DOI:
10.1039/D4SC03802A
(Edge Article)
Chem. Sci., 2024,
15, 15819-15824
meta-C–H functionalization of phenylethyl and benzylic alcohol derivatives via Pd/NBE relay catalysis†
Received
10th June 2024
, Accepted 3rd September 2024
First published on 4th September 2024
Abstract
The transition metal-catalyzed meta-C–H functionalization of alcohols and their hydroxylamine derivatives remains underdeveloped. Herein, we report an efficient meta-C–H arylation of both phenylethyl and benzylic alcohols and their hydroxylamine derivatives using a readily removable oxime ether directing group. Using electronically activated 2-carbomethoxynorbornene as the transient mediator and 3-trifluoromethyl-2-pyridone as the enabling ligand, this reaction features a broad substrate scope and good functional group tolerance. More importantly, with this oxime-directed meta-C–H functionalization, this method provides a dual approach for efficient access to both meta-substituted alcohols and hydroxylamines using two sets of simple deprotection conditions. This protocol leads to the efficient synthesis of bioactive compounds possessing promising reactivities for the treatment of pulmonary fibrosis.
Introduction
Phenylethyl and benzylic alcohols and their hydroxylamine derivatives are commonly found in a wide range of bioactive molecules, including tolvaptan, prothioconazole, tramadol, fenaminstrobin, siponimod etc. (Scheme 1a).1 In addition, they are also significant and widely utilizable synthetic building blocks that can engage in many fundamental transformations2 and heterocycle constructions.3 Thus, developing general and practical C–H transformations of alcohols and their hydroxylamine derivatives are highly valuable for late-stage diversification of alcohol or hydroxylamine containing bioactive scaffolds. Although transition metal catalyzed ortho-C–H functionalization of aryl alcohols has been well studied,4 diverse meta-C–H functionalizations of those substrates are relatively less common and inefficient in both substrate and coupling partner scope5,6 in spite of significant progress in the field of transition metal catalyzed meta-C–H activation.7–10 Taking advantage of the Pd/NBE relay approach6,9 for efficient meta-C–H functionalization, the only example of meta-arylation of benzylic alcohol derivatives has been reported by the Ferreira group (Scheme 1b). However, this reaction is limited to benzylic alcohols and a large excess of aryl iodides (4.0 equiv.) is required to give synthetically useful yields.6 Furthermore, the preparation of the directing group in this reaction is not straightforward. Herein, we report a palladium-catalyzed meta-C–H functionalization of oxime ethers using 2-carbomethoxynorbornene (NBE-CO2Me) as a transient mediator and commercially available 3-trifluoromethyl-2-pyridone as the ligand (Scheme 1d). This reaction features a broad substrate scope and good functional group tolerance. More importantly, with this oxime-directed meta-C–H functionalization, this method provides a “one stone two birds” approach (Scheme 1c) for efficient access to meta-substituted alcohols and hydroxylamines by the removal of the directing group under different conditions.
