A chloride-responsive molecular switch: driving ion transport and empowering antibacterial properties†
Received
8th November 2023
, Accepted 21st November 2023
First published on 24th November 2023
Abstract
A molecular switch was developed to recognize and transport Cl− across lipid bilayers. The XRD-crystal structure and NOESY NMR spectra of a potent 4-aminoquinazoline analogue confirmed Cl−-induced conformation changes. Systematic biophysical studies revealed that the quinazoline moiety forms cooperative interactions of H+ and Cl− ions with the thiourea moiety, resulting in the transport of H+/Cl− across the membranes. A pH-dependent analysis revealed that the transport of Cl− by the potent compound increased in an acidic environment. The potent compound could also transport H+/Cl− across Gram-positive bacteria, leading to antibacterial activities.
Introduction
Dysfunction of natural ion channels present in the membranes of all cells and many cellular organs has been identified as being directly or indirectly associated with several diseases, including cancer and cystic fibrosis. Hence, restoring the influx and efflux of ions across defective cellular membranes through synthetic ion transporters to fight against channelopathies has become an important subject of medical research. Recent studies validated that the transmembrane transport of Cl− by synthetic ion transporters in whole or in part could also induce antibacterial activities.1 Such synthetic ion transporters are of great interest in combating drug-resistant bacterial strains, given that over 80% of hospital-acquired infections are becoming increasingly resistant to commercially available antibiotics. Hence, the development of new approaches or chemical entities for combating infections caused by ESKAPE pathogens is the need of the hour.2–5
Membrane-directing molecules with ion transport properties are considered a novel class of antibacterial agents. These membrane-directing lipophilic molecules provide the additional advantage of minimizing drug resistance against the majority of bacterial strains. Furthermore, these synthetic ion carriers are structurally much simpler than protein-based natural ion carriers; hence their transport behaviour can easily be scrutinized. Lately, a wide range of synthetic ion transporters have been developed. However, developing stimuli-responsive synthetic ion transporters with properties similar to those of natural ones remains quite challenging. In this regard, several stimuli-responsive (such as pH, enzyme, redox and light) synthetic ion transporters have been developed to have regulated ion transport properties.5–11 However, little effort has been made in utilizing biologically active moieties for obtaining stimuli-responsive ion transport properties. This approach would provide compounds with stimuli-responsive antibacterial, anticancer, or other therapeutic activities due to the presence of biologically active moieties and additional ion recognition and transport moieties.
So far, a few ion transporters, such as gramicidin and monensin, have demonstrated antibacterial activities owing to their cation-selective membrane permeability.3 However, the antibacterial activities of transmembrane anion transporters continue to be less explored.3,12,13 Earlier, Cl− transport and antibacterial activities were reported for TREN-based tris(thiourea)12 and benzimidazolium-based13 compounds. Recently, imine-based indolyl analogues have demonstrated Cl− transport and antibacterial activities. However, the stability of these imine-based compounds could be an issue for their Cl− transport activities.3 Continuing our effort in designing and exploring the biological activities of synthetic ion transporters, we describe the transmembrane H+/Cl− cotransport and antimicrobial activities of 4-aminoquinazoline-based compounds against Gram-positive and Gram-negative bacterial strains. Recently, several thiourea-based Cl− transporters have been reported.6,14,15 However, the anion recognition and transport efficacy of 4-aminoquinazoline along with the thiourea moiety has never been explored. The 4-aminoquinazoline moiety could provide multivalent NH bond interactions within the binding cavity to design a new class of thiourea-based anion carriers with pH-responsivity. Quinazole derivatives have been found to exhibit antibacterial, anticancer, antituberculosis, and other activities.16 We hypothesize that the potent molecule would embrace an open or closed conformation in the absence and presence of an anion, respectively, leading to the formation of an anion-induced molecular switch that could recognize and transport anions across the membrane.4,17 Therefore the anion itself would act as the stimulus, minimizing the need for an additional stimulus to regulate anion transport, and the presence of a free amine group would offer additional pH-responsive anion transport properties in an acidic environment. To our knowledge, 4-aminoquinazoline analogues with anion-recognition and transport properties have never been explored as pH-responsive Cl− transporters. Hence, we present a dynamic and adaptable system for anion recognition and transmembrane transport.
