DOI:
10.1039/D4SC00056K
(Edge Article)
Chem. Sci., 2024,
15, 3670-3672
The power of microdroplet photochemistry†
Received
4th January 2024
, Accepted 1st February 2024
First published on 1st February 2024
Abstract
This study presents compelling evidence demonstrating that irradiation of the air–solution interface, whether achieved through the spraying of microdroplets into the air or by bubbling air through a solution, significantly accelerates the rate of photochemical reactions by orders of magnitude compared to identical reaction conditions in bulk solutions. We propose this approach as a novel and versatile method for harnessing solar energy in chemical transformations.
While most photochemical reactions are conventionally conducted in laboratory settings using liquid samples, this approach poses notable challenges. In bulk solutions, uneven distribution of radiation arising from absorption is a common issue, often addressed through microflow reactors that limit the thickness (pathlength) of the liquid sample.1 However, the presence of solvent introduces sluggishness to the process. Photoexcited molecules frequently collide with surrounding solvent molecules, dissipating absorbed energy before chemical reactions can occur. Furthermore, even if a photochemical reaction does take place, the resulting photofragments are often confined by solvent molecules, leading to undesired recombination. In response to these challenges, we propose an alternative approach involving the irradiation of sprayed microdroplets. This method exhibits remarkable advantages in accelerating photochemical reaction rates, as demonstrated in a recent study on the photochemical oxidation of methyl orange dissolved in water droplets containing ferric oxalate,2,3 and in a study on the photoionization of phenol at the air–water interface.4
An illustrative case is the renowned Paternò–Büchi reaction, involving a photochemical [2 + 2] cycloaddition of a carbonyl with an olefin, producing an oxetane that can subsequently undergo decomposition upon collision. Historically, this photochemical reaction has proven valuable for pinpointing the positions of double bonds in alkenes,5–7 a topic of considerable interest in the field of lipidomics.8
To delve into specifics, let's examine the Paternò–Büchi reaction involving oleic acid (cis-9-octadecenoic acid), as illustrated in Fig. 1a. The resulting oxetane undergoes efficient decomposition upon collisions with helium inside the mass spectrometer. Subsequent analysis of the photofragments using tandem mass spectrometry (Fig. 1b) provides a straightforward method for determining the precise location of the double bond within the oleic acid molecule.
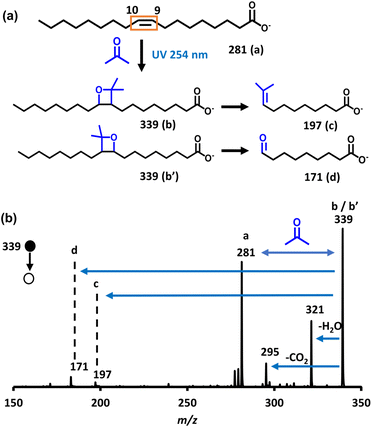 |
| Fig. 1 Using the Paternò–Büchi reaction to locate the C C double bond position in oleic acid by detection of photofragments by means of mass spectrometry. (a) Process of the photocatalytic cycloaddition between acetone and oleic acid and follow-up fragmentation of the product ion in the collision-induced dissociation experiment; (b) the MS/MS spectrum to generate diagnostic ions for confirmation of the double bond position. The solid black circle denotes the parent ion and the open circle the resulting fragment ion. | |
This experiment was conducted on a 2 mL sample comprising 5 μM sodium oleate dissolved in a 2 mL solution of water and acetone in a 10
:
1 ratio. Utilizing a 254 nm UV light source (mercury lamp), we repeated the experiment employing a straightforward setup depicted in Fig. 2. The resulting photoproduct yield is also illustrated in Fig. 2.
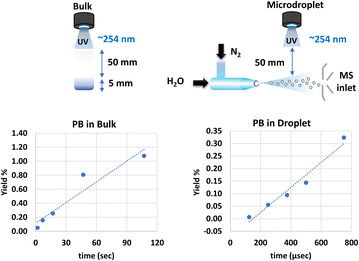 |
| Fig. 2 Photochemistry performance comparison. Diagram of photocatalytic cycloaddition reaction processed in bulk water (top left) and with water microdroplets (top right); product yield vs. reaction time for the bulk solution (bottom left) and microdroplet spray (bottom right). The intensity of the incident 254 nm radiation is made identical in both cases. | |
The outcomes are immediately evident, photochemistry conducted on sprayed droplets, with an average diameter of ∼10 μm as determined by light scattering, yields identical products, but at a rate exceeding 104 times faster. Details of this determination are presented in the ESI.† The ability to execute photochemical reactions within sprayed droplets suggests the potential for significant advantages. This observation forms the basis for terming this phenomenon “the power of microdroplet photochemistry.”
