DOI:
10.1039/D3PM00024A
(Review Article)
RSC Pharm., 2024,
1, 9-36
Nanotechnology-driven wound healing potential of asiaticoside: a comprehensive review
Received
23rd October 2023
, Accepted 12th February 2024
First published on 26th February 2024
Abstract
Asiaticoside (AC) is a naturally occurring phytoconstituent that aids in wound healing by stimulating collagen biosynthesis. However, the physical properties of AC, such as its high molecular weight (959.12 g mol−1), poor water solubility, and low permeability, restrict its therapeutic benefits. Additionally, the management of inflammation and angiogenesis in wound healing using AC-loaded wound dressings can be challenging in terms of its delivery across the skin layers. These challenges can be rectified by utilizing nanotechnology. The concept of nanotechnology is widely utilized in dermatology to boost the therapeutic efficacy of the entrapped drug. The AC-loaded nano-carriers deliver the drug at their target site in order to increase their efficacy, stability, and safety. These carriers efficiently distribute the loaded drug to the different skin layers. The current review focuses on the limitations associated with the topical administration of asiaticoside and the many initiatives made so far for effective and safe topical delivery using innovative constituents and techniques, along with other potential benefits of AC in wound healing, diabetes, inflammation, and depression.
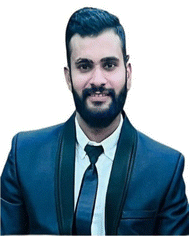 Mohit Kumar | Mohit Kumar is a PhD scholar and research assistant in the Indian Council of Medical Research-sponsored project at the Department of Pharmaceutical Sciences & Technology, Maharaja Ranjit Singh Punjab Technical University, Bathinda, India. With a remarkable four years of research background in pharmaceutical sciences and drug delivery, Mohit has dedicated his expertise to addressing the global concern of burn-related wound infections, affecting approximately 11 million people globally every year. His prolific academic journey includes the authorship of 8 book chapters and 39 publications, published in high-impact science journals. His outstanding contributions have earned him prestigious accolades such as the Young Researcher Award at an international conference in Pondicherry (India 2023) and the Best Paper Award at ICONICA 2020 India. He has been recognized by the Central Library of Medicine Foundation of Argentina for groundbreaking research focused on perfumes. |
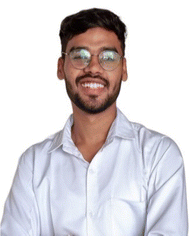 Devesh Kumar | Devesh Kumar is an M. Pharmacy Research Scholar in the Department of Pharmaceutical Sciences & Technology, Maharaja Ranjit Singh Punjab Technical University, Bathinda, India. His academic journey includes the authorship of 1 book chapters and 7 publications published in high-impact science journals. His research interests are focused on the design of multifunctional peptides and nanomaterials in biomedicine. |
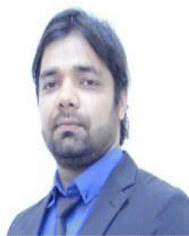 Syed Mahmood | Dr Syed Mahmood completed a Bachelor's in Pharmacy in 2011 and a Master's in Pharmacy (Pharmaceutics) in 2013. He also holds a post-graduate diploma in Pharmaceutical Drug Regulatory Affairs. In 2014 he started his PhD and completed it in 2017. His PhD research was on drug delivery and technologies. After his PhD, he worked at University Malaysia Pahang, as a senior lecturer teaching in the pharmaceutical engineering department. His specialization is in the field of pharmaceutical technology and engineering areas which include drug delivery animal models, analytical chemistry (LC-MS, HPLC), wound healing studies (hydrogel and nanofibers) and system validation and qualification. Currently he is working at Universiti Malaya in the pharmacy faculty attached to the pharmaceutical technology department. |
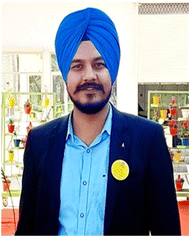 Varinder Singh | Dr Varinder Singh is working as Assistant Professor at the Department of Pharmaceutical Sciences & Technology, Maharaja Ranjit Singh Punjab Technical University, Bathinda, Punjab. He obtained his Ph.D. (Pharmaceutical Sciences) from Punjabi University, Patiala, Punjab, India. His research interests primarily lie in the discovery and development of natural products for therapeutic use. He is well-versed in the fields of natural product chemistry (isolation, and characterization of bioactive natural compounds), drug discovery, and pharmacology. He has a strong record of publications in high-impact peer-reviewed journals (40 peer-reviewed publications), with many of his papers cited extensively in the field of natural products. He has 1 patent granted. He has been awarded numerous travel grants by government and non-government organisations including Alzheimer's Association International, DST, ICMR and IBRO. |
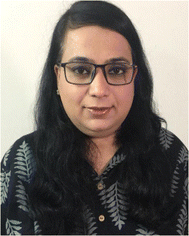 Shruti Chopra | Dr Shruti Chopra is working as Assistant Professor with the Department of Pharmaceutical Sciences & Technology, Maharaja Ranjit Singh Punjab Technical University, Bathinda, Punjab. Her area of expertise is pharmaceutical analysis, particularly analytical method development & validation, liquid chromatography and mass spectroscopy (LC-MS). She is a recipient of the Startup Young Scientist project by the Science and Engineering Research Board (SERB), Government of India. She is guiding three doctorate scholars and has guided 1 PhD and 10 M. Pharm. theses. Dr Chopra has 5 patents and 37 publications in reputed international as well as national journals with an equal number of presentations and invited lectures to her credit. She is member of editorial and reviewers’ boards of various prestigious journals. |
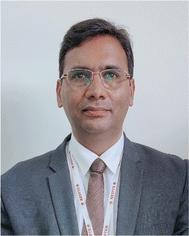 Amit Bhatia | Dr Amit Bhatia is working as a Professor at the Department of Pharmaceutical Sciences & Technology, Maharaja Ranjit Singh Punjab Technical University, Bathinda, Punjab. Dr Bhatia has been working in the area of drug delivery, formulation development and strategies. He has gained vast and varied exposure through countrywide interaction with regulatory and research experts while leading initiatives contributing to strategic inputs and operational oversight in formulation development. He has transferred 3 technologies to industry and has 7 patents granted to him. He has guided 3 PhD and 23 PG students. He has published 70 research and review articles, in prestigious journals. He is on the board of the reviewers’ panel for various international journals of high repute. He has delivered various invited lectures on research and academics at different institutions. |
1. Introduction
A wound refers to a physical trauma or harm to the body that disrupts the structure of the skin or underlying tissues. Injuries may arise from several factors, such as accidents, trauma, surgeries, or underlying medical disorders.1 They manifest in different forms, such as incisions resulting from sharp objects, lacerations with jagged edges caused by blunt force, abrasions from friction against rough surfaces, contusions or bruises. These result in damaged blood vessels, punctures from pointed objects, avulsions where a portion of skin or tissue is forcefully torn away, and gunshot wounds inflicted by projectiles fired from firearms.2,3 The degree of a wound may range from superficial lacerations and abrasions that can be treated with simple first aid whereas more intricate injuries require expert medical intervention. Appropriate wound management is crucial for preventing infections, promoting healing, and minimising the risk of complications.4 Despite advancements in wound dressing and healthcare, wounds continue to be a global concern. The global prevalence and incidence of wounds are detailed in Table 1.
Table 1 Global prevalence and incidence of wounds
Type of wound |
Population demographics |
Incidence rate |
Prevalence rate (globally) |
Risk factors |
Geographic distribution |
Ref. |
Venous leg ulcers |
Adult population aged >65 years |
0.17% overall |
3%–4% |
Family history, obesity, deep venous thrombosis, and increasing age |
England, Western countries |
5 and 6 |
Diabetic foot ulcers |
Adult population aged 45 years and above |
2–5% annually |
6.3% |
Poor glycemic control, calluses, foot deformities, improper foot care, ill-fitting footwear, underlying peripheral neuropathy and poor circulation, dry skin |
Highest in North America, Belgium, Canada and the USA |
5 and 7 |
Lowest in Asia, Europe, Africa and Oceania |
Pressure ulcers |
Senior population aged above 70 years |
Ranged 4.5%–78.4% |
5.2%–12.3% at admission and at discharge respectively |
Immobility, incontinence, lack of sensory perception, poor nutrition and hydration, and medical conditions affecting blood flow |
Highest in US as compared to 195 other low, middle, and high sociodemographic index countries |
5, 8 and 9 |
Surgical wound infection |
Adult population aged >50 |
2.5% |
0.5–3% |
Patient-related factors include existing infection, low serum albumin concentration, older age, obesity, smoking, diabetes mellitus, and ischemia secondary to vascular disease or irradiation |
Africa and US |
10 and 11 |
Burn wound infections |
All ages (mostly adults injured with flame burns and young children with scald burns) |
26.93% |
11 million annually |
Nosocomial infection, immunocompromised patients (burn injury, cutaneous and respiratory tract injury, long Intensive Care Unit (ICU) stays) |
Africa, Australia & New Zealand, Asia, Europe, Middle and South America, North America |
12 and 13 |
Arterial ulcers |
Geriatric population |
12–14% |
0.4% to 0.8% |
Atherosclerosis, hypertension, diabetes, and atrial fibrillation, all of which are more prevalent in the geriatric population |
USA |
14 and 15 |
1.1 Wound classification
Wounds can be classified as either acute or chronic, depending on how long they take to heal.16 Acute wounds are caused by various factors such as surgical procedures, mechanical trauma, or burns, and they follow a predictable healing process.17 Chronic wounds occur when there is a failure in the processes involved in tissue repair.18 Certain medical conditions can hinder the healing process and lead to persistent wounds, such as infections caused by microorganisms,19 venous or arterial disorders,20 and diabetes.21 The characteristics of acute and chronic wounds are shown in Table 2.
Table 2 The characteristics of chronic and acute wounds
Characteristics |
Acute wound |
Chronic wound |
Ref. |
Onset |
Usually short, it goes through every stage that regular wound healing goes through |
Prolonged treatment, may cause a delay in one or more healing stages, leaving a permanent disorder |
22 and 23 |
The stages of infection |
Normally there are 4 stages – haemostasis, inflammation, proliferation, and remodelling |
There is prolonged inflammation, and inadequate proliferation and remodelling phase |
24
|
Inflammatory response |
Generally, heals quickly as a part of the healing process |
It is normal for chronic inflammation to persist and obstruct recovery |
25 and 26 |
Appearance of the wounds |
Typically, there is little tissue loss, and the outer edges are clean |
Irregular wound edges, often accompanied by venous tracts, undermining, or tissue necrosis |
27 and 28 |
Duration of wound healing |
Three months in cases of acute wound |
Three months or longer, and up to seven months |
29
|
Skin pathology related to the epidermis |
The epithelial layer in cases of acute wound remains normal |
Hyperkeratotic (in which the outer layer becomes thicker) or parakeratotic (anucleate keratinocytes) |
30
|
Granulation tissue |
Normal production |
Hypoxia of the tissue due to low oxygen levels, fibroblasts, and neoangiogenesis |
31
|
Tissue hypoxia: insufficient granulation tissue formation |
Immunology |
Normal |
Immunology is not normal |
4
|
Clinical examples |
Abrasions, surgical wounds superficial burns |
Cardiovascular lower-limb ulcers, diabetes wounds, heterogeneous aetiology ulcers, ulcers caused by pressure, infections in wounds |
32
|
Wounds, which are frequently caused by tissue injury, recover through a series of intricate processes involving the interplay between growth factors and cytokines. These processes replace the injured cells and restore the organ's integrity.33–35 The intricate wound healing system has been divided into four stages: inflammation, proliferation and re-epithelialization, and tissue remodelling. Although these mechanisms try to replace damaged tissue at the wound site, they often result in scarring (particularly in adults). Scars are one of the leading causes of physical, psychological, and physiological illness.36,37 While the process of wound healing and scar formation, as well as how to avoid them, are not entirely understood, advances in the underlying mechanism have resulted in successful therapies. The major cause of dermal injuries associated with burns, surgery, and trauma frequently results in scar development during the healing process, which reduces the functionality of normal skin and causes aesthetic and psychological damage.38,39 Numerous therapeutic interventions and non-surgical methods, such as silicon dressings, radiation, laser therapy, and radiotherapy, have been used for scarless repair.40–44
Wound healing is a dynamic and complicated process that involves a balance of different cells, cytokines, growth factors, pathways, and extracellular matrix (ECM) formation.45 The pro-inflammatory cytokines interleukin-6 (IL-6) and IL-8 promote scarring, whereas the anti-inflammatory cytokine IL-10 has the opposite effect.46–48 Less scar tissue has developed as a result of the simultaneous addition of several growth factors,49–51 cytokines, and cells to wound matrices, but the problem of scarless outcomes has not yet been resolved.52 As the healing process progresses through remodeling phases, inflammatory responses and angiogenesis undergo dynamic alterations at various stages to affect the development of the extracellular matrix (ECM) and, eventually, scar formation.53,54 The complex variables (growth factors, cytokines, cells, and ECM) control angiogenesis and inflammation at different phases of the healing process to determine the outcomes of scars.55,56 To promote the healing of scarless wounds, a variety of biomaterials, together with cell-laden matrices, have been developed to actively control angiogenesis and inflammation. These matrices typically generate suitable microenvironments for wound healing.55
Recently, there has been an increase in interest in and demand for herbal medicines due to several reasons. These include claims about their effectiveness, a change in patients’ preferences towards natural remedies, the expensive and adverse effects of modern medicines, and the progress made in herbal medicines through scientific research and technological advancements.57 The diverse range of chemicals found in phytochemicals and organically produced compounds provide notable benefits in facilitating the process of wound healing. These compounds contribute to the recovery process through a variety of mechanisms, making them advantageous for promoting overall healing and tissue repair.33,58 For example, in one study, Khan et al. investigated the wound-healing potential of Berberis lycium extract. The authors revealed that the prepared polyvinyl alcohol nanofibers loaded with Berberis lycium possess excellent wound healing and antibacterial activity.59 In another study, Manconi and colleagues investigated the use of baicalin for the treatment of wounds.60 The same research discovered that baicalin had skin-restorative capabilities utilizing the nanohydrogel platform.61 Medicinal plants used to treat wounds have been demonstrated to be effective in combating infection and accelerating wound healing.62,63 Among the commonly accessible therapeutic herbs, it has been claimed that extracts of Centella asiatica (L.) (CA) Urban, also known as Asiatic Penny-wort,64,65 belonging to the Apiaceae family, have qualities capable of curing specific ailments, such as ulcerous skin abnormalities,66 burns-related wounds,67 duodenal and stomach ulcers.68 These effects of Centella asiatica (L.) have been attributed to the presence of AC, an important compound. AC is a triterpene glycoside compound found in various plant species, most notably in Centella asiatica (L)., commonly known as gotu kola. Gotu kola is a small herbaceous plant that has been traditionally used in traditional medicine systems in Asia for its potential health benefits.69 The biological activities of AC are diverse and include anti-inflammatory,70 antioxidant action,71 stimulation of collagen,72 and angiogenesis production.73 AC has been utilized therapeutically to promote the healing of wounds and lessen the development of scar tissue. However, the therapeutic use of AC as a topical medication or as a bioactive ingredient in wound matrices and dressings is restricted by its insolubility in water.74 In addition, various studies have revealed that AC loaded into wound dressings and in liposomal carriers improved wound healing processes; however, these face challenges in managing inflammation and angiogenesis during wound healing.75,76 This could be due to poor penetration of AC across the skin. Improving the loading and release management of AC would lead to better results for scarless wound healing. One solution to this problem is to use nanotechnology, which can improve the delivery of drug to the target site, and increase its efficacy, stability, and safety.77 Nanocarriers play an important role in enhancing drug penetration through the stratum corneum, the skin's outermost layer.78 The stratum corneum is a major barrier, limiting the passage of drugs. Nanocarriers circumvent this difficulty by using their distinct characteristics and interactions with the skin.79 Nanocarriers interact more favourably with the stratum corneum due to their smaller particle size, which allows deeper penetration.80 Furthermore, the lipid-based composition of many nanocarriers allows them to fuse with the lipids in the stratum corneum, increasing drug diffusion through intercellular gaps.81 Some nanocarriers can also encapsulate both hydrophilic and hydrophobic drugs, making them suitable for a wider spectrum of therapeutic agents. Furthermore, surface changes to nanocarriers may be designed to improve adhesion and contact with the skin, hence enhancing drug retention and penetration.82 Nanocarriers control the release of loaded drug which also helps to ensure long-term drug delivery and therapeutic benefits.83 Overall, nanocarriers provide a viable pathway for improving drug absorption across the stratum corneum, furthering the science of transdermal drug administration with potential uses in dermatology.84 In this review, the authors discussed the limitations of the topical administration of AC and highlighted various initiatives for effective and safe topical delivery using novel techniques, along with other potential benefits of AC in managing diabetes, inflammation, and depression. The authors gathered information about AC from articles published up to 2024 and listed in PubMed, Web of Science, Elsevier, Google Scholar and similar databases. The keywords used in our search included “asiaticoside”, “Centella asiatica”, “wound healing”, “anti-inflammatory”, “neuroprotective”, “skin”, etc. By carefully analyzing the research on asiaticoside, it was found that there is an increase in AC-based research, which can be demonstrated by contemplating the PubMed search engine results using asiaticoside in wound healing as a keyword (Fig. 1).
