DOI:
10.1039/D3RA08088A
(Paper)
RSC Adv., 2024,
14, 6598-6602
Enhancing continuous-flow reactions via compression-molding of solid catalysts and dilutants in packed-bed systems†
Received
26th November 2023
, Accepted 15th February 2024
First published on 22nd February 2024
Abstract
In this study, we present an improved packed-bed system designed for continuous-flow reactions using platinum (Pt)-black powder and silica gel (SiO2). The Pt-leaching from the reaction column is suppressed via compression-molding of the Pt and SiO2. Scanning electron microscopy results and particle-size distribution analysis demonstrate that crushed and downsized SiO2 is effective in suppressing outflow. Furthermore, we successfully conducted a scaled-up experiment of the flow reaction using a large column, achieving excellent productivity.
Introduction
The continuous-flow (CF) method represents a novel technology that offers several advantages over traditional batch methods. These benefits encompass ease of scalability, enhanced safety, and improved environmental friendliness.1–5 In particular, the development of packed-bed flow methods utilizing columns filled with solid catalysts is in high demand.6–10 Fine powder catalysts (metal-on-support catalyst, polymer, etc.) which are used for the packed-bed flow method are generally premixed with a solid dilutant, such as Celite, silica gel (SiO2), or metal oxide. This precautionary measure prevents the clogging of the reaction column and the increment of the reaction pressure. We also developed various CF reactions employing the reaction column packed with premixed solid catalysts and dilutants.11–13
Recently, the CF-oxidation of alcohols using hydrogen peroxide (H2O2) was developed in a reaction column.14 This column was packed with platinum (Pt)-black powder and SiO2, and equipped with a Teflon column filter. Since H2O2 and oxygen gas are a clean oxidant that forms water after oxidation,15 this flow oxidation is remarkable in terms of environmental friendliness. As part of our efforts to scale up the process, we conducted a preliminary experiment using a large column (inner diameter (ID): 10 mm and length (L): 100 mm). In the previous work we employed a small reaction column (ID: 5 mm, L: 100 mm) in conjunction with tert-amyl alcohol (t-amylOH) as the solvent. Initially, the substrate and water were simultaneously introduced into the prepared reaction column packed with Pt-black and SiO2 before transitioning to the substrate solution and H2O2. For the scaled-up experiment involving a large reaction column (ID: 10 mm, L: 100 mm), only water was introduced into the column before the reaction to avoid the risk of Pt-ignition. Unfortunately, the treatment of the column with water led to the undesired leaching of Pt-black from the reaction column. Based on this result, it was determined that stable operation for scaled-up experiments is infeasible owing to the risk of catalyst leaching and clogging of the column. Our analysis suggests that the leaching occurred due to the void space within the reaction column, which resulted from the disparity in particle size difference between the Pt powder and SiO2. Substituting other solid dilutants, such as aluminum oxide and magnesium oxide, for controlling the leaching was not suitable due to the resulting decrease in catalyst reactivities.14 Consequently, we initiated an investigation into a method for suppressing the leaching of Pt-black powder from the reaction column by diluting it with SiO2. The void space can be reduced by packing the column with a catalyst in which Pt is supported on silica. However, with the supported catalyst, Pt is slightly oxidized by silica, which reduces its reactivity.14 Thus, a method is needed to pack the column without void spaces while maintaining Pt-black in its zero-valency state.