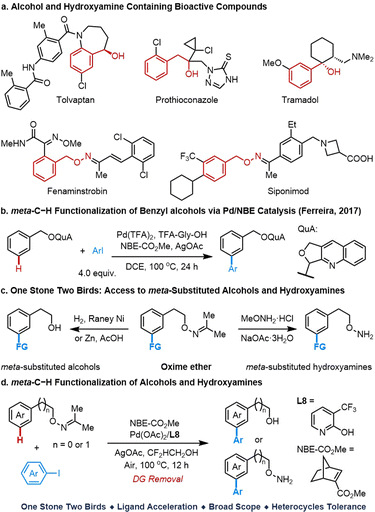 |
| Scheme 1
meta-C–H functionalization of alcohol and hydroxylamine via Pd/NBE relay catalysis. | |
Results and discussion
Our group has reported palladium-catalyzed hydroxy-directed ortho-C–H olefination of tertiary phenethyl alcohols with the assistance of an MPAA ligand.4a–c However, directed meta-C–H arylation using the native hydroxy group under our previous meta-C–H arylation conditions resulted in no reaction and the slight decomposition of the primary alcohol. Despite methyl ether (DG2) being stable under these conditions, no desired product was observed either. Although the pyridine directing group DG3 is inert, we are pleased to find that the acetone oxime directing group (DG4) could provide the desired meta-arylated product in 14% NMR yield. Cyclohexanone oxime ether (DG5) also showed similar reactivity (Table 1). Following this finding, systematic evaluation of reaction parameters was conducted to further improve the outcomes using the acetone oxime ether as the directing group (Table 2). Notably, fluorinated alcohols can raise the yield drastically, and 2,2-difluoroethanol was identified as the most efficient solvent for this reaction due to the better mass balance (for more details, see the ESI†). With 2,2-difluoroethanol as the optimal solvent, the ligand effect was further evaluated, and we are pleased to find that the pyridone ligands are superior to the mono-protected amino acid (MPAA) ligand (L1), pyridine ligand (L2), and bulky carboxylic acid (L3). In general, both electron-rich (L4, L5) and electron-deficient (L6–8) 2-pyridone ligands could dramatically improve the yield of this reaction, in which commercially available 3-trifluoromethyl-2-pyridone L8 gave the best outcome (87% yield). Switching the CF3 group to other positions at the 2-pyridone scaffold (L9, L10) or more electron-deficient 2-pyridone ligands (L11, L12) cannot further enhance the reactivity. The mono-protected 3-amino-2-pyridone ligands (L13, L14) also provide the desired product in 68% and 85% yields, respectively. It is worth mentioning that the control experiments indicate the pyridone ligand is crucial for accelerating this meta-arylation of phenethyl alcohol-derived oxime ether, and only 21% NMR yield of the desired product was obtained in the absence of ligand.
Table 1 Directing group evaluation for meta-C–H functionalization of alcohol derivativesab
Reaction conditions: substrate (0.1 mmol), 2j (52.4 mg, 0.2 mmol), Pd(OAc)2 (2.2 mg, 10 mol%), L14 (4.4 mg, 20 mol%), AgOAc (25.0 mg, 0.15 mmol), NBE-CO2Me (22.8 mg, 0.15 mmol), CHCl3 (0.5 mL), air, 100 °C, 12 h.
The yield was determined by 1H NMR analysis of the crude product using CH2Br2 as the internal standard.
|
|
Table 2 Ligand evaluation for Pd-catalyzed meta-C–H functionalization of oxime ethersab
Reaction conditions: 1 (19.1 mg, 0.1 mmol), 2j (52.4 mg, 0.2 mmol), Pd(OAc)2 (2.2 mg, 10 mol%), L (20 mol%), AgOAc (25.0 mg, 0.15 mmol), NBE-CO2Me (22.8 mg, 0.15 mmol), CF2HCH2OH (0.1 mL), air, 100 °C, 12 h.
The yield was determined by 1H NMR analysis of the crude product using CH2Br2 as the internal standard.
|
|
Under the optimal conditions, the scope of aryl iodides was evaluated using 2-(m-tolyl)ethan-1-ol derived acetone oxime 1 as the model substrate (Table 3). In general, para-substituted aryl iodides containing both electron-rich substituents (2b–d) and electron-deficient substituents (2e–l) were all suitable coupling partners, providing the desired meta-arylated products in 40–84% yields. The 3-substituted (2m, 2n) aryl iodides showed similar reactivities in comparison to the para-substituted aryl iodides. Under the highly acidic conditions, 2-substituted aryl iodides are highly active, resulting in more side products via the classic Catellani reaction pathway. Accordingly, less reactive aryl bromides (2o, 2p) provide higher yields than the corresponding aryl iodides. Multiple substituted aryl iodides (3q–t) were also evaluated, affording the desired products in good yields. Notably, the heteroaryl iodides, containing pyridine (2u, 2v) and thiophene (2w) motifs, were well tolerated with this procedure. Aryl iodides derived from estrone (2x) and ciprofibrate (2y) were also investigated, which further demonstrated the generality of this reaction towards the complex scaffold.