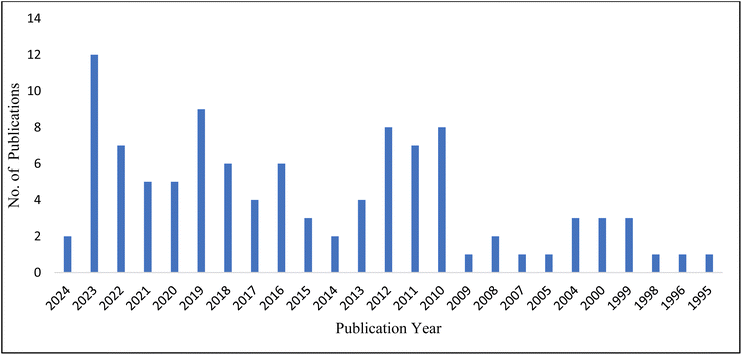 |
| Fig. 1 PubMed search engine result demonstrating research publications published w.r.t. years starting from 1995 to 2024. | |
2. Phases of wound healing
2.1 Phase 1: haemostasis phase
Haemostasis is a vital physiological response triggered when blood is present outside the body or escapes from the blood vessels, serving as the body's innate mechanism to stop bleeding and prevent excessive blood loss.39,85 This complex process unfolds in three rapid and sequential steps. The initial response involves a vascular spasm, where blood vessels constrict to minimize blood loss. Following this, the second step, platelet plug formation, occurs as platelets adhere together to create a temporary seal, covering the breach in the vessel wall.85 Platelet plug formation is a crucial step in the haemostatic process, activated by the presence of von Willebrand factor (vWF), a glycoprotein found in plasma. Platelets play a pivotal role in haemostasis, undergoing significant changes when encountering injured endothelial cells.86 Upon activation, platelets change shape, release granules, and acquire a “sticky” quality. Platelets express receptors, including glycoprotein receptors, facilitating their adhesion to collagen. Activated platelets, in turn, express glycoprotein receptors that enable interactions with other platelets, leading to aggregation and adhesion,87 and release cytoplasmic granules, such as adenosine diphosphate (ADP), serotonin, and thromboxane A2. ADP attracts more platelets to the affected area, serotonin acts as a vasoconstrictor, and thromboxane A2 assists in platelet aggregation, vasoconstriction, and degranulation.22,88,89 This release of chemicals creates a positive feedback loop, as more platelets are attracted to the site, adhere, and release their chemicals, reinforcing the platelet plug. This intricate process highlights the dynamic and coordinated role of platelets in ensuring effective haemostasis and preventing excessive bleeding.90 The third and final step, known as coagulation or blood clotting, reinforces the platelet plug by introducing fibrin threads that act as a molecular glue to solidify the clot.91 Within seconds of vessel wall disruption, platelets adhere to the sub-endothelial surface, and within minutes, fibrin strands intersperse among the wound, completing the formation of the platelet plug.92 This orchestrated sequence ensures effective haemostasis, safeguarding the body against excessive bleeding and contributing to the overall maintenance of vascular integrity.93
2.2 Phase 2: inflammatory phase
The inflammatory phase is a critical component of the body's response to injury, infection, or tissue damage, and is responsible for initiating the healing process. This dynamic phase is distinguished by a series of processes coordinated by the immune system.85 Blood arteries dilate when injured, resulting in increased blood flow to the damaged region. This vasodilation is accompanied by increased permeability, which allows immune cells like neutrophils and macrophages to move to the area of damage.94 These immune cells are essential for removing debris, infections, and injured tissue. Chemical mediators such as cytokines and prostaglandins help to attract and activate immune cells, which amplifies the inflammatory response.95 While inflammation is a defensive process that helps to eliminate risks and initiate healing, a too lengthy or severe inflammatory response may contribute to chronic diseases. The inflammatory phase prepares the groundwork for subsequent stages of tissue repair by stimulating the elimination of injured cells and providing an environment favourable to tissue regeneration and healing. Balancing the inflammatory response is critical for effective wound healing and avoiding challenges caused by chronic inflammation.96
2.3 Phase 3: proliferative phase
The proliferative phase, which occurs after the inflammatory phase, is characterised by the active repair and regeneration of injured tissue. Several cellular activities take place during this period to aid in tissue healing.97 Fibroblasts, which are specialised cells responsible for collagen production, move to the wound site and form a new extracellular matrix, providing structural support to the healing tissue.98 Angiogenesis, or the development of new blood vessels, occurs to restore the vascular network required for nutrition delivery to the healing region.99 Furthermore, epithelial cells within the wound boundaries multiply and move to cover the wound surface, providing a protective layer. The granulation tissue, which is rich in blood vessels and fibroblasts, covers the wound gap and acts as a temporary scaffold for tissue repair.100 The proliferative phase is distinguished by an integrated approach of cellular activity, extracellular matrix synthesis, and angiogenesis to restore and reinforce the injured tissue. This phase provides the groundwork for the ensuing remodelling phase, which ensures the restoration of tissue integrity and function.101 Proper synchronisation of these processes is required for effective wound healing and to avoid excessive scarring.102
2.4 Phase 4: maturation phase
The maturation phase, also known as the remodelling phase, is the final step of wound healing, after the inflammatory and proliferative stages.103 It entails gradually reshaping and refining the newly developed tissue in order to improve its strength and effectiveness. During maturation, collagen fibres undergo reorganisation and cross-linking, which increases the tensile strength and endurance of the repaired tissue.104 This phase is distinguished by the elimination of superfluous or excess collagen and cells by apoptosis, a programmed cell death process. The tissue is constantly altered to better tolerate mechanical forces and recover its original structure. While the maturation phase can take weeks, months, or even years, the ultimate aim is to have repaired tissue that nearly mimics its original, uninjured shape.105 The maturation phase is critical for improving the quality of tissue healing and reducing scar formation, assuring the regenerated tissue's long-term functioning and durability. Proper wound care and support during this phase help to achieve the ideal balance of tissue strength and flexibility in the ultimate healed result.1,106 The schematic diagram of wound healing phases is depicted in Fig. 2.
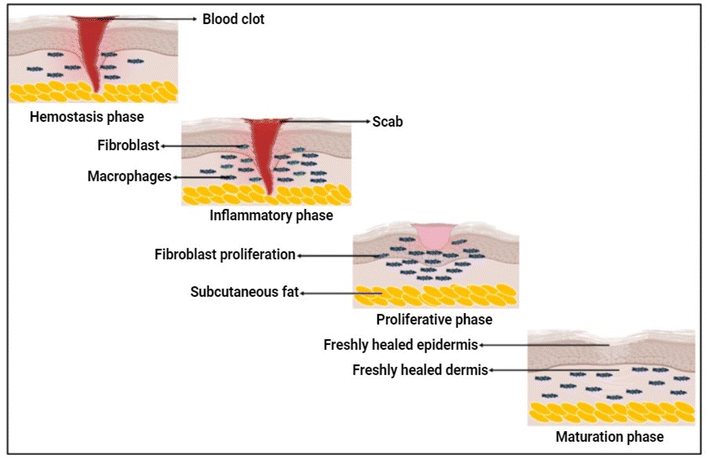 |
| Fig. 2 Phases of wound healing. | |
3. Method of extracting asiaticoside
Extraction is the process of carefully and deliberately extracting certain components or substances from a larger entity, such as a natural resource, combination, or chemical. In general, plant material is extracted by washing the analyte from the matrix into the solvent and diffusion through the cell wall.107 Many methods are adopted for the extraction of AC.
3.1 Maceration
Maceration is a technique used in a variety of areas, most notably culinary arts, pharmacy, and herbal medicine. This method involves the soaking of an item, usually plant material, in a liquid to soften and break down its cellular structure, enabling the extraction of flavours, fragrances, or active molecules.108 Maceration is a popular procedure used in pharmaceutical and herbal applications to extract therapeutic components from plants using solvents such as alcohol or oil. This gentle but efficient method allows for the absorption of beneficial elements, making maceration a flexible and commonly used treatment in both ancient and contemporary settings.109 Organic solvents such as methanol, ethanol, or a mixture of water and alcohol are employed when dealing with CA. Typically, aqueous extracts produced via this method exhibit cytotoxic and antioxidant properties. The cosmetics industry has also implemented maceration, using propylene glycol and water as solvents for extracting the leaves and stalks of CA over the course of several days.110 In one study, Pittella et al. isolated flavonoid and phenolic components from CA. Additionally, CA can be macerated to extract a wide range of compounds, including carotenoids, flavonoids, phenolics, saponins, alkaloids, and tannins, depending on the solvents employed and the duration of the extraction process.111
3.2 Distillation
Distillation is a prevalent separation technique that separates components of a mixture or purifies liquids by utilising differences in boiling points. It involves heating a liquid to produce vapour and then cooling that vapour to condense it back into a liquid state.112 Prior to the vaporisation of components with higher boiling points, those with lower boiling points persist in the liquid phase. The aforementioned method is frequently implemented in the manufacturing of compounds, intoxicating beverages, and essential oils. As a crucial method for extracting purified substances from complex mixtures, distillation is utilised in laboratories and across a variety of industries.113 In one study, dry and fresh leaves of CA were extracted by steam distillation with distilled water and vinegar. Using fresh leaves instead of dried ones during the extraction process facilitates the identification of numerous compounds. Steam distillation is an effective method for producing the highest-grade oil.114 In one study, authors extracted 47 components using distillation extraction.115
3.3 Soxhlet extraction
Soxhlet extraction is a widely utilised method for extracting compounds from solid materials. The process involves placing the solid sample in a thimble and repeatedly passing a solvent, typically an organic one such as hexane or ethanol, through the sample.116 The solvent evaporates, ascends through a vertical condenser, and then returns to the sample chamber. This continuous cycle enables efficient extraction of the desired compounds.117 Soxhlet extraction is particularly advantageous for extracting compounds that are not easily vaporised and are sensitive to heat. It is commonly employed in various fields, including chemistry, pharmaceuticals, and food analysis, to separate and concentrate specific components from solid matrices.118 In one study, Thamarai Selvi et al. discovered that by subjecting 500 g of powdered CA to Soxhlet extraction for 8 hours, using an ethanol-to-solid ratio of 1
:
4, they obtained extracts that included saponins, terpenoids, alkaloids, and phenols. However, these extracts did not contain steroids, flavonoids, tannins, proteins, carbohydrates, or glycosides.119 Rahman et al. conducted another study where they utilised 100% ethanol, 50% ethanol, and water as solvents for Soxhlet extraction in order to acquire total polyphenols, flavonoids, β-carotene, tannins, and vitamin C from CA. The research demonstrated that the 50% ethanol extract of CA exhibited a markedly elevated concentration of polyphenols and flavonoids, whilst the 100% ethanol extract yielded the greatest levels of β-carotene and tannins. In contrast, the water extract of CA exhibited a higher concentration of vitamin C compared with the 50% and 100% ethanol extracts.120
3.4 Ultrasonic extraction
Ultrasonic extraction is a contemporary and effective method used to extract chemicals from diverse materials, including plants, herbs, and seeds. This technique involves the application of ultrasonic waves to a liquid medium that contains the sample, resulting in the development and subsequent collapse of small bubbles, a phenomenon known as cavitation.121 Cavitation induces fast changes in pressure and temperature, generating microjets and shockwaves that aid in the liberation of bioactive substances from the solid matrix into the solvent. Ultrasonic extraction is renowned for its capacity to augment extraction rates and boost yields in comparison with conventional approaches. This procedure is extensively used in sectors such as food, pharmaceuticals, and cosmetics to get superior extracts while minimising the time and amount of solvents used in the process.122 In one study, Shen et al. utilised ultrasonic extraction for the extraction of asiaticoside. For ultrasonic extraction, 2.0 g of dried, finely powdered sample was mixed with 50 mL of a 9
:
1 mixture of methanol and water in a flask. The flask was then put in an ultrasonic bath for 1 hour. The extraction was done twice, and then the extracts were mixed. The combined extract was put through a filter, and the filtrate was evaporated using a rotary evaporator at 50 °C until it was dry. The residue was then mixed with 9 parts methanol and 1 part water to make 10 mL. Before analysis, the extract was filtered through a 0.45 μm nylon filter membrane.123
3.5 Microwave extraction
Microwave extraction (MAE) is a fast and efficient technique used to extract chemicals from diverse materials, including plants, seeds, and other organic components. During this procedure, the sample is exposed to microwave radiation in the presence of a suitable solvent.123 The microwaves generate thermal energy in the sample, resulting in expedited extraction operations. The approach is renowned for its capacity to substantially decrease extraction durations, boost product quantities, and optimise the overall efficacy of the extraction procedure.124 Microwave extraction is a widely used method in labs and enterprises due to its rapidity, accuracy, and efficiency in extracting desired chemicals from various sources.125 The powder sample (1.0 g) was transferred to the microwave extraction containers and suspended in 25 mL of a 9
:
1 methanol–water mixture. According to a pre-designed scientific trial, the temperature rises to 70 °C in 0–5 minutes, and then remains steady at 70 °C for 15 minutes. The required solvent quantities were introduced to the vessels and tested under MAE for 20 minutes. Following extraction, the containers were chilled to room temperature before being opened. The residue was then mixed with 9 parts methanol and 1 part water to make 10 mL. Before analysis, the extract was filtered through a 0.45 μm nylon filter membrane.123
3.5 Multi-step extraction method
Method-1.
Step-I: Dry Centella is mixed with ethanol in the concentration of 50%–90% v/v. The solution is filtered, then ethanol is removed via reduced pressure concentration, the ethanol-free concentrated liquid is diluted with water, and finally, the Centella total saponin water solution is obtained via centrifugal filtration. To obtain Centella total saponin water solution, the dry powder of Centella is mixed with ethanol in the concentration of 50–90% v/v, and then heat extracted 3 times, each for 180 minutes, and the united extraction liquid is evaporated to density 1.01–1.15 g mL−1, then diluted with water, and centrifuging obtains Centella total saponin water solution.
Step-II: Total saponin water solution is entered into the macroporous resin column (model AB-8, HPD100, fraction size – 0.3–1.2 mm), and the washed resin was colourless, indicating that the effluent liquid is free from any significant impurities. A 15–30% ethanol (v/v) and 50–80% ethanol (v/v) solution were used to wash post more respectively, and a 50–80% ethanol (v/v) elutriant was collected, pooled and concentrated to density 1.01–1.05 g ml−1; 3–5% activated carbon was added, and filtering while hot obtained the eluant in fractions (pressure 73.8 bar, temp. 70 °C).
Step-III: Take a 50%–80% (v/v) ethanol eluant, add activated carbon, and perform a decolourisation to obtain a column-loading liquid, filter the column-loading liquid, add the filtered liquid to a preparative chromatography column, with a filler of C18 bonded silica gel filler (preparative chromatography is used to purify sufficient quantities of a substance for further use, rather than analysis), and collect a fraction of the eluant containing madecassoside and asiaticoside using methanol/water as the moving phase; concentrating and drying then gives the resulting product. In this step, the consumption of 40–70% methanol (v/v) is 3–5 times the column volume. There are two sections of collection: the first is the asiaticoside component, and the second is the CA glucoside (madecassoside and asiaticoside) component; correspondingly, concentrate drying yields the product.126,127
4. Challenges associated with topical delivery of asiaticoside
The skin is the body's primary protective barrier, consisting of three major layers: the epidermis, dermis, and subcutaneous tissue. Each layer has unique structural properties that contribute to its barrier function.128,129 The epidermis, or outermost layer, is made up of densely packed cells known as keratinocytes, which provide mechanical strength and waterproofing. The stratum corneum, a specialised layer of the epidermis, is essential for restricting substance penetration. The dermis contains blood arteries, nerves, and connective tissue, while the subcutaneous tissue provides insulation.130,131
Skin barrier properties play an important role in controlling drug penetration. Because of its compact, lipid-rich composition, the stratum corneum serves as an effective barrier, preventing most substances from entering.132,133 Certain substances, however, can get inside the skin via a variety of pathways, including intercellular, transcellular, and appendageal. Intercellular permeation occurs between keratinocytes via the lipid matrix, while transcellular permeation occurs through the cells themselves.134,135 Appendageal channels, such as hair follicles and sweat glands, provide alternate penetration routes.136 Factors such as molecular size, lipophilicity, and charge impact compounds’ ability to cross the skin barrier, making knowing these routes critical for drug delivery and skincare applications.137
The major challenges associated with the topical delivery of AC are its insolubility and bioavailability.138 AC is a pentacyclic triterpene with a high molecular weight of 959.12 g mol−1 and is difficult to ionise; its maximum solubility in water is 307.347 pg mL−1 and it has a short plasma half-life. The equilibrium oil–water partition coefficient is 2.24.139 Poor solubility in aqueous and oil media reduces dose-dependent effects and absorption, severely limiting its applicability and mechanistic investigation. The low water solubility of drugs has always posed a significant challenge to the development of drug delivery devices. Poor solubility may restrict drug dissolution in vivo, resulting in limited absorption, which can pharmacologically impair the therapeutic effectiveness of the medicine.140 The drug's physicochemical characteristics, such as pKa, solubility, log
P and molecular mass, are also significant when choosing the components for the topical delivery vehicle.141,142 Many chemicals lack the physical parameters [such as low molecular weight, appropriate lipophilicity [log
P (o/w = 1–3)], and low melting point] to passively penetrate the skin at therapeutic levels, restricting the topical delivery of drugs.143 The development of technologies to enhance delivery into the skin has been a major research focus for over half a century. ‘Passive’ technologies involve the use of formulation excipients, chemical penetration enhancers, and various types of micro and nano-delivery system.144 When choosing an excipient for a topical vehicle for acidic and unstable drugs, special care must be taken to ensure that it will not only mask the drug's acidic group's potential for irritation but also offer the environment for efficient topical delivery and the preservation of chemical integrity.145,146 The many difficulties presented by AC while creating the optimal topical formulations are shown in Fig. 3.