Results and discussion
EDX analysis for the Pt content in the column after the reaction
To confirm the composition of the catalyst in the column before and after the CF-oxidation of alcohols, we performed energy-dispersive X-ray spectroscopy (EDX) for the Pt content in the reaction column (Table 1). Initially, the 0.42 wt% Pt-black powder (particle size: 2–20 μm) and SiO2 were premixed and packed into a glass reaction column (ID: 10 mm, L: 100 mm). The columns were installed in a flow reactor (EYELA, MCR-1000). Following the introduction of only water into the column, the flow reactor was heated to 90 °C, and a 2.0 wt% H2O2 aqueous solution and a benzyl alcohol (1) solution in t-amylOH were pumped to the reaction column at a flow rate of 0.4 mL min−1 for 24 h. Subsequently, the column was cooled to room temperature and rinsed with t-amylOH and water. The catalysts were then removed from the column and dried in vacuo. Detailed information on the reaction setup and procedures are provided in the ESI.† The Pt content in the column before and after the flow reaction was measured via EDX at four different locations (Table 1). When employing the reaction column packed with Pt-black powder and Wakogel C-200 (75–150 μm), the Pt content in the column significantly decreased from 0.42% to 0.07–0.26% after the reaction (entry 1). Notably, the Pt in the column at the inlet side (parts A and B) leached 1.2–2.2 times faster than at the outlet side (parts C and D) considering the ratio of reduction for Pt amount. Afterward, the reaction column was packed with Pt and 60-spherical SiO2 (SiO2-60-S, Kanto Chemical Co. Inc.), which has a smaller particle size (40–50 μm) than Wakogel C-200 (entry 2). The leaching of Pt from the reaction column was improved compared with that of Wakogel C-200, although some Pt flowed out even when using SiO2-60-S.
Table 1 Energy-dispersive X-ray spectroscopy (EDX) analysis of Pt in the reaction column before and after the continuous-flow (CF) oxidation reaction
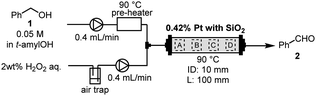
|
Entry |
SiO2 |
EDX analysis of the Pt rate (%) |
A |
B |
C |
D |
Compression-molded Pt with SiO2-60-S was used. SiO2-60-S was compression-molded alone and then mixed with Pt. |
1 |
Wakogel C-200 |
0.07 |
0.07 |
0.12 |
0.26 |
2 |
SiO2-60-S |
0.32 |
0.27 |
0.38 |
0.31 |
3a |
SiO2-60-S |
0.42 |
0.43 |
0.42 |
0.42 |
4b |
SiO2-60-S |
0.37 |
0.26 |
0.36 |
0.37 |
Compression-molding (CM) is a forming method that provides a desired-shaped powder using a mold with optional pressure.16,17 Consequently, we envisaged that Pt could adhere to the solid dilutant, and by employing CM, the void space within Pt and the solid dilutant could be minimized. Premixed Pt and SiO2-60-S were pressed using a press machine (RIKEN KIKI Co. Ltd., CDM-5PA) and mold (AS ONE CORPORATION, mold for heat press machine φ14) under a pressure of 20 MPa for 2 h. After CM, the resulting cylindrical-shaped solid was crushed, filtrated through a 300 μm-filter, and then packed into the reaction column. Importantly, the amount of Pt remained unchanged after the reaction within the prepared column (entry 3). Thus, the leaching of Pt during the reaction can be suppressed by CM. On the other hand, using the reaction column that SiO2-60-S was compression-molded alone and then mixed with Pt, the Pt content decreased after the reaction (entry 4). Therefore, CM of Pt and SiO2-60-S together was effective for the Pt leaching.
SEM and EDX analysis for catalysts
Scanning electron microscopy (SEM) and EDX mapping of the catalysts were performed to evaluate the impact of CM on Pt and SiO2-60-S (Fig. 1). At first, SiO2-60-S exhibited a spherical morphology before CM (Fig. 1(a)); however, after CM, these spherical SiO2-60-S were crushed (Fig. 1(b)). In the EDX mapping images, SiO2-60-S appeared orange while Pt was blue (Fig. 1(c) and (d)). These images reveal that a portion of the Pt powder was attached to SiO2-60-S. Furthermore, we confirmed the void volume of the reaction column packed with Pt and SiO2-60-S. The void volume of the reaction column without CM was 6.36 mL, while that with CM was 4.72 mL. Detailed results were provided in the ESI.† The presence of crushed SiO2-60-S contributed to a reduction in void space. Consequently, it is evident that the adhesion between Pt and SiO2-60-S, coupled with the smaller void space, effectively suppressed the leaching of Pt powder.