Results and discussion
Design and synthesis of 4-aminoquinazoline analogues
The reaction of anthranilonitrile with sodium hydride provided 2-(2-aminophenyl)quinazolin-4-amine, which upon condensation with various arylisothiocyanates provided the targeted 4-aminoquinazoline thiourea derivatives (1a–1f and 3a–3b) (Scheme 1).18 We hypothesized that the introduction of the thiourea motif into 2-(2-aminophenyl)quinazoline-4-amine would help the quinazoline base scaffold assist in the transmembrane transport of ions. The strongly electron-withdrawing groups, such as –CF3, at the phenyl groups were varied to increase the lipophilicity (log
P) and acidity (pKa) of the N–H proton, which is beneficial for improved ion binding aptitude, membrane permeability, and ion transport efficiency. For the same reason, benzoyl and tosyl moieties were also installed in 2-(2-aminophenyl)quinazolin-4-amine (2a and 2b). The protonation of the nitrogen atom of quinazoline could additionally play a pivotal role in enhanced ion recognition, and the pH of the surrounding medium can modulate ion binding affinity. Compounds 3a and 3b were also prepared to examine the significance of the bis(iminourea) moiety. Additional control compounds 4 and 5 were synthesized to investigate the role of the 4-aminoquinazoline moiety in ion transport efficacy (Scheme 1).
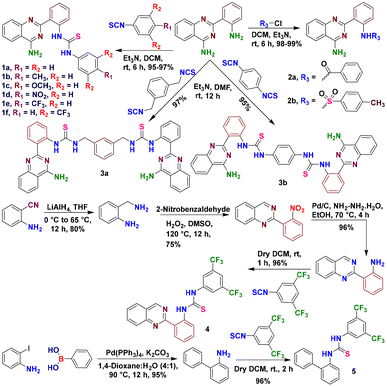 |
| Scheme 1 Synthetic routes to 4-aminoquinazoline analogs. | |
Solid-state crystal structure analysis
Slow evaporation of a solution of 1f in an acetonitrile/methanol (1
:
1) mixture provided a single orange crystal. Tetrabutylammonium chloride (TBACl) was used as a Cl− source for co-crystallization with 1f in the presence of DMSO solvent. The X-ray solid-state structures of 1f revealed that the amine and thiourea moieties adopt an anti-conformation. After complexation with Cl−, the thiourea groups adopt a syn-conformation with respect to the amine group, which could be due to the strong intramolecular hydrogen bonding between the thiourea–N–H and the NH2 protons with Cl− (Fig. 1A). The crystallographic data revealed that the N4–H⋯N hydrogen bond distance increases from 1.905 Å to 2.11 Å and the torsional angle of C14–C9–C8–N2 decreases from 179.28 to 25.64° (i.e., ±153.64° rotation of the 4-aminoquinazoline moiety), and also the torsional angle of C9–C14–N4–H increases from 14.98 to 38.45° (i.e., ±23.47° rotation of the H atom), and N4–C15–N5–H changes from 162.95 to 9.61° (i.e., ±153.34° rotation of the H atom and aryl ring) after Cl− binding (Fig. S1 and S2†).