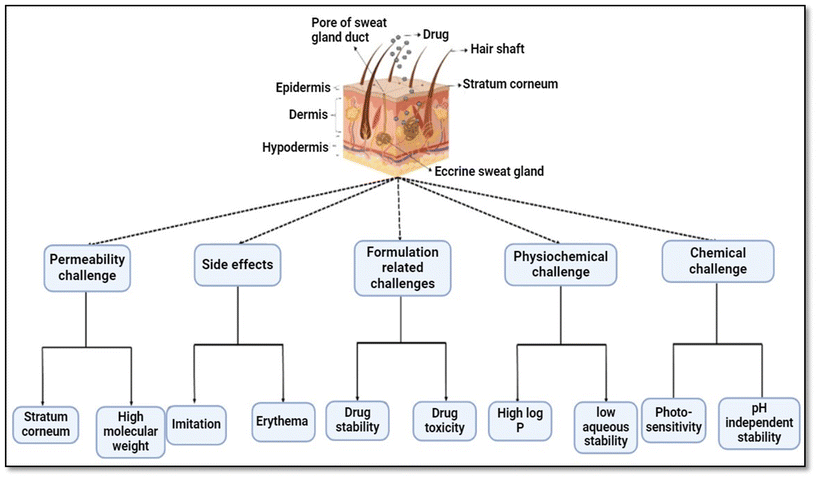 |
| Fig. 3 Challenges associated with the topical delivery of asiaticoside.147–149 | |
5. Wound healing potential of asiaticoside
AC is well-known for its anti-keloid and anti-hypertrophic scar properties.150 Many researchers discovered that AC improved initial skin cell adhesion and increased the quantity of normal human dermal fibroblasts.151,152 Initial cell adhesion is the process by which cells interact and attach to neighbouring cells through specialized molecules (cadherins, integrins and selectins) of the cell surface such as cell junctions, or through indirect interaction, where cells attach to the surrounding extracellular matrix, a gel-like structure containing molecules released by the cells into the spaces between them.153,154 AC increases the skin cell activity involved in wound healing and may have therapeutic benefits.155 Shukla et al. reported the wound-healing activity of AC. AC (0.2%, topical) application to rats (excision-type wound) resulted in improved enzymatic and non-enzymatic antioxidants which ultimately help in healing.156 Ruszymah et al.157 investigated the effects of CA on the proliferation and migration of rabbit corneal epithelial (RCE) cells. The CA was utilised in different concentrations such as 7.8, 15.6, 31.2, 62.5, 125, 250, 500, and 1000 ppm (ppm – part pers million). The study revealed that the proliferation of RCE cells was not substantially impacted by CA supplementation at concentrations up to 500 ppm. However, the proliferation of rabbit corneal epithelial (RCE) cells was significantly inhibited at a concentration of 1000 ppm. Nevertheless, when exposed to concentrations as high as 62.5 ppm, the migration rate of RCE cells significantly increases in comparison with the control group.157 Shetty et al.158 investigated the influence of CA on dexamethasone-suppressed and normal wound healing in a rat model. The 4 g kg−1 dose of CA was used in wound healing. The author observed a significant increase in wound-breaking strength in the extract-treated group.158 Ahmed et al. investigated the wound healing activity of the AC-rich fraction on a rabbit model.159 The authors demonstrated that there is a reduction in wound size, and epithelialization, in the AC-treated group.159 Sung et al. reported the wound-healing activity of CA-loaded hydrocolloid wound dressing (HCD) on Sprague–Dawley rats. After one month of study, the authors reported that there is a decrease in the excision wound size. The developed HCD exhibited outstanding swelling, drug release, and mechanical characteristics. In comparison with the commercial product, it increased the curative effect on excision, infection, and abrasion wounds in rats. Therefore, this hydrogel containing CA has the potential to be considered a viable option for treating different types of wound.160 Azis et al. studied the wound-healing activity of the methanolic fraction of AC in New-Zealand white albino rabbits. The AC was utilized in the concentration of 119.89 μg mL−1,161 which accomplished wound healing.161 The authors used this concentration of asiaticoside because prior studies conducted by Lee et al. and colleagues151 showed that, at this concentration, the number of treated fibroblast cells started increasing consistently. AC had no effect on the keratinocyte development rate, according to the same study by Lee et al.151 Because of this, the present investigation just used asiaticoside in its human dermal fibroblast (HDF) scratch assay. Ahmed et al.162 developed asiaticoside-loaded cross-linked polyvinyl alcohol/polyethylene glycol (PVA/PEG) hydrogel film. The prepared formulation is able to release 90% of the drug within 12 hours. The cytotoxicity investigations show that the developed formulation is compatible with cells and does not exhibit toxicity. Additionally, the microbiological limit tests revealed the absence of any microbial growth in any of the samples. The PVA/PEG hydrogel, developed by the freeze-thaw process, exhibited excellent fluid absorption capacity, elasticity, and safety. Consequently, it has significant promise as a material for wound dressing. Nevertheless, it is advisable to do further study on the formulation's in vivo effectiveness and long-term storage stability.162 A study by Chatterjee and co-workers was conducted to examine the wound healing effects of AC on Wistar albino rats (either sex). The authors revealed that there was a reduction in the wound area when the asiaticoside-rich cream was applied to the rats.163 In another study,164 the authors investigated the wound healing activity of asiaticoside. Topical administrations of asiaticoside (0.2%) solution to guinea pig punch wounds resulted in an increase in tensile strength, rise in hydroxyproline, higher collagen content, and improved epithelialization. In streptozotocin-diabetic rats, where healing is tardy, a 0.4% solution of asiaticoside, applied topically to punch wound epithelization, enhanced the collagen content, hydroxyproline content and tensile strength.164 A study by Liu et al. investigated the wound-healing potential of AC in diabetic rats/patients. The authors revealed that there was an increase in the expression of miRNA-21-5p among individuals with diabetic wounds (DW) and defined its function in signalling pathways associated with the process of chronic ulcer wound healing. The proliferation of cells was notably enhanced by LV-miRNA-21-5p overexpression, whereas the application of AC-Medium dose (AC-M) and AC-Low dose (AC-L) in combination with nitroprusside (SNP) augmented migration and proliferation. Additional examination unveiled potential targets of miRNA-21-5p, including TGF-β1, SMAD7, and TIMP3. The confirmation of their interaction with miRNA-21-5p was achieved via dual luciferase assays. This study revealed that the anti-DW drugs increased the expression of TGF-1 and SMAD7 while inhibiting the expression of TIMP3 in a high-glucose environment.165 Paocharoen et al. investigated the different extracts of AC for the wound healing activity. In this study, male Sprague-Dawley rats, weighing between 250–300 g, were randomly allocated into incision and burn wound groups. Each group was further divided into seven subgroups for treatment: (1) untreated; (2) normal saline; (3) Tween 20®- (vehicle control); (4) hexane extract-; (5) ethyl acetate extract-; (6) methanol extract-; and (7) aqueous extract-treated groups. Topical application of the respective test substances was conducted once daily. For the incision wound group, the tensile strength of the wound was assessed on the seventh day post-wounding. In the burn wound group, the overall appearance and progress of wound healing were evaluated on days 3, 7, 10, and 14 following the burn injury, prior to histopathological examination. The various extracts derived from CA demonstrated positive effects in promoting the wound healing process for both incision and burn wounds. Notably, the ethyl acetate extract, containing asiatic acid, exhibited the highest efficacy among the components studied, suggesting that asiatic acid plays a crucial role in facilitating wound healing.166 In one randomized controlled study involving 200 diabetic patients, the application of CA extract was found to accelerate the wound healing process. The participants were administered two capsules of CA extract, each containing 50 mg of AC, three times a day. The outcomes revealed improved wound contraction compared with the placebo group. Additionally, the CA extract demonstrated the ability to inhibit the formation of scar tissue. This suggests that the use of CA extract may be a beneficial intervention in promoting efficient wound healing and minimizing scar formation in diabetic patients.167
6. Mechanism of asiaticoside
6.1. Wound healing activity
AC affects many metabolic processes that are critical to human tissue, which ultimately results in collective tissue healing. During the process of healing, AC induces exposed tissue to produce and secrete antioxidants, which is important element for wound healing.156 CA may aid wound healing due to enhanced angiogenesis. This might be the result of its action on collagen I, fibroblast growth factor (FGF), and vascular endothelial growth factor (VEGF) synthesis.168,169 FGF increases the proliferation of endothelial cells in early angiogenesis. VEGF similarly promotes the development of new capillaries by regulating cell proliferation, differentiation, and migration.170 In a tilt wound model, AC isolated from CA improves hydroxyproline and collagen levels, as well as tensile strength and the rate of epithelialization. Additionally, AC plays a role in the process of wound healing by contributing to the proliferation of fibroblasts and the synthesis of extracellular matrix (ECM).171 Lu et al.172 reported that cell cycle progression, collagen production, and cell proliferation were seen in human dermal fibroblast cells in response to AC.172 According to Lu et al., asiaticoside can boost extracellular matrix (ECM) formation and fibroblast proliferation, both of which are known to be crucial for wound healing.173,174 The diagrammatic representation of the mechanism of AC is depicted in Fig. 4.
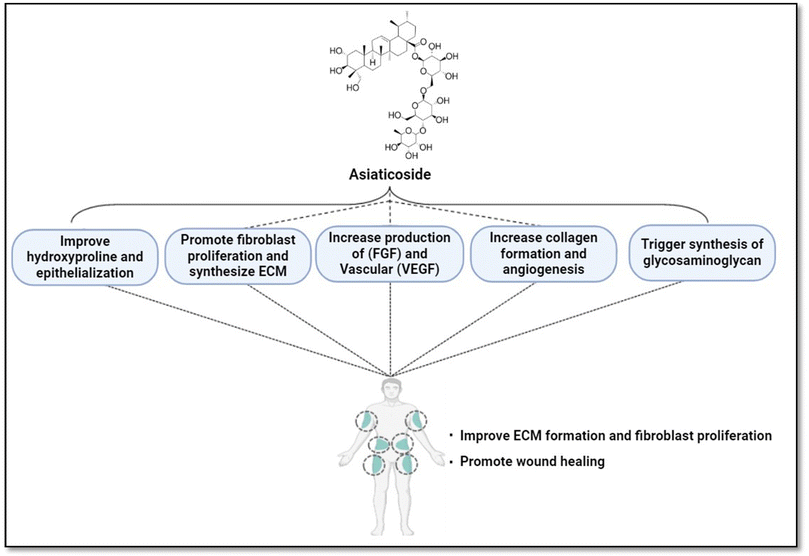 |
| Fig. 4 Mechanism of action of asiaticoside. | |
6.2 Anti-inflammatory, antipyretic, and antioxidant activity and neuroprotective activity
Glutamate is crucial in the process of transmitting signals between neurons, as well as synaptic plasticity. Glutamate attaches to ionotropic receptors such as N-methyl-D-aspartate (NMDA), kainate, and α-amino-3-hydroxy-5-methyl-4-isoxazolepropionic acid (AMPA) receptors.175 During pathological situations such as stroke, traumatic injury, and inflammation, a significant accumulation of cytoplasmic calcium influx via NMDA receptors leads to excitotoxicity and neuronal destruction. The research found that AC inhibits the increase in Ca2+ levels caused by NMDA, indicating that AC partially contributes to neuroprotection by inhibiting the entry of calcium via NMDA receptors.176,177 In one study, the authors found that administering AC can change depressive-like behaviour, raise monoamine neurotransmitter levels, lower inflammation in the hippocampus, and improve pNF-κBp65 and NLRP3 inflammasome levels in the chronic unpredictable mild stress (CMS) mouse model. It was additionally believed that AC might have an antidepressant-like effect by changing the cAMP/PKA/NF-κB/NLRP3/CREB/BDNF signalling system.178
Some studies showed that inflammatory cytokines play a crucial role in the development of depression in both humans and animals. It has also been shown that suppressing inflammatory processes could help people with depression.179–181 In particular, inflammation factors have been shown to have an impact on the breakdown pathways of monoamine neurotransmitter precursors. This may cause the levels of these neurotransmitters to rise.182 Another study found that AC had a strong anti-inflammatory effect in animal models of brain damage, spinal cord injury, and ischemia-reperfusion injury.183–185 A study demonstrated that CMS raised IL-1β, IL-6, and TNF-α levels in the hippocampus, which were lowered through the administration of asiaticoside. This suggests that AC has an antidepressant effect which may be due to its anti-inflammatory potential.178
7. Novel drug delivery systems
Novel drug delivery systems (NDDS) seem to be a better option than developing a new drug as they are associated with many benefits like less expenditure, being less time-consuming, and more profit for small companies who cannot afford huge costs to develop new drugs.39,186,187 NDDS are used to improve the activity of the drug and to reduce the side effects as shown in Table 3.
Table 3 Various drug delivery carriers studied as a part of NDDS for topical drug delivery
Carrier system |
Composition |
Size |
Special comments |
Ref. |
Micellar carrier |
Polymeric micelles |
Polystyrene, poly(ethylene glycol)–poly(ε-caprolactone) and poloxamers |
30–100 nm |
Micellar carriers are essential for improving the delivery of drugs to the skin, as they employ different mechanisms to enhance drug solubility, skin penetration, and overall effectiveness. When it comes to topical drug delivery, surfactants or amphiphilic polymers are frequently utilised to form these micelles. The main process involves enclosing hydrophobic drug molecules within the core of the micelles, which enhances their solubility in the aqueous vehicle of the topical formulation. This solubilization helps to keep the drug in a stable and evenly distributed state, preventing any unwanted precipitation or clustering. In addition, micellar carriers have the ability to interact with the skin's lipids, which helps the loaded drug penetrate through the stratum corneum, the outermost layer of the skin. Micelles have the unique ability to interact with both the water-loving and oil-loving parts of the skin, facilitating the effective delivery of drugs through the skin |
188 and 189 |
Mixed micelles |
Ionic surfactants, polymers and co-polymers |
10–100 nm |
190 and 191 |
Phospholipid-based micelles |
Phosphatidylcholine, sugars, glycolipids, and triglycerides |
5–20 nm |
192
|
Particulate carriers |
Solid lipid nanoparticles |
Cholesterol, triglycerides, fatty acids and waxes |
50–1000 nm |
Particulate carriers, including nanoparticles, microparticles, and nanocarriers, are used in the topical administration of drugs to improve the effectiveness and regulated release of the drugs. These carriers enable the transportation of pharmaceutical substances via several processes. Nanoparticles possess a diminutive size that facilitates their interaction with the skin at a tiny scale, hence facilitating effective permeation through the stratum corneum, which is the outermost layer of the skin. Moreover, the particulate carriers possess a favourable ratio of surface area to volume, which effectively increases the interaction between the carrier and the skin. This interaction promotes attachment and facilitates the release of drugs |
193 and 194 |
Nanostructured lipid carriers |
Lipids, surfactants and co-surfactants |
10–1000 nm |
195 and 196 |
Polymeric nanoparticles |
Polysaccharides and proteins |
1–1000 nm |
197 and 198 |
Emulsified carriers |
Microemulsion |
Water, oil, a surfactant, and a cosurfactant |
10–300 nm |
Emulsions are colloidal dispersions of two immiscible liquids, usually oil and water, stabilised by surfactants. In the context of topical drug administration, the emulsified carrier acts as a vehicle for incorporating lipophilic and hydrophilic drugs, enabling a broader spectrum of therapeutic agents to be developed. The emulsion structure ensures that the drugs are distributed evenly and precisely, improving skin contact and boosting drug absorption. Furthermore, the emulsified carrier may function as a reservoir mechanism, slowly releasing the drug into the skin over time. The emulsion's composition and surfactant characteristics also influence skin penetration by modifying the stratum corneum's barrier function. Furthermore, emulsified carriers may increase the stability of certain drugs, preventing deterioration and preserving effectiveness during storage. Overall, emulsified carriers provide a diverse platform for topical drug administration by resolving solubility issues, optimising drug release kinetics, and increasing the overall performance of pharmaceutical formulations applied to the skin |
199 and 200 |
Nano-emulsion |
Oil, water, surfactant |
10–1000 nm |
201 and 202 |
Lipid emulsion |
Phospholipids, emulsifiers, oil and water |
0.5–5 μm |
203 and 204 |
Vesicular carriers |
Liposomes |
Phospholipid, cholesterol and API |
0.025–2.5 μm |
The primary functions of vesicular carriers, including liposomes, ethosomes, and niosomes, are to improve the stability of the encapsulated compounds, facilitate sustained release, and enhance drug permeation. An essential mechanism involves the potential of vesicles to alter the structure of the stratum corneum, which is the outermost layer of epidermis. Vesicles are capable of interacting with the lipid bilayers of the stratum corneum, thereby transiently creating pathways for drug penetration and temporarily disrupting its integrity. Furthermore, it is possible to engineer vesicles with a diminutive size that can traverse the intercellular spaces of the stratum corneum, thereby facilitating improved drug transportation to the dermal layer |
205 and 206 |
Niosomes |
Non-ionic surfactants, hydration medium and cholesterol |
0.025–0.1 μm |
207
|
Ethosomes |
Phospholipid, alcohol, polyglycerol and water |
100–1000 nm |
208
|
Transferosomes |
Edge activators such as Span 80 and phospholipids |
50–500 nm |
209 and 210 |
Phytosomes |
Phospholipids, such as phosphatidylcholine, produce a lipid-compatible molecular complex with the herbal ingredients |
100–1000 nm |
211
|
The Nobel Prize winner Sir Paul Ehlrich, who imagined drug molecules as “magic bullets” that could only reach the intended place to demonstrate their action, is credited with the idea of NDDS (in 1905). The idea of “magic bullets” changed into “magic guns”, or NDDS.212Fig. 5 illustrates how these carriers interact with skin elements to efficiently distribute the loaded medicine to the different skin layers.145,213 These carriers can carry the drug to different skin layers through one or many mechanisms, as shown in Fig. 5, depending on their compositional characteristics.214,215 (1) Biocompatible and biosimilar excipient carriers, such as liposomes and microemulsions, can integrate with the lipids of biological membranes. (2) Smaller carriers, such as lipid nanoparticles (SLNs/NLCs), micro and nanoemulsions, and flexible carriers, such as ethosomes and flexible membrane vesicles (FMVs), can transfer drugs via the intercellular gaps of skin cells. (3) The osmoregulated delivery of elastic vesicles such as FMVs and ethosomes can be triggered by the moisture cloud beneath the SC. (4) Small lipid-based carriers, such as SLNs/NLCs, also infiltrate the skin via a transcellular channel, namely keratinocytes. (5) A significant number of colloidal carriers are adsorbed to the SC and release the medication via diffusion. (6) The transappendageal pathway, which involves passing via hair follicles and sweat and sebaceous glands, is currently recognised as one of the key routes of drug delivery by NDDS.216,217 The NDDS are utilized as carriers to deliver the drugs at their target site in order to increase their efficacy, stability and safety. For example, niosomes are used as a delivery carrier for many drugs like gliclazide,205 ketoconazole,218 transdermal formulations,219 timolol maleate,220 folic acid221etc. Nowadays liposomes are utilized to deliver many drugs such as paclitaxel,222 cyclosporine,223 cefepime,224 cabazitaxel,225 glycyrrhetinic acid,226 curcumin,227 ibuprofen,228 tedizolid phosphate229etc. in order to improve their efficacy and stability. Similarly, ethosomes may impair drug molecules with various physicochemical characteristics, including hydrophilic,230 lipophilic and amphiphilic.231 Many drugs such as aceclofenac,232 diclofenac diethylamine,233 mycophenolic acid,234etc. have been delivered by ethosomes. In order to increase the therapeutic efficacy of drugs phytosomes are utilised to supply numerous medicines including mitomycin C,235Boswellia serrata extract,236 curcumin,237 quercetin238etc. For instance, several drug carriers, such as Lipusu (liposomal paclitaxel), Ambisome (liposomal amphotericin B), Psorisome (liposomal dithranol), and Fungisome (liposomal amphotericin B), are successful examples of NDDS systems.239–242
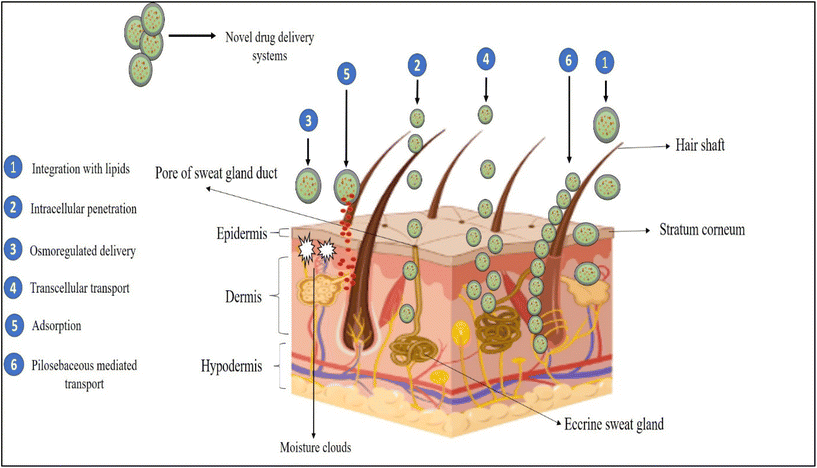 |
| Fig. 5 The drug-loaded NDDS penetration across the skin through different mechanisms. | |
8. Advantages of nanocarriers
Nanocarriers offer a breakthrough paradigm in topical drug delivery, offering several benefits that boost pharmaceutical innovation to new heights.243 These tiny carriers, often on the nanoscale scale, have revolutionary potential for overcoming the limits of traditional formulations.244 One of their foremost advantages lies in their ability to enhance the bioavailability of drugs by facilitating efficient penetration through the intricate layers of the skin.245 The nanoscale dimensions confer an increased surface area and an augmented drug payload, ensuring a profound and targeted therapeutic impact.246 Furthermore, nanocarriers have an excellent ability to encapsulate both hydrophobic and hydrophilic drug moieties, increasing the range of pharmacological agents suitable for topical administration.247 This flexibility extends beyond traditional limitations, providing a complete answer to the issues faced by various drug physicochemical features.248 Nanocarriers’ regulated and sustained release kinetics provides unparalleled precision to formulations, carefully influencing the temporal components of drug delivery for optimal therapeutic effects.249 Additionally, the inherent potential of nanocarriers to circumvent biological barriers and reach the targeted skin layers with clinical accuracy is illustrative of their ability to overcome obstacles that are associated with traditional drug delivery.250
9. Topical delivery of asiaticoside-employing NDDS
NDDS are currently widely utilized in order to deliver the drug topically. These systems enhance drug stability, bioavailability and efficacy.251,252 The carriers that are used in the topical delivery of AC are shown in Fig. 6.
