DOI:
10.1039/D4SC02075H
(Edge Article)
Chem. Sci., 2024,
15, 11550-11556
Synthesis and application of well-defined [Ph4P]+[Cu(CF2CF3)2]− complex as a versatile pentafluoroethylating reagent†
Received
28th March 2024
, Accepted 7th June 2024
First published on 20th June 2024
Abstract
We herein describe the preparation and application of a new bispentafluoroethylated organocuprate [Ph4P]+[Cu(CF2CF3)2]−. This complex has demonstrated a remarkable range of reactivities towards carboxylic acids, diazonium salts, organic halides, boronic esters, terminal alkynes and (hetero)arenes as a versatile pentafluoroethylating reagent. The construction of C(sp3)–/C(sp2)–/C(sp)–CF2CF3 bonds can therefore be achieved using a single reagent.
Introduction
Perfluoroalkylated molecules represent a significant portion of existing pharmaceutical compounds or drug candidates owing to their desirable biological and chemophysical properties.1 Consequently, methods for introducing perfluoroalkyl groups into organic molecules have become increasingly important.2 Trifluoromethylation, the introduction of the smallest perfluoroalkyl group CF3, has reached a certain level of maturity.3 In contrast, the development of analogous pentafluoroethylation for introducing the CF2CF3 group has been lagging drastically behind.4 Biologically active compounds containing the pentafluoroethyl group do exist including the angiotensin II receptor antagonist DuP 532,5a antihypertensive potassium channel opener KC-515 (ref. 5b) and fulvestrant for treating breast cancer.5c The number of marketed drugs containing CF2CF3, however, is much lower than that of the CF3-containing drugs, despite some evidence pointing to the superior biological activities of the pentafluoroethylated congeners.6 Thus, new methods for synthesizing pentafluoroethylated molecules with diverse structures will be crucial for their future applications as therapeutic agents.7
The identification of accessible and easy-to-handle pentafluoroethylating reagents has been the bottleneck for developing pentafluoroethylation reactions. In this regard, pioneering works on the preparation and applications of transition-metal-based reagents (MCF2CF3; M = Cu,8 Zn,9 Ag,10 Pd,11 Ir12) have significantly broadened the reaction scope. Yet, with few exceptions, many of these reagents were not well-characterized and often in situ generated. For instance, Hu et al.8i,j,n,p and Ogoshi et al.8k reported the in situ generation of CuCF2CF3 using TMSCF3 and tetrafluoroethylene (TFE), respectively, and studied their reactions with aryl iodides. In terms of well-defined CuCF2CF3 complexes, several examples can be found in the literature (Scheme 1). Daugulis et al. first described the synthesis of [K(DMPU)3]+[(CF2CF3)CuCl]− from CuCl, KF and TMSCF3 in DMPU/THF (14% yield), which was characterized by NMR spectroscopy and X-ray crystallography (Scheme 1a).8q This anionic complex was a temperature and moisture sensitive solid that decomposed at room temperature under argon over few hours. Subsequently, Hartwig et al. reported the preparation of [(phen)CuCF2CF3] from [Cu(Mesityl)]5, t-BuOH, 1,10-phenanthroline (phen) and TMSCF2CF3 (Scheme 1b).8b This complex was effective for the pentafluoroethylation of arylboronate esters and heteroaryl bromides.8e Grushin et al. described the cupration of pentafluoroethane (HCF2CF3) gas with [K(DMF)][(t-BuO)2Cu] to generate the [K(DMF)2][(t-BuO)CuCF2CF3] complex with X-ray structural proof (Scheme 1c).8c A broad scope of pentafluoroethylation of aryl/vinyl iodides and bromides was demonstrated with this complex.8g Moreover, the same group prepared four [LnCuCF2CF3] complexes bearing different ligands (L) using [K(DMF)2][(t-BuO)CuCF2CF3] and obtained their X-ray crystal structures (Scheme 1d).8f The [(Ph3P)Cu(phen)CF2CF3] complex was shown to be useful for the pentafluoroethylation of acid chlorides.
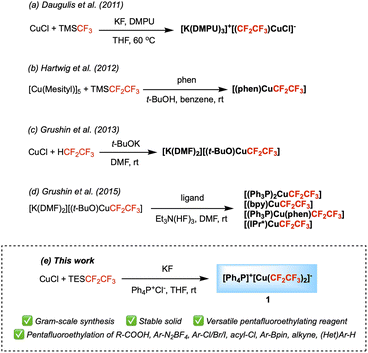 |
| Scheme 1 Preparation of well-defined [CuCF2CF3] complexes. | |
We herein describe the synthesis of a novel bispentafluoroethylated organocuprate [Ph4P]+[Cu(CF2CF3)2]−1 (Scheme 1e). This complex can be prepared on gram-scale and is a stable solid at room temperature under argon for months. More importantly, 1 has demonstrated a broad spectrum of reactivity as a versatile pentafluoroethylating reagent that is superior than previous CuCF2CF3 complexes. For instance, 1 was highly reactive towards not only aryl diazonium salts and iodides, but also difficult substrates such as aryl bromides and chlorides. In addition, 1 could be applied in challenging decarboxylative pentafluoroethylation, oxidative pentafluoroethylation of aryl pinacol boronic esters/alkynes, or even direct C–H bond pentafluoroethylation.
