DOI:
10.1039/D3RA07058A
(Paper)
RSC Adv., 2024,
14, 8810-8818
An innovative transportable immune device for the recognition of α-synuclein using KCC-1-nPr-CS2 modified silver nano-ink: integration of pen-on-paper technology with biosensing toward early-stage diagnosis of Parkinson's disease†
Received
17th October 2023
, Accepted 18th February 2024
First published on 15th March 2024
Abstract
Parkinson's disease (PD), the second most frequent neurodegenerative illness, is a neurological ailment that produces unintentional or uncontrolled body movements, which should be diagnosed in its early stages to hinder the progression. Monitoring the concentration of α-synuclein (α-Syn) in body fluids can be one of the most efficient ways for PD early detection. In this work, a paper-based electrochemical immunosensor was designed for α-Syn bio-assay in human plasma samples based on encapsulation of the biotinylated antibody on novel dendritic fibrous nanosilica ((KCC-1-nPr-CS2)-Ab). For this purpose, a three-electrode system was prepared using stabilization of silver nano-ink on photographic paper. Then, the (KCC-1-NH-CS2)-Ab was immobilized on its surface and used to detect the target antigen (α-Syn). After characterization of the prepared substrate by FE-SEM and EDS, the redox behavior of the biosensor was evaluated using chronoamperometry techniques. Under optimal experimental conditions and using a label-free strategy, the engineered immunosensor showed a linear relationship between peak current and antigen concentration in the linear range from 0.002 to 128 ng mL−1 with the lower limit of quantification of 0.002 ng mL−1. Moreover, this work involves unprecedented use of conductive nano-inks for the manufacture of α-Syn immunosensor, which is aided by the use of a mesoporous silicate dendrimer in encapsulating the α-Syn antibody, thus offering a robust and simple point-of-care device for early PD diagnosis. The ability of the proposed platform to detect small amounts of α-Syn offers a promising approach to developing low-cost, sensitive, and transportable biosensors for Parkinson's disease screening in its early stages.
1. Introduction
Parkinson's disease (PD) is the most prevalent movement condition and the second most frequent neurodegenerative illness that affects the central nervous system.1 Also, 1% of the elderly above the age of 60 and 4% of the elderly older than 80 years old are affected by PD.2 The diagnosis of PD typically occurs after the appearance of clinical symptoms, which indicates that the disease has already progressed to a moderate or advanced stage.3 Nevertheless, the process of neurodegeneration associated with PD starts many years prior to diagnosis.4 One approach to achieving early detection of PD is to identify particular biomarkers associated with the disease and then detect them using a cost-effective and dependable analytical system. One such biomarker is α-synuclein (α-Syn), a 14 kDa protein encoded by the SNCA gene, which shows potential for non-invasive PD detection in clinical settings.5,6 The unusual clustering of α-Syn protein, which leads to the formation of Lewy bodies and Lewy neurites within neurons of the substantia nigra, is recognized as a crucial factor contributing to the PD progression.7 Numerous studies have indicated that the anomalous concentration of α-Syn can be identified in bodily fluids, including peripheral blood.8 Therefore, sensitive determination of this biomarker in human biofluids is important for clinicians.
Several analytical methods including enzyme-linked immunosorbent assays (ELISA),9 immunomagnetic reduction (IMR),10 fluorescent immunoassay,11 and real-time PCR12 have been employed for detection and quantification of α-Syn. The main limitations of these approaches are that they need costly laboratory equipment and specialist manpower, and they involve somewhat a long response time. Owing to these restrictions, miniaturizing of these methods and their use in places other than well-equipped laboratories are unimaginable. Electrochemical sensors and biosensors which work by interacting with the target analyte to provide an electrical signal proportionate to the analyte concentration13 can be a suitable option to overcome these limitations. These analytical devices have been making strides in becoming reliable analytical tools that can replace older, more cumbersome, and more costly analytical equipment.14
One kind of electrochemical biosensor is an immunosensor. Immunosensors are designed to have a unique electrical response when an antibody and its specific antigen match and connect. Immunosensors can be more sensitive and selective than other biosensors as the antigen–antibody reaction is unique for every species and can trigger electrochemical responses even in tiny amounts of the analyte.15 Due to their excellent sensitivity, low cost, and naturally compact size, electrochemical immunosensors have recently gained a lot of attention.16 In this respect, some electrochemical immunosensors for α-Syn have been reported.17–19
The emergence of portable on-site diagnostic tools, also called point-of-care devices (POCD), has ignited a swiftly growing field of exploration with numerous clinical and commercial applications.20–22 For devising such devices, a variety of platforms have been used, including glass, ceramic, plastic, and paper.23–25 Due to its great sensitivity, mobility, low production cost, simplicity of use, biodegradability, and capacity for customization and adjustment, paper has become more popular than other materials.26–28 Also, paper-based analytical devices (PADs) have come into existence. Moreover, because of the excellent electrical resistance and flexibility of paper, PADs have widespread uses in designing electrochemical biosensors.29,30
The development of nanomaterials as well as conductive nano-inks has created intriguing novel opportunities for using them to immobilize biological molecules efficiently in electrochemical biosensors and amplify the electrical response pulse.31 Silver, copper, platinum, aluminum, and gold are most commonly used to make conductive inks.32 Among them, silver because of its unique properties such as proper thermal and electrical characteristics as well as low toxicity which can cover electrocatalytic reactions stands out from other nanomaterials at a reasonable price.33,34
Although polymers are the most often used substrates for immobilizing active bio-species, inorganic substrates such as silica may offer certain advantages, including greater durability, chemical and heat resistance, and the absence of swelling in aqueous or organic solutions.35 Fibrous nano-silica (KCC-1) with decent appropriated stability could be easily modified with various functional groups in order to acquire the desirable properties.36 Notably, the high surface-to-volume ratio of these advanced mesoporous nanomaterials can be a determinant factor in the sensitivity of the sensor.37 So far, some of the modified KCC-1 including KCC-1-NH2-FA for sensing HT 29 cancer cells,38 KCC-1-NH2-DPA for sensing prostate-specific antigen,39 and KCC-1-NH2-Tb for detection of aflatoxin M1
40 have been applied successfully.