Table 3 Scope of aryl iodidesabc
Reaction conditions: 1 (38.3 mg, 0.2 mmol), 2 (0.4 mmol), Pd(OAc)2 (4.5 mg, 10 mol%), L8 (6.5 mg, 20 mol%), AgOAc (50.1 mg, 0.30 mmol), NBE-CO2Me (45.7 mg, 0.30 mmol), CF2HCH2OH (0.2 mL), 100 °C, 12 h.
Isolated yield.
Ar–Br was used instead of Ar–I.
|
|
Next, we turned our attention to check the breadth of aromatic oxime substrates (Table 4). The simple phenethyl alcohol-derived acetone oxime ether (4a) gave a mixture of mono- and di-products in 69% total yield with a mono/di ratio of 1.5/1.0. The phenethyl alcohol-derived oxime ethers bearing both electron-donating and electron-withdrawing substituents at the 3-position (4b–h), such as phenyl, methoxy, trifluoromethoxy, and acetylamino groups and halides, were all tolerated, providing the desired products in high efficiency. For ortho-substituted phenethyl alcohol derivatives (4i–l), 2,2,2-trifluoroethanol (TFE) was found to be a more efficient solvent than CF2HCH2OH, giving the arylated products in 43–76% yields. The meta-C–H bond on the thiophene ring could also be arylated (4m) with a lower yield probably due to the stability of the substrate under the reaction conditions.
Table 4 Scope of acetone oxime ethersabcde
Reaction conditions: 4 (0.2 mmol), 2j (104.8 mg, 0.4 mmol), Pd(OAc)2 (4.5 mg, 10 mol%), L8 (6.5 mg, 20 mol%), AgOAc (50.1 mg, 0.30 mmol), NBE-CO2Me (45.7 mg, 0.30 mmol), CF2HCH2OH (0.2 mL), 100 °C, 12 h.
Isolated yield.
TFE was used.
AgOAc (100.1 mg, 0.60 mmol) was used.
L14 was used instead of L8.
|
|
To our delight, phenethyl alcohols bearing both α- (4n–o) and β-substituents (4p–r) were tolerated with this procedure (4n–s), indicating the great potential of the current method for late-stage modification of complex primary and secondary alcohols. Less challenging benzylic alcohol derivatives with various substituents on the aromatic ring (4t–v) and the benzyl position (4w–y) were also investigated. Generally, the benzylic alcohol derived substrates showed higher reactivities than phenethyl alcohols.
The scalability of this reaction was demonstrated by conducting this reaction on a 5.0 mmol scale, affording the desired arylated product 3j in 86% yield (Scheme 2a). Gratifyingly, the acetone oxime directing group could be selectively converted to alcohol 7 and hydroxylamine 6 in high yields under reductive conditions and mild hydrolysis conditions, respectively. The newly developed protocol could not only provide an efficient strategy for the late-stage installation of functionalities at the meta-position of the aromatic alcohols and their hydroxylamine derivatives, but also paved a new way for the synthesis of bioactive compounds. For example, the 2,5-diaryl substituted phenylethanol 11 and its hydroxylamine derivative 12, both possessing promising reactivities for the treatment of pulmonary fibrosis,11 could be prepared in high efficiency within three steps by leveraging our method as the key synthetic step (Scheme 2c). For comparison, those compounds were prepared in nine (for 11) or eleven steps (for 12) starting from 5-bromo-2-hydroxybenzaldehyde.11
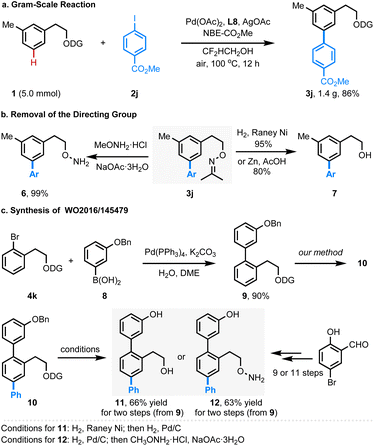 |
| Scheme 2 Gram-scale reaction and synthetic applications. | |
Based on the previous works on the meta-C–H activation via Pd/NBE relay catalysis,9 a proposed mechanism is depicted in Scheme 3. Firstly, oxime directed ortho-C–H activation occurs to give Int I, followed by the migration insertion of NBE-CO2Me, and sequential meta-C–H activation to generate Int II. The meta-functionalization happened via the oxidative addition with aryl iodide and reductive elimination. NBE-CO2Me could be regenerated by β-C elimination, and the product was formed via protodemetalation of Int IV.