Results and discussion
We have recently reported the first preparation of [Ph4P]+[Cu(CF3)2]− complex13a from TMSCF3 and its usage in trifluoromethylation of organic halides with detailed mechanistic investigation.13b,c Subsequently, [Ph4P]+[Cu(CF2H)2]− was synthesized using TMSCF2H and proved to be a powerful difluoromethylating reagent.14 However, the pentafluoroethylated analogue remained unknown. We decided to use TESCF2CF3 as a potential pentafluoroethyl source for the synthesis of CuCF2CF3 complex (Scheme 2). The TESCF2CF3 was conveniently prepared from the low-cost pentafluoroethane (HFC-125, fire suppression agent/ozone-friendly refrigerant) and triethylchlorosilane on 350 mmol scale (Scheme 2a).15 When initially applying the original protocol for the preparation of [Ph4P]+[Cu(CF3)2]− complex13a using TESCF2CF3, only trace product was detected. By increasing the equivalents of TESCF2CF3 and KF with prolonged reaction time, the product formation was significantly improved. Thus, a two-step procedure was followed (Scheme 2b): (1) reacting CuCl with TESCF2CF3 (4.0 equiv.) and KF (8.0 equiv.) in THF at room temperature overnight; (2) adding tetraphenylphosphonium chloride Ph4P+Cl− (1.0 equiv.) at room temperature and stirring for 2 h. To our delight, the [Ph4P]+[Cu(CF2CF3)2]− complex 1 was successfully isolated in 92% yield (5.86 g, white solid) on 10 mmol scale. This complex was fully characterized by 1H, 19F and 31P NMR spectroscopy as well as high-resolution mass spectrometry. The structure was unambiguously confirmed by X-ray crystallography.
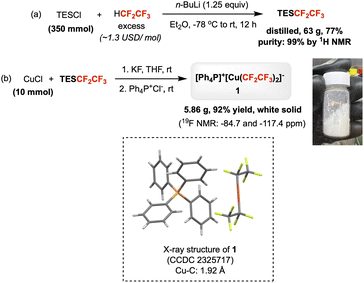 |
| Scheme 2 Synthesis of bispentafluoroethylated organocuprate [Ph4P]+[Cu(CF2CF3)2]−1. | |
Carboxylic acids are naturally abundant materials that are readily available, inexpensive and stable. As a result, decarboxylative cross-coupling of carboxylic acids has drawn tremendous attention in the synthetic community in recent years.16 The conversion of CO2H group to CF3via decarboxylative trifluoromethylation has emerged as a viable perfluoroalkylation strategy.17 To the best of our knowledge, decarboxylative pentafluoroethylation remains unknown. Li et al. pioneered the decarboxylative trifluoromethylation of aliphatic carboxylic acids using a Cu(III) complex (bpy)Cu(CF3)3 as the reagent.17a In their proposed mechanism, (bpy)Cu(CF3)3 reacts with ZnMe2 to generate the [Cu(CF3)3Me]− anion, which undergoes reductive elimination to form the key [Cu(CF3)2]− anion (Scheme 3a). In the presence of an oxidant, [CuI(CF3)2]− anion becomes CuII(CF3)2. Decarboxylation of carboxylic acid 2 would lead to the alkyl radical, in Li's report a silver(II) salt was employed for this purpose. This radical can react with the CuII(CF3)2 species to generate product 3 with the formation of a C–CF3 bond (Scheme 3b). We envisioned that our Cu(I) complex [Ph4P]+[Cu(CF2CF3)2]−1 can intercept this reaction pathway bypassing the reductive elimination from the Cu(III) anion to achieve decarboxylative pentafluoroethylation. However, the reactivity and compatibility of this complex in the decarboxylation conditions were unclear to us at the outset.
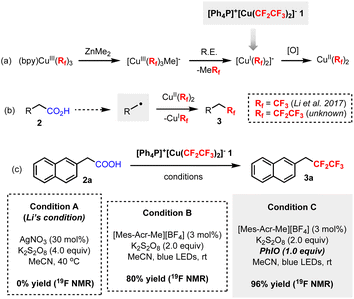 |
| Scheme 3 Initial investigation of decarboxylative pentafluoroethylation of aliphatic carboxylic acids using 1. | |
An initial attempt using Li's conditions (A)17a with 1 and 2a failed to provide any products (Scheme 3c). However, we were delighted to find that by using the photocatalyst 9-mesityl-10-methylacridinium tetrafluoroborate [Mes-Acr-Me][BF4] under blue light irradiation (condition B), the desired product 3a could be obtained in 80% yield. Although visible-light-induced decarboxylative functionalization of carboxylic acids has been well-studied,18a its application to perfluoroalkylation is limited.18b,c Extensive screening of reaction parameters including photocatalysts, oxidants, additives and solvents was carried out to improve the yield.19 It was found that adding a hypervalent iodine(III) reagent PhIO (iodosylbenzene)20 could enhance the yield to 96% (condition C).