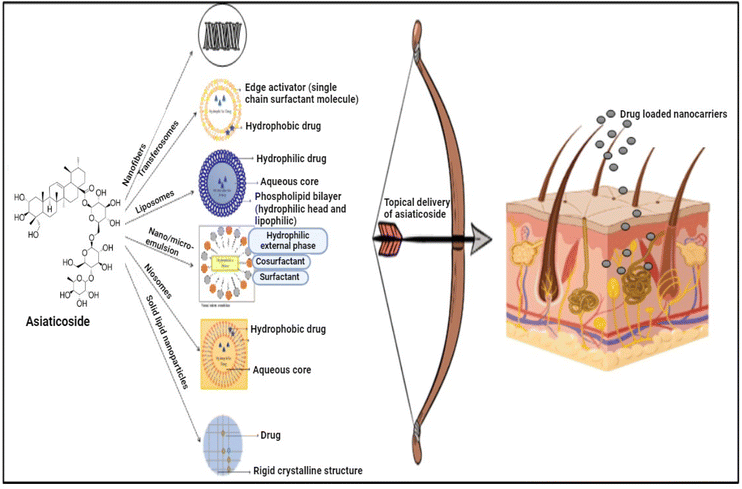 |
| Fig. 6 Topical delivery of asiaticoside employing NDDS. | |
9.1 Liposomes
A liposome combines the Greek terms lipo, which means fat, and somes, which means body. Alec D. Bangham created the first liposomes in England in 1961. Liposomes are tiny, spherical synthetic vesicles composed of phospholipids (non-poisonous) and cholesterol.253,254 Due to their small sizes and hydrophilic and hydrophobic characteristics, liposomes can be used as medication delivery vehicles. Their characteristics are dependent on the lipid content, surface charge, size, and production technique.255 Liposomes are spherical-shaped micro-vesicles epitomizing the first generation of nanocarriers that have been fortunately expanding from the laboratory scale up to clinical applications.256 These liposomal systems have been an entrenched drug delivery platform with a major influence on patient well-being.257,258 Liposomes are composed of a bi-layer of natural or synthetic non-toxic phospholipids which instinctively form closed structures at the time of hydration. These kinds of vesicle have one or more bi-layer membranes known as lamellae.259–262 However, the different structure of liposomes enables drug encapsulation with diverse lipophilicities.263,264 A study reported by Paolino et al. on ultra-deformable liposomes provided the significantly enhanced in vitro skin penetration of AC showing a 10-fold increase with respect to the free drug solution and promoting in vivo collagen production. Liposome-based AC delivery through the topical route is a promising delivery system for effective wound healing.265 Feng et al. prepared injectable hydrogel with AC-loaded liposomes for burn wound healing. In vitro studies have demonstrated that the hydrogels possess remarkable antibacterial efficacy against Escherichia coli and Staphylococcus aureus. The results of the in vivo study conducted on Sprague–Dawley rats using a burn-wound infection model indicate that the hydrogel demonstrated significant efficacy in promoting wound healing and exhibited superior wound healing properties.266
9.2 Microspheres
Microspheres are “monolithic spheres or therapeutic agents, distributed throughout the matrix either as a molecular dispersion of particles”267 or as a structure composed of one or more miscible polymers in a continuous phase in which molecular or macroscopic droplet particles are distributed. They are tiny spherical particles with sizes in the micrometer range (usually 1–1000 micrometres). Starches, gums, proteins, fats, and waxes that are biodegradable man-made polymers and modified natural materials are used to prepare microspheres.268 In one study, Sharma et al.269 used these biodegradable polymers in order to prepare microspheres. Albumin and gelatin are natural polymers, while polyglycolic acid and polylactic acid are manufactured polymers. Albumin controls the release of drugs from microspheres270 whereas polyglycolic acid and polylactic acid improve the stability of encapsulated drugs.271 The solvents which are utilized to dissolve the polymeric materials are chosen based on the solubilities and stabilities of polymer and drug, as well as process safety and economic factors.272,273 Zhang et al. studied AC-loaded microspheres for wound healing. They investigated the release kinetics and cellular uptake profiles of AC-microspheres, exploring their therapeutic impact on wound healing and skin appendage regeneration through both in vitro and in vivo assessments. The results revealed that the optimized AC-microspheres exhibited a spherical spongy structure with cylindrical holes. Efficient loading and sustained release of AC were achieved within the microspheres. Cellular uptake of AC from the microspheres was notably enhanced, being 9.1 times higher than that of the free solution. In vitro experiments demonstrated that AC-microspheres significantly promoted the proliferation and migration of keratinocytes, along with accelerated wound healing. Moreover, these microspheres exhibited marked effects on re-epithelization, collagen synthesis, and pro-angiogenesis during rat full-skin wound healing in vivo. The porous microsphere emerged as an innovative carrier for the sustained delivery of poorly soluble AC, leading to improved absorption and therapeutic outcomes. AC-microspheres, as a promising topical preparation, demonstrated excellent regenerative effects in wound therapy.274
9.3 Nano-emulsions
The nano-emulsion is either the oil-in-water (O/W) or water-in-oil (W/O) type, which primarily depends on the method of preparation. The water acts as a continuous phase whereas oil is the dispersed phase in the O/W type of emulsion,202,275 whereas the inversed condition results in a W/O emulsion. Nano-emulsions (NEs) have tiny droplet sizes which enable a uniform diffusion of active ingredients throughout the skin.276 The nano-emulsion has a low surface tension and wide surface area, and due to this only 3 to 10% of surfactants are required during nano-emulsion preparation.277,278 Huimin et al. developed AC-loaded NEs and nano-emulsion-based gel (NBGs) for the topical delivery of AC. The ex vivo permeation study revealed that the permeation rate of AC-NEs is very high. According to Huimin et al., the ex vivo skin permeation of AC from prepared NEs was 13.65 times more than that of ordinary gel when determined by using Franz diffusion. The prepared AC-NBGs also showed good penetrability when compared with the enhancer group. The pharmacokinetic study showed that the prepared AC-NEs when applied to the mouse model achieved the peak level of the drug (Cmax/μg g−1 – 656.28 ± 19.42) in the skin very quickly (tmax/h-6), retained the drug mass concentration in the subcutaneous tissue and maintained the plasma drug concentration for a long time with high bioavailability. Additionally, the mechanism of AC-NEs and AC-NBGs was studied by HE (hematoxylin and eosin) staining and CLSM (confocal laser scanning microscopy).279 In a similar study, Muthia and team prepared AC-NE lotion. The prepared NEs exhibited a mean particle size of 19.88 ± 2.3 nm, while the size in the lotion formulation was 198.4 ± 11.52 nm. The polydispersity index indicated good uniformity (0.329 ± 0.065), and the zeta potential was −30.9 mV, suggesting stability. Stability tests over 8 weeks at varying temperatures (4 ± 2 °C, 28 ± 2 °C, 40 ± 2 °C) confirmed the resilience of the NE lotion, with no notable changes in physical properties or pH values observed. The cumulative amount of AC penetration was significantly higher for the NE lotion (1558.65 ± 66.93 μg cm−2) compared with the lotion alone (1260.364 ± 71.42 μg cm−2). The flux of the NE lotions (2.1255 ± 0.31 μg cm−2 h−1) surpassed that of the lotion (1.4506 ± 0.49 μg cm−2 h−1). These findings underscore the enhanced penetration and stability of the NE lotion, suggesting its potential as an effective carrier for AC in topical applications.280
9.4 Nanoparticles
Nanoparticles are novel drug carriers with diameters of 1 to 100 nm. The nanoparticles are the carrier systems in which the drug dissolves, encapsulates or binds to the polymeric matrix.281 The nano-capsule or nanosphere formation is based on the method of preparation. Nano-spheres are matrix systems in which the drug is physically and evenly distributed, while nano-capsules are systems in which the drug is contained in a cavity surrounded by a unique polymer membrane.282–285 A study conducted by Rocha et al. developed AC-loaded nanoparticles for topical delivery with both dried (D) and glycolic (G) extracts encapsulated in nanostructured lipid carriers (NLC). They formulated two different forms, NLC-D and NLC-G, respectively, for effective skin delivery. The investigation involved in vitro release and skin permeation studies. Additionally, electron paramagnetic resonance (EPR) analysis was conducted to elucidate the lipid dynamics within the NLC matrices and the impact on stratum corneum (SC) membrane fluidity. The release of AC from NLC-D was approximately 23.7%, while NLC-G demonstrated a notable 2-fold increase in AC release over the same period. EPR data revealed that NLC effectively enhanced SC membrane fluidity, with NLC-G exhibiting a more pronounced effect. Furthermore, NLC-G facilitated the penetration of AC into deeper skin layers, with quantifiable amounts detected in the epidermis/dermis (9.14 ± 0.54 μg cm−2) within 24 h. In contrast, similar AC permeation from NLC-D was achieved only after 48 h (10.42 ± 1.41 μg cm−2). The accelerated release of AC from NLC-G contributed to enhanced skin permeation, and the augmented SC fluidity induced by NLC-G appeared to further increase AC penetration into deeper skin layers. As a result, nanoparticles appear to have a potential for topical delivery.286 In another research study Kwon et al. investigated the wound healing and skin protective property of CA (includes AC, madecassoside, asiatic acid, and madecassic acid) loaded nanoparticles. An approx. 67% drug was encapsulated into the prepared nanoparticles and showed negligible cytotoxicity in human skin fibroblast cells. The prepared nanoparticles have the ability to decrease the expression of matrix metalloproteinase (MMP-1). The developed nanoparticles when tested on mouse skin showed high flux and were retained in the skin with a large concentration of drug.287 Liu et al. developed bioactive composite hydrogels by incorporating decellularized extracellular matrix (ECM), GelMA, and AC-loaded polydopamine nanoparticles (AC@PDA). These hydrogels were designed as wound dressings with the potential to enhance the healing process. In vitro cytotoxicity assessments conducted on human skin and fibroblasts demonstrated favourable biocompatibility for all hydrogels. To evaluate the in vivo wound healing efficacy, tests were performed using a full-thickness excisional wound model in mice. Notably, the AC@PDA/ECM-G hydrogel exhibited fast wound closure without scarring, and showcased the highest formation of hair follicles. The superior performance of the AC@PDA/ECM-G hydrogel in promoting wound healing indicates its potential utility as a promising wound dressing.288 Similarly, Narisepalli et al. developed AC-loaded polymeric nanoparticles for diabetic wound healing. The presence of AC in the formulation was found to induce a notable proliferation and migration of fibroblasts in in vitro cell culture studies. Furthermore, the administration of AC-loaded polymeric nanoparticles to diabetic rats for the treatment of the wounds of diabetic rats resulted in enhanced wound healing efficacy, as evidenced by increased collagen biosynthesis, upregulated levels of COL-1 protein, and enhanced expression of α-SMA in comparison with the control groups.289
9.5 Niosomes
Niosomes are vesicular nanocarriers that self-assemble after being hydrated with synthetic surfactants and sufficient concentrations of cholesterol or other amphiphilic compounds. Handjani-Vila et al. describe for the first time this kind of vesicle.290 Niosomes are vesicular structures similar to liposomes and have the capability to transport both lipophilic and hydrophilic medicines. The reason for making niosomes is that surfactants are thought to have greater chemical stability than phospholipids, which are utilised to make liposomes. Phospholipids are quickly hydrolysed due to the presence of an ester bond.291 Because of the unreliable reproducibility caused by the use of lecithins in liposomes, scientists are looking for vesicles made from alternative materials, such as non-ionic surfactants. Niosomes are a potential drug delivery vehicle, and because they are less toxic, they are non-ionic and increase the therapeutic index of drugs by limiting their action to the target cells. They are small laminary structures that are also called non-ionic surfactant vesicles, formed in combination with cholesterol and hydrated by aquatic media.292 Wichayapreechar et al.293 created CA extract-loaded niosomes (CAE-Nio) and niosomes with hyaluronic acid surface modifications (CAE-Nio-HA). The niosomes had high drug loading capacity (% DL) and encapsulation efficiency (% EE), which were respectively 3–7% and 71–77%. When HA was added to niosomes, % DL reduced, zeta-potential elevated, particle size increased in a dose-dependent manner, and % EE was unaltered. The sustained-release profile of CAE-loaded niosomes was controlled by a diffusion-based mechanism. In comparison with CAE-Nio and CAE solution, asiaticoside, a moderately polar molecule from CAE-Nio-HA, was better able to enter the stratum corneum and dermis. For periods longer than four months, CAE-Nio-HA formulations demonstrated better stability at low temperatures (4 °C and 25 °C). The created Nio-HA is a potential AC delivery method that can increase dermal absorption, permeability, and accumulation in healthy epidermis and dermis layers.293 Kim et al.294 investigated the wound-healing activity of AC-loaded niosomes. The study revealed that prepared niosomes showed better penetration of the drug and the wound healing activity was 3.2 times more than the control group when tested on a full-thickness rat model.294
9.6 Nanofibers
Nanofibers have outstanding qualities that promote wound healing.295 The nanofibers’ high surface area to volume ratio, coupled with their microporous structure, promotes cell adhesion, proliferation, migration, and differentiation, which are all very desirable qualities for tissue engineering applications.296–298 At the same time, the high permeability and absorption rate may absorb the exudate that has accumulated on the wound surface and keep the environment moist so that it is conducive to the healing process. Additionally, the large surface area makes it easier to load and transport bioactive components like medicines and growth hormones.299,300 Nanofibers have been proposed as topical drug delivery systems for natural substances.301,302 Due to increased water retention, weight loss, and a large surface area of the nanofiber mat, the AC was added to ultrafine cellulose acetate electrospun nanofibers, which demonstrated enhanced skin permeability of the drug.302 Similarly, compared with as-cast films, an ultra-fine cellulose acetate nanofiber mat containing asiaticoside or curcumin demonstrated improved drug release.303 Zhu et al. prepared AC-loaded coaxial electrospun nanofibers for the treatment of profound partial-thickness burn injuries. Vascular endothelial growth factor, proliferating cell nuclear antigen and tumour necrosis factor were upregulated, and interleukin 6 and tumour necrosis factor-alpha were downregulated, in order to improve wound healing in vivo.304 Anand et al. prepared AC-loaded nanofibers. In this study, the authors develop and assess a multifunctional nanofibrous scaffold comprising polyvinyl alcohol (PVA), sodium alginate (SA), and silk fibroin (SF), loaded with AC for application in diabetic rats. Scanning electron microscopy (SEM) analysis revealed fibre diameters ranging from 100 to 200 nm and tensile strengths between 12.41 and 16.80 MPa. The crosslinked nanofibers exhibited a sustained release of AC over an extended period. Evaluation on HaCaT cells through MTT and scratch assays confirmed minimal cytotoxicity and significant cell migration, respectively. Antimicrobial testing demonstrated the scaffold's excellent efficacy against P. aeruginosa and S. aureus bacteria. In vivo studies further showcased enhanced wound healing in diabetic rats, and histopathological examinations highlighted the scaffold's ability to restore a normal skin structure. Overall, these findings underscore the potential of the PVA-SA-SF-based nanofibrous scaffold loaded with AC as a multifunctional and effective approach for wound healing in diabetic conditions.305 Lie et al. prepared AC-loaded nanofibers for wound healing. A straightforward blending–centrifugation transport method was utilized to efficiently load hydrophobic AC. The polymer and active ingredients are dissolved in an appropriate solvent throughout the preparation procedure. The drug-soluble solvent was added to the polymer-dissolving solvent in order to dissolve the drug and polymer simultaneously. AC accelerated skin regeneration and reduced scar formation by modulating inflammatory reactions and angiogenesis. The in vivo experiments revealed that these AC-laden silk hydrogels have intriguing applications in scarless tissue regeneration.306
Due to its extensive therapeutic characteristics, CA has substantial commercial potential. More in-depth study of this plant is clearly required to fully realize its medicinal potential. AC is sold under the brand name of TECA™. TECA™ is CA extract.307 It contains AC, asiatic acid, and madecassic acid. It improves wound healing, collagen formation, microcirculation, and stretch marks. The therapeutic efficacy of AC is shown in Table 4.