Utilizing the numerous benefits of the paper-based sensors and employing encapsulated Abs in dendritic fibrous nano-silica for EC immunosensors assembly, we have devised a portable electrochemical immune platform for the surveillance of α-Syn in human biofluids. In the first step, conductive Ag nano-ink was synthesized and this ink was drawn on photographic paper to form three conventional electrodes. In the second step, the working electrode was modified with the synthesized (KCC-1-NH-CS2)-Ab which make the α-Syn immunosensor ready to be evaluated by relative electrochemical techniques.
2. Material and methods
2.1. Substances and chemicals
A human a-synuclein kit was obtained from ZellBio GmbH (Germany) by Padgin TEB Company. Sodium dihydrogen phosphate monohydrate (H2NaPO4·H2O), potassium chloride (KCl), sodium hydrogen phosphate (Na2HPO4), potassium ferrocyanide (K4Fe(CN)6), and potassium ferricyanide (K3Fe(CN)6) were purchased from Merck (Germany). Bovine serum albumin (BSA), glutaraldehyde (GA), silver nitrate (AgNO3), and cysteamine (CysA) were obtained from Sigma-Aldrich (Ontario, Canada). A human plasma sample was supplied by the Iranian Blood Transfusion Research Center (Tabriz, Iran).
2.2. Apparatuses
Paper-based three-electrode system was prepared using a synthesized conductive silver nano-ink. Electrochemical measurements were performed using the PalmSens 4c system. TEM (Transmission electron microscopy), Philips-CM30, Adelaide, Australia with an operating voltage of 200 kV was utilized for the investigation of the crystallographic structure, morphology, and size of the synthesized Ag nano-ink. FE-SEM (high-resolution field emission scanning electron microscope), Hitachi-SU8020, Czech operated at 3 kV was also employed for capturing the microstructure images of the bulk nano-ink and characterization of the surface modification at diverse steps of immunosensor preparation. Chemical composition was also evaluated by EDS (energy dispersive spectroscopy) coupled with the FE-SEM. The resistance of the synthesized Ag nano-ink was assessed using Ohmmeter (XIOLE, XL830L, China, multimeter).
2.3. Synthesis of conductive Ag nano-ink
Silver nano-ink was synthesized based on our previous work.41 To synthesize conductive nano-ink, firstly, a mixture containing poly acrylic acid (PAA) as a capping agent, diethanolamine (DEA) as a reducing agent and water was stirred in a water bath for about 2 h, after that, silver nitrate solution was added and was stirred for 22 h. After keeping the solution in an ultrasonic water bath for about 1.5 h, it was mixed with ethanol and centrifuged (20 min, 9000 rpm). To wash the resulting precipitate, it was mixed with water and centrifuged (3 times). The final precipitate was dissolved in a 2 wt% solution of hydroxypropyl cellulose and homogenized for 3 min at 2000 rpm.
2.4. Conductivity study of the synthesized Ag nano-ink
On the surface of photographic paper, various templates with different thicknesses were created and stabilized in order to evaluate the conductivity of the synthesized Ag nano-ink. For this purpose, the cathode portion of the battery was connected to the lines via a conductive wire, and the anode portion was connected to the lamp. A battery and an LED lamp (both three volts) were placed on the surface of photographic paper. An electrical circuit was created and the lamp was turned on when the current passed from the anode to the cathode. An ohmmeter was also used to measure the lines' resistance. In addition, in order to investigate the effect of ink printing on its conductivity, photographic paper was dipped in ink. After drying, its conductivity and resistance were evaluated. As seen in Fig. 1, there is not much difference in the resistance which suggests that the surface of the photographic paper is suitable for the synthetically created conductive Ag-ink and its stabilization.