The scope of aliphatic carboxylic acids 2 in the decarboxylative pentafluoroethylation using 1 was subsequently studied (Scheme 4). Under the optimized conditions, a variety of carboxylic acids containing benzylic CO2H group could be converted to the corresponding pentafluoroethylated products 3a–j. The reaction tolerated electron-donating (3d) and electron-withdrawing (3j) aryl substituents. Halogens including fluoro (3f), chloro (3g), bromo (3h) and iodo (3i) groups were also compatible. A ɣ-keto acid was suitable substrate in the reaction (3k). Moreover, the anti-inflammatory agent Isoxepac could be pentafluoroethylated in excellent yield (3l). However, a secondary carboxylic acid only gave a low yield.
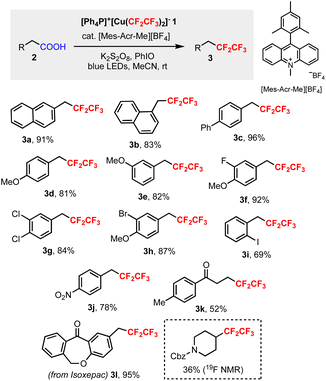 |
| Scheme 4 Scope of decarboxylative pentafluoroethylation using 1.a aGeneral conditions: 1 (0.2 mmol), 2 (0.4 mmol), [Mes-Acr-Me][BF4] (3 mol%), K2S2O8 (0.4 mmol), PhIO (0.2 mmol), MeCN (2.0 mL), blue LEDs, rt for 12 h under argon. Isolated yields based on 1. | |
Based on literature evidence,18c the following plausible reaction mechanism is proposed for the decarboxylative pentafluoroethylation of 2 with reagent 1 (see the ESI† for proposed mechanisms and control experiments). The photocatalyst [Mes-Acr-Me]+ is activated by visible light, which then undergoes single-electron oxidation of carboxylate R–CH2–COO− (from 2) to give acyloxyl radical R–CH2–COO˙ and generate the [Mes-Acr-Me]˙ species. Decarboxylation of R–CH2–COO˙ leads to
alkyl radical. Meanwhile, [CuI(CF2CF3)2]−1 is oxidized to CuII(CF2CF3)2 which then reacts with the
radical to form the R–CH2–CF2CF3 product 3 (cf.Scheme 3b).17a Finally, [Mes-Acr-Me]˙ is oxidized via SET to regenerate the catalyst [Mes-Acr-Me]+. Adding PhIO can lead to the formation of phenyliodine(III) dicarboxylates (RCH2COO)2IPh, which are known to undergo decarboxylation in the presence of copper(I) complexes to generate
alkyl radical.20 It is possible that both pathways contributed to the formation of 3 from 2.
Arenediazonium salts are useful substrates for Sandmeyer-type trifluoromethylation and can be made from inexpensive aromatic amines.21 However, the corresponding pentafluoroethylation is far less explored. One report in the literature by Hu et al.8n described the copper-mediated pentafluoroethylation of arenediazonium tetrafluoroborates with in situ generated tetrafluoroethylene (TFE) from TMSCF3 and NaI. By adding CuSCN and CsF to TFE, the “CuCF2CF3” species is formed which can react with the diazonium salt.22 We found that 1 was remarkably reactive with arenediazonium salts 4 even at −20 °C (Scheme 5).23 Within 30 min, various arenediazonium tetrafluoroborates could be converted directly to pentafluoroethyl arenes 5 in good yields. The reaction exhibited good functional group tolerability towards halo (5b, 5c), amine (5d), sulfone (5e), nitro (5f), ketone (5g), ester (5h) and heteroaryl (5i) groups. This protocol was operationally straightforward without the use of separate reagents/additives and the conditions were mild and fast.