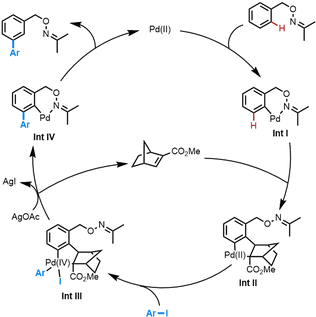 |
| Scheme 3 Proposed mechanism. | |
Conclusions
In summary, a Pd-catalyzed meta-functionalization of oxime ethers was realized by using Pd/NBE relay catalysis. The 3-trifluoromethyl pyridone ligand was identified as the enabling ligand, which could significantly enhance the efficiency of this meta-functionalization. This procedure features a broad substrate scope and remarkable functional group compatibility, thus paving a practical way for the construction of meta-substituted alcohols and their hydroxylamine derivatives.
Data availability
The data supporting this article have been included as part of the ESI.†
Author contributions
H.-C. S. and J.-J. L. performed the experiments and analysed the data. P. W. guided the experiments. P. W. and J.-Q. Y. conceived this concept and prepared this manuscript with feedback from H.-C. S. and J.-J. L.
Conflicts of interest
There are no conflicts to declare.
Acknowledgements
We gratefully acknowledge the National Key R&D Program of China (2023YFF0723900), National Natural Science Foundation of China (22371293, 22171277, 22101291), Strategic Priority Research Program of the Chinese Academy of Sciences (XDB0610000), Program of Shanghai Academic/Technology Research Leader (23XD1424500), Shanghai Institute of Organic Chemistry (SIOC), and State Key Laboratory of Organometallic Chemistry for financial support. We also thank J.-P. Wang at SIOC for verifying the reproducibility of this work.
Notes and references
-
(a) R. W. Schrier, P. Gross, M. Gheorghiade, T. Berl, J. G. Verbalis, F. S. Czerwiec and C. Orlandi, N. Engl. J. Med., 2006, 355, 2099–2112 CrossRef CAS PubMed;
(b) J. E. Parker, A. G. Warrilow, H. J. Cools, C. M. Martel, W. D. Nes, B. A. Fraaije, J. A. Lucas, D. E. Kelly and S. L. Kelly, Appl. Environ. Microbiol., 2011, 77, 1460–1465 CrossRef CAS PubMed;
(c) W. Richter, H. Barth, L. Flohe and H. Giertz, Arzneimittelforschung, 1985, 35, 1742–1744 CAS;
(d)
M. Ishaque, G. Schnabel and D. D. Anspaugh, WO2009/153231A2, 2009;
(e) P. Gergely, B. Nuesslein-Hildesheim, D. Guerini, V. Brinkmann, M. Traebert, C. Bruns, S. Pan, N. Gray, K. Hinterding, N. Cooke, A. Groenewegen, A. Vitaliti, T. Sing, O. Luttringer, J. Yang, A. Gardin, N. Wang, W. Crumb, M. Saltzman, M. Rosenberg and E. Wallström, Br. J. Pharmacol., 2012, 167, 1035–1047 CrossRef CAS PubMed.