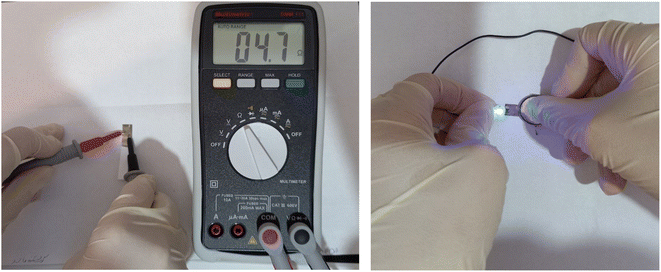 |
| Fig. 1 Photographic image of conductivity and resistance of photographic paper impregnated with conductive silver nano-ink. | |
2.5. Encapsulation of Ab on KCC-1-NH-CS2
KCC-1-NH-CS2 features two-dimensional pore structures, enabling efficient transfer of the reactant and product biomolecules. As a mesoporous silica material, KCC-1-NH-CS2 provides a large surface area (133 m2 g−1), appropriate pore volume (0.4 cm3 g−1), and suitable pore size (12 nm), which allows for high loading of biomolecules such as antibodies. This high loading capacity is essential for enhancing the sensitivity of the immunosensor and achieving excellent detection limits. Additionally, the uniform pore structure of KCC-1-NH-CS2 ensures efficient mass transport of analytes to the immobilized biomolecules, leading to rapid and accurate detection. Furthermore, the functional groups on the surface of KCC-1-NH-CS2 can be easily modified to facilitate the immobilization of specific biomolecules, enabling the development of highly selective immunosensors and biosensors. Overall, the unique characteristics of KCC-1-NH-CS2 make it an excellent choice for the construction of immunosensors, offering great potential for biomedical monitoring of various biomedicals. Therefore, this polymeric silica material was synthesized according to our previous report.42 Following the creation of fibrous nano-silica KCC-1-NH-CS2, a biotinylated antibody combined with NHS/EDC 1
:
1 (v/v) and was stirred for 24 h at room temperature in the solution of 0.1 M of PBS (pH 7.4) with the addition of KCC-1-NH-CS2. Centrifugation was used to separate the supernatant at 6000 rpm for 4 min.
2.6. Fabrication of the paper-based electrochemical immunosensor
To prepare the electrochemical paper-based immunosensor, the synthesized Ag nano-ink was stabilized on the surface of the photographic paper using a suitable mask and allowed to dry at room temperature for 5 minutes. Then, Ab encapsulated into KCC-1-NH-CS2 was immobilized on the surface of the sensing zone (working electrode) and incubated for 1 h at 4 °C. In the next step, after rinsing the electrode with washing buffer, the BSA solution was immobilized on the working electrode modified KCC-1-NH-CS2-Ab for 30 min to block the remaining connection points. After incubation, the electrode surface was rewashed with deionized water to remove contamination. The α-Syn antigen was incubated on the surface of the working electrode. Finally, the working electrode was washed with buffer and dried at room temperature which is necessary for electrochemical evaluation by CV technique. Scheme 1 displays the fabrication and detection process of the electrochemical paper-based immunosensor of α-Syn. Also, Scheme S1 (see ESI†) indicated the connection of the prepared paper-based immunosensor to the three-electrode cell.
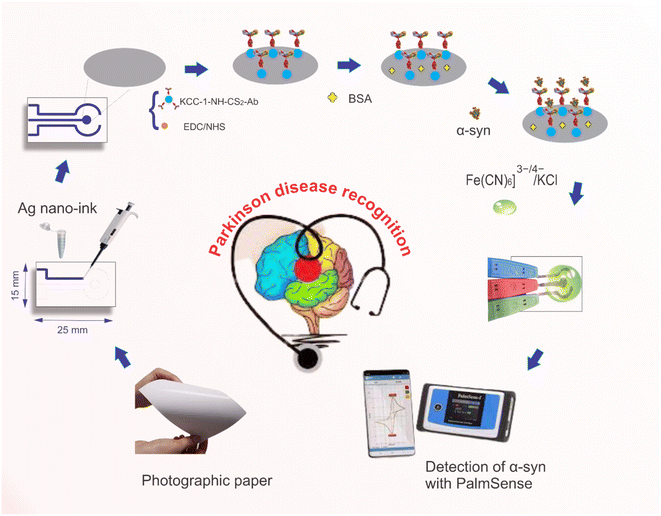 |
| Scheme 1 The construction process of the paper-based immunosensor for the detection of α-Syn biomarker. | |
3. Results and discussion
3.1. Characterization
FE-SEM imaging was used to investigate the morphology of the produced silver nano inks. Fig. S1 (see ESI†) depicts the spherical and homogeneous structure of the silver nanoparticles. Nanoparticle aggregation did not exist. Additionally, as seen in Fig. S1A,† nanoparticles typically have a size of 50 nanometers or less. FE-SEM imaging was also used to record the morphology of each stage of the safety sensor fabrication. As seen in Fig. S1 (see ESI†), conductive silver nano-inks consist of antibodies encapsulated in the spherical silicate nanoparticles and cover the surface of the paper unevenly. The appearance of the surface changes completely following the incubation of BSA and antigen, indicating that the antibodies and antigens are successfully immobilized on the surface of the photographic paper created with Ag ink. The elements were also examined, and the prepared assignment was examined using an EDS spectrometer. As seen in Fig. S2 (see ESI†), the abundance of silver nanoparticles indicates that conductive Ag-ink was successfully synthesized. In the second stage, after antibody incubation, the amount of elemental sulfur increases, which indicates the successful incubation of biological materials on the electrode surface. Also, it is obvious that, after the interaction of the antibody with a specific antigen of α-Syn, the intensity of the sulfur element increased, which confirmed the formation of the immunocomplex.