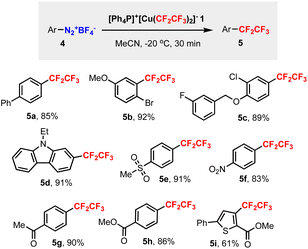 |
| Scheme 5 Pentafluoroethylation of arenediazonium tetrafluoroborates using 1.a aGeneral conditions: 1 (0.2 mmol), 4 (0.2 mmol), MeCN (2.0 mL), under argon. Isolated yields. | |
(Hetero)aryl halides could be pentafluoroethylated using 1 based on analogous trifluoromethylation conditions13a (Scheme 6). Aryl iodides were quite reactive within 12 h to provide products 5a, 5j–l in good yields. An example of alkenyl iodide in pentafluoroethylation was also demonstrated (5m). Aryl and heteroaryl bromides gave products 5a, 5n–q in good yields after 24 h. In contrast, aryl chlorides were much less reactive than aryl iodide and aryl bromide, often were inert in previously reported pentafluoroethylation protocols.24 Actually, reaction of unactivated 4-biphenyl chloride with 1 did not generate compound 5a at all. However, activated heteroaryl chlorides containing isoquinoline (5r), quinoline (5s), quinoxaline (5t), pyrimidine (5u), triazine (5q), nicotinate (5v–x), benzothiazole (5y) and benzoxazole (5z) cores did react with reagent 1 to afford pentafluoroethylated heteroarenes in 73–92% yields. Intriguingly, an acid chloride (2-naphthoyl chloride) was able to generate the pentafluoroethylated ketone 5aa at lower temperature (60 °C) without the CuI additive.8f
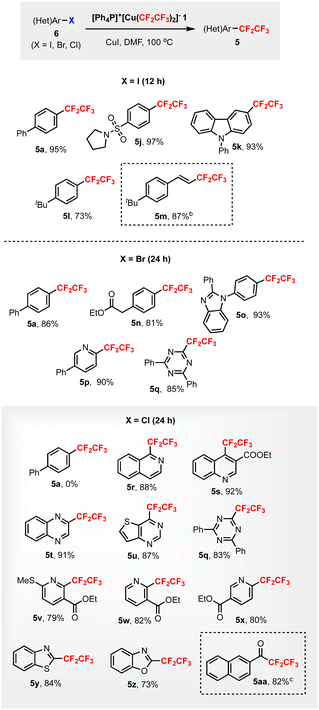 |
| Scheme 6 Pentafluoroethylation of (hetero)aryl halides using 1.a aGeneral conditions: 1 (0.3 mmol), 6 (0.2 mmol), CuI (0.2 mmol), DMF (2.0 mL), under argon. Isolated yields. bdr = 17 : 1 from starting alkenyl iodide. cConditions: 2-naphthoyl chloride (0.2 mmol), 1 (0.3 mmol), THF (2.0 mL), 60 °C for 12 h, under argon. | |
Furthermore, reagent 1 was effective in oxidative pentafluoroethylation of aryl boronic esters 7 and terminal alkynes 8 under mild conditions (Scheme 7).25 Thus, pentafluoroethylated arenes 5 were synthesized from the corresponding aryl-Bpin tolerating aldehyde, ester, amide, nitro and nitrile groups. An alkenyl-Bpin substrate also gave the product (5ah). Readily available terminal alkynes were directly converted to pentafluoroethylated internal alkynes 9a–g showing good functional group tolerance. The simple operations (open to air) and the use of air as a green oxidant were the advantages of these reactions.
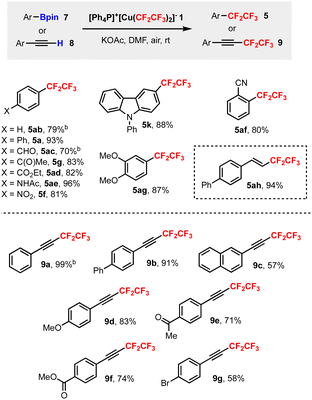 |
| Scheme 7 Aerobic pentafluoroethylation of boronic esters and terminal alkynes using 1.a aGeneral conditions using boronic esters: 1 (0.3 mmol), 7 (0.2 mmol), KOAc (0.3 mmol), DMF (2.0 mL), open to air, 12 h. Isolated yields. General conditions using terminal alkynes: 1 (0.24 mmol), 8 (0.2 mmol), KOAc (0.3 mmol), DMF (2.0 mL), open to air, 12 h. Isolated yields. bYield determined by 19F NMR using benzotrifluoride as the internal standard. | |
The direct conversion of C–H bonds to C–Rf bonds bypassing the requirement of prefunctionalized substrates is a very desirable strategy. Trifluoromethylation of arene C–H bonds has been reported by Zhang et al. using a high-valent Cu(III)-CF3 complex.26 However, to the best of our knowledge, the corresponding pentafluoroethylation using copper-based reagents is unknown. In the literature, the C–H bond pentafluoroethylation has been achieved by employing nickel catalysts,27a,b cobalt complexes27c and photocatalytic methods.27d,e We discovered that our CuCF2CF3 complex 1 was capable of pentafluoroethylating arenes and heteroarenes in the presence of an oxidant (Scheme 8). Different oxidants including K2S2O8, PIDA, Selectfluor, Oxone and NFSI were screened and NFSI showed the best reactivity.19 Thus, at room temperature, aromatic C–H bonds could be converted to C–CF2CF3 bonds (5ag, 5ai–5al). Heteroaromatic C–H bonds including pyrazine (5am), pyridine (5an), thiophene (5ao) and furan (5ap) were also reactive. Interestingly, the C–H bond of caffeine could be smoothly functionalized to afford the pentafluoroethylated caffeine 5aq. Moreover, the alkenyl C–H bond pentafluoroethylation was achieved to give compound 5ar and an uracil derivative 5as. It was found that the electron-rich arenes were more reactive than the electron-poor ones. In comparison with 1,4-dimethoxybenzene (5ai, 65%), 1,4-dibromobenzene (14%) and 1,4-dinitrobenzene (<5%) gave low yields even at elevated temperature (60 °C in DCE). This is likely due to the electrophilic nature of the pentafluoroethyl radical generated in situ, which is more reactive towards the electron-rich arenes.