-
R. C. Larock, Comprehensive Organic Transformations: A Guide to Functional Group Preparations, Wiley-VCH, New York, 2nd edn, 1989 Search PubMed.
- For selected examples, see:
(a) T. Kondo, S. Yang, K.-T. Huh, M. Kobayashi, S. Kotachi and Y. Watanabe, Chem. Lett., 1991, 20, 1275–1278 CrossRef;
(b) J. Zhou and J. Fang, J. Org. Chem., 2011, 76, 7730–7736 CrossRef CAS PubMed;
(c) H.-J. Li, C.-C. Wang, S. Zhu, C.-Y. Dai and Y.-C. Wu, Adv. Synth. Catal., 2015, 357, 583–588 CrossRef CAS.
- For free hydroxyl group directed ortho-C–H activation, see:
(a) Y. Lu, D.-H. Wang, K. M. Engle and J.-Q. Yu, J. Am. Chem. Soc., 2010, 132, 5916–5921 CrossRef CAS PubMed;
(b) X. Wang, Y. Lu, H.-X. Dai and J.-Q. Yu, J. Am. Chem. Soc., 2010, 132, 12203–12205 CrossRef CAS PubMed;
(c) Y. Lu, D. Leow, X. Wang, K. M. Engle and J.-Q. Yu, Chem. Sci., 2011, 2, 967–971 RSC;
(d) K. Morimoto, K. Hirano, T. Satoh and M. Miura, J. Org. Chem., 2011, 76, 9548–9551 CrossRef CAS PubMed;
(e) L. Li, Q. Liu, J. Chen and Y. Huang, Synlett, 2019, 30, 1366–1370 CrossRef CAS;
(f) D. A. Strassfeld, C.-Y. Chen, H. S. Park, D. Q. Phan and J.-Q. Yu, Nature, 2023, 622, 80–86 CrossRef CAS PubMed ; For selected examples of ortho-C–H activation with a directing group, see: ;
(g) E. M. Simmons and J. F. Hartwig, J. Am. Chem. Soc., 2010, 132, 17092–17095 CrossRef CAS PubMed;
(h) K. Guo, X. Chen, M. Guan and Y. Zhao, Org. Lett., 2015, 17, 1802–1805 CrossRef CAS PubMed;
(i) Z. Ren, J. E. Schulz and G. Dong, Org. Lett., 2015, 17, 2696–2699 CrossRef CAS PubMed;
(j) L.-Y. Shao, C. Li, Y. Guo, K.-K. Yu, F.-Y. Zhao, W.-L. Qiao, H.-W. Liu, D.-H. Liao and Y.-F. Ji, RSC Adv., 2016, 6, 78875–78880 RSC;
(k) B. J. Knight, J. O. Rothbaum and E. M. Ferreira, Chem. Sci., 2016, 7, 1982–1987 RSC;
(l) Y.-J. Mao, S.-J. Lou, H.-Y. Hao and D.-Q. Xu, Angew. Chem., Int. Ed., 2018, 57, 14085–14089 CrossRef CAS PubMed.
-
(a) D. S. Leow, G. Li, T.-S. Mei and J.-Q. Yu, Nature, 2012, 486, 518–522 CrossRef CAS PubMed;
(b) S. Lee, H. Lee and K. L. Tan, J. Am. Chem. Soc., 2013, 135, 18778–18781 CrossRef CAS PubMed;
(c) L. Chu, M. Shang, K. Tanaka, Q. H. Chen, N. Pissarnitski, E. Streckfuss and J.-Q. Yu, ACS Cent. Sci., 2015, 1, 394–399 CrossRef CAS PubMed;
(d) L. Zhang, C. Zhao, Y. Liu, J. Xu, X. Xu and Z. Jin, Angew. Chem., Int. Ed., 2017, 56, 12245–12249 CrossRef CAS PubMed;
(e) S.-D. Li, H. Wang, Y.-X. Weng and G. Li, Angew. Chem., Int. Ed., 2019, 58, 18502–18507 CrossRef CAS PubMed;
(f) H. Xu, M. Liu, L.-J. Li, Y.-F. Cao, J.-Q. Yu and H.-X. Dai, Org. Lett., 2019, 21, 4887–4891 CrossRef CAS PubMed.