3.2. Electrochemical evaluation of the constructed immunosensor
Studying the electrochemical performance of immunosensors is of considerable importance in examining their analytical capacity. Therefore, the electrochemical performance of the preparation steps of the immunosensor was evaluated using the chronoamperometry technique. Fig. 2 shows the chronoamperograms obtained from different steps of the electrode preparation, which were recorded at a potential of 0.25 V in the presence of 0.1 M [Fe(CN)6]3−/4−/KCl as an electrochemical probe. As can be seen, Ag nano-ink is an effective compound in shuttling electrons in the presence of ferro/ferry (supporting electrolytes). Nevertheless, by adding (KCC-1-NH-CS2)-Ab on the surface of the working electrode a slight decrease in current was observed. With the addition of BSA (blocking agent), the current decreases, indicating that the non-antibody-coated spots from the surface were successfully blocked with BSA. After the introduction of α-Syn to the structure of the immunosensor, an antigen–antibody complex was formed, activating the immunosensor and switching it to the signal-ON mode. KCC-1-NH-CS2 was then able to enhance conductivity upon exposure to the ferro/ferry solution, leading to a significant increment in the observed current. It is important to point out that, KCC-1-NH-CS2 is an ideal candidate for the encapsulation of antibodies due to its unique properties. The large surface area and high pore volume of KCC-1-NH-CS2 provide ample space for the immobilization of antibodies, ensuring efficient encapsulation. Additionally, its biocompatibility and low cytotoxicity make it a safe option for use in biomedical applications. The chemical stability of KCC-1-NH-CS2 further ensures the preservation of the encapsulated antibodies, making it suitable for long-term storage and use. Furthermore, the controlled release capabilities of KCC-1-NH-CS2 allow for the sustained delivery of antibodies, enhancing their therapeutic potential. Overall, the combination of its structural, chemical, and biocompatible properties makes KCC-1-NH-CS2 an excellent choice for the encapsulation of antibodies, offering numerous opportunities for biomedical and pharmaceutical advancements.
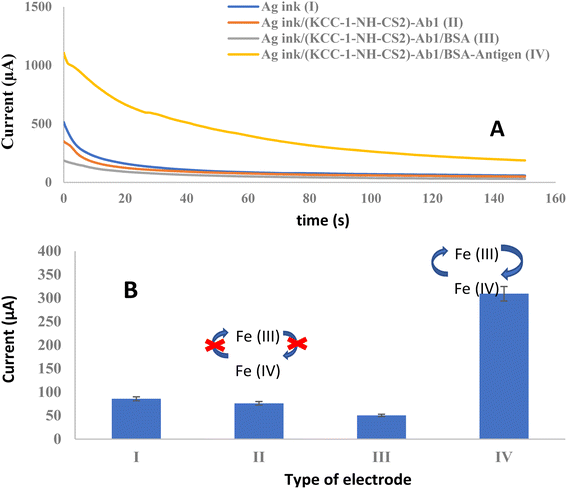 |
| Fig. 2 (A) ChAs of immunosensor fabrication steps in the presence of 0.01 M [Fe(CN)6]3−/4−/KCl solution as an electrochemical probe in E = 0.25 V, duration time = 150 s, (B) histogram of peak current versus type of the electrode. | |
3.3. Analytical performance
The realization of the immunosensor was investigated using Ab interaction with various concentrations of α-Syn antigen (0.002, 2, 8, 32, and 128 ng mL−1). Fig. 3 shows the chronoamperograms in different concentrations of the analyte. The results indicate an increase in the peak current resulting from an increase in target Ag concentration. The calibration curve was plotted from the peak current and the α-Syn concentration under optimum conditions. Using an engineered immune device a linear range of 0.002 to 128 ng mL−1 and a lower limit of quantification (LLOQ) of 0.002 ng mL−1 with regression equations I (μA) = 2.5153 CAg (ng mL−1) + 280.1 (R2 = 0.9953) were obtained. The results indicate the suitable capability of the designed immunosensor for α-Syn identification.
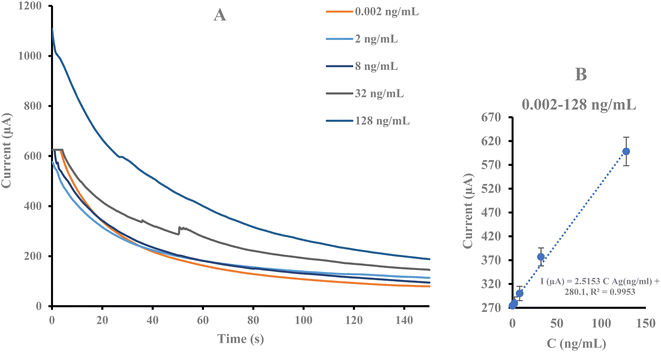 |
| Fig. 3 (A) ChAs of the prepared immunosensor in various concentrations of α-Syn (0.002, 2, 4, 8, 16, 32, and 128 ng mL−1) in the presence of 0.01 M [Fe(CN)6]3−/4−/KCl solution as an electrochemical probe in E = 0.6 V, duration time = 150, (B) calibration curve of current intensity changes against α-Syn antigen concentrations. | |
A comparison of the analytical results of this immunosensor and those from prior studies, presented in Table 1, reveals its high sensitivity and linear range compared to others. The results reveal that the engineered immunosensor provides an appropriate substrate for monitoring α-Syn at low concentrations.