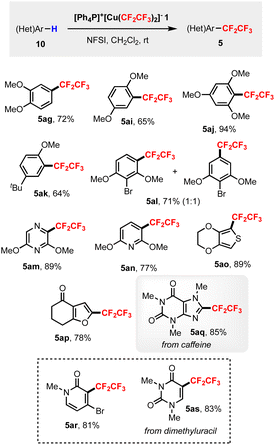 |
| Scheme 8 Oxidative pentafluoroethylation of (hetero)aromatic C–H bond using 1.a aGeneral conditions: 1 (0.2 mmol), 10 (1.0 mmol), NFSI (0.6 mmol), CH2Cl2 (2.0 mL), under argon, 12 h. Isolated yields. | |
Conclusions
In conclusion, we have synthesized and characterized a novel [Ph4P]+[Cu(CF2CF3)2]− complex 1. This complex proved to be a highly versatile pentafluoroethylating reagent. A diverse array of pentafluoroethylation reactions of carboxylic acids, diazonium salts, organic halides, boronic esters, terminal alkynes and (hetero)arenes were developed using 1. Therefore, one reagent was capable of constructing all C(sp3)–, C(sp2) and C(sp)–CF2CF3 bonds. It is worth mentioning that the [Ph4P]+[Cu(CF2CF3)2]− complex 1 has demonstrated superior reactivities than its [Ph4P]+[Cu(CF3)2]− counterpart.19 For instance, pentafluoroethylation of carboxylic acid, diazonium salt, arene C–H bond and heteroaryl chloride using 1 gave significantly higher yields than the corresponding trifluoromethylation with [Ph4P]+[Cu(CF3)2]−. Further transformations of 1 and studies of reaction mechanisms are ongoing in our laboratories.
Data availability
The data supporting this article have been included as part of the ESI.†
Author contributions
The manuscript was written through the contributions of all authors. All authors have approved the final version of the manuscript.
Conflicts of interest
There are no conflicts to declare.
Acknowledgements
This work was supported by the Research Grants Council of Hong Kong (NSFC/RGC Joint Research Scheme, N_CUHK403/20), the Chinese University of Hong Kong (Faculty of Science – Direct Grant for Research), the National Key Research and Development Program of China (No. 2021YFF0701700), the National Natural Science Foundation of China (22061160465) and the Strategic Priority Research Program of the Chinese Academy of Sciences (XDB0590000). We also thank the Key Laboratory of Organofluorine Chemistry, Shanghai Institute of Organic Chemistry, Chinese Academy of Sciences for funding.
Notes and references
- Representative reviews:
(a) K. Müller, C. Faeh and F. Diederich, Science, 2007, 317, 1881 CrossRef PubMed;
(b) S. Purser, P. R. Moore, S. Swallow and V. Gouverneur, Chem. Soc. Rev., 2008, 37, 320 RSC;
(c) D. O'Hagan, Chem. Soc. Rev., 2008, 37, 308 RSC;
(d) T. Furuya, A. S. Kamlet and T. Ritter, Nature, 2011, 473, 470 CrossRef CAS PubMed;
(e) J. Wang, M. J. Sánchez-Roselló, L. Aceña, C. del Pozo, A. E. Sorochinsky, S. Fustero, V. A. Soloshonok and H. Liu, Chem. Rev., 2014, 114, 2432 CrossRef CAS PubMed;
(f) M. Inoue, Y. Sumii and N. Shibat, ACS Omega, 2020, 5, 10633 CrossRef CAS PubMed;
(g) N. Wang, H. Mei, G. Dhawan, W. Zhang, J. Han and V. A. Soloshonok, Molecules, 2023, 28, 3651 CrossRef CAS PubMed.
- A recent review: R. Britton, V. Gouverneur, J.-H. Lin, M. Meanwell, C. Ni, G. Pupo, J.-C. Xiao and J. Hu, Nat. Rev. Methods Primers, 2021, 1, 47 CrossRef CAS.