Table 4 Nanocarrier mediated delivery of asiaticoside
S. no. |
Nanocarrier formulation |
Size |
In vitro/in vivo model |
Application |
Special outcomes |
Ref. |
1 |
Nanofiber |
— |
Sprague–Dawley (SD) rats |
Burn wound healing |
Nanofiber dressing also showed excellent wound-healing properties in the SD rat burn model |
308
|
2 |
Nanoparticle |
100.2 nm size |
Sprague–Dawley rats |
Neuroprotective activity |
The prepared nanoparticles have the potential to function as a platform technology for neurodegenerative disease treatments |
309
|
3 |
Lipid nanocarrier (liposome and niosomes) |
Liposomes (42.33 ± 0.47 nm) and niosomes (55.73 ± 2.52 nm) |
In vitro study |
Antioxidant activity |
Liposome and noisome formulations show promise as transdermal delivery systems for CA extract to enhance antioxidant activity |
310
|
4 |
Hydrogels |
|
In vitro study |
Wound healing potential and anti-bacterial activity |
The chitosan-based hydrogel preparation was optimized to provide the essential rheological characteristics for the release of the bioactive from the chitosan delivery system. Additionally, it showed adequate antibacterial activity |
311
|
5 |
Polymeric colloidal |
210 nm |
In vitro
|
Dermatological and cosmetic applications |
The author demonstrated that polymeric colloidal nanocarriers CA are proven to be safe, noncytotoxic, and do not cause cell transformation |
312
|
6 |
Microneedle |
200 μm × 200 μm × 500 μm (W × L × H) |
Diabetic mice |
Diabetic foot ulcer |
The author concluded that the efficacy of microneedles made of γ-PGA hydrogel combined with AC in promoting the healing process of diabetic foot ulcers, thus providing support for the application of these microneedles in the treatment of chronic wounds |
313
|
7 |
Transferosomes |
27.15 ± 0.95 to 63.54 ± 2.51 nm |
In vitro study |
Hypertrophic scars |
These pilot study outcomes support the effectiveness of the asiatic acid-entrapped transferosomal gel |
314
|
8 |
Micro- and nanoparticles |
Nanoparticles (200 ± 5.09 nm) and microparticle (8.79 ± 3.51 μm) |
In vitro study |
Anti-inflammatory |
The epigallo-catechin-3-gallate-loaded microscale particles are biocompatible and have a long-lasting anti-inflammatory effect |
315
|
9 |
Nanostructured lipid carriers |
Asiatic acid nanostructured lipid carriers (AA-NLC) (139.30 ± 1.68 nm) and PEGylated asiatic acid nanostructured lipid carriers (P-AA-NLC) (160.50 ± 4.16 nm) |
In vitro and in vivo study |
Anti-fibrosis effects |
P-AA-NLC may improve asiatic acid's anti-liver fibrosis effects in SD rats and increase AA's gastrointestinal absorption |
316
|
10 |
Polyurethane foam |
228–262 μm |
New Zealand white albino rabbit |
Partial thickness wound |
The prepared polyurethane foam dressing promotes wound healing in rabbits |
317
|
11 |
Alginate chitosan nanoparticles |
486.2 nm |
Mouse |
Anti-excitotoxicity and neuroprotective action |
Biological assessment studies demonstrated that ACNPs exhibit non-toxic effects on mouse neural stem cells (mNSCs). Moreover, these nanoparticles exhibited improved permeability across the blood–brain barrier, leading to a reduction in seizure activity |
318
|
10. Other potential benefits of asiaticoside
AC has numerous therapeutic effects. Apart, from wound healing AC showed antidepressant,319 anti-diabetic,320 anti-inflammatory and anti-pyretic activity321 The versatile therapeutic benefits of AC are discussed below:
10.1 Anti-depressant activity
AC showed excellent anti-depressant-like activity. Liang et al. reported the anti-depressant activity of AC. AC was administered to mice in order to observe whether it had any antidepressant effects using three different tests: a splash test in a chronic mild stress (CMS) model, a tail suspension test (TST), and a forced swimming test (FST). Stressed mice exhibited considerably more grooming activity when AC was administered (10 mg kg−1). AC (10, 20 mg kg−1, P.O) substantially reduced immobility time in the tail suspension test. These findings point to the possibility that AC acts similarly to anti-depressants.322 Luo et al. determined whether AC induces an antidepressant-like effect by way of BDNF (brain-derived neurotrophic factor) signalling activation under chronic unpredictable moderate stress (CUMS). The findings demonstrated that the reduced sucrose preference and increased immobility time exhibited in CUMS mice may be corrected by administering asiaticoside to the animals for four weeks. Furthermore, authors discovered that in both non-stressed and CUMS animals, AC up-regulated BDNF, PSD-95, and synapsing-I expression only in the hippocampus. The antidepressant-like effects of AC, however, were entirely nullified by the addition of K252a, an inhibitor of the BDNF receptor tropomyosin-related kinase receptor B (TrkB). The results suggested that the AC may stimulate BDNF signalling in the hippocampus to produce its antidepressant-like effect.323 Verma et al. analyzed the oral administration of AC and its effects on AC distribution in the AC-borneol formula (FAB). AC was detected using HPLC.324 The effectiveness of FAB and AC as antidepressants was analysed. Behavioural depression paradigms and the chronic unpredictable stress model (CUS) were utilized on rats that had been given the drugs either acutely or chronically. Pathological alterations were revealed by H&E staining, and levels of 5-HT, norephedrine (NE), BDNF, and TNF- were measured in the hippocampus. The injection of FAB, but not AC alone, resulted in the detection of AC in brain tissues from the rats, suggesting that borneol (BOR) facilitated the dispersion of AC in the brain.325 Wang et al. explored the anti-depressant-like activity of AC. Authors observed that AC increased the monoamine neurotransmitter levels and counteracted the rise in inflammatory cytokines caused by CMS. Additionally, AC antidepressant and anti-inflammatory effects may be mediated via modulation of the cyclic adenosine monophosphate (cAMP)/protein kinase A (PKA) signalling pathway.326 These findings have the potential for advancing AC development as an innovative therapy for the management of depression. However, further research is needed to regulate the therapeutic efficacy of AC in individuals with depression or related diseases and to determine the mechanism by which it activates PKA signalling in depression.
10.2 Antidiabetic activity
AC also has antidiabetic activity. Fitrianda et al. reported the anti-diabetic action of AC in a mouse model. The saponin-rich-fraction, ethanolic extract of AC showed anti-diabetic activity in alloxan-induced diabetic mice. The AC inhibited glucose uptake by healthy cells in the pancreas.327 In one study, the authors investigated the anti-diabetic activity of AC and Andrographis paniculata (AP) in the rat model. AC and AP used in a 30
:
70 ratio, when tested on rat model, exhibit excellent anti-diabetic activity. The combination also showed synergistic HDL-increasing and cholesterol-lowering activity.328
10.3 Anti-inflammatory and anti-pyretic activity
Researchers studied how AC affected the activity and expression of nitric oxide synthase in gastric ulcers, finding that it decreased ulcer size in a dose-dependent fashion. Additionally, it suppressed the activity and protein expression of inducible nitric oxide synthase, indicating its anti-inflammatory effect.329 In a study where the authors compared the anti-inflammatory effects of AC-G and other pure phytoconstituents in lipopolysaccharide (LPS)-stimulated RAW 264.7 cells, it was shown that AC-G greatly suppressed the production of nitric oxide and tumour necrosis factor-alpha.330 In a dose-dependent way, AC was shown to boost fibronectin mRNA and protein expression in human periodontal ligament cells, while also increasing proliferation and protein synthesis. Matrix metalloproteinase-1 mRNA expression was suppressed by AC, although tissue inhibitor of metalloproteinase-1 mRNA expression was boosted.331 The anti-inflammatory and antipyretic effects of AC were studied, and the results showed that the compound reduced the fever and inflammatory response brought on by LPS in a dose-dependent manner.332 Analysis of the effects of AC on typical human skin cell activities associated with healing was performed. AC improved initial cell adhesion and migration in skin cells. Even more so, AC induced a proliferation boost in healthy human dermal fibroblasts in cell proliferation assays.333 In a recent study, AC was investigated for its potential as an anti-inflammatory and immunomodulatory agent during the in vivo implantation of electro-spun polylactic-co-glycolic acid (PLGA) fibrous scaffolds. These scaffolds are widely used in tissue engineering for various applications, including bone, cartilage, skin, and neural regeneration, as well as drug delivery systems. However, the accumulation of degradation products from the implanted scaffolds triggers a host inflammatory response mediated by innate immune cells, such as dendritic cells, mast cells, granulocytes, and macrophages, hindering the desired tissue regeneration. The study revealed that AC effectively suppressed the expression of M1 (inflammatory) macrophages and inhibited the production of pro-inflammatory cytokines. Simultaneously, it promoted the expression of M2 macrophages, known for releasing anti-inflammatory cytokines. This dual action of AC is crucial in modulating macrophage polarization, which is reversible and holds therapeutic value, particularly in conditions where an imbalance between M1 and M2 macrophages contributes to pathogenesis. By blocking the host inflammatory response, AC demonstrated its potential as a favourable anti-inflammatory drug, offering promising outcomes for the successful implantation of PLGA fibrous scaffolds and, consequently, facilitating desirable tissue regeneration results.334,335
10.4 Antibacterial activity
CA shows antibacterial activity against Bacillus subtilis, Staphylococcus aureus, Escherichia coli and Pseudomonas aeruginosa. CA methanolic extract exhibited an inhibitory zone against Vibrio alginolyticus, Vibrio vulnificus, and Streptococcus sp.336 Antibacterial activity against three Vibrio species (V. harveyi, V. alginolyticus, and V. parahaemolyticus) was found in a methanolic extract of CA reported by Sankar et al.337 but not in an acetone, chloroform, or hexane extract.
10.5 Neuroprotective effect
According to research by Ramanathan et al.,338 CA extract prevents the neurodegeneration caused by monosodium glutamate. CA water extract improved glutathione levels and antioxidant defences in brain areas in prepubescent mice exposed to oxidative stress caused by 3-nitropropionic acid.339,340 A study investigated the in vitro performance of asiatic acid (AA) liposomes and surface-modified liposomes of AA, specifically chitosan-coated AA liposomes (CAAL) and stealth AA liposomes (SAAL), as potential therapeutic interventions for Alzheimer's disease. Utilizing Design-Expert software, an optimized formula was derived, and liposomes were prepared through the thin-film hydration method. The formulations were then comprehensively evaluated for various parameters, including compatibility, liposomal vesicle size, drug entrapment, drug content, dispersibility index, and surface morphology using transmission electron microscopy and atomic force microscopy. The release profiles demonstrated that both AA liposome (AAL) and SAAL exhibited sustained-release characteristics, releasing 97.00 ± 0.56% and 86.42 ± 0.38% of the drug over 18 hours, respectively. Notably, chitosan-coated AA liposomes (CAAL) demonstrated a comparable sustained release, with 85.45 ± 0.43% of the drug released within 24 hours. Moreover, the ex vivo permeation study indicated that CAAL exhibited superior permeation compared with the other two forms of liposomes.341 A study conducted by Renju et al., prepared AC-loaded alginate chitosan nanoparticles (ACNPs) for the management of antiproliferative activity in C6 glioma cells. The synthesized ACNPs, characterized by their spherical shape, particle size (200 nm), thermal stability, and acceptable polydispersity index, demonstrated promising properties for drug delivery. The encapsulation of AC within the ACNPs exhibited high efficiency, with 90% encapsulation, and controlled drug release, with only 10% released within 24 hours. The application of ACNPs showed positive outcomes in terms of cell viability and morphology in mouse primary brain astrocyte cells (mBA) cells. However, contrasting results were observed in C6 glioma cells, where ACNP treatment led to dose-dependent cytotoxicity, late apoptosis, and necrosis. The results of this study indicate that ACNPs exhibit antiproliferative effects on C6 glioma cells by activating the intracellular reactive oxygen species (ROS) pathway, ultimately leading to apoptosis and necrosis. These findings shed light on the intricate mechanistic details involved in addressing brain disorders through the utilization of CA and its phytoconstituents.342
11. Future prospective
In the future, the combination of AC and nanotechnology is poised to revolutionize the field of wound care/343,344 The synergistic potential of AC, a compound derived from CA with known wound healing properties, when harnessed alongside cutting-edge nanotechnological approaches, presents a groundbreaking avenue for transforming traditional wound care paradigms.345 Further exploration into the optimization of nanocarriers and nano-formulations encapsulating AC could lead to enhanced targeted delivery, controlled release kinetics, and improved stability, increasing its therapeutic efficacy at wound sites.346 Advanced nanotechnological approaches, such as stimulus-responsive or smart delivery systems, may enable the on-demand release of AC, responding to specific cues present in the wound microenvironment for precise and timely therapeutic interventions.347 Moreover, the incorporation of innovative biomaterials and bioengineering techniques may facilitate the development of multifunctional scaffolds or dressings capable of simultaneously providing structural support, promoting tissue regeneration, and delivering AC in a controlled manner.348 Additionally, combining AC with other bioactive agents or growth factors within nanocarriers could potentially create synergistic therapeutic effects, accelerating wound closure, reducing inflammation, and minimizing scar formation.349 As research progresses, addressing challenges related to standardization, scalability, safety, and regulatory aspects will be pivotal in translating the AC-nanotechnology-driven approaches from bench to bedside. Collaborative efforts across multidisciplinary fields, including nanotechnology, pharmacology, biomaterials science, and clinical medicine, will be crucial in advancing these futuristic wound healing strategies and eventually offering more effective and personalized treatments for diverse wound types and patient populations.
12. Conclusion
The therapeutic potential of AC in wound healing is well-established, attributed to its ability to stimulate collagen biosynthesis. However, the inherent physical properties of AC, including its high molecular weight, poor water solubility, and low permeability, pose significant challenges to its effective topical administration. Nanotechnology emerges as a promising solution to overcome the hurdles associated with AC delivery. By encapsulating AC in nano-carriers, the drug's efficacy, stability, and safety can be significantly improved. These nano-carriers play a pivotal role in facilitating targeted delivery, ensuring efficient distribution of the loaded drug across various skin layers. The utilization of nanotechnology in dermatology represents a breakthrough in enhancing the therapeutic efficacy of AC, particularly in the context of wound healing. The integration of nanotechnology into the delivery of AC presents a transformative approach to unlocking its full therapeutic potential. As research and development in this field continue to advance, it is anticipated that innovative strategies will further enhance the topical delivery of AC, opening new avenues for its application in various medical conditions.
Abbreviations
AC | Asiaticoside |
ECM | Extracellular matrix |
FGF | Fibroblast growth factor |
VEGF | Vascular endothelial growth factor |
NDDS | Novel drug delivery systems |
SC | Stratum corneum |
MMP | Matrix metalloproteinase |
AP |
Andrographis paniculata
|
CA |
Centella asiatica
|
LPS | Lipopolysaccharide |
NBGs | Nano-emulsion-based gel |
Author contributions
Mohit Kumar: writing – original draft preparation, collecting information, methodology. Devesh Kumar: collecting information, methodology. Syed Mahmood, Varinder Singh and Ayah R. Hilles: conceptualization, collecting information, revising draft. Shruti Chopra and Amit Bhatia: revising draft, and finalizing the manuscript.
Conflicts of interest
The author declares no conflict of interest, financial or otherwise.
Acknowledgements
Research grant (5/8-4/5/Env/2020-NCD-II Dated 22/12/2021) under Indian Council of Medical Research (ICMR), New Delhi, India. The authors would like to thank the Maharaja Ranjit Singh Punjab Technical University (MRSPTU) in Bathinda, India, for providing the research facilities. The authors would like to thank the Indian Council of Medical Research in New Delhi, India.
References
- M. Kumar, D. Kumar, Y. Garg, S. Mahmood, S. Chopra and A. Bhatia, Int. J. Biol. Macromol., 2023, 127331 CrossRef CAS PubMed.
- M. Kumari and D. K. Nanda, Burns, 2023, 49, 1003–1016 CrossRef PubMed.
- I. Kuchyn and V. Horoshko, BMC Anesthesiol., 2023, 23, 1–10 CrossRef PubMed.
- K. Raziyeva, Y. Kim, Z. Zharkinbekov, K. Kassymbek, S. Jimi and A. Saparov, Biomolecules, 2021, 11, 700 CrossRef CAS PubMed.
- N. Graves, C. J. Phillips and K. Harding, Br. J. Dermatol., 2022, 187, 141–148 CrossRef CAS PubMed.
- S. Probst, C. Saini, G. Gschwind, A. Stefanelli, P. Bobbink, M. Pugliese, S. Cekic, D. Pastor and G. Gethin, Int. Wound J., 2021, 10, 148 CAS.
- K. McDermott, M. Fang, A. J. M. Boulton, E. Selvin and C. W. Hicks, Diabetes Care, 2023, 46, 209–221 CrossRef PubMed.
- P. M. G. Sardo, J. P. F. Teixeira, A. M. S. F. Machado, B. F. Oliveira and I. M. Alves, J. Tissue Viability, 2023, 32, 179–187 CrossRef PubMed.
- C. Siotos, A. M. Bonett, G. Damoulakis, A. Z. Becerra, G. Kokosis, K. Hood, A. H. Dorafshar and D. S. Shenaq, ePlasty, 2022, 22, e19 Search PubMed.
- J. L. Seidelman, C. R. Mantyh and D. J. Anderson, J. Am. Med. Assoc., 2023, 329, 244–252 CrossRef PubMed.
- D. A. Mengistu, A. Alemu, A. A. Abdukadir, A. Mohammed Husen, F. Ahmed and B. Mohammed, Inq. J. Heal. Care Organ. Provision, Financ., 2023, 60, 00469580231168746 Search PubMed.
- E. Opriessnig, H. Luze, C. Smolle, A. Draschl, R. Zrim, M. Giretzlehner, L.-P. Kamolz and S. P. Nischwitz, Burns, 2023, 49, 1–14 CrossRef PubMed.
- A. Markiewicz-Gospodarek, M. Kozioł, M. Tobiasz, J. Baj, E. Radzikowska-Büchner and A. Przekora, Int. J. Environ. Res. Public Health, 2022, 19, 1338 CrossRef CAS PubMed.
- N. W. Shammas, Vasc. Health Risk Manag., 2007, 3, 229–234 CrossRef PubMed.
- M. A. Allison, D. G. Armstrong, P. P. Goodney, N. M. Hamburg, L. Kirksey, K. J. Lancaster, C. I. Mena-Hurtado, S. Misra, D. J. Treat-Jacobson and K. T. White Solaru, Circulation, 2023, 148, 286–296 CrossRef PubMed.
-
T. F. Herman and B. Bordoni.
- L. Cañedo-Dorantes and M. Cañedo-Ayala, Int. J. Inflammation, 2019, 3706315 Search PubMed.
- V. Falanga, R. R. Isseroff, A. M. Soulika, M. Romanelli, D. Margolis, S. Kapp, M. Granick and K. Harding, Nat. Rev. Dis. Primers, 2022, 8, 1–21 CrossRef PubMed.
- L. Qiao, Y. Liang, J. Chen, Y. Huang, S. A. Alsareii, A. M. Alamri, F. A. Harraz and B. Guo, Bioact. Mater., 2023, 30, 129–141 CAS.
- S.-Y. Ren, Y.-S. Liu, G.-J. Zhu, M. Liu, S.-H. Shi, X.-D. Ren, Y.-G. Hao and R.-D. Gao, World J. Clin. Cases, 2020, 8, 5070 CrossRef PubMed.
- S. Zhang, G. Ge, Y. Qin, W. Li, J. Dong, J. Mei, R. Ma, X. Zhang, J. Bai and C. Zhu, Mater. Today Bio, 2023, 18, 100508 CrossRef CAS PubMed.
- S. al Guo and L. A. DiPietro, J. Dent. Res., 2010, 89, 219–229 CrossRef PubMed.
- M. B. Dreifke, A. A. Jayasuriya and A. C. Jayasuriya, Mater. Sci. Eng., C, 2015, 48, 651–662 CrossRef CAS PubMed.
- T. Maheswary, A. A. Nurul and M. B. Fauzi, Pharmaceutics, 2021, 13, 981 CrossRef CAS PubMed.
- J. M. Reinke and H. Sorg, Eur. Surg. Res., 2012, 49, 35–43 CrossRef CAS PubMed.
- W. R. Perera, J. R. Hurst, T. M. A. Wilkinson, R. J. Sapsford, H. Müllerova, G. C. Donaldson and J. A. Wedzicha, Eur. Respir. J., 2007, 29, 527–534 CrossRef CAS PubMed.
- C. Politis, J. Schoenaers, R. Jacobs and J. O. Agbaje, Front. Physiol., 2016, 7, 507 Search PubMed.
-
H. Kirwan and R. Pignataro, in Patholology and Intervention in Musculoskeletal Rehabilitation, Elsevier, Philadelphia, US, 2nd edn, 2016, ch. 2, pp. 25–62 Search PubMed.
- D. C. Bosanquet and K. G. Harding, Wound Repair Regen., 2014, 22, 143–150 CrossRef PubMed.
- E. Lebrun, M. Tomic-Canic and R. S. Kirsner, Wound Repair Regen., 2010, 18, 433–438 CrossRef PubMed.
- G. Petruk, J. Petrlova, F. Samsudin, R. Del Giudice, P. J. Bond and A. Schmidtchen, Biomolecules, 2020, 10, 1572 CrossRef CAS PubMed.
- M. H. E. Hermans and T. Treadwell, Microbiol. Wounds, 2010, 83–134 CAS.
- M. Kumar, P. Keshwania, S. Chopra, S. Mahmood and A. Bhatia, AAPS PharmSciTech, 2023, 24, 1–26 Search PubMed.
- H. J. Lee and Y. J. Jang, Int. J. Mol. Sci., 2018, 19, 711 CrossRef PubMed.