Table 1 Comparison of the analytical performance of various electrochemical biosensors with our designed immunosensor for α-Syn identification
Platform |
Biorecognition element |
Technique |
Linear range |
LOD/LLOQ |
Ref. |
Screen-printed gold electrode |
Aptamer |
DPV, CV |
60 pM to 150 nM |
10 pM |
43 |
Peptide-PEG mixed layer |
Peptide |
EIS, CV |
— |
50 μM (S/N = 3) |
44 |
PEG self-assembled monolayer (SAM) |
Antibody |
EIS |
7.5 ng mL−1 to 0.15 μg mL−1 |
∼3.1 ng mL−1 |
45 |
PEG-thiol monolayer |
Antibody |
EIS |
0.5 to 10 nM |
55 ± 3 pM |
46 |
AuNCs-graphene |
Antibody |
DPV, ELS |
4 to 128 ng mL−1 |
4 ng mL−1 |
19 |
Paper-based electrode/Ag ink |
Antibody |
ChA |
0.002 to 128 ng mL−1 |
0.002 ng mL−1 |
This work |
3.4. Real sample analysis
To study the realization of the prepared immunosensor to blood-based detection, human plasma was spiked with known concentrations of α-synuclein antigen (0.002, 0.02, 16, 64, and 128 ng mL−1) 1
:
1 (v/v). It was drop cast on the surface of the Ag ink/(KCC-1-NH-CS2)-Ab/BSA paper-based electrode. Fig. S3 (see ESI†) demonstrates the ChA responses recorded with different concentrations of the target antigen in a human plasma specimen in [Fe(CN)6]−3−/−4/KCl as the supporting electrolyte. The results exhibit that an increase in the concentration of the analyte leads to an increase in the peak current. The linear ranges of 0.02 to 32 ng mL−1 and lower limits of quantification (LLOQ) of 0.02 ng mL−1 were obtained under optimum conditions. Calibration curves plotted the linear regression equation to ChAs is as follows: I (μA) = 2.8941 Cα-Syn (ng mL−1) + 211.89 (R2 = 0.9466).
3.5. Evaluation of specificity of the biosensor
The selectivity of the sensor was evaluated due to the possibility that the same concentration of interfering substances such as CYFRA 21.1, CA 15-3, CEA, and PSA were also detected with the same method. As shown in Fig. 4, it was clear that the chronoamperometric response to the target was higher than the response to the interferents. This result demonstrates the high selectivity of the sensor to α-synuclein. However, among the interferers, CEA has a higher current and shows that it can lead to interference in target recognition. CA 15-3 has the least intervention effect.
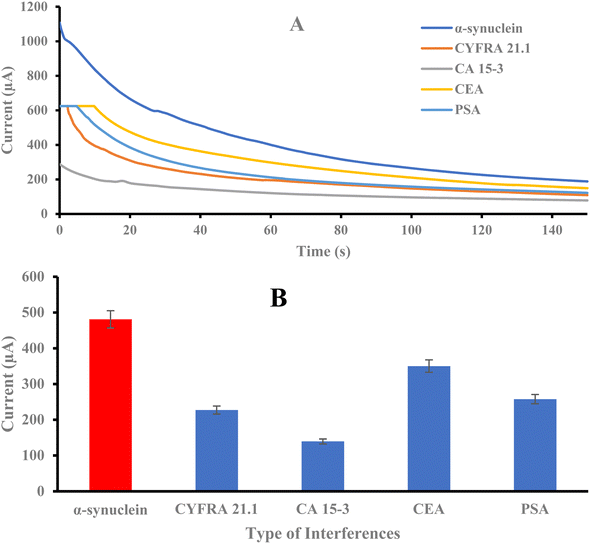 |
| Fig. 4 (A) ChAs of the immunosensor in the presence of various interferences in 0.01 M [Fe(CN)6]3−/4−/KCl solution as the electrochemical probe in E = 0.25 V, duration time = 150, (B) histogram of current intensity changes versus type of interferences. | |
3.6. Repeatability and stability of the immunosensor
The stability of the designed immunosensor was evaluated by keeping the paper-based electrode modified with antibody-encapsulated KCC-1-NH-CS2 in a refrigerator at 4 °C for 5 days. The current response for the designed immunosensor decreased dramatically after the first day (Fig. S4A, see ESI†). The repeatability of the designed electrodes was evaluated without the presence of a bioreceptor in the ferrocyanide solution. The results shown in Table S1 (see ESI†) reveal that the electrodes have almost similar current responses in different repetitions (AVESTDV = 2.26). In addition, the stability of the fabricated paper electrodes was also evaluated in terms of cycles. For this purpose, first, 1, 5, 10 and 50 cycles were performed with the cyclic voltammetry technique, and after each of them, a response current was recorded using the chronoamperometry technique. The obtained results showed that after the first cycle, the current decreased significantly (Fig. S4C, see ESI†). The results of the stability investigation show that the prepared paper electrode is disposable and its best performance is in its first use.