The pronounced acceleration prompts curiosity about its underlying causes. We posit that multiple factors contribute to this phenomenon. Firstly, a water microdroplet functions as a spherical lens, concentrating incident radiation at the air–water interface (AWI).9 Another contributing factor is the substantial increase in the surface-to-volume ratio of microdroplets compared to bulk liquid. While this can accelerate reactions primarily utilizing O2 in the air, as seen in methyl orange oxidation,2,3 it is noteworthy that this mechanism does not apply to the Paternò–Büchi reaction. Instead, various factors come into play. Oleic acid's limited solubility in water results in the concentration of oleic acid molecules at the AWI of the microdroplet. At the AWI, a robust electric field arises from the differential adsorption of positive and negative ions in the water–acetone solution by the hydrophobic air enveloping the microdroplet.10 This electric field, coupled with partial solvation of molecules at the AWI, fosters reactivity through radical creation, electron transfer, O2− formation from O2 in the air, and molecular alignment at the AWI.11–13 It's crucial to acknowledge that different mechanisms are anticipated to dominate in diverse photochemical reactions.14
Upon acknowledging the pivotal role that the AWI plays in enhancing photochemical reactions, it becomes apparent that various avenues exist to harness its potential in photochemistry. Alternative approaches, such as employing thin films15 or opting for microbubbles instead of microdroplets,16–18 provide additional means to leverage the power of the AWI. Notably, the use of microbubbles may offer specific advantages, facilitating a relatively straightforward method for scaling up photoreactions.19
Conclusions
In summary, it is sufficient to emphasize that the surface of a water-containing microdroplet exhibits remarkable reactivity, enhancing photochemical yields for various reasons for any irradiation time at a given intensity. This assertion is substantiated by our demonstration, comparing the photoproduct yield in the Paternò–Büchi photochemical reaction of oleic acid with acetone under identical radiation intensity and duration. While our current study utilized UV light, recent experiments indicate the feasibility of achieving the same Paternò–Büchi reaction with visible light.3 The potential practical value of microdroplet photochemistry becomes particularly compelling if sunlight can serve as the light source, considering that solar energy striking the Earth exceeds the world's total energy usage by about ten thousand times. We advocate for further extensive studies to fully assess the promise of microdroplet photochemistry as the observed benefits appear to be broadly applicable.
Data availability
Data are available from ESI.†
Author contributions
XS and RNZ carried out the experiments and both authors checked the analysis of the results and wrote this manuscript together.
Conflicts of interest
The authors declare that they have no conflicts of interest.
Acknowledgements
This work is supported by the Air Force Office of Scientific Research through the Multidisciplinary University Research Initiative (MURI) program (AFOSR FA9550-21-1-0170).
Notes and references
- C. Sambiagio and T. Noël, Flow photochemistry: Shine some light on those tubes, Trends Chem., 2020, 2, 92–106 CrossRef CAS
.
- Y. Wang, M. Brigante, G. Mailhot, D. Talaga, Y. Wu, W. Dong and S. Sobanska, Toward a better understanding of ferric-oxalate complex photolysis: The role of the aqueous/air interface of droplet, Chemosphere, 2022, 289, 133127 CrossRef CAS PubMed
.
- K. Li, K. Gong, J. Liu, L. Ohnoutek, J. Ao, Y. Liu, X. Chen, G. Xu, X. Ruan, H. Cheng, J. Han, G. Sui, M. Ji, V. K. Valev and L. Zhang, Significantly accelerated photochemical and photocatalytic reactions in microdroplets, Cell Rep. Phys. Sci., 2022, 3, 100917 CrossRef CAS
.