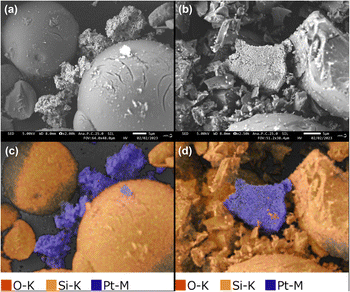 |
| Fig. 1 (a) A scanning electron microscopy (SEM) image of premixed Pt and SiO2-60-S. (b) A SEM image of compression-molded Pt and SiO2-60-S. (c) Energy-dispersive X-ray spectroscopy (EDX) mapping of premixed Pt and SiO2-60-S. (d) EDX mapping of compression-molded Pt and SiO2-60-S. | |
Particle-size distribution of catalysts
We measured the particle-size distribution of the SiO2-60-S and/or Pt-black powder with and without CM (Fig. 2 and 3). In Fig. 2, the particle-size distribution of only Pt was assessed, revealing particles (derived from Pt) with diameters of approximately 10 μm. Fig. 3 clearly illustrates the differences in the size distribution between SiO2-60-S and Pt without CM (the peaks of around 10 μm and 50 μm). Conversely, the difference in particle size SiO2-60-S and Pt with CM was small. The particle-size distributions of only SiO2-60-S with and without CM are provided in the ESI.† Based on the SEM results and particle-size distribution, we concluded that the crushed- and downsized-SiO2-60-S effectively suppressed Pt flow from the reaction column during the reaction.
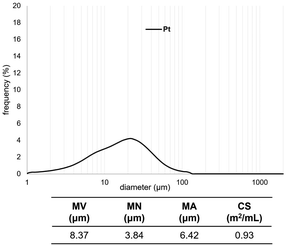 |
| Fig. 2 Particle size distributions of Pt. MV: mean volume diameter, MN: mean number diameter, MA: mean area diameter, CS: specific surface area. | |
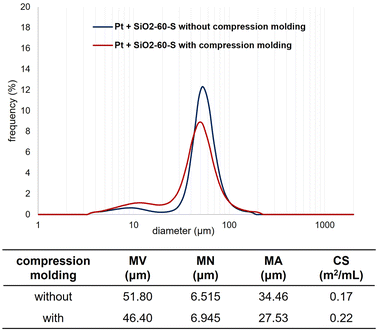 |
| Fig. 3 Particle size distributions of Pt and SiO2-60-S without and with compression-molding (CM). | |
ICP-AES analysis of Pt with/without CM for the CF reaction
We analysed the Pt content after introducing water for 3 h using inductively coupled plasma atomic emission spectroscopy (ICP-AES), with the results shown in Table 2. The analysis employed a flow reactor equipped with a reaction column (ID: 10 mm, L: 100 mm) and a flow reactor (EYELA, MCR-1000). Water was pumped into the reaction column packed with Pt and SiO2-60-S at 0.4 mL min−1 for 3 h. The solutions that passed through the column for 3 h were subjected to ICP-AES analysis (ESI†). ICP-AES analysis without CM indicated that 0.48 mg (2.66%) of Pt flowed out from the reaction column packed with 17.95 mg of Pt (entry 1). In contrast, when using a column packed with compression-molded catalysts, the rate of Pt leaching from the column considerably reduced to 0.13% (entry 2). This clearly demonstrates the effectiveness of CM of Pt and SiO2-60-S in suppressing the flow of Pt powder from the column. The reproducibility of the leaching tests was described in ESI.†
Table 2 Inductively coupled plasma atomic emission spectroscopy (ICP-AES) analysis of Pt with/without CM for the CF reaction
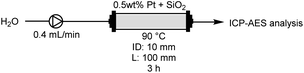
|
Entry |
CM |
Amount of Pt packed in the column |
ICP-AES analysis of Pt |
Amount of Pt flowing out |
Rate of Pt flowing out from column |
1 |
Without |
17.95 mg |
0.48 mg |
2.66% |
2 |
With |
21.20 mg |
0.03 mg |
0.13% |
XPS analysis of Pt with/without CM
X-ray photoelectron spectroscopy (XPS) was performed with and without CM of Pt with SiO2-60-S catalysts (Fig. 4). The peaks at 71.2 and 74.2 represent the binding energies (BEs) of the CM catalysts, which are ascribed to Pt 4f7/2 and Pt 4f5/2, respectively. These values of the Pt 4f peaks were close to 70.9 and 74.2 eV, which were assigned to the BEs of the bulk Pt metal, and the observed peaks corresponded to Pt(0).18,19 This indicates that whether compression-molded or not, there was no change in the Pt 4f7/2 and Pt 4f5/2 peaks, affirming the preservation of Pt(0) even after CM.