4. Conclusion
In this study, a paper-based electrochemical immunosensor was presented for the quantification of α-Syn antigen towards early-stage diagnosis of Parkinson's disease. Three working, reference, and counter electrodes were fabricated on a single substrate using silver conductive nano-ink using a hand-writing strategy. The main innovation of the present work is the encapsulation of the biotinylated antibody on KCC-1-NH-CS2 which led to better performance of the fabricated immunosensor toward its interaction with specific antigen (α-Syn). The lowest amount of antigen that the immunosensor could detect was 0.002 ng mL−1. Therefore, it can be concluded that this platform has the ability to detect targets at low concentrations which could be a promising, inexpensive, portable, and disposable device for Parkinson's screening. To sum up, this work represents the first use of conductive nano-inks to create an α-Syn immunosensor, which offers a reliable and straightforward point-of-care device for the early detection of PD in place with the innovative deploying of mesoporous silica dendrimer in the process of immunosensor fabrication. Benefiting from the simplicity and high sensitivity, the proposed method shows a potential for employment in clinical applications and high-throughput screening of Parkinson's disease using POC. It is expected that the proposed immunosensor (after full analytical validation) can be utilized as a versatile system for other biomarkers quantification especially in clinical diagnostics owing to good performance and high sensitivity.
Conflicts of interest
There are no conflicts to declare.
Acknowledgements
The research reported in this publication was supported by the Elite Researcher Grant Committee under award number [4002318] from the National Institutes for Medical Research Development (NIMAD), Tehran, Iran, [IR.NIMAD.REC.1402.318].
References
- J. Parkinson, An essay on the shaking palsy, J. Neuropsychiatry Clin. Neurosci., 2002, 14(2), 223–236 CrossRef PubMed.
- A. Atik, T. Stewart and J. Zhang, Alpha-synuclein as a biomarker for Parkinson's disease, Brain Pathol., 2016, 26(3), 410–418 CrossRef CAS PubMed.
- W. Le, J. Dong, S. Li and A. D. Korczyn, Can biomarkers help the early diagnosis of Parkinson's disease?, Neurosci. Bull., 2017, 33, 535–542 CrossRef CAS PubMed.
- L. Parnetti, A. Castrioto, D. Chiasserini, E. Persichetti, N. Tambasco and O. El-Agnaf, et al., Cerebrospinal fluid biomarkers in Parkinson disease, Nat. Rev. Neurol., 2013, 9(3), 131–140 CrossRef CAS PubMed.
- H. A. Lashuel, C. R. Overk, A. Oueslati and E. Masliah, The many faces of α-synuclein: from structure and toxicity to therapeutic target, Nat. Rev. Neurosci., 2013, 14(1), 38–48 CrossRef CAS PubMed.
- W. Poewe, K. Seppi, C. M. Tanner, G. M. Halliday, P. Brundin and J. Volkmann, et al., Parkinson disease, Nat. Rev. Dis. Prim., 2017, 3(1), 1–21 Search PubMed.
- M. G. Spillantini, M. L. Schmidt, V. M.-Y. Lee, J. Q. Trojanowski, R. Jakes and M. Goedert, α-Synuclein in Lewy bodies, Nature, 1997, 388(6645), 839–840 CrossRef CAS PubMed.
- L. Parnetti, L. Gaetani, P. Eusebi, S. Paciotti, O. Hansson and O. El-Agnaf, et al., CSF and blood biomarkers for Parkinson's disease, Lancet Neurol., 2019, 18(6), 573–586 CrossRef CAS PubMed.
- B. W. Schlichtmann, N. Kondru, M. M. Hepker, A. G. Kanthasamy, V. Anantharam and M. John, et al., Enzyme immunoassay-based platform for accurate detection of serum pathological α-synuclein in Parkinson's disease patients, ACS Chem. Neurosci., 2020, 11(24), 4179–4190 CrossRef CAS PubMed.
- C.-W. Chang, S.-Y. Yang, C.-C. Yang, C.-W. Chang and Y.-R. Wu, Plasma and serum alpha-synuclein as a biomarker of diagnosis in patients with Parkinson's disease, Front. Neurol., 2020, 10, 1388 CrossRef PubMed.
- Y. Herrmann, T. Bujnicki, C. Zafiu, A. Kulawik, K. Kühbach and L. Peters, et al., Nanoparticle standards for immuno-based quantitation of α-synuclein oligomers in diagnostics of Parkinson's disease and other synucleinopathies, Clin. Chim. Acta, 2017, 466, 152–159 CrossRef CAS PubMed.