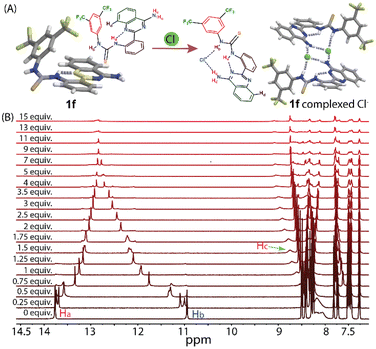 |
| Fig. 1 XRD-crystal structures of 1f and 1f complexed with Cl− (A). The partially stacked plot of 1H NMR titration experiment of 1f (10 mM) with successive addition of TBACl in DMSO-d6 solvent (B). | |
Anion binding studies
1H NMR titration of 1f in DMSO-d6 solvent was performed with different concentrations of TBACl to investigate their interaction pattern in the solution phase (Fig. 1B). A significant chemical shift of N–Ha and N–Hb protons of the thiourea groups and free amine NH2 groups suggests the interaction of the compound with Cl−via hydrogen bonding. The polarization of N–Hb and N–Hc protons could be prompted by the through-space effect due to the presence of Cl−. This interaction is generally electrostatic and generates a partial positive charge on the proton, resulting in a deshielding effect leading to a downfield shift.19 Interestingly, the N–Ha proton of 1f showed an upfield chemical shift. The through-bond propagation could enhance the electron density in the aryl moiety in the presence of Cl−, resulting in a shielding effect leading to an upfield shift.20 Hence, these changes in the chemical shifts of the N–H protons could be due to structural switching upon Cl− coordination. The binding analysis of the concentration-dependent chemical shifts revealed the formation of a 1
:
1 host–guest interaction-based complex with a binding affinity of 159.74 ± 7.81 M−1, suggesting the Cl− recognition aptitude of 1f in the solution (Fig. S3†). Similarly, the association constant was measured in CD3CN solvent. It was found to be 258.87 ± 0.1075 M−1 (Fig. S4†). The difference in Ka values of 1f could be due to the competition of DMSO with TBACl in Cl− ion recognition. A similar change in the chemical shifts of the N–H protons in CD3CN suggests that the structural switching occurs upon Cl− coordination, and not due to the solvent effect (interaction of DMSO with 1f). The 1H NMR titration of 1f with TBAF showed a strong binding affinity of 4323.64 ± 98.2 M−1 for F− (Fig. S4†). In comparison, the 1H NMR titration of 1f with TBABr, TBAI, and TBANO3 showed a very low binding affinity for Br−, I−, and NO3− (Fig. S6 and S7†).
Conformational analysis
Further conformational analysis of 1f in the presence of Cl− by 1H–1H NOESY NMR revealed the interaction of the Ha proton with the Hb proton and that of the Hc proton with the Hd proton (Fig. 2A and B). However, these interactions were not observed when NOESY NMR data of only 1f were recorded in DMSO-d6. Hence, the interactions of 1f in the presence of Cl− could be the consequence of the conformational alterations across the phenyl–quinazoline bond, indicating that 1f remains in dynamic equilibrium with open and closed conformations, but it folds into a closed conformation upon binding with Cl−. A photographic image of 1f in DMSO solvent under UV-Vis light (365 nm) showed an enhancement of fluorescence intensity with Cl− concentration (Fig. 2C), suggesting that Cl− binding could restrict the access to the conical intersection to produce a fluorescence enhancement.21 Concentration-dependent absorption studies did not show any significant alternation in the spectral properties of 1f in the presence of TBACl (0–42 mM) (Fig. S9A†). In contrast, concentration-dependent fluorescence studies showed a significant (over 4.2-fold) enhancement in fluorescence intensity of 1f (λex = 290/350 nm, λem = 420 nm) in the presence of TBACl (0–42 mM) (Fig. 2D and S9†). This augmentation of fluorescence intensity of 1f in the presence of TBACl could be due to the hydrogen-bonding interaction with Cl−, which enhances the electron density of the NH fragment, reinforcing the electrical dipole on the aromatic moieties of 1f. Hence, the charge-transfer transition is enhanced with the addition of Cl−.
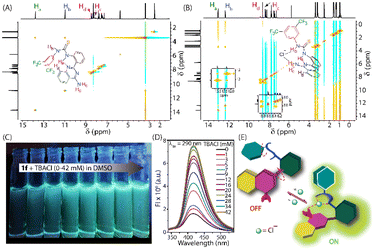 |
| Fig. 2 Partial 1H–1H NOESY NMR spectra of 1f (10.0 mM) (A) and 1f with TBACl (B) in DMSO-d6 solvent. Image of 1f in the presence of different concentrations of TBACl in DMSO solvent under UV-Vis (365 nm) light (C). Change in the fluorescence intensity (λex = 290 nm) of 1f in the presence of different concentrations of TBACl in DMSO solvent (D). Schematic diagram of the conformational change of 1f in the presence of Cl− (E). | |
Anion transportation across lipid bilayers
The transmembrane ion transport properties of these 4-aminoquinazoline derivatives were investigated using large unilamellar vesicles (LUVs). The vesicles were prepared using dipalmitoylphosphatidylcholine (DPPC), 1-palmitoyl-2-oleoyl-sn-glycero-3-phospho-L-serine (POPS), and cholesterol (CHOL) lipids with a molar ratio of 6
:
2
:
2 by entrapping lucigenin dye in the presence of 100 mM NaNO3 in 20 mM HEPES buffer at pH 7.2 and pH 5.4.22 In an extravesicular medium, an isotonic solution of NaCl (100 mM), 20 mM HEPES was used. A compound pulse was given to initiate the transport of Cl−, and the fluorescence intensity of lucigenin was monitored. The initial screening revealed that all 4-aminoquinazoline derivatives (20 μM at pH 7.2) proficiently enhanced the Cl− influx rate, indicating their transmembrane transport aptitude. It also revealed that 1f has the highest Cl− transport efficacy among the tested compounds (Fig. 3A).