- S. R. Nussbaum, M. J. Carter, C. E. Fife, J. DaVanzo, R. Haught, M. Nusgart and D. Cartwright, Value Heal., 2018, 21, 27–32 CrossRef PubMed.
- D. M. Bermudez, D. A. Canning and K. W. Liechty, J. Pediatr. Urol., 2011, 7, 324–331 CrossRef PubMed.
- W. H. Peranteau, L. Zhang, N. Muvarak, A. T. Badillo, A. Radu, P. W. Zoltick and K. W. Liechty, J. Invest. Dermatol., 2008, 128, 1852–1860 CrossRef CAS.
- G. G. Gauglitz, H. C. Korting, T. Pavicic, T. Ruzicka and M. G. Jeschke, Mol. Med., 2011, 17, 113–125 CAS.
- M. Kumar, S. Mahmood and U. K. Mandal, Curr. Pharm. Des., 2022, 28, 1480–1492 CrossRef CAS PubMed.
- D. Zhang, G. Cai, S. Mukherjee, Y. Sun, C. Wang, B. Mai, K. Liu, C. Yang and Y. Chen, ACS Appl. Mater. Interfaces, 2020, 12, 5542–5556 CrossRef CAS PubMed.
- S. Guo, Q. Sun, Y. Zhou, S. Tong, X. Sun, K. Li and M. Lv, Med. Sci. Monit. Int. Med. J. Exp. Clin. Res., 2020, 26, e921263–e921261 CAS.
- Y. Har-Shai and C. C. Zouboulis, Plast. Reconstr. Surg., 2015, 136, 397e–398e CrossRef CAS PubMed.
- W.-R. Lee, S.-C. Shen, S. A. Al-Suwayeh, H.-H. Yang, C.-Y. Yuan and J.-Y. Fang, J. Controlled Release, 2011, 153, 240–248 CrossRef CAS PubMed.
- Z. Zhang, J. Chen, J. Huang, Y. Wo, Y. Zhang and X. Chen, Nanoscale Res. Lett., 2018, 13, 1–12 CrossRef.
- A. J. Singer and R. A. F. Clark, N. Engl. J. Med., 1999, 341, 738–746 CrossRef CAS.
- C. D. Marshall, M. S. Hu, T. Leavitt, L. A. Barnes, H. P. Lorenz and M. T. Longaker, Adv. Wound Care, 2018, 7, 29–45 CrossRef.
- C. Profyris, C. Tziotzios and I. Do Vale, J. Am. Acad. Dermatol., 2012, 66, 1–10 CrossRef CAS PubMed.
- L. Wang, J. Yang, B. Ran, X. Yang, W. Zheng, Y. Long and X. Jiang, ACS Appl. Mater. Interfaces, 2017, 9, 32545–32553 CrossRef CAS PubMed.
- X. Wan, S. Liu, X. Xin, P. Li, J. Dou, X. Han, I.-K. Kang, J. Yuan, B. Chi and J. Shen, Chem. Eng. J., 2020, 400, 125964 CrossRef CAS.
- J. Li, C. Zhou, C. Luo, B. Qian, S. Liu, Y. Zeng, J. Hou, B. Deng, Y. Sun and J. Yang, Theranostics, 2019, 9, 5839 CrossRef CAS PubMed.
- U. Park, M. S. Lee, J. Jeon, S. Lee, M. P. Hwang, Y. Wang, H. S. Yang and K. Kim, Acta Biomater., 2019, 90, 179–191 CrossRef CAS PubMed.
- M. Hesketh, K. B. Sahin, Z. E. West and R. Z. Murray, Int. J. Mol. Sci., 2017, 18, 1545 CrossRef CAS PubMed.
- H. D. Zomer, T. da Silva Jeremias, B. Ratner and A. G. Trentin, Cytotherapy, 2020, 22, 247–260 CrossRef CAS PubMed.
- C. Kalirajan and T. Palanisamy, Adv. Healthcare Mater., 2020, 9, 2000247 CrossRef CAS PubMed.
- X. Zheng, Z. Ding, W. Cheng, Q. Lu, X. Kong, X. Zhou, G. Lu and D. L. Kaplan, Adv. Healthcare Mater., 2020, 9, 2000041 CrossRef CAS PubMed.
- J. Chen, H. Wang, L. Mei, B. Wang, Y. Huang, G. Quan, C. Lu, T. Peng, X. Pan and C. Wu, J. Mater. Chem. B, 2020, 8, 2573–2588 RSC.
- M. Ekor, Front. Pharmacol., 2014, 4, 177 Search PubMed.
- M. Sharma, S. Chopra and S. B. Prasad, Int. J. Pharmacogn. Phytochem. Res., 2015, 7, 197–200 Search PubMed.
- A. K. Khan, S. Kaleem, F. Pervaiz, T. A. Sherazi, S. A. Khan, F. A. Khan, T. Jamshaid, M. I. Umar, W. Hassan and M. Ijaz, J. Drug Delivery Sci. Technol., 2023, 79, 103987 CrossRef CAS.
- M. Manconi, M. L. Manca, C. Caddeo, D. Valenti, C. Cencetti, O. Diez-Sales, A. Nacher, S. Mir-Palomo, M. C. Terencio and D. Demurtas, Nanomedicine, 2018, 14, 569–579 CrossRef CAS PubMed.
- M. Manconi, M. L. Manca, C. Caddeo, C. Cencetti, C. di Meo, N. Zoratto, A. Nacher, A. M. Fadda and P. Matricardi, Eur. J. Pharm. Biopharm., 2018, 127, 244–249 CrossRef CAS PubMed.
- G. Di Fabio, V. Romanucci, C. Di Marino, A. Pisanti and A. Zarrelli, Curr. Pharm. Biotechnol., 2015, 16, 506–516 CAS.
- P. Monika, M. N. Chandraprabha, A. Rangarajan, P. V. Waiker and K. N. C. Murthy, Front. Nutr., 2022, 127, 104803 CAS.
- P. S. Murphy and G. R. D. Evans, Plast. Surg. Int., 2012, 190436 Search PubMed.
- T. Dongala, N. K. Katari, S. K. Ettaboina, A. Krishnan, M. M. Tambuwala and K. Dua, Front. Mol. Biosci., 2021, 441 Search PubMed.
- T. Kartnig, Herbs, Spices, Med. Plants Recent Adv. Bot. Hortic. Pharmacol., 2024, 10, 86 Search PubMed.
- Q. Hou, M. Li, Y. Lu, D. Liu and C. Li, Exp. Ther. Med., 2016, 12, 1269–1274 CrossRef CAS PubMed.
- C. L. Cheng, J. S. Guo, J. Luk and M. W. L. Koo, Life Sci., 2004, 74, 2237–2249 CrossRef CAS PubMed.
- S. Bandopadhyay, S. Mandal, M. Ghorai, N. K. Jha, M. Kumar, D. Radha and A. Ghosh, J. Cell. Mol. Med., 2023, 27, 593–608 CrossRef CAS PubMed.
- Y. Zhou, S. Wang, J. Zhao and P. Fang, Ann. Transl. Med., 2016, 761–769 Search PubMed.
- Z. He, Y. Hu, Z. Niu, K. Zhong, T. Liu, M. Yang, L. Ji and W. Hu, J. Ethnopharmacol., 2023, 302, 115865 CrossRef CAS PubMed.
- G. M. Seon, M. H. Lee, M.-A. Koo, S. H. Hong, Y. J. Park, H. K. Jeong, B.-J. Kwon, D. Kim and J.-C. Park, Mater. Sci. Eng., C, 2021, 121, 111837 CrossRef CAS PubMed.
- Y. Zhang, Y. Han, J. Dong, F. Li and Y. Sun, Balkan Med. J., 2024, 41, 23 CrossRef PubMed.
-
S. Barge, D. Jade and N. C. Talukdar, in Potent Anticancer Medicinal Plants, Apple Academic Press, 2024, pp. 19–37 Search PubMed.
- T. Phaechamud, K. Yodkhum, J. Charoenteeraboon and Y. Tabata, Mater. Sci. Eng., C, 2015, 50, 210–225 CrossRef CAS PubMed.
- N. Namviriyachote, P. Muangman, K. Chinaroonchai, C. Chuntrasakul and G. C. Ritthidej, Int. J. Biol. Macromol., 2020, 143, 510–520 CrossRef CAS PubMed.
- M. Kumar, R. Dogra and U. K. Mandal, J. Drug Delivery Sci. Technol., 2022, 103533 CrossRef CAS.
-
M. Kumar, U. K. Mandal and S. Mahmood, Adv. Mod. Approaches Drug Delivery, 2023, pp. 1–32 Search PubMed.
- R. Jain, I. Sarode, G. Singhvi and S. K. Dubey, Curr. Pharm. Des., 2020, 26, 4615–4623 CrossRef CAS PubMed.
- J. Prabha, M. Kumar, D. Kumar, S. Chopra and A. Bhatia, Curr. Drug Delivery, 2024, 21, 1082–1105 CrossRef CAS PubMed.
- D. Patel, B. Patel and H. Thakkar, Eur. J. Lipid Sci. Technol., 2021, 123, 2000264 CrossRef CAS.
-
D. Medina-Cruz, B. Saleh, A. Vernet-Crua, A. Ajo, A. K. Roy and T. J. Webster, in Wound Healing, Tissue Repair, and Regeneration in Diabetes, Elsevier, 2020, pp. 439–488 Search PubMed.
- M. R. Farahpour and H. Hamishehkar, Colloids Surf., A, 2021, 610, 125748 CrossRef.
- A. Barroso, H. Mestre, A. Ascenso, S. Simões and C. Reis, Nano Sel., 2020, 1, 443–460 CrossRef.
- A. El Ayadi, J. W. Jay and A. Prasai, Int. J. Mol. Sci., 2020, 21, 1105 CrossRef CAS PubMed.
- C. Liu, M. H. Y. Teo, S. L. T. Pek, X. Wu, M. L. Leong, H. M. Tay, H. W. Hou, C. Ruedl, S. E. Moss and J. Greenwood, Diabetes, 2020, 69, 2467–2480 CrossRef CAS PubMed.
- S. Wichaiyo, S. Lax, S. J. Montague, Z. Li, B. Grygielska, J. A. Pike, E. J. Haining, A. Brill, S. P. Watson and J. Rayes, Haematologica, 2019, 104, 1648 CrossRef CAS PubMed.
- P. A. Borges, I. Waclawiak, J. L. Georgii, V. d. S. Fraga-Junior, J. F. Barros, F. S. Lemos, T. Russo-Abrahão, E. M. Saraiva, C. M. Takiya and R. Coutinho-Silva, Front. Immunol., 2021, 12, 651740 CrossRef CAS PubMed.
- R. J. Boucek, Otolaryngol. Clin. North Am., 1984, 17, 243–264 CrossRef CAS PubMed.
- N. Laurens, P. d Koolwijk and M. P. M. De Maat, J. Thromb. Haemost., 2006, 4, 932–939 CrossRef CAS PubMed.
- D. M. Monroe and M. Hoffman, Haemophilia, 2012, 18, 11–16 CrossRef PubMed.
- S. Singh, A. Young and C.-E. McNaught, Surgery, 2017, 35, 473–477 Search PubMed.
- B. Guo, R. Dong, Y. Liang and M. Li, Nat. Rev. Chem., 2021, 5, 773–791 CrossRef CAS PubMed.
- S. Willenborg, L. Injarabian and S. A. Eming, Cold Spring Harbor Perspect. Biol., 2022, 14, a041216 CrossRef CAS PubMed.
- A. Nurkesh, A. Jaguparov, S. Jimi and A. Saparov, Front. Cell Dev. Biol., 2020, 8, 638 CrossRef PubMed.
- Z. Xu, S. Han, Z. Gu and J. Wu, Adv. Healthcare Mater., 2020, 9, 1901502 CrossRef CAS PubMed.
- M. G. Visha and M. Karunagaran, Int. J. Clin. Correl., 2019, 3, 50–59 Search PubMed.
- M. G. El Baassiri, L. Dosh, H. Haidar, A. Gerges, S. Baassiri, A. Leone, F. Rappa and A. Jurjus, Burns, 2023, 49, 989–1002 CrossRef PubMed.
- E. Grambow, H. Sorg, C. G. G. Sorg and D. Strüder, Med. Sci., 2021, 9, 55 CAS.
- P. Wei, C. Zhong, X. Yang, F. Shu, S. Xiao, T. Gong, P. Luo, L. Li, Z. Chen and Y. Zheng, Burns Trauma, 2020, 8, tkaa020 CrossRef PubMed.
-
S. R. Goldberg and R. F. Diegelmann, Crit. Limb Ischemia Acute Chronic, 2017, pp. 131–136 Search PubMed.
- A. W. Jatoi, H. Ogasawara, I. S. Kim and Q.-Q. Ni, Mater. Lett., 2019, 241, 168–171 CrossRef CAS.
- H. Ren, F. Zhao, Q. Zhang, X. Huang and Z. Wang, Burns Trauma, 2022, 10, tkac003 CrossRef PubMed.
- J. M. Baron, M. Glatz and E. Proksch, Dermatology, 2020, 236, 593–600 CrossRef CAS PubMed.
- F. Yang, X. Bai, X. Dai and Y. Li, Regen. Med., 2021, 16, 373–390 CrossRef CAS PubMed.
- P. Krzyszczyk, R. Schloss, A. Palmer and F. Berthiaume, Front. Physiol., 2018, 9, 419 CrossRef PubMed.
- F. Liaqat, L. Xu, M. I. Khazi, S. Ali, M. U. Rahman and D. Zhu, Ind. Crops Prod., 2023, 204, 117372 CrossRef CAS.
- B. R. Albuquerque, M. A. Prieto, M. F. Barreiro, A. Rodrigues, T. P. Curran, L. Barros and I. C. F. R. Ferreira, Ind. Crops Prod., 2017, 95, 404–415 CrossRef CAS.
- M. N. Safdar, T. Kausar, S. Jabbar, A. Mumtaz, K. Ahad and A. A. Saddozai, J. Food Drug Anal., 2017, 25, 488–500 CrossRef CAS PubMed.
- C. Monton, S. Settharaksa, C. Luprasong and T. Songsak, Rev. Bras. Farmacogn., 2019, 29, 254–261 CrossRef CAS.
- F. Pittella, R. C. Dutra, D. D. Junior, M. T. P. Lopes and N. R. Barbosa, Int. J. Mol. Sci., 2009, 10, 3713–3721 CrossRef CAS PubMed.
- C. Zhao, X. He, C. Li, L. Yang, Y. Fu, K. Wang, Y. Zhang and Y. Ni, Appl. Sci., 2016, 6, 19 CrossRef.
-
M. Garcia-Vaquero, G. Rajauria and B. Tiwari, in Sustainable seaweed technologies, Elsevier, 2020, pp. 171–189 Search PubMed.
- M. C. Florczak, Neurobiol. Aging, 2015, 36, 2577–2586 CrossRef PubMed.
- I. E. Orhan, E. Atasu, F. S. Senol, N. Ozturk, B. Demirci, K. Das and N. Sekeroglu, Ind. Crops Prod., 2013, 47, 316–322 CrossRef CAS.
-
M. A. López-Bascón and M. D. L. De Castro, in Liquid-phase extraction, Elsevier, 2020, pp. 327–354 Search PubMed.
- P. N. Kunene and P. N. Mahlambi, J. Environ. Chem. Eng., 2020, 8, 103665 CrossRef CAS.
- K. O. Fagbemi, D. A. Aina and O. O. Olajuyigbe, Sci. World J., 2021, 2021, 1–8 CrossRef PubMed.
- P. T. Selvi, M. S. Kumar, R. Rajesh and T. Kathiravan, Asian J. Res. Pharm. Sci., 2012, 2, 76–79 Search PubMed.
- M. Rahman, S. Hossain, A. Rahaman, N. Fatima, T. Nahar, B. Uddin and M. A. Basunia, J. Pharmacogn. Phytochem., 2013, 1, 27–32 Search PubMed.
- W. Thong-On, T. Pathomwichaiwat, S. Boonsith, W. Koo-Amornpattana and S. Prathanturarug, Sci. Rep., 2021, 11, 22026 CrossRef CAS PubMed.
- A. Yaqub, Z. Iqbal, T. Toyota, N. Chaudhary, A. Altaf and S. R. Ahmad, Clin Med Bio Chem, 2020, 8, 1–9 Search PubMed.
- Y. Shen, A. Liu, M. Ye, L. Wang, J. Chen, X. Wang and C. Han, Chromatographia, 2009, 70, 431–438 CrossRef CAS.
- S. B. Bagade and M. Patil, Crit. Rev. Anal. Chem., 2021, 51, 138–149 CrossRef CAS PubMed.
-
L. Gomez, B. Tiwari and M. Garcia-Vaquero, in Sustainable seaweed technologies, Elsevier, 2020, pp. 207–224 Search PubMed.
- C. Duval, J. Parkinson's Dis., 2016, 6, 685–698 Search PubMed.
- C. Duval, Quantum Grav., 2014, 31, 092001 CrossRef.
- A. Bhatia, B. Singh, S. Wadhwa, K. Raza and O. P. Katare, Pharm. Dev. Technol., 2014, 19, 160–163 CrossRef CAS PubMed.
-
M. Kumar, U. K. Mandal and S. Mahmood, Dermatological formulations, Academic Press, 1st edn, 2024 Search PubMed.
- E. Proksch, E. Berardesca, L. Misery, J. Engblom and J. Bouwstra, J. Dermatolog. Treat., 2020, 31, 716–722 CrossRef PubMed.
- M. J. Blair, J. D. Jones, A. E. Woessner and K. P. Quinn, Adv. Wound Care, 2020, 9, 127–143 CrossRef PubMed.
- X. Liu, B. Testa and A. Fahr, Pharm. Res., 2011, 28, 962–977 CrossRef CAS PubMed.
- M. Kumar, A. Sharma, S. Mahmood, A. Thakur, M. A. Mirza and A. Bhatia, J. Dispersion Sci. Technol., 2023, 1–14 Search PubMed.
-
K. Sugibayashi, Ski. Permeat. Dispos. Ther. Cosmeceutical Compd, 2017, pp. 3–11 Search PubMed.
-
M. S. Balda and M. R. García-Villegas.
-
S. Nafisi and H. I. Maibach, in Emerging nanotechnologies in immunology, Elsevier, 2018, pp. 47–88 Search PubMed.
- M. Elmowafy, Colloids Surf., B, 2021, 203, 111748 CrossRef CAS PubMed.
- E. Arribas-López, N. Zand, O. Ojo, M. J. Snowden and T. Kochhar, Int. J. Environ. Res. Public Health, 2022, 19, 3266 CrossRef PubMed.
-
Y. Y. Zhang, L. Chen, L. Zhang and L. Zhang, in Annual Conference Proceedings of the World Federation of Chinese Materia Medica Committee (11), 2011, pp. 352–355.
- S. Pattnaik, S. Mohanty, S. K. Sahoo and C. Mohanty, J. Drug Delivery Sci. Technol., 2023, 104546 CrossRef CAS.
- K. Raza, B. Singh, S. Lohan, G. Sharma, P. Negi, Y. Yachha and O. P. Katare, Int. J. Pharm., 2013, 456, 65–72 CrossRef CAS PubMed.