- S. J. Lincoln, O. A. Ross, N. M. Milkovic, D. W. Dickson, A. Rajput and C. A. Robinson, et al., Quantitative PCR-based screening of α-synuclein multiplication in multiple system atrophy, Parkinsonism Relat. Disord., 2007, 13(6), 340–342 CrossRef PubMed.
- J. L. Hammond, N. Formisano, P. Estrela, S. Carrara and J. Tkac, Electrochemical biosensors and nanobiosensors, Essays Biochem., 2016, 60(1), 69–80 CrossRef PubMed.
- E. T. da Silva, D. E. Souto, J. T. Barragan, F. de, J. Giarola, A. C. de Moraes and L. T. Kubota, Electrochemical biosensors in point-of-care devices: recent advances and future trends, ChemElectroChem, 2017, 4(4), 778–794 CrossRef CAS.
- P. Skládal, Advances in electrochemical immunosensors, Electroanalysis, 1997, 9(10), 737–745 CrossRef.
- J. Wang, Nanomaterial-based amplified transduction of biomolecular interactions, Small, 2005, 1(11), 1036–1043 CrossRef CAS PubMed.
- C.-Y. Ge, M. M. Rahman, W. Zhang, N. S. Lopa, L. Jin and S. Yoon, et al., An electrochemical immunosensor based on a self-assembled monolayer modified electrode for label-free detection of α-synuclein, Sensors, 2020, 20(3), 617 CrossRef CAS PubMed.
- Y. An, X. Jiang, W. Bi, H. Chen, L. Jin and S. Zhang, et al., Sensitive electrochemical immunosensor for α-synuclein based on dual signal amplification using PAMAM dendrimer-encapsulated Au and enhanced gold nanoparticle labels, Biosens. Bioelectron., 2012, 32(1), 224–230 CrossRef CAS PubMed.
- E. D. Aminabad, A. Mobed, M. Hasanzadeh, M. A. H. Feizi, R. Safaralizadeh and F. Seidi, Sensitive immunosensing of α-synuclein protein in human plasma samples using gold nanoparticles conjugated with graphene: an innovative immuno-platform towards early stage identification of Parkinson's disease using point of care (POC) analysis, RSC Adv., 2022, 12(7), 4346–4357 RSC.
- M. Safavieh, M. K. Kanakasabapathy, F. Tarlan, M. U. Ahmed, M. Zourob and W. Asghar, et al., Emerging loop-mediated isothermal amplification-based microchip and microdevice technologies for nucleic acid detection, ACS Biomater. Sci. Eng., 2016, 2(3), 278–294 CrossRef CAS PubMed.
- B. Mojsoska, S. Larsen, D. A. Olsen, J. S. Madsen, I. Brandslund and F. A. Alatraktchi, Rapid SARS-CoV-2 detection using electrochemical immunosensor, Sensors, 2021, 21(2), 390 CrossRef CAS PubMed.
- S. Osaki, S.-i Wakida, M. Saito and E. Tamiya, Towards on-site determination of secretory IgA in artificial saliva with gold-linked electrochemical immunoassay (GLEIA) using portable potentiostat and disposable printed electrode, Appl. Biochem. Biotechnol., 2021, 193, 1311–1320 CrossRef CAS PubMed.
- V. B. C. Lee, N. F. Mohd-Naim, E. Tamiya and M. U. Ahmed, Trends in paper-based electrochemical biosensors: from design to application, Anal. Sci., 2018, 34(1), 7–18 CrossRef CAS PubMed.
- C.-H. Wu, W.-H. Wang, C.-C. Hong and K. C. Hwang, A disposable breath sensing tube with on-tube single-nanowire sensor array for on-site detection of exhaled breath biomarkers, Lab Chip, 2016, 16(22), 4395–4405 RSC.
- W. Hu, Z. Lu, Y. Liu, T. Chen, X. Zhou and C. M. Li, A portable flow-through fluorescent immunoassay lab-on-a-chip device using ZnO nanorod-decorated glass capillaries, Lab Chip, 2013, 13(9), 1797–1802 RSC.
- M. Hasanzadeh and N. Shadjou, Electrochemical and photoelectrochemical nano-immunesensing using origami paper based method, Mater. Sci. Eng. C, 2016, 61, 979–1001 CrossRef CAS PubMed.
- J. Qi, B. Li, N. Zhou, X. Wang, D. Deng and L. Luo, et al., The strategy of antibody-free biomarker analysis by in-situ synthesized molecularly imprinted polymers on movable valve paper-based device, Biosens. Bioelectron., 2019, 142, 111533 CrossRef CAS PubMed.
- Y.-S. Choi, M. R. Lee, C. S. Kim and K.-H. Lee, Detection of proline using a novel paper-based analytical device for on-site diagnosis of drought stress in plants, Rev. Sci. Instrum., 2019, 90(4), 045002 CrossRef PubMed.
- A. Yakoh, U. Pimpitak, S. Rengpipat, N. Hirankarn, O. Chailapakul and S. Chaiyo, based electrochemical biosensor for diagnosing COVID-19: Detection of SARS-CoV-2 antibodies and antigen, Biosens. Bioelectron., 2021, 176, 112912 CrossRef CAS PubMed.