- Q. Li and E. M. Ferreira, Chem.–Eur. J., 2017, 23, 11519–11523 CrossRef CAS PubMed.
- For a review on template-directed meta-C–H functionalization, see:
(a) G. Meng, N. Y. S. Lam, E. L. Lucas, T. G. Saint-Denis, P. Verma, N. Chekshin and J.-Q. Yu, J. Am. Chem. Soc., 2020, 142, 10571–10591 CrossRef CAS PubMed . For selected examples, see: ;
(b) L. Wan, N. Dastbaravardeh, G. Li and J.-Q. Yu, J. Am. Chem. Soc., 2013, 135, 18056–18059 CrossRef CAS PubMed;
(c) R. Tang, G. Li and J.-Q. Yu, Nature, 2014, 507, 215–220 CrossRef CAS PubMed;
(d) Y. Kuninobu, H. Ida, M. Nishi and M. Kanai, Nat. Chem., 2015, 7, 712–717 CrossRef CAS PubMed;
(e) H. J. Davis, M. T. Mihai and R. J. Phipps, J. Am. Chem. Soc., 2016, 138, 12759–12762 CrossRef CAS PubMed;
(f) Z. Zhang, K. Tanaka and J.-Q. Yu, Nature, 2017, 543, 538–542 CrossRef CAS PubMed.
- For a review on ruthenium(II)-catalyzed meta-C–H functionalization by ortho-cyclometallation, see:
(a) J. A. Leitch and C. G. Frost, Chem. Soc. Rev., 2017, 46, 7145–7153 RSC ; for selected examples, see:;
(b) O. Saidi, J. Marafie, A. E. W. Ledger, P. M. Liu, M. F. Mahon, G. Kociok-Kçhn, M. K. Whittlesey and C. G. Frost, J. Am. Chem. Soc., 2011, 133, 19298–19301 CrossRef CAS PubMed;
(c) N. Hofmann and L. Ackermann, J. Am. Chem. Soc., 2013, 135, 5877–5884 CrossRef CAS PubMed;
(d) C. J. Teskey, A. Y. W. Lui and M. F. Greaney, Angew. Chem., Int. Ed., 2015, 54, 11677–11680 CrossRef CAS PubMed;
(e) Z. Fan, J. Ni and A. Zhang, J. Am. Chem. Soc., 2016, 138, 8470–8475 CrossRef CAS PubMed;
(f) C. C. Yuan, X. L. Chen, J. Y. Zhang and Y. S. Zhao, Org. Chem. Front., 2017, 4, 1867–1871 RSC;
(g) Y. Wang, S. Chen, X. Chen, A. Zangarelli and L. Ackermann, Angew. Chem., Int. Ed., 2022, 61, e202205562 CrossRef CAS PubMed;
(h) J. Wu, N. Kaplaneris, J. Pöhlmann, T. Michiyuki, B. Yuan and L. Ackermann, Angew. Chem., Int. Ed., 2022, 61, e202208620 CrossRef CAS PubMed.