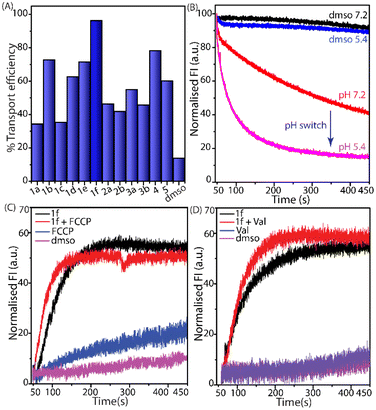 |
| Fig. 3 Assessment of the Cl− transport activity of the synthesized compounds (A). The pH-dependent Cl− transport activity of 1f (B). The Cl− transport activity of 1f in the absence and presence of FCCP (C) and valinomycin (Val) (D). | |
The presence of a free amino group suggests that the protonation of the 4-aminoquinazoline moiety could significantly affect the anion-binding properties of the compounds. Hence, lucigenin-based Cl− transport studies were performed at pH 5.4 and 7.2 (Fig. 3B). The outcome of this assay showed that 1f has a superior Cl− transport aptitude in an acidic medium (pH 5.4) compared to that under physiological conditions. The calculated EC50 values of the tested 1f were 1.63 and 0.22 μM at pH 7.2 and 5.4, respectively (Fig. S13 and S14†). Compound 1f showed a 7.41-fold augmentation in Cl− transport aptitude at pH 5.4 compared to that at pH 7.2, signifying pH-dependent Cl− transport properties. The degree of protonation of the quinazoline moiety would be much higher in an acidic medium than under physiological conditions, resulting in a higher Cl− transport ability.22 A chloride ion selective electrode (Cl-ISE)-based transport assay also showed the Cl− transport aptitude of 1f (Fig. S17†).
A HPTS assay was used to investigate the effects of extravesicular anions on the Cl− transport activities of 1f.22–24 In this assay, NaCl-containing buffer was used as the intravesicular solution, but isosmotic buffer solutions of different NaX salts (X = F−, Cl−, Br−, I−, NO3−, ClO4−, and SCN−) were utilized as the extravesicular solution (Fig. S18†). Interestingly, significant differences in the anion transport properties were observed, with the highest transport ability for Cl− among the other tested anions. Although 1f showed stronger binding affinity for F− over Cl−, its transport efficacy was much lower for F− over Cl−, suggesting that the very strong binding affinity restricted its releasing efficacy. A preferable transporter should have comparable binding and releasing affinity. This selective anion transport ability of 1f could be due to its comparable recognition and release properties of Cl− through the cavity of the thiourea and 4-aminoquinazoline scaffolds. Similarly, a lucigenin assay was used to investigate the effects of extravesicular cations on the Cl− transport activities of 1f. Isosmotic buffer solutions of various monovalent metal chloride salts (Li+, Na+, K+, Rb+, and Cs+) in an extravesicular solution were utilized to investigate the role of various cations in the Cl− transport efficiency of the compounds (Fig. S19†).8,22,23,25 Hence, the tested cations might have a minor effect on the transmembrane Cl− transport activities of 1f.