- Representative reviews on trifluoromethylation:
(a) O. A. Tomashenko and V. V. Grushin, Chem. Rev., 2011, 111, 4475 CrossRef CAS PubMed;
(b) T. Liu and Q. Shen, Eur. J. Org. Chem., 2012, 2012, 6679 CrossRef CAS;
(c) T. Liang, C. N. Neumann and T. Ritter, Angew. Chem., Int. Ed., 2013, 52, 8214 CrossRef CAS PubMed;
(d) L. Chu and F.-L. Qing, Acc. Chem. Res., 2014, 47, 1513 CrossRef CAS PubMed;
(e) C. Alonso, E. Martínez de Marigorta, G. Rubiales and F. Palacios, Chem. Rev., 2015, 115, 1847 CrossRef CAS PubMed;
(f) C. Ni, M. Hu and J. Hu, Chem. Rev., 2015, 115, 765 CrossRef CAS PubMed;
(g) X. Yang, T. Wu, R. J. Phipps and F. D. Toste, Chem. Rev., 2015, 115, 826 CrossRef CAS PubMed;
(h) T. Chatterjee, N. Iqbal, Y. You and E. J. Cho, Acc. Chem. Res., 2016, 49, 2284 CrossRef CAS PubMed;
(i) X. Yang and G. C. Tsui, Synlett, 2022, 33, 713 CrossRef CAS.
- A topic search on SciFinder during the preparation of this manuscript using “trifluoromethylation” and “pentafluoroethylation” as keywords revealed ca. 31
000 vs. 800 references, respectively.
-
(a) A. T. Chiu, D. J. Carini, J. V. Duncia, K. H. Leung, D. E. McCall, J. Price, A. William, P. C. Wong, R. D. Smith, R. R. Wexler and P. B. M. W. M. Timmermans, Biochem. Biophys. Res. Commun., 1991, 177, 209 CrossRef CAS PubMed;
(b) N. Taka, H. Koga, H. Sato, T. Ishizawa, T. Takahashi and J. Imagawa, Bioorg. Med. Chem., 2000, 8, 1393 CrossRef CAS PubMed;
(c) I. Vergote and P. Abram, Ann. Oncol., 2006, 17, 200 CrossRef CAS PubMed.
-
(a) M. Andrzejewska, L. Yépez-Mulia, R. Cedillo-Rivera, A. Tapia, L. Vilpo, J. Vilpo and Z. Kazimierczuk, Eur. J. Med. Chem., 2002, 37, 973 CrossRef CAS PubMed;
(b) A. Johansson, A. Poliakov, E. Åkerblom, K. Wiklund, G. Lindeberg, S. Winiwarter, U. H. Danielson, B. Samuelsson and A. Hallberg, Bioorg. Med. Chem., 2003, 11, 2551 CrossRef CAS PubMed;
(c) G. Kokotos, Y.-H. Hsu, J. E. Burke, C. Baskakis, C. G. Kokotos, V. Magrioti and E. A. Dennis, J. Med. Chem., 2010, 53, 3602 CrossRef CAS PubMed;
(d) C. Philippe, J. Kaffy, T. Milcent and D. Bonnet-Delpon, J. Fluorine Chem., 2012, 134, 136 CrossRef CAS;
(e) F. Pertusati, S. Ferla, M. Bassetto, A. Brancale, S. Khandil, A. Westwell and C. McGuigan, Eur. J. Med. Chem., 2019, 180, 1 CrossRef CAS PubMed.
- We have a continuing interest in developing pentafluoroethylation reactions. For our previous works, see:
(a) T. Dong, Y. Tang and G. C. Tsui, Org. Chem. Front., 2023, 10, 5092 RSC;
(b) Z. Fu, X. Yang and G. C. Tsui, Org. Lett., 2023, 25, 4945 CrossRef CAS PubMed;
(c) Z. Luo and G. C. Tsui, Org. Chem. Front., 2022, 9, 4969 RSC;
(d) X. Yang and G. C. Tsui, Org. Lett., 2020, 22, 4562 CrossRef CAS PubMed.