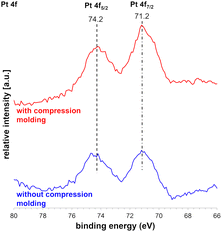 |
| Fig. 4 XPS analysis of Pt and SiO2-60-S with and without CM. | |
Scale-up experiment for the CF-oxidation using compression-molded Pt and SiO2-60-S
Finally, we conducted a 10 g-scale-up experiment at the laboratory scale for the CF-oxidation reaction of benzyl alcohol (1) to benzaldehyde (2) using compression-molded Pt and SiO2-60-S (Fig. 5). A stainless-steel (SUS) reaction column (ID: 37 mm, L: 300 mm) and flow reactor (EYELA, HCR-1000) were employed for the bench–scale reaction. The mesh size of the SUS column filter (EYELA, SUS laminated metal filter) was 10 μm. To scale up the CM experiment, a press machine (RIKEN KIKI Co. Ltd., CDM-5PA) and mold (Labonect, LD-3025, φ30 mm × 25 mm) were utilized. Premixed Pt and SiO2-60-S were pressed at 40 MPa for 2 h to obtain the compression-molded catalysts. A solution of 1 in t-amylOH (0.67 M) and 10 wt% of an aqueous H2O2 solution were introduced at a flow rate of 3.0 mL min−1 into the reaction column and heated to 90 °C. The yield of 2 was maintained at over 80%, with its productibility being 10.4 g h−1. In comparison, a previous study reported a productibility of 0.7 g h−1 for 2.14 Thus, a significant fifteen-fold scale-up of the reaction was achieved through the use of a large column (ID: 37 mm, L: 300 mm).
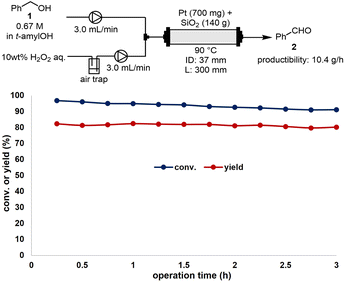 |
| Fig. 5 The scale-up experiment for the CF-oxidation reaction. | |
Conclusions
We have successfully developed an improved packed-bed system for CF-oxidation reactions. This system employs a compression-molded Pt-black catalyst and SiO2-60-S to effectively suppress Pt leaching from the column during reactions. Stable flow oxidation was conducted by utilizing CM. According to the SEM and particle-size distribution results, the crushed- and downsized-SiO2-60-S was effective in avoiding Pt leaching. Furthermore, we scaled up the CF-oxidation of benzyl alcohol to benzaldehyde using compression-molded Pt and SiO2-60-S. This scale-up was performed, resulting in a final productivity of 10.4 g h−1 for benzaldehyde. We believe that our CM method for solid catalysts and dilutants holds great promise for small-to large-scale experiments of CF reactions because it effectively prevents catalyst leaching from the reaction columns.
Conflicts of interest
There are no conflicts to declare.