- R. Kusaka, S. Nihonyanagi and T. Tahara, The photochemical reaction of phenol becomes ultrafast at the air–water interface, Nat. Chem., 2021, 13, 306–311 CrossRef CAS PubMed
.
- K. A. Rykaczewski and C. S. Schindler, Visible-light-enabled Paterno–Buchi reaction via triplet energy transfer for the synthesis of Oxetanes, Org. Lett., 2020, 22, 6516–6519 CrossRef CAS PubMed
.
- X. Ma and Y. Xia, Pinpointing double bonds in lipids by Paternò-Büchi reactions and mass spectrometry, Angew. Chem., 2014, 53, 2592–2596 CrossRef CAS PubMed
.
- K. Luo, H. Chen and R. N. Zare, Location of Carbon-Carbon Double Bonds in Unsaturated Lipids Using Microdroplet Mass Spectrometry, Analyst, 2021, 146, 2550–2558 RSC
.
- S. Tang, H. Cheng and X. Yan, On-demand electrochemical epoxidation in nano-electrospray ionization mass spectrometry to locate carbon–carbon double bonds, Angew. Chem., Int. Ed., 2020, 59, 209–214 CrossRef CAS PubMed
.
- Y. Bai, P. Luan, Y. Bai, R. N. Zare and J. Ge, Enzyme-photo-coupled catalysis in gas-sprayed microdroplets, Chem. Sci., 2022, 13, 8341–8348 RSC
.
- H. Xiong, J. K. Lee, R. N. Zare and W. Min, Strong Electric Field Observed at the Interface of Aqueous Microdroplets, J. Phys. Chem. Lett., 2020, 11, 7423–7428 CrossRef CAS PubMed
.
- A. J. Colussi, Mechanism of Hydrogen Peroxide Formation on Sprayed Water Microdroplets, J. Am. Chem. Soc., 2023, 145, 16315–16317 CrossRef CAS PubMed
.
- X. Chen, Y. Xia, Z. Zhang, L. Hua, X. Jia, F. Wang and R. N. Zare, Hydrocarbon degradation by contact with anoxic water microdroplets, J. Am. Chem. Soc., 2023, 145, 21538–21545 CrossRef CAS PubMed
.
- Z. Zhou, X. Yan, Y.-H. Lai and R. N. Zare, Fluorescence Polarization Anisotropy in Microdroplets, J. Phys. Chem. Lett., 2018, 9, 2928–2932 CrossRef CAS PubMed
.
- Z. Wei, Y. Li, R. G. Cooks and X. Yan, Accelerated reaction kinetics in microdroplets: Overview and recent developments, Annu. Rev. Phys. Chem., 2020, 71, 31–51 CrossRef CAS PubMed
.
- J. Li, Y. Xia, J. Li, K. Long, F. Chen, M. Feng, S. Guo and B. Chen, Localized photo-Fenton reaction boosted by spontaneous generation of H2O2 from thin water membrane, J. Water Process. Eng., 2023, 52, 103521 CrossRef
.
- T. Tasaki, T. Wada, K. Fujimoto, S. Kai, K. Ohe, T. Oshima, Y. Baba and M. Kukizaki, Degradation of methyl orange using short-wavelength UV irradiation with oxygen microbubbles, J. Hazard. Mater., 2009, 162, 1103–1110 CrossRef CAS PubMed
.
- W. Fan, J. Cui, Q. Li, Y. Huo, D. Xiao, X. Yang, H. Yu, C. Wang, P. Jarvis, T. Lyu and M. Huo, Bactericidal efficiency and photochemical mechanisms of micro/nano
bubble–enhanced visible light photocatalytic water disinfection, Water Res., 2021, 203, 117531 CrossRef CAS PubMed
.
- M. Takahashi, Y. Shirai and S. Sugawa, Free-radical generation from bulk nanobubbles in aqueous electrolyte solutions: ESR spin-trap observation of microbubble-treated water, Langmuir, 2021, 37, 5005–5011 CrossRef CAS PubMed
.
- J. Li, Y. Xia, X. Song, B. Chen and R. N. Zare, Continuous Ammonia Synthesis from Water and Nitrogen via Contact Electrification,
Proc. Natl. Acad. Sci.
, 2024, 121, e2318408121 CrossRef PubMed
.
|
This journal is © The Royal Society of Chemistry 2024 |