- [CuCF2CF3]:
(a) M. M. Kremlev, W. Tyrra, A. I. Mushta, D. Naumann and Y. L. Yagupolskii, J. Fluorine Chem., 2010, 131, 212 CrossRef CAS;
(b) N. D. Litvinas, P. S. Fier and J. F. Hartwig, Angew. Chem., Int. Ed., 2012, 51, 536 CrossRef CAS PubMed;
(c) A. Lishchynskyi and V. V. Grushin, J. Am. Chem. Soc., 2013, 135, 12584 CrossRef CAS PubMed;
(d) H. Serizawa, K. Aikawa and K. Mikami, Org. Lett., 2014, 16, 3456 CrossRef CAS PubMed;
(e) M. G. Mormino, P. S. Fier and J. F. Hartwig, Org. Lett., 2014, 16, 1744 CrossRef CAS PubMed;
(f) L. I. Panferova, F. M. Miloserdov, A. Lishchynskyi, M. Martinez Belmonte, J. BenetBuchholz and V. V. Grushin, Angew. Chem., Int. Ed., 2015, 54, 5218 CrossRef CAS PubMed;
(g) A. Lishchynskyi, Z. Mazloomi and V. V. Grushin, Synlett, 2015, 26, 45 CAS;
(h) K. Negishi, K. Aikawa and K. Mikami, Eur. J. Org. Chem., 2016, 23, 4099 CrossRef;
(i) L. Li, C. Ni, Q. Xie, M. Hu, F. Wang and J. Hu, Angew. Chem., Int. Ed., 2017, 56, 9971 CrossRef CAS PubMed;
(j) Q. Xie, L. Li, Z. Zhu, R. Zhang, C. Ni and J. Hu, Angew. Chem., Int. Ed., 2018, 57, 13211 CrossRef CAS PubMed;
(k) M. Ohashi, N. Ishida, K. Ando, Y. Hashimoto, A. Shigaki, K. Kikushima and S. Ogoshi, Chem.–Eur. J., 2018, 24, 9794 CrossRef CAS PubMed;
(l) J. Mestre, S. Castillón and O. Boutureira, J. Org. Chem., 2019, 84, 15087 CrossRef CAS PubMed;
(m) E. D. Kalkman, M. G. Mormino and J. F. Hartwig, J. Am. Chem. Soc., 2019, 141, 19458 CrossRef CAS PubMed;
(n) B. Xing, L. Li, C. Ni and J. Hu, Chin. J. Chem., 2019, 37, 1131 CrossRef CAS;
(o) F. Ye, F. Berger, H. Jia, J. Ford, A. Wortman, J. Börgel, C. Genicot and T. Ritter, Angew. Chem., Int. Ed., 2019, 58, 14615 CrossRef CAS PubMed;
(p) S. Pan, Q. Xie, X. Wang, Q. Wang, C. Ni and J. Hu, Chem. Commun., 2022, 58, 5156 RSC;
(q) I. Popov, S. Lindeman and O. Daugulis, J. Am. Chem. Soc., 2011, 133, 9286 CrossRef CAS PubMed.
- [ZnCF2CF3]:
(a) I. Popov, S. Lindeman and O. Daugulis, J. Am. Chem. Soc., 2011, 133, 9286 CrossRef CAS PubMed;
(b) K. Aikawa, Y. Nakamura, Y. Yokota, W. Toya and K. Mikami, Chem.–Eur. J., 2015, 21, 96 CrossRef CAS PubMed;
(c) X. Bao, L. Liu, J. Li and S. Fan, J. Org. Chem., 2018, 83, 463 CrossRef CAS PubMed;
(d) X. Zeng, W. Yan, M. Paeth, S. B. Zacate, P.-H. Hong, Y. Wang, D. Yang, K. Yang, T. Yan, C. Song, Z. Cao, M.-J. Cheng and W. Liu, J. Am. Chem. Soc., 2019, 141, 19941 CrossRef CAS PubMed;
(e) L. Liu, X. Bao, H. Xiao, J. Li, F. Ye, C. Wang, Q. Cai and S. Fan, J. Org. Chem., 2019, 84, 423 CrossRef CAS PubMed.
- [AgCF2CF3]:
(a) Y. Zeng and J. Hu, Chem.–Eur. J., 2014, 20, 6866 CrossRef CAS PubMed;
(b) M.-L. Fu, J.-B. Liu, X.-H. Xu and F.-L. Qing, J. Org. Chem., 2017, 82, 3702 CrossRef CAS PubMed;
(c) J.-X. Xiang, Y. Ouyang, X.-H. Xu and F.-L. Qing, Angew. Chem., Int. Ed., 2019, 58, 10320 CrossRef CAS PubMed.
- [PdCF2CF3]:
(a) A. Maleckis and M. S. Sanford, Organometallics, 2014, 33, 3831 CrossRef CAS;
(b) D. M. Ferguson, J. R. Bour, A. J. Canty, J. W. Kampf and M. S. Sanford, J. Am. Chem. Soc., 2017, 139, 11662 CrossRef CAS PubMed.
- [IrCF2CF3]: C. J. Bourgeois, R. P. Hughes, J. Yuan, A. G. Di Pasquale and A. L. Rheingold, Organometallics, 2006, 25, 2908 CrossRef CAS.
-
(a) H. Liu and Q. Shen, Org. Chem. Front., 2019, 6, 2324 RSC;
(b) H. Liu, J. Wu, Y. Jin, X. Leng and Q. Shen, J. Am. Chem. Soc., 2021, 143, 14367 CrossRef CAS PubMed;
(c) Y. Luo, Y. Li, J. Wu, X.-S. Xue, J. F. Hartwig and Q. Shen, Science, 2023, 381, 1072 CrossRef CAS PubMed.
- H. Zhao, X. B. Leng, W. Zhang and Q. Shen, Angew. Chem., Int. Ed., 2022, 61, e202210151 CrossRef CAS PubMed.
- For a literature procedure on the preparation of TESCF2CF3 from HCF2CF3, see: D. Katayev, J. Vaclavk, F. Bruning, B. Commarea and A. Togni, Chem. Commun., 2016, 52, 4049 RSC . The corresponding TMSCF2CF3 is commercially available, however, at exorbitant prices..