Acknowledgements
The authors thank Dr Koichiro Masuda (National Institute of Advanced Industrial Science and Technology) and Yasuharu Morii (EYELA) for their valuable contributions and insightful discussions. This study was partially supported by the New Energy and Industrial Technology Development Organization (JPNP19004).
Notes and references
- G. Gambacorta, J. S. Sharley and I. R. Baxendale, Beilstein J. Org. Chem., 2021, 17, 1181–1312 CrossRef CAS.
- N. C. Neyt and D. L. Riley, React. Chem. Eng., 2021, 6, 1295–1326 RSC.
- R. Porta, M. Benaglia and A. Puglisi, Org. Process Res. Dev., 2016, 20, 2–25 CrossRef CAS.
- M. Guidi, P. H. Seeberger and K. Gilmore, Chem. Soc. Rev., 2020, 49, 8910–8932 RSC.
- J. Britton and C. L. Raston, Chem. Soc. Rev., 2017, 46, 1250–1271 RSC.
- J. N. Appaturi, R. Ratti, B. L. Phoon, S. M. Batagarawa, I. U. Din, M. Selvaraj and R. J. Ramalingam, Dalton Trans., 2021, 50, 4445–4469 RSC.
- J. C. Pastre, D. L. Browne and S. V. Ley, Chem. Soc. Rev., 2013, 42, 8849 RSC.
- K. Masuda, T. Ichitsuka, N. Koumura, K. Sato and S. Kobayashi, Tetrahedron, 2018, 74, 1705–1730 CrossRef CAS.
- A. Tanimu, S. Jaenicke and K. Alhooshani, Chem. Eng. J., 2017, 327, 792–821 CrossRef CAS.
- C. G. Thomson, A.-L. Lee and F. Vilela, Beilstein J. Org. Chem., 2020, 16, 1495–1549 CrossRef CAS PubMed.
- T. Ichitsuka, N. Suzuki, M. Sairenji, N. Koumura, S. Onozawa, K. Sato and S. Kobayashi, ChemCatChem, 2019, 11, 2427–2431 CrossRef CAS.
- T. Ichitsuka, I. Takahashi, N. Koumura, K. Sato and S. Kobayashi, Angew. Chem., Int. Ed., 2020, 59, 15891–15896 CrossRef CAS PubMed.
- K. Kobayashi, K. Masuda, F. Feng, Md. N. Rashed, K. Sato, N. Koumura and S. Kobayashi, Adv. Synth. Catal., 2023, 365, 1618–1622 CrossRef CAS.
- Y. Kon, T. Nakashima, A. Yada, T. Fujitani, S. Onozawa, S. Kobayashi and K. Sato, Org. Biomol. Chem., 2021, 19, 1115–1121 RSC.
- Y.-H. Lu, C. Wu, J.-C. Hou, Z.-L. Wu, M.-H. Zhou, X.-J. Huang and W.-M. He, ACS Catal., 2023, 13, 13071–13076 CrossRef CAS.
- Y. Nagai, K. Takeshita and T. Okane, Mater. Trans., 2020, 61, 734–739 CrossRef CAS.
- S.-W. Jin, Y.-J. Choi, H.-C. Yu, S.-H. Lee, Y.-J. Jin, I.-S. Lee, H.-S. Lee and C.-M. Chung, ACS Sustainable Chem. Eng., 2022, 10, 1910–1919 CrossRef CAS.
- C. D. Wagner, W. M. Riggs, L. E. Davis and J. F. Moulder, Handbook of X-Ray Photoelectron Spectroscopy, ed. G. E. Mullemberg, PerkinElmer, Eden Prairie, MN, 1978 Search PubMed.
- Y. Yamauchi, T. Ohsuna and K. Kuroda, Chem. Mater., 2007, 19, 1335–1342 CrossRef CAS.
|
This journal is © The Royal Society of Chemistry 2024 |
Click here to see how this site uses Cookies. View our privacy policy here.