- K. Raza, O. P. Katare, A. Setia, A. Bhatia and B. Singh, J. Microencapsul., 2013, 30, 225–236 CrossRef CAS PubMed.
- S. Chaturvedi and A. Garg, J. Drug Delivery Sci. Technol., 2021, 62, 102355 CrossRef CAS.
- M. S. Roberts, Y. Mohammed, M. N. Pastore, S. Namjoshi, S. Yousef, A. Alinaghi, I. N. Haridass, E. Abd, V. R. Leite-Silva and H. A. E. Benson, J. Controlled Release, 2017, 247, 86–105 CrossRef CAS PubMed.
- O. Katare, K. Raza, B. Singh and S. Dogra, Indian J. Dermatol. Venereol. Leprol., 2010, 76, 612 CrossRef PubMed.
- A. Okyar, M. Nuriyev, A. Yildiz, Z. Pala-Kara, N. Ozturk and E. Kaptan, Arch. Pharmacal Res., 2010, 33, 1781–1788 CrossRef CAS PubMed.
- J. Zhou, G. Feng, W. Zhou, A. Ren, Y. Wu, D. Zhang and H. Dai, J. Orofac. Orthop., 2011, 72, 457–468 CrossRef PubMed.
- S. Sawatdee, K. Choochuay, W. Chanthorn and T. Srichana, Acta Pharm., 2016, 66, 233–244 CrossRef CAS PubMed.
- C. Choipang, T. Buntum, P. Chuysinuan, S. Techasakul, P. Supaphol and O. Suwantong, Polym. Adv. Technol., 2021, 32, 1187–1193 CrossRef CAS.
- J. Huang, X. Zhou, L. Xia, W. Liu, F. Guo, J. Liu and W. Liu, Int. Wound J., 2021, 18, 598–607 CrossRef PubMed.
- J.-H. Lee, H.-L. Kim, M. H. Lee, K. E. You, B.-J. Kwon, H. J. Seo and J.-C. Park, Phytomedicine, 2012, 19, 1223–1227 CrossRef CAS PubMed.
- U. K. M. M. Kumar, J. Clin. Exp. Dermatol. Res., 2021, 12, 1–7 Search PubMed.
-
B. Alberts, Molecular biology of the cell, Garland science, 2017 Search PubMed.
- H. N. Wilkinson and M. J. Hardman, Open Biol., 2020, 10, 200223 CrossRef CAS PubMed.
- P. Shu, L. Tianzeng, L. Yeyang, C. Xiaodong, X. Julin, X. Yingbin and Q. Shaohai, Acta Acad. Med. Mil. Tertiae, 2005, 27, 49–52 Search PubMed.
- A. Shukla, A. M. Rasik and B. N. Dhawan, Phyther. Res., 1999, 13, 50–54 CrossRef CAS.
- B. H. I. Ruszymah, S. R. Chowdhury, N. A. B. A. Manan, O. S. Fong, M. I. Adenan and A. Bin Saim, J. Ethnopharmacol., 2012, 140, 333–338 CrossRef PubMed.
- B. S. Shetty, S. L. Udupa, A. L. Udupa and S. N. Somayaji, Int. J. Low. Extrem. Wounds, 2006, 5, 137–143 CrossRef PubMed.
- A. S. Ahmed, M. Taher, U. K. Mandal, J. M. Jaffri, D. Susanti, S. Mahmood and Z. A. Zakaria, BMC Complementary Altern. Med., 2019, 19, 1–7 CrossRef PubMed.
- S. G. Jin, K. S. Kim, A. M. Yousaf, D. W. Kim, S. W. Jang, M.-W. Son, Y. H. Kim, C. S. Yong, J. O. Kim and H.-G. Choi, Int. J. Pharm., 2015, 490, 240–247 CrossRef CAS PubMed.
- H. A. Azis, M. Taher, A. S. Ahmed, W. Sulaiman, D. Susanti, S. R. Chowdhury and Z. A. Zakaria, S. Afr. J. Bot, 2017, 108, 163–174 CrossRef.
- A. S. Ahmed, U. K. Mandal, M. Taher, D. Susanti and J. M. Jaffri, Pharm. Dev. Technol., 2018, 23, 751–760 CrossRef CAS PubMed.
- S. Chatterjee, T. Prakash, D. Kotrsha, N. R. Rao and D. Goli, Chin. Med., 2011, 2, 138 CrossRef.
- A. Shukla, A. M. Rasik, G. K. Jain, R. Shankar, D. K. Kulshrestha and B. N. Dhawan, J. Ethnopharmacol., 1999, 65, 1–11 CrossRef CAS PubMed.
- Y. Liu, J. Zhao, X. Mu, J. Deng, X. Wu, W. He, Y. Liu, R. Gu, F. Han and X. Nie, J. Ethnopharmacol., 2024, 319, 117266 CrossRef CAS PubMed.
- J. Somboonwong, M. Kankaisre, B. Tantisira and M. H. Tantisira, BMC Complementary Altern. Med., 2012, 12, 1–7 CrossRef PubMed.
- V. Paocharoen, J. Med. Assoc. Thai., 2010, 93, S166–S170 Search PubMed.
- G. Calapai, Eur. Med. Agency, 2012, 44 Search PubMed.
- X. Feng, D. Huang, D. Lin, L. Zhu, M. Zhang, Y. Chen and F. Wu, J. Investig. Surg., 2021, e202116068 Search PubMed.
- F. Zhang and W. Lineaweaver, Ann. Plast. Surg., 2011, 66, 581–582 CrossRef CAS PubMed.
- A. Devkota, S. Dall'Acqua, S. Comai, G. Innocenti and P. K. Jha, Biochem. Syst. Ecol., 2010, 38, 12–22 CrossRef CAS.
- L. Lu, K. Ying, S. Wei, Y. Fang, Y. Liu, H. Lin, L. Ma and Y. Mao, Int. J. Dermatol., 2004, 43, 801–807 CrossRef CAS PubMed.
- L. Lu, K. Ying, S. Wei, Y. Liu, H. Lin and Y. Mao, Br. J. Dermatol., 2004, 151, 571–578 CrossRef CAS PubMed.
- H. Rosen, A. Blumenthal and J. McCallum, Proc. Soc. Exp. Biol. Med., 1967, 125, 279–280 CrossRef CAS PubMed.
- J. C. Watkins and D. E. Jane, Br. J. Pharmacol., 2006, 147, S100–S108 CrossRef CAS PubMed.
- S. Jayanarayanan, S. Smijin, K. T. Peeyush, T. R. Anju and C. S. Paulose, Chem.-Biol. Interact., 2013, 201, 39–48 CrossRef CAS PubMed.
- F. Qi, L. Yang, Z. Tian, M.-G. Zhao, S. Liu and J. An, Neural Regen. Res., 2014, 9, 1275 CrossRef CAS PubMed.
- L. Wang, T. Guo, Y. Guo and Y. Xu, Mol. Med. Rep., 2020, 22, 2364–2372 CrossRef CAS PubMed.
- H. Yu, Z. Yin, S. Yang, S. Ma and R. Qu, Phyther. Res., 2016, 30, 469–475 CrossRef CAS PubMed.
- R.-H. Du, J. Tan, X.-Y. Sun, M. Lu, J.-H. Ding and G. Hu, Int. J. Neuropsychopharmacol., 2016, 19, pyw037 CrossRef PubMed.
- X.-Y. Deng, H.-Y. Li, J.-J. Chen, R.-P. Li, R. Qu, Q. Fu and S.-P. Ma, Behav. Brain Res., 2015, 291, 12–19 CrossRef CAS PubMed.
- C. C. Barua, P. Haloi, B. Saikia, K. Sulakhiya, D. C. Pathak, S. Tamuli, H. Rizavi and X. Ren, Pharm. Biol., 2018, 56, 245–252 CrossRef CAS PubMed.
- C. Zhang, S. Chen, Z. Zhang, H. Xu, W. Zhang, D. Xu, B. Lin and Y. Mei, Med. Sci. Monit., 2020, 26, e920325–e920321 CAS.
- S. Chen, Z.-J. Yin, C. Jiang, Z.-Q. Ma, Q. Fu, R. Qu and S.-P. Ma, Pharmacol. Biochem. Behav., 2014, 122, 7–15 CrossRef CAS PubMed.
- Y. Luo, C. Fu, Z. Wang, Z. Zhang, H. Wang and Y. Liu, Mol. Med. Rep., 2015, 12, 8294–8300 CrossRef CAS PubMed.
- A. Bandawane and R. Saudagar, J. Drug Delivery Ther., 2019, 9, 517–521 CrossRef.
- M. Kumar, A. R. Hilles, S. H. A. Almurisi, A. Bhatia and S. Mahmood, JCIS Open, 2023, 100095 CrossRef.
- M. Ghezzi, S. Pescina, C. Padula, P. Santi, E. Del Favero, L. Cantù and S. Nicoli, J. Controlled Release, 2021, 332, 312–336 CrossRef CAS PubMed.
- B. S. Makhmalzade and F. Chavoshy, J. Adv. Pharm. Technol. Res., 2018, 9, 2 CrossRef CAS PubMed.
- A. S. Manjappa, P. S. Kumbhar, A. B. Patil, J. I. Disouza and V. B. Patravale, Crit. Rev. Ther. Drug Carr. Syst., 2019, 1–58 Search PubMed.
- A. Parra, I. Jarak, A. Santos, F. Veiga and A. Figueiras, Materials, 2021, 14, 7278 CrossRef CAS PubMed.
- P. A. Penttilä, S. Vierros, K. Utriainen, N. Carl, L. Rautkari, M. Sammalkorpi and M. Österberg, Langmuir, 2019, 35, 8373–8382 Search PubMed.
- R. Paliwal, S. R. Paliwal, R. Kenwat, B. Das Kurmi and M. K. Sahu, Expert Opin. Ther. Pat., 2020, 30, 179–194 CrossRef CAS PubMed.
- M. Liu, J. Wen and M. Sharma, Curr. Pharm. Des., 2020, 26, 3203–3217 CrossRef CAS PubMed.
- V. R. Salvi and P. Pawar, J. Drug Delivery Sci. Technol., 2019, 51, 255–267 CrossRef CAS.
- I. Chauhan, M. Yasir, M. Verma and A. P. Singh, Adv. Pharm. Bull., 2020, 10, 150 CrossRef CAS PubMed.
- B. Begines, T. Ortiz, M. Pérez-Aranda, G. Martínez, M. Merinero, F. Argüelles-Arias and A. Alcudia, Nanomaterials, 2020, 10, 1403 CrossRef CAS PubMed.
- N. S. Ayumi, S. Sahudin, Z. Hussain, M. Hussain and N. H. A. Samah, Drug Delivery Transl. Res., 2019, 9, 482–496 CrossRef CAS PubMed.
- L.-L. Wang, S. Huang, H.-H. Guo, Y.-X. Han, W.-S. Zheng and J.-D. Jiang, Drug Des., Dev. Ther., 2020, 14, 2125–2126 CrossRef PubMed.
- S. Hiranphinyophat, A. Otaka, Y. Asaumi, S. Fujii and Y. Iwasaki, Colloids Surf., B, 2021, 197, 111423 CrossRef CAS PubMed.
- N. H. Che Marzuki, R. A. Wahab and M. Abdul Hamid, Biotechnol. Biotechnol. Equip., 2019, 33, 779–797 CrossRef.
- Y. Singh, J. G. Meher, K. Raval, F. A. Khan, M. Chaurasia, N. K. Jain and M. K. Chourasia, J. Controlled Release, 2017, 252, 28–49 CrossRef CAS PubMed.
- W. Cai, P. C. Calder, M. F. Cury-Boaventura, E. De Waele, J. Jakubowski and G. Zaloga, Nutrients, 2018, 10, 776 CrossRef PubMed.
- J. Alvarez-Trabado, Y. Diebold and A. Sanchez, Int. J. Pharm., 2017, 532, 204–217 CrossRef CAS PubMed.
- S. Tamizharasi, A. Dubey, V. Rathi and J. C. Rathi, J. Young Pharm., 2019, 1, 205–209 Search PubMed.
- B. A. Witika, L. L. Mweetwa, K. O. Tshiamo, K. Edler, S. K. Matafwali, P. V. Ntemi, M. T. R. Chikukwa and P. A. Makoni, J. Pharm. Pharmacol., 2021, 73, 1427–1441 CrossRef PubMed.
- R. Bartelds, M. H. Nematollahi, T. Pols, M. C. A. Stuart, A. Pardakhty, G. Asadikaram and B. Poolman, PLoS One, 2018, 13, e0194179 CrossRef PubMed.
-
G. Sharma, K. Thakur, A. Mahajan, G. S. Randhawa, B. Singh and O. P. Katare, in NanoAgroceuticals & NanoPhytoChemicals, CRC Press, 2018, pp. 277–295 Search PubMed.
- A. Saxena and M. L. Kori, J. Adv. Sci. Res., 2020, 11, 27–34 CAS.
-
A. A. Kassem and S. H. Abd El-Alim, Nanopharmaceuticals Princ. Appl, 2021, vol. 2, 155–209 Search PubMed.
- M. Barani, E. Sangiovanni, M. Angarano, M. A. Rajizadeh, M. Mehrabani, S. Piazza, H. V. Gangadharappa, A. Pardakhty, M. Mehrbani and M. Dell'Agli, Int. J. Nanomed., 2021, 16, 6983 CrossRef CAS PubMed.
- P. Morganti, E. Ruocco, R. Wolf and V. Ruocco, Clin. Dermatol., 2001, 19, 489–501 CrossRef CAS PubMed.
- K. Raza, M. Kumar, P. Kumar, R. Malik, G. Sharma, M. Kaur and O. P. Katare, BioMed. Res. Int., 2014, 1–10 Search PubMed.
- P. Desai, R. R. Patlolla and M. Singh, Mol. Membr. Biol., 2010, 27, 247–259 CrossRef CAS PubMed.
- S. Chatterjee, K. Ghosal, M. Kumar, S. Mahmood and S. Thomas, J. Drug Delivery Sci. Technol., 2022, 104095 Search PubMed.
- S. Onoue, S. Yamada and H.-K. Chan, Int. J. Nanomed., 2014, 9, 1025 CrossRef CAS PubMed.
- L. B. Lopes, Pharmaceutics, 2014, 6, 52–77 CrossRef PubMed.
- S. B. Shirsand, M. S. Para, D. Nagendrakumar, K. M. Kanani and D. Keerthy, Int. J. Pharm. Investig., 2012, 2, 201 CrossRef CAS PubMed.
- R. Muzzalupo, L. Tavano, R. Cassano, S. Trombino, T. Ferrarelli and N. Picci, Eur. J. Pharm. Biopharm., 2011, 79, 28–35 CrossRef CAS PubMed.
- D. Aggarwal and I. P. Kaur, Int. J. Pharm., 2005, 290, 155–159 CrossRef CAS PubMed.
- N. Ravouru, P. Kondreddy and D. Korakanchi, Curr. Drug Discovery Technol., 2013, 10, 270–282 CrossRef CAS PubMed.
- J. Jiménez-López, I. Bravo-Caparrós, L. Cabeza, F. R. Nieto, R. Ortiz, G. Perazzoli, E. Fernández-Segura, F. J. Cañizares, J. M. Baeyens and C. Melguizo, Biomed. Pharmacother., 2021, 133, 111059 CrossRef PubMed.
- M. Walunj, S. Doppalapudi, U. Bulbake and W. Khan, J. Liposome Res., 2020, 30, 68–79 CrossRef CAS PubMed.
- M. L. Moyá, M. López-López, J. A. Lebrón, F. J. Ostos, D. Pérez, V. Camacho, I. Beck, V. Merino-Bohórquez, M. Camean and N. Madinabeitia, Pharmaceutics, 2019, 11, 69 CrossRef PubMed.
- S. Mahira, N. Kommineni, G. M. Husain and W. Khan, Biomed. Pharmacother., 2019, 110, 803–817 CrossRef CAS PubMed.
- M. Chang, M. Wu and H. Li, Drug Delivery, 2018, 25, 1984–1995 CrossRef CAS PubMed.
- A. Jose, S. Labala and V. V. K. Venuganti, J. Drug Targeting, 2017, 25, 330–341 CrossRef CAS PubMed.
- X. Gai, L. Cheng, T. Li, D. Liu, Y. Wang, T. Wang, W. Pan and X. Yang, AAPS PharmSciTech, 2018, 19, 700–709 CrossRef CAS PubMed.
- Z. Yang, L. Tian, J. Liu and G. Huang, J. Liposome Res., 2018, 28, 322–330 CrossRef CAS PubMed.
- A. Jafari, S. Daneshamouz, P. Ghasemiyeh and S. Mohammadi-Samani, J. Liposome Res., 2022, 1–19 Search PubMed.
- M. M. Maniyar, A. S. Deshmukh and S. J. Shelke, Asian J. Pharm. Res., 2022, 12, 225–228 Search PubMed.
- V. Dave, D. Kumar, S. Lewis and S. Paliwal, Int. J. Drug Delivery, 2010, 81–92 CrossRef CAS.
- S. Jain, N. Patel, P. Madan and S. Lin, Pharm. Dev. Technol., 2015, 20, 473–489 CrossRef CAS PubMed.
- T. Limsuwan and T. Amnuaikit, Procedia Chem., 2012, 4, 328–335 CrossRef CAS.
- Z. Hou, Y. Li, Y. Huang, C. Zhou, J. Lin, Y. Wang, F. Cui, S. Zhou, M. Jia and S. Ye, Mol. Pharm., 2013, 10, 90–101 CrossRef CAS PubMed.
- A. R. Sahu and S. B. Bothara, Int. J. Res. Med., 2015, 4, 94–99 CAS.
-
N. Karimi, B. Ghanbarzadeh, H. Hamishehkar, F. Keyvani, A. Pezeshki and M. M. Gholian.
- A. Riva, M. Ronchi, G. Petrangolini, S. Bosisio and P. Allegrini, Eur. J. Drug Metab. Pharmacokinet., 2019, 44, 169–177 CrossRef CAS PubMed.
- Š. Koudelka and J. Turánek, J. Controlled Release, 2012, 163, 322–334 CrossRef PubMed.
- K. V. Clemons, J. Capilla, R. A. Sobel, M. Martinez, A.-J. Tong and D. A. Stevens, Antimicrob. Agents Chemother., 2009, 53, 1858–1862 CrossRef CAS PubMed.
- A. K. Singh and S. S. Narsipur, J. Transplant., 2011, 480642 CAS.
- J. Pardeike, A. Hommoss and R. H. Müller, Int. J. Pharm., 2009, 366, 170–184 CrossRef CAS PubMed.
- T. Liu, Y. Lu, R. Zhan, W. Qian and G. Luo, Adv. Drug Delivery Rev., 2023, 193, 114670 CrossRef CAS PubMed.
- E. A. Madawi, A. R. Al Jayoush, M. Rawas-Qalaji, H. E. Thu, S. Khan, M. Sohail, A. Mahmood and Z. Hussain, Pharmaceutics, 2023, 15, 657 CrossRef CAS PubMed.