- S. Boonkaew, I. Jang, E. Noviana, W. Siangproh, O. Chailapakul and C. S. Henry, Electrochemical paper-based analytical device for multiplexed, point-of-care detection of cardiovascular disease biomarkers, Sens. Actuators, B, 2021, 330, 129336 CrossRef CAS.
- S. Hassanpour, M. Hasanzadeh, A. Saadati, N. Shadjou, J. Soleymani and A. Jouyban, A novel paper based immunoassay of breast cancer specific carbohydrate (CA 15.3) using silver nanoparticles-reduced graphene oxide nano-ink technology: A new platform to construction of microfluidic paper-based analytical devices (μPADs) towards biomedical analysis, Microchem. J., 2019, 146, 345–358 CrossRef CAS.
- F. R. Maia, R. L. Reis and J. M. Oliveira, Nano inks for tissue engineering, Smart Multifunctional Nano-Inks, 2023, p. 505 Search PubMed.
- X.-F. Zhang, Z.-G. Liu, W. Shen and S. Gurunathan, Silver nanoparticles: synthesis, characterization, properties, applications, and therapeutic approaches, Int. J. Mol. Sci., 2016, 17(9), 1534 CrossRef PubMed.
- N. I. Ikhsan, P. Rameshkumar and N. M. Huang, Electrochemical properties of silver nanoparticle-supported reduced graphene oxide in nitric oxide oxidation and detection, RSC Adv., 2016, 6(108), 107141–107150 RSC.
- R. Eivazzadeh-Keihan, E. B. Noruzi, E. Chidar, M. Jafari, F. Davoodi and A. Kashtiaray, et al., Applications of carbon-based conductive nanomaterials in biosensors, Chem. Eng. J., 2022, 442, 136183 CrossRef CAS.
- V. Polshettiwar, D. Cha, X. Zhang and J. M. Basset, High-surface-area silica nanospheres (KCC-1) with a fibrous morphology, Angew. Chem., Int. Ed., 2010, 49(50), 9652–9656 CrossRef CAS PubMed.
- V. Polshettiwar and J.-M. Basset, High surface area fibrous silica nanoparticles, US8883308B2, 2014.
- J. Soleymani, M. Hasanzadeh, M. H. Somi, N. Shadjou and A. Jouyban, Highly sensitive and specific cytosensing of HT 29 colorectal cancer cells using folic acid functionalized-KCC-1 nanoparticles, Biosens. Bioelectron., 2019, 132, 122–131 CrossRef CAS PubMed.
- M. B. Behyar, F. Farshchi and M. Hasanzadeh, Sensitive recognition of prostate-specific antigen using biotinylated antibody encapsulated on D-penicillamine decorated wrinkled silicate nanoparticles (WSN): An innovative sandwich-type biosensor toward diagnosis of prostate cancer, J. Mol. Recognit., 2022, 35(8), e2960 CrossRef CAS PubMed.
- M.-H. Moosavy, M. Hasanzadeh, J. Soleymani and A. Mokhtarzadeh, Determination of aflatoxin M1 using an aptamer-based biosensor immobilized on the surface of dendritic fibrous nano-silica functionalized by amine groups, Anal. Methods, 2019, 11(30), 3910–3919 RSC.
- F. Farshchi, A. Saadati and M. Hasanzadeh, An innovative Immunoanalysis strategy towards sensitive recognition of PSA biomarker in human plasma samples using flexible and portable paper based biosensor: a new platform towards POC detection of cancer biomarkers using integration of pen-on paper technology with immunoassays methods, ImmunoAnalysis, 2021, 1(1), 6 CrossRef.
- M. Anvari Gharabaghlou, N. Shadjou and A. Poursattar Marjani, Appl. Organomet. Chem., 2020, 34(10), e5868 CrossRef CAS.
- S. M. Taghdisi, N. M. Danesh, M. A. Nameghi, M. Ramezani, M. Alibolandi and M. Hassanzadeh-Khayat, et al., A novel electrochemical aptasensor based on nontarget-induced high accumulation of methylene blue on the surface of electrode for sensing of α-synuclein oligomer, Biosens. Bioelectron., 2019, 123, 14–18 CrossRef CAS PubMed.
- S. Li and K. Kerman, Electrochemical detection of interaction between copper (II) and peptides related to pathological α-synuclein mutants, Anal. Chem., 2019, 91(6), 3818–3826 CrossRef CAS PubMed.
- Q. Xu, S. Evetts, M. Hu, K. Talbot, R. Wade-Martins and J. J. Davis, An impedimetric assay of α-synuclein autoantibodies in early stage Parkinson's disease, RSC Adv., 2014, 4(102), 58773–58777 RSC.
- T. Bryan, X. Luo, L. Forsgren, L. A. Morozova-Roche and J. J. Davis, The robust electrochemical detection of a Parkinson's disease marker in whole blood sera, Chem. Sci., 2012, 3(12), 3468–3473 RSC.
|
This journal is © The Royal Society of Chemistry 2024 |