Transport mechanism and pathway
The transmembrane transport of Cl− by 1f could follow either a symport (H+/A−) or an antiport (OH−/A−) mechanism to maintain the overall charge neutrality. In this regard, we performed HPTS assays in the absence and presence of 4-(trifluoromethoxy)phenylhydrazone (FCCP; a protonophore). The compound showed a similar Cl− transport efficacy of 1f both in the absence and presence of FCCP (Fig. 3C). This observation suggests that the rate of dissipation of the pH gradient was not affected by FCCP. Therefore, the association of Cl− is the rate-limiting step of the H+/Cl− symport process. In addition, a HPTS-based valinomycin (a potassium ionophore) assay was performed at pH 7.2. Simultaneous OH− influx and/or Cl− efflux is anticipated to uphold the charge neutrality during the influx of K+ by valinomycin. On the other hand, the similar transport rates in the presence and absence of valinomycin indicate higher transport efficacy for Cl− over OH− (Fig. 3D). Therefore, both FCCP and valinomycin assays indicate the cotransport of H+/Cl− by the compound under physiological conditions. A U-tube-based ion transport measurement was performed to investigate the evidence of the cotransport of H+/Cl− by 1f in an acidic medium. The left and right sides of the U-tube contain 0.1 M aqueous HCl solution (pH 1.2) and isotonic NaNO3 solution, respectively. Chloroform (mimic of the lipid bilayer) separated these two aqueous solutions. The Cl− and H+ transport was recorded using Cl-ISE and a pH meter (Fig. S20†). The continuous increase in the Cl− concentration and steady diminution in the pH value directly established the cotransport of H+/Cl− by the compound.
A temperature-dependent Cl− transport assay was performed to distinguish whether the compound follows a carrier or channel mechanism. A sharp increase in the Cl− transport efficiencies of 1f was observed at 45 °C compared to that at 25 °C, suggesting that the Cl− transport activity of 1f is directly associated with the fluidity of the membrane (Fig. S21 and S22†). The U-tube experiment also confirmed the transport of Cl− across the lipid bilayer mimic (chloroform layer). Therefore, 1f transports Cl−via a carrier pathway (Fig. S20†). Meanwhile, the non-leakage of carboxyfluorescein from the vesicles in the absence and presence of the compound supports retaining of vesicle integrity during the measurements (Fig. S23†).
The higher transmembrane Cl− transport efficacy in an acidic medium (pH 5.4) than that in physiological media (pH 7.2) suggests that the Cl− transport aptitude of 1f is enhanced in the acidic medium.22 The presence of the CF3 moiety in the benzene ring could make the neighboring nitrogen atoms more electron deficient, resulting in the N–H proton of the aryl ring being more electron deficient. This increase in the N–H proton acidity could provide a much stronger interaction with Cl−. The CF3 moiety also provides extra hydrophobicity to the compound, which would contribute to the higher Cl− transport of 1f. Additional studies showed that the ‘apparent pKa’ value of 1f was 6.8 (Fig. S24†), indicating that the pH of the surrounding media could be instrumental in Cl− transport activity.22
Antibacterial activities
The potent Cl− transporter 1f was tested for its antibacterial activity against Gram-positive and Gram-negative bacterial strains to investigate its biological applicability.5,26 Compound 1f exhibited strong antibacterial activities against Staphylococcus aureus (S. aureus; MIC value of 2.34 ± 0.39 μM) and methicillin-resistant S. aureus (MRSA; MIC value of 2.50 ± 0.50 μM) (Table S2 and Fig. S25–S27†). The minimal bactericidal concentration (MBC) calculations revealed that 1f showed a bactericidal effect at a concentration as low as the MIC (Fig. S25†). However, 1f failed to exhibit any significant activity against E. coli. This may be because Gram-negative bacteria have an extra outer membrane shielding them from such compounds (Table S2†). Compounds 1a–f, 2a, 2b, 4, 5, and 2-(2-aminophenyl)quinazolin-4-amine were also tested against S. aureus cells. It should be noted that only compounds 1e and 1f showed antibacterial activities, with 1f being a better antibacterial compound. These data correlate with the Cl− transport activity of the mentioned compounds (Fig. 3A). 1f showed a bactericidal effect at a concentration as low as the MIC. Thus, it can be inferred that the compound is highly potent and is able to kill Gram-positive bacteria at a very low dose. This finding is very encouraging as it suggests that this chemical entity has promising potential in the treatment of bacterial infection, primarily caused by drug-resistant strains. The efficacy of 1f in achieving a bactericidal effect demonstrates its robust and precise mode of action against bacteria and indicates its potential significance in altering essential physiological and biological pathways necessary for the survival of the organism. Moreover, the ability of 1f to transport Cl− ions across membranes suggests its potential role in disrupting Cl− homeostasis, potentially resulting in bacterial cell death. The FESEM images revealed that there was a significant deformation in the S. aureus cells after the treatment with 1f (Fig. S28†). The H+/Cl− cotransport property of 1f across the model membranes suggests that the transport of H+ across the bacterial membrane could induce changes in bacterial membrane pH and accumulation of Cl− inside the bacterial cells, together leading to bacterial cell damage. The S. aureus cells were also treated with MQAE dye, a cell-permeable fluorescence indicator of Cl−.25 The addition of 1f resulted in a time-dependent decrease in the fluorescence intensity of MQAE dye, suggesting that the 1f-mediated Cl− transport activity could modify the biological processes that rely on Cl− cofactors, potentially resulting in bacterial cell death (Fig. S29†). Additional studies with 4-aminoquinazoline, control compounds 4 and 5, showed a much lower antibacterial activity against S. aureus cells, suggesting that the bacterial damage was induced by the Cl− transport activity of 1f.