- Representative reviews:
(a) N. Rodríguez and L. J. Goossen, Chem. Soc. Rev., 2011, 40, 5030 RSC;
(b) T. Patra and D. Maiti, Chem.–Eur. J., 2017, 23, 7382 CrossRef CAS PubMed;
(c) G. Laudadio, M. D. Palkowitz, T. E.-H. Ewing and P. S. Baran, ACS Med. Chem. Lett., 2022, 13, 1413 CrossRef CAS PubMed.
- Representative examples:
(a) X. Tan, Z. Liu, H. Shen, P. Zhang, Z. Zhang and C. Li, J. Am. Chem. Soc., 2017, 139, 12430 CrossRef CAS PubMed;
(b) J. A. Kautzky, T. Wang, R. W. Evans and D. W. C. MacMillan, J. Am. Chem. Soc., 2018, 140, 6522 CrossRef CAS PubMed ; For a review, see:;
(c) A. Monfared, S. Ebrahimiasl, M. Babazadeh, S. Arshadi and E. Vessally, J. Fluorine Chem., 2019, 220, 24 CrossRef CAS.
- A review:
(a) J. Xuan, Z. Zhang and W. Xiao, Angew. Chem., Int. Ed., 2015, 54, 15632 CrossRef CAS PubMed ; Trifluoromethylation:;
(b) P. Xu, A. Abdukader, K. Hu, Y. Cheng and C. Zhu, Chem. Commun., 2014, 50, 2308 RSC ; Trifluoromethylselenolation:;
(c) Q.-Y. Han, K.-L. Tan, H.-N. Wang and C.-P. Zhang, Org. Lett., 2019, 21, 10013 CrossRef CAS PubMed.
- See the ESI† for details.
- Z. Li, G. Zhang, Y. Song, M. Li, Z. Li, W. Ding and J. Wu, Org. Lett., 2023, 25, 3023 CrossRef CAS PubMed.
- Representative examples:
(a) X. Wang, Y. Xu, F. Mo, G. Ji, D. Qiu, J. Feng, Y. Ye, S. Zhang, Y. Zhang and J. Wang, J. Am. Chem. Soc., 2013, 135, 10330 CrossRef CAS PubMed;
(b) J.-J. Dai, C. Fang, B. Xiao, J. Yi, J. Xu, Z.-J. Liu, X. Lu, L. Liu and Y. Fu, J. Am. Chem. Soc., 2013, 135, 8436 CrossRef CAS PubMed;
(c) A. Lishchynskyi, G. Berthon and V. V. Grushin, Chem. Commun., 2014, 50, 10237 RSC;
(d) G. Danoun, B. Bayarmagnai, M. F. Grunberg and L. J. Goossen, Angew. Chem., Int. Ed., 2013, 52, 7972 CrossRef CAS PubMed.
- For a related transformation (3 examples) using “NiCF2CF3”, see: S. T. Shreiber and D. A. Vicic, Angew. Chem., Int. Ed., 2021, 60, 18162 CrossRef CAS PubMed.
- At higher temperatures, e.g., 0 °C and rt, bis-pentafluoroethylated side products were observed.
- Pentafluoroethylation of heteroaryl chlorides is very rare. Most literature examples use (het)aryl iodides and bromides, see ref. 8c, 8e and 8j.
- For related transformations, see ref. 8b and 8p, phen ligand was required for the CuCF2CF3 complex for the pentafluoroethylation of boronic esters.
- H. Zhang, C. Feng, N. Chen and S. Zhang, Angew. Chem., Int. Ed., 2022, 61, e202209029 CrossRef CAS PubMed.
-
(a) S. Deolka, R. Govindarajan, T. Gridneva, M. C. Roy, S. Vasylevskyi, P. K. Vardhanapu, J. R. Khusnutdinova and E. Khaskin, ACS Catal., 2023, 13, 13127 CrossRef CAS;
(b) S. Deolka, R. Govindarajan, S. Vasylevskyi, M. C. Roy, J. R. Khusnutdinova and E. Khaskin, Chem. Sci., 2022, 13, 12971 RSC;
(c) H. M. Dinh, R. Govindarajan, S. Deolka, R. R. Fayzullin, S. Vasylevskyi, E. Khaskin and J. R. Khusnutdinova, Organometallics, 2023, 42, 2632 CrossRef CAS;
(d) M. Zhang, J. Chen, S. Huang, B. Xu, J. Lin and W. Su, Chem. Catal., 2022, 2, 1793 CrossRef CAS;
(e) J. W. Beatty, J. J. Douglas, R. Miller, R. C. McAtee, K. P. Cole and C. R. J. Stephenson, Chem, 2016, 1, 456 CrossRef CAS PubMed.
|
This journal is © The Royal Society of Chemistry 2024 |
Click here to see how this site uses Cookies. View our privacy policy here.