- For selected examples on meta-C–H arylation using a palladium/norbornene relay process, see:
(a) X.-C. Wang, W. Gong, L.-Z. Fang, R.-Y. Zhu, S. Li, K. M. Engle and J.-Q. Yu, Nature, 2015, 519, 334–338 CrossRef CAS PubMed;
(b) Z. Dong, J. Wang and G. Dong, J. Am. Chem. Soc., 2015, 137, 5887–5890 CrossRef CAS PubMed;
(c) P.-X. Shen, X.-C. Wang, P. Wang, R.-Y. Zhu and J.-Q. Yu, J. Am. Chem. Soc., 2015, 137, 11574–11577 CrossRef CAS PubMed;
(d) P. Wang, M. E. Farmer, X. Huo, P. Jain, P.-X. Shen, M. Ishoey, J. E. Bradner, S. R. Wisniewski, M. D. Eastgate and J.-Q. Yu, J. Am. Chem. Soc., 2016, 138, 9269–9276 CrossRef CAS PubMed;
(e) P. Wang, G.-C. Li, P. Jain, M. E. Farmer, J. He, P.-X. Shen and J.-Q. Yu, J. Am. Chem. Soc., 2016, 138, 14092–14099 CrossRef CAS PubMed;
(f) H. Shi, P. Wang, S. Suzuki, M. E. Farmer and J.-Q. Yu, J. Am. Chem. Soc., 2016, 138, 14876–14879 CrossRef CAS PubMed;
(g) J. Han, L. Zhang, Y. Zhu, Y. Zheng, X. Chen, Z.-B. Huang, D.-Q. Shi and Y. Zhao, Chem. Commun., 2016, 52, 6903–6906 RSC;
(h) H. Shi, A. N. Herron, Y. Shao, Q. Shao and J.-Q. Yu, Nature, 2018, 558, 581–585 CrossRef CAS PubMed;
(i) L.-Y. Liu, J. X. Qiao, K.-S. Yeung, W. R. Ewing and J.-Q. Yu, J. Am. Chem. Soc., 2019, 141, 14870–14877 CrossRef CAS PubMed;
(j) R. Li, Y. Zhou, X. Xu and G. Dong, J. Am. Chem. Soc., 2019, 141, 18958–18963 CrossRef CAS PubMed;
(k) V. Sukowski, M. van Borselen, S. Mathew and M. Á. Fernández-Ibáñe, Angew. Chem., Int. Ed., 2022, 61, e202201750 CrossRef CAS PubMed.
- For meta-C–H functionalization by either steric or electronic influences:
(a) T. Ishiyama, J. Takagi, K. Ishida, N. Miyaura, N. R. Anastasi and J. F. Hartwig, J. Am. Chem. Soc., 2002, 124, 390–391 CrossRef CAS PubMed;
(b) J.-Y. Cho, M. K. Tse, D. Holmes, R. E. Maleczka Jr and M. R. Smith III, Science, 2002, 295, 305–308 CrossRef CAS PubMed;
(c) Y. Saito, Y. Segawa and K. Itami, J. Am. Chem. Soc., 2015, 137, 5193–5198 CrossRef CAS PubMed;
(d) R. Bisht and B. Chattopadhyay, J. Am. Chem. Soc., 2016, 138, 84–87 CrossRef CAS PubMed;
(e) B. Ramadoss, Y. Jin, S. Asako and L. Iles, Science, 2022, 375, 658–663 CrossRef CAS PubMed;
(f) R. J. Phipps and M. J. Gaunt, Science, 2009, 323, 1593–1597 CrossRef CAS PubMed;
(g) Y.-H. Zhang, B.-F. Shi and J.-Q. Yu, J. Am. Chem. Soc., 2009, 131, 5072–5074 CrossRef CAS PubMed . For an example using a traceless directing group relay strategy: ;
(h) J. Luo, S. Preciado and I. Larrosa, J. Am. Chem. Soc., 2014, 136, 4109–4112 CrossRef CAS PubMed.
-
K. A. Duggan, WO2016/145479A1, 2016.
|
This journal is © The Royal Society of Chemistry 2024 |
Click here to see how this site uses Cookies. View our privacy policy here.