Conclusions
Herein, we established that the potent 4-aminoquinazoline analog selectively transports Cl− across the lipid bilayers via a carrier pathway. The solid-state crystal structure, NMR, and fluorescence studies validated the Cl− binding mediated conformational change of 1f. The outcomes also revealed that the quinazoline moiety has cooperative interactions of H+ and Cl− ions with the thiourea moiety, resulting in the transport of H+/Cl− across the membranes. A pH-dependent analysis revealed that the transport of Cl− by the potent compound increased in an acidic environment. We hypothesize that such an anion-induced molecular switch is of great interest because of its dynamic and adaptable properties for anion recognition and transmembrane transport. Fluorescence studies showing conformational fluctuations in response to Cl− binding could be explored for biomedical imaging. Such pH-dependent selective Cl− transporters could also be useful in designing novel pharmaceutical agents and exploring ion transport-mediated biological activities.
Author contributions
D. M. conceived the project. S. D. carried out the synthesis and ion transport activity studies. M. K. and S. S. assisted in ion transport activity studies. R. K. performed antibacterial studies. S. D. and D. M. wrote the manuscript.
Conflicts of interest
There are no conflicts to declare.
Acknowledgements
The authors acknowledge the Science & Engineering Research Board, Govt. of India (CRG/2021/000306), for financial support. The authors also are thankful to the Department of Science and Technology, Government of India (SR/FST/CS-II/2017/23C), the Central Instrument Facility, and the Department of Chemistry, Centre for the Environment, Indian Institute of Technology Guwahati, for the instrument facility.
Notes and references
- J. Yang, G. C. Yu, J. L. Sessler, I. Shin, P. A. Gale and F. H. Huang, Chem, 2021, 7, 3256–3291 CAS.
- G. Zilahi, A. Artigas and I. Martin-Loeches, Ann. Intensive Care, 2016, 6, 96 CrossRef PubMed.
- I. Carreira-Barral, C. Rumbo, M. Mielczarek, D. Alonso-Carrillo, E. Herran, M. Pastor, A. Del Pozo, M. Garcia-Valverde and R. Quesada, Chem. Commun., 2019, 55, 10080–10083 RSC.
- J. V. Gavette, C. J. Evoniuk, L. N. Zakharov, M. E. Carnes, M. M. Haley and D. W. Johnson, Chem. Sci., 2014, 5, 2899–2905 RSC.
- S. Dey, A. Patel, N. Haloi, S. Srimayee, S. Paul, G. K. Barik, N. Akhtar, D. Shaw, G. Hazarika, B. M. Prusty, M. Kumar, M. K. Santra, E. Tajkhorshid, S. Bhattacharjee and D. Manna, J. Med. Chem., 2023, 66, 11078–11093 CrossRef CAS PubMed.
- N. Akhtar, O. Biswas and D. Manna, Chem. Commun., 2020, 56, 14137–14153 RSC.
- A. Roy and P. Talukdar, ChemBioChem, 2021, 22, 2925–2940 CrossRef CAS PubMed.
