Artificial Intelligence Can Make Healthcare Human Again, by Eric Topol, Basic Books, New York, 2010, 400 pp., $17.99 (paperback), ISBN 9781541644649, Taylor & Francis, 2020 Search PubMed.
- C. A. Ramseier, A. Anerud, M. Dulac, M. Lulic, M. P. Cullinan and G. J. Seymour,
et al., Natural history of periodontitis: Disease progression and tooth loss over 40 years, J. Clin. Periodontol., 2017, 44(12), 1182–1191 CrossRef PubMed.
- R. Raj, K. Mehrotra, I. Narayan, T. M. Gowda and D. Mehta, Natural tooth pontic: an instant esthetic option for periodontally compromised teeth—a case series, Case Rep. Dent., 2016, 2016, 8502927 Search PubMed.
- J.-H. Lee, D.-h. Kim, S.-N. Jeong and S.-H. Choi, Diagnosis and prediction of periodontally compromised teeth using a deep learning-based convolutional neural network algorithm, J. Periodontal Implant Sci., 2018, 48(2), 114–123 CrossRef PubMed.
- S. Vishwanathaiah, H. N. Fageeh, S. B. Khanagar and P. C. Maganur, Artificial intelligence its uses and application in pediatric dentistry: a review, Biomedicines, 2023, 11(3), 788 CrossRef PubMed.
- Y. H. Cheng, R. Kargupta, D. Ghoshal, Z. Li, C. Chande and L. Feng,
et al., ESSENCE - A rapid, shear-enhanced, flow-through, capacitive electrochemical platform for rapid detection of biomolecules, Biosens. Bioelectron., 2021, 182, 113163 CrossRef CAS PubMed , PubMed PMID: 33826991. Epub 20210319. eng.
- W. Zhang, J. Du, K. Wang, Y. Li, C. Chen and L. Yang,
et al., Integrated dual-channel electrochemical immunosensor for early diagnosis and monitoring of periodontitis by detecting multiple biomarkers in saliva, Anal. Chim. Acta, 2023, 1247, 340878 CrossRef CAS PubMed , PubMed PMID: 36781246. Epub 2023/02/14.
- X. Qiao, K. Li, J. Xu, N. Cheng, Q. Sheng and W. Cao,
et al., Novel electrochemical sensing platform for ultrasensitive detection of cardiac troponin I based on aptamer-MoS(2) nanoconjugates, Biosens. Bioelectron., 2018, 113, 142–147 CrossRef CAS PubMed , PubMed PMID: 29754053. Epub 2018/05/14.
Footnote |
† Charmi Chande and Alexandra Griffith contributed equally to the manuscript. |
|
This journal is © The Royal Society of Chemistry 2024 |
Click here to see how this site uses Cookies. View our privacy policy here.