-
I. Theochari, A. Xenakis and V. Papadimitriou, in Smart Nanocontainers, Elsevier, 2020, pp. 315–341 Search PubMed.
- A.-M. Matei, C. Caruntu, M. Tampa, S. R. Georgescu, C. Matei, M. M. Constantin, T. V. Constantin, D. Calina, D. A. Ciubotaru and I. A. Badarau, Appl. Sci., 2021, 11, 4915 CrossRef CAS.
- B. Farasati Far, M. R. Naimi-Jamal, M. Sedaghat, A. Hoseini, N. Mohammadi and M. Bodaghi, J. Funct. Biomater., 2023, 14, 115 CrossRef CAS PubMed.
- M. Liu, X. Wei, Z. Zheng, Y. Li, M. Li, J. Lin and L. Yang, Int. J. Nanomed., 2023, 1537–1560 CrossRef PubMed.
-
A. A. Shaikh, J. B. Pawar, S. J. Anbhule and V. V. Kakade.
- S. Borkar, H. Yadav and A. Raizaday, Nasal drug delivery, 2023, 361–379 Search PubMed.
- M. Kumar, R. Dogra and U. K. Mandal, Curr. Drug Delivery, 2023, 20, 841–856 CrossRef CAS PubMed.
- M. Kumar, D. Kumar, S. Kumar, A. Kumar and U. K. Mandal, Curr. Pharm. Des., 2022, 28, 3212–3224 CrossRef CAS PubMed.
- D. Guimarães, A. Cavaco-Paulo and E. Nogueira, Int. J. Pharm., 2021, 601, 120571 CrossRef PubMed.
- M. Kumar, A. Thakur, U. K. Mandal, A. Thakur and A. Bhatia, AAPS PharmSciTech, 2022, 23, 244 CrossRef PubMed.
- S. S. Chrai, R. Murari and I. Ahmad, Biopharm, 2001, 14, 10 CAS.
- T. M. Allen and P. R. Cullis, Adv. Drug Delivery Rev., 2013, 65, 36–48 CrossRef CAS PubMed.
- L. Sercombe, T. Veerati, F. Moheimani, S. Y. Wu, A. K. Sood and S. Hua, Front. Pharmacol., 2015, 6, 286 Search PubMed.
- X. Huang, M. Li, R. Bruni, P. Messa and F. Cellesi, Int. J. Pharm., 2017, 524, 279–289 CrossRef CAS PubMed.
- B. S. Pattni, V. V. Chupin and V. P. Torchilin, Chem. Rev., 2015, 115, 10938–10966 CrossRef CAS PubMed.
- C. Zylberberg and S. Matosevic, Drug Delivery, 2016, 23, 3319–3329 CrossRef CAS PubMed.
- A. Bhatia, B. Singh, S. Bhushan and O. P. Katare, Drug Dev. Ind. Pharm., 2010, 36, 350–354 CrossRef CAS PubMed.
- A. Bhatia, B. Singh, K. Raza, A. Shukla, B. Amarji and O. P. Katare, J. Drug Targeting, 2012, 20, 544–550 CrossRef CAS PubMed.
- M. Rahman, V. Kumar, S. Beg, G. Sharma, O. P. Katare and F. Anwar, Artif. Cells, Nanomed., Biotechnol., 2016, 44, 1597–1608 CrossRef CAS PubMed.
- S. Saesoo, I. Sramala, A. Soottitantawat, T. Charinpanitkul and U. R. Ruktanonchai, J. Microencapsul., 2010, 27, 436–446 CrossRef CAS PubMed.
- D. Paolino, D. Cosco, F. Cilurzo, E. Trapasso, V. M. Morittu, C. Celia and M. Fresta, J. Controlled Release, 2012, 162, 143–151 CrossRef CAS PubMed.
- L. Feng, Y. Liu, Y. Chen, Q. Xiang, Y. Huang, Z. Liu, W. Xue and R. Guo, Adv. Healthcare Mater., 2024, 2203201 Search PubMed.
- C. Singh, S. Purohit, M. Singh and B. L. Pandey, J. Drug Delivery Res., 2013, 2, 18–27 Search PubMed.
- M. Saini and J. K. Malik, SAR J. Anat. Physiol., 2022, 3, 9–16 CrossRef.
- S. B. Sharma, S. Jain and K. Ganesan, J. Drug Delivery Ther., 2019, 9, 338–342 CrossRef CAS.
- R. Arshady, J. Controlled Release, 1990, 14, 111–131 CrossRef CAS.
- P. Blasi, J. Pharm. Investig., 2019, 49, 337–346 CrossRef CAS.
-
Y. W. Chien, Novel Drug Delivery Systems, CRC Press, Florida, US, 2nd edn, 1991 Search PubMed.
- B. S. Prasad, V. R. M. Gupta, N. Devanna and K. Jayasurya, J. Glob. Trends Pharm. Sci., 2014, 5, 1961–1972 CAS.
- C.-Z. Zhang, J. Niu, Y.-S. Chong, Y.-F. Huang, Y. Chu, S.-Y. Xie, Z.-H. Jiang and L.-H. Peng, Eur. J. Pharm. Biopharm., 2016, 109, 1–13 CrossRef CAS PubMed.
- T. G. Mason, J. N. Wilking, K. Meleson, C. B. Chang and S. M. Graves, J. Phys.: Condens. Matter, 2006, 18, R635 CrossRef CAS.
- O. Sonneville-Aubrun, J.-T. Simonnet and F. L′alloret, Adv. Colloid Interface Sci., 2004, 108, 145–149 CrossRef PubMed.
- K. Bouchemal, S. Briançon, E. Perrier and H. Fessi, Int. J. Pharm., 2004, 280, 241–251 CrossRef CAS PubMed.
- S. F. Tan, H. R. F. Masoumi, R. A. Karjiban, J. Stanslas, B. P. Kirby, M. Basri and H. Bin Basri, Ultrason. Sonochem., 2016, 29, 299–308 CrossRef CAS PubMed.
- H. Li, Q. Peng, Y. Guo, X. Wang and L. Zhang, Int. J. Nanomed., 2020, 15, 3123 CrossRef CAS PubMed.
- M. Hanifah and M. Jufri, J. Young Pharm., 2018, 10, 404 CrossRef CAS.
- V. J. Mohanraj and Y. Chen, Trop. J. Pharm. Res., 2006, 5, 561–573 Search PubMed.
-
S. Bhatia, in Natural polymer drug delivery systems, Springer, 2016, pp. 33–93 Search PubMed.
- M. Dadwal, D. Solan and H. Pradesh, J. Adv. Pharm. Educ. Res., 2010, 381–387 Search PubMed.
- V. B. Kadam, K. B. Dhanawade, V. A. Salunkhe and A. Ubale, J. Curr. Pharm. Res., 2014, 4, 1318 CrossRef.
- M. Kumar and U. K. Mandal, Drug Delivery Lett., 2021, 11, 195–202 CrossRef CAS.
- P. B. R. da Rocha, B. dos Santos Souza, L. M. Andrade, J. L. V. dos Anjos, S. A. Mendanha, A. Alonso, R. N. Marreto and S. F. Taveira, J. Drug Delivery Sci. Technol., 2019, 50, 305–312 CrossRef.
- M. C. Kwon, W. Y. Choi, Y. C. Seo, J. S. Kim, C. S. Yoon, H. W. Lim, H. S. Kim, J. hee Ahn and H. Y. Lee, J. Biotechnol., 2012, 157, 100–106 CrossRef CAS PubMed.
- S. Liu, Y. Zhao, M. Li, L. Nie, Q. Wei, O. V. Okoro, H. Jafari, S. Wang, J. Deng and J. Chen, Chem. Eng. J., 2023, 143016 CrossRef CAS.
- S. Narisepalli, S. A. Salunkhe, D. Chitkara and A. Mittal, Int. J. Pharm., 2023, 631, 122508 CrossRef CAS PubMed.
- R. M. Handjani-Vila, A. Ribier, B. Rondot and G. Vanlerberghie, Int. J. Cosmet. Sci., 1979, 1, 303–314 CrossRef CAS PubMed.
- J. Kemps and D. A. Crommelin, Pharm. Weekbl, 1988, 123, 355–363 CAS.
- M. Malhotra and N. K. Jain, Indian Drugs, 1994, 31, 81 CAS.
- P. Wichayapreechar, S. Anuchapreeda, R. Phongpradist, W. Rungseevijitprapa and C. Ampasavate, J. Liposome Res., 2020, 30, 197–207 CrossRef CAS PubMed.
- D.-W. Kim, M.-H. Cho, S.-Y. Park, J.-H. Lee, G.-W. Lee, M.-S. Park, J.-K. Park and U.-K. Jee, J. Pharm. Investig., 2002, 32, 291–297 CrossRef CAS.
- M. Kumar, Y. Ge, A. R. Hilles, A. Bhatia and S. Mahmood, Int. J. Biol. Macromol., 2023, 123696 CrossRef CAS PubMed.
- Z. Ma, M. Kotaki, R. Inai and S. Ramakrishna, Tissue Eng., 2005, 11, 101–109 CrossRef CAS PubMed.
- M. Alavi and A. Nokhodchi, Cellulose, 2022, 1–17 Search PubMed.
- M. Momin, Z. Patel, S. Gharat, M. Altabakha, A. Ashames and S. Boddu, Crit. Rev. Ther. Drug Carr. Syst., 2022, 83–118 Search PubMed.
-
Z. Aytac and T. Uyar, Appl. Polym. Nanofibers, 2022, pp. 202–254 Search PubMed.
- C. Gao, L. Zhang, J. Wang, M. Jin, Q. Tang, Z. Chen, Y. Cheng, R. Yang and G. Zhao, J. Mater. Chem. B, 2021, 9, 3106–3130 RSC.
- L. Kumar, S. Verma, K. Joshi, P. Utreja and S. Sharma, Future J. Pharm. Sci., 2021, 7, 1–17 CrossRef.
- O. Suwantong, U. Ruktanonchai and P. Supaphol, Polymer, 2008, 49, 4239–4247 CrossRef CAS.
- O. Suwantong, U. Ruktanonchai and P. Supaphol, J. Biomed. Mater. Res., Part A, 2010, 94, 1216–1225 CrossRef PubMed.
- L. Zhu, X. Liu, L. Du and Y. Jin, Biomed. Pharmacother., 2016, 83, 33–40 CrossRef CAS PubMed.
- S. Anand, P. S. Rajinikanth, D. K. Arya, P. Pandey, R. K. Gupta, R. Sankhwar and K. Chidambaram, Pharmaceutics, 2022, 14, 273 CrossRef CAS PubMed.
- L. Liu, Z. Ding, Y. Yang, Z. Zhang, Q. Lu and D. L. Kaplan, Biomater. Sci., 2021, 9, 5227–5236 RSC.
-
J. T. James, Determination and manipulation of biologically active triterpenoid secondary metabolites in Centella asiatica, University of Johannesburg, South Africa, 2013 Search PubMed.
- W. Han, L. Wang, J. Sun, Y. Shi, S. Cui, D. Yang, J. Nie and G. Ma, ACS Appl. Bio Mater., 2013, 10418–10422 Search PubMed.
- N. Raval, P. Barai, N. Acharya and S. Acharya, Artif. Cells, Nanomed., Biotechnol., 2018, 46, 832–846 CrossRef PubMed.
-
B. Pamornpathomkul, W. Rangsimawong, T. Rojanarata, P. Opanasopit, C. Chaiyodsilp and T. Ngawhirunpat, in MATEC Web of Conferences, EDP Sciences, 2018, vol. 192, p. 1016.
- K. Witkowska, M. Paczkowska-Walendowska, T. Plech, D. Szymanowska, B. Michniak-Kohn and J. Cielecka-Piontek, Int. J. Mol. Sci., 2023, 24, 17229 CrossRef CAS PubMed.
- A. G. M. Perez, J. M. Machado, K. C. Manhani, P. Leo, P. Noriega and M. H. A. Zanin, SN Appl. Sci., 2020, 2, 1–12 Search PubMed.
- P. Wang, Y. Wang, Y. Yi, Y. Gong, H. Ji, Y. Gan, F. Xie, J. Fan and X. Wang, J. Nanobiotechnol., 2022, 20, 259 CrossRef CAS PubMed.
- S. A. T. Opatha, R. Chutoprapat, P. Khankaew, V. Titapiwatanakun, W. Ruksiriwanich and K. Boonpisuttinant, Int. J. Pharm., 2023, 123738 Search PubMed.
- Y. R. Wu, H. J. Choi, Y. G. Kang, J. K. Kim and J.-W. Shin, Int. J. Nanomed., 2017, 7007–7013 CrossRef CAS PubMed.
- X. Chen, Y. Zhang, P. Zhao, Y. Chen, Y. Zhou, S. Wang and L. Yin, Drug Dev. Ind. Pharm., 2020, 46, 57–69 CrossRef CAS PubMed.
- N. Namviriyachote, V. Lipipun, Y. Akkhawattanangkul, P. Charoonrut and G. C. Ritthidej, Asian J. Pharm. Sci., 2019, 14, 63–77 CrossRef PubMed.
- R. Kunjumon, G. Viswanathan, D. V. Jayasree, P. G. Biju, P. Prakash, B. C. P. Sasidharan and S. Baby, Can. J. Chem., 2022, 100, 396–404 CrossRef CAS.
- A. G. Bertollo, M. E. D. Mingoti, J. Medeiros, G. B. da Silva, G. T. Capoani, H. Lindemann, J. V. Cassol, D. Manica, T. Oliveira and M. L. Garcez, Pharmacol. Res. - Mod. Chin., 2022, 100032 CrossRef.
- M. Oboh, L. Govender, M. Siwela and B. N. Mkhwanazi, Molecules, 2021, 26, 7243 CrossRef CAS PubMed.
- K. Wu, G. Yao, X. Shi, H. Zhang, Q. Zhu, X. Liu, G. Lu, L. Hu, W. Gong and Q. Yang, Mol. Immunol., 2021, 130, 122–132 CrossRef CAS PubMed.
- X. Liang, Y. N. Huang, S. W. Chen, W. J. Wang, N. Xu, S. Cui, X. H. Liu, H. Zhang, Y. N. Liu and S. Liu, Pharmacol. Biochem. Behav., 2008, 89, 444–449 CrossRef CAS PubMed.
- L. Luo, X.-L. Liu, R.-H. Mu, Y.-J. Wu, B.-B. Liu, D. Geng, Q. Liu and L.-T. Yi, Brain Res. Bull., 2015, 114, 62–69 CrossRef CAS PubMed.
- R. K. Verma, K. G. Bhartariya, M. M. Gupta and S. Kumar, Phytochem. Anal., 1999, 10, 191–193 CrossRef CAS.
- T. Hou, X. Li and C. Peng, Neurosci. Lett., 2017, 646, 56–61 CrossRef CAS PubMed.
- Y. Zhang, X. Meng and K. Liu, J. Bioenerg. Biomembr., 2022, 54, 9–16 CrossRef CAS PubMed.
- E. Fitrianda, E. Y. Sukandar, E. Elfahmi and I. K. Adnyana, Asian J. Pharm. Clin. Res., 2017, 10, 268–272 CrossRef CAS.
-
A. E. Nugroho, N. Y. Lindawati, K. Herlyanti, L. Widyastuti and S. Pramono.
- J. S. Guo, C. L. Cheng and M. W. L. Koo, Planta Med., 2004, 70, 1150–1154 CrossRef CAS PubMed.
- N. X. Nhiem, B. H. Tai, T. H. Quang, P. Van Kiem, C. Van Minh, N. H. Nam, J.-H. Kim, L.-R. Im, Y.-M. Lee and Y. H. Kim, Bioorg. Med. Chem. Lett., 2011, 21, 1777–1781 CrossRef PubMed.
- N. Nowwarote, T. Osathanon, P. Jitjaturunt, S. Manopattanasoontorn and P. Pavasant, Phyther. Res., 2013, 27, 457–462 CrossRef CAS PubMed.
- J. Wan, X. Gong, R. Jiang, Z. Zhang and L. Zhang, Phyther. Res., 2013, 27, 1136–1142 CrossRef CAS PubMed.
- Y. He, X. Peng, L. Zheng, Y. Tang, J. Li and X. Huang, J. Gastrointest. Oncol., 2021, 12, 196 CrossRef PubMed.
- S. C. Funes, M. Rios, J. Escobar-Vera and A. M. Kalergis, Immunology, 2018, 154, 186–195 CrossRef CAS PubMed.
- J. Huang, X. Zhou, Y. Shen, H. Li, G. Zhou, W. Zhang, Y. Zhang and W. Liu, J. Biomed. Mater. Res., Part A, 2020, 108, 69–80 CrossRef CAS PubMed.
- L. S. Wei, N. Musa, C. T. Sengm, W. Wee and N. A. M. Shazili, Afr. J. Biotechnol., 2008, 7, 2275–2278 CAS.
- G. K. Sankar, K. Ramamoorthy, K. Sakkaravarthi and A. Elavarsi, Der Pharm. Sin., 2010, 1, 17–22 CAS.
- M. Ramanathan, S. Sivakumar, P. R. Anandvijayakumar, C. Saravanababu and P. R. Pandian, Indian J. Exp. Biol., 2007, 45, 425–431 CAS.
- G. K. Shinomol, Neurotoxicology, 2008, 29, 948–957 CrossRef CAS PubMed.
- G. K. Shinomol and H. Ravikumar, Phyther. Res., 2010, 24, 885–892 CrossRef PubMed.
- N. Dhas, H. S. Preetha, A. Dubey, G. Ravi, I. Govindan, A. Rama, A. Naha and S. Hebbar, J. Appl. Pharm. Sci., 2022, 12, 71–81 CAS.
- R. Kunjumon, G. Viswanathan and S. Baby, Brain Behav. Immun. Integr., 2024, 100043 CrossRef.
- A. Roy and N. Bharadvaja, Curr. Trends Biomed. Eng. Biosci., 2017, 5, 1–5 Search PubMed.
-
M. A. Barkat, Harshita, S. S. Das, S. Beg and F. J. Ahmad, Nanophytomedicine Concept to Clin, 2020, pp. 1–17 Search PubMed.
-
S. Ghosh, B. Sarkar, C. S. Ranadheera and S. Thongmee, in Nanotechnology and In Silico Tools, Elsevier, 2024, pp. 75–87 Search PubMed.
- F. Ebau, A. Scano, M. L. Manca, M. Manconi, V. Cabras, M. Pilloni and G. Ennas, J. Biomed. Mater. Res., Part A, 2023, 111, 300–308 CrossRef CAS PubMed.
- N. A. Jusril, S. I. Abu Bakar, K. A. Khalil, W. M. Md Saad, N. K. Wen and M. I. Adenan, Evidence-Based Complement. Altern. Med., 2022, 1–18 Search PubMed.
- M.-S. Huang, P. Chanapongpisa, P. Yasurin, K. Kitsubthawee, J. Phetsom and I. Lindayani, Appl. Sci. Eng. Prog., 2020, 13, 11–18 Search PubMed.
- J. Huang, Y. Gong, K. Liu, J. Chen and X. Zhou, Explor. Res. Hypothesis Med., 2023, 8, 319–337 CrossRef.
|
This journal is © The Royal Society of Chemistry 2024 |
Click here to see how this site uses Cookies. View our privacy policy here.