- S. Srimayee, S. R. Badajena, N. Akhtar, M. K. Kar, S. Dey, P. Mohapatra and D. Manna, Chem. Commun., 2023, 59, 12759–12762 RSC.
- B. Prusty, R. Karn, A. Patel, P. Mazumder, S. Kumar and D. Manna, Chem. Commun., 2023, 59, 10624–10627 RSC.
- A. Patel, S. Dey, K. Shokeen, T. M. Karpiński, S. Sivaprakasam, S. Kumar and D. Manna, RSC Med. Chem., 2021, 12, 1005–1015 RSC.
- A. Patel, S. Paul, N. Akhtar, S. Das, S. Kar, S. Bhattacharjee and D. Manna, ACS Appl. Nano Mater., 2022, 5, 16602–16611 CrossRef CAS.
- A. I. Share, K. Patel, C. Nativi, E. J. Cho, O. Francesconi, N. Busschaert, P. A. Gale, S. Roelens and J. L. Sessler, Chem. Commun., 2016, 52, 7560–7563 RSC.
- C. R. Elie, G. David and A. R. Schmitzer, J. Med. Chem., 2015, 58, 2358–2366 CrossRef CAS.
- K. Maslowska-Jarzyna, M. L. Korczak and M. J. Chmielewski, Front. Chem., 2021, 9, 690035 CrossRef CAS.
- H. Valkenier, L. W. Judd, H. Li, S. Hussain, D. N. Sheppard and A. P. Davis, J. Am. Chem. Soc., 2014, 136, 12507–12512 CrossRef CAS.
- P. S. Auti, G. George and A. T. Paul, RSC Adv., 2020, 10, 41353–41392 RSC.
- A. Docker, X. B. Shang, D. H. Yuan, H. Kuhn, Z. Y. Zhang, J. J. Davis, P. D. Beer and M. J. Langton, Angew. Chem., Int. Ed., 2021, 60, 19442–19450 CrossRef CAS PubMed.
- J. E. van Muijlwijk-Koezen, H. Timmerman, H. van der Goot, W. M. P. B. Menge, J. F. V. Kunzel, M. de Groote and A. P. IJzerman, J.
Med. Chem., 2000, 43, 2227–2238 CrossRef CAS.
- D. Mondal, M. Ahmad, P. Panwaria, A. Upadhyay and P. Talukdar, J. Org. Chem., 2022, 87, 10–17 CrossRef CAS PubMed.
- M. Boiocchi, L. Del Boca, D. E. Gomez, L. Fabbrizzi, M. Licchelli and E. Monzani, J. Am. Chem. Soc., 2004, 126, 16507–16514 CrossRef CAS PubMed.
- J. Q. Shi, L. E. A. Suarez, S. J. Yoon, S. Varghese, C. Serpa, S. Y. Park, L. Luer, D. Roca-Sanjuan, B. Milian-Medina and J. Gierschner, J. Phys. Chem. C, 2017, 121, 23166–23183 CrossRef CAS.
- A. Saha, N. Akhtar, V. Kumar, S. Kumar, H. K. Srivastava, S. Kumar and D. Manna, Org. Biomol. Chem., 2019, 17, 5779–5788 RSC.
- N. Akhtar, N. Pradhan, G. K. Barik, S. Chatterjee, S. Ghosh, A. Saha, P. Satpati, A. Bhattacharyya, M. K. Santra and D. Manna, ACS Appl. Mater. Interfaces, 2020, 12, 25521–25533 CrossRef CAS PubMed.
- X. Wu and P. A. Gale, Chem. Commun., 2021, 57, 3979–3982 RSC.
- A. Mondal, S. N. Save, S. Sarkar, D. Mondal, J. Mondal, S. Sharma and P. Talukdar, J. Am. Chem. Soc., 2023, 145, 9737–9745 CrossRef CAS.
- S. Dey, A. Patel, K. Raina, N. Pradhan, O. Biswas, R. P. Thummer and D. Manna, Chem. Commun., 2020, 56, 1661–1664 RSC.
|
This journal is © The Royal Society of Chemistry 2024 |
Click here to see how this site uses Cookies. View our privacy policy here.