FeCl3-catalyzed regioselective ring-opening of aryl oxirane with 4-hydroxycoumarin for the synthesis of furo[3,2-c]coumarins†‡
Received
22nd October 2023
, Accepted 17th November 2023
First published on 22nd November 2023
Abstract
The regioselective ring-opening of aryl oxiranes was investigated with various 4-hydroxycoumarins in dimethyl sulfoxide in the presence of 20 mol% FeCl3 as a catalyst at 110 °C. This approach provided a short and concise synthetic route for the regioselective synthesis of 2-aryl-4H-furo[3,2-c] coumarin derivatives. Product formation occurred through regioselective ring-opening of the aryl oxirane at a less hindered site, followed by dehydration and concomitant cyclization. The salient features of our protocol were: cost-effectiveness; short reaction time; step- and atom economy; easy handling; broad scope of substrates; regioselectivity; good-to-excellent yields; non-requirement of dry solvents, co-catalysts, ligands, or any other additives; inert atmospheric conditions.
Introduction
4-Hydroxycoumarin is an important coumarin derivative and an integral part of many naturally occurring compounds, for example, dicoumarol (I)1a and furocoumarins such as pterophyllin 2 (II), flemichapparin C (III), wedelolactone (IV), and neo-tanshinlactone (V).1b These derivatives exhibit interesting pharmacological activities.1c Some non-natural compounds derived from 4-hydroxycoumarin are also used as medicines due to their anticoagulant activity, such as warfarin.1d These compounds are shown in Fig. 1.
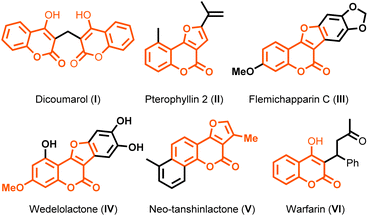 |
| Fig. 1 Some bioactive molecules containing a 4-hydroxycoumarin backbone. | |
These derivatives display various biological activities: anticancer,1e anti-depressant,2 antioxidant,3 anticoagulant,4 anti-inflammatory,5 and antidiabetic.6 They are also utilized as ingredients in cosmetics and perfumes and as additives in the food industry.7 Due to their incredible fluorescent properties,8 some of them are also used as fluorescent probes. Moreover, coumarins and furans are crucial heterocyclic scaffolds in many bioactive natural and non-natural products.9,10 Combining coumarin with furan introduces some unique and valuable biological activities into the molecule.11,12 An epoxide ring–ring of styrene oxides13 has been explored extensively in organic synthesis, and our research group has recently demonstrated the usefulness of aryl oxiranes (styrene oxides) in the synthesis of various new molecules.14a–c Taking cues from our earlier work, we envisioned that these aryl oxiranes could be explored further to synthesize furocoumarin derivatives on reaction with 4-hydroxycoumarins in the presence of FeCl3 (which acts as a Lewis acid).15a–d In 2007, Hu and co-workers reported16a an elegant approach for the synthesis of furocoumarins from 3-(phenylethynyl)-4H-chromen-4-one through one-pot cascade addition–cyclization–oxidation, as shown in Scheme 1a. In 2010, Xu and co-workers demonstrated the synthesis of furocoumarins via a sequential Pd/Cu-catalyzed alkynylation and intramolecular hydroalk-oxylation, as depicted in Scheme 1b.16b In 2017, Li and colleagues and Wang et al. showed17 the tandem cyclization of hypervalent iodine reagent (HIR) derived from 4-hydroxycoumarin with propiolic acids via Rh-catalyzed decarboxylation along with Ag2O as a co-catalyst for the synthesis of 4H-furo[3,2-c]chromen-4-one derivatives (Scheme 1c). In the same year, Hajra et al. reported the FeCl3/ZnI2-catalyzed synthesis of furocoumarin derivatives by intermolecular coupling between 4-hydroxycoumarins and alkynes, in which ZnI2 acted as an additive, as shown in Scheme 1d.18a Most of the earlier reported methods involved (directly or indirectly) aryl acetylenes or their derivatives to synthesize furocoumarin derivatives. Apart from aryl acetylenes, Chen et al. reported the synthesis of 2-aryl-3-allyl-furo[3,2-c]coumarins using a ZnCl2-mediated three-component reaction using a combination of 4-hydroxycoumarin, arylglyoxal monohydrate, and allyl trimethyl silane in toluene in addition to 2,3-diaryl-furo[3,2-c]coumarins by employing 20% mol% FeCl3 as a catalyst. Recently, Choudhary et al. explored18c the synthesis of 2-aryl-furo[3,2-c]coumarins and 2-aryl-3-thioaryl-furo[3,2-c]coumarins derivatives depending upon reaction conditions from 4-hydroxycoumarin, arylglyoxal monohydrate, and thiophenol in toluene at 111 °C in the presence of 10 mol% Sc(OTf)3. Due to unique structural features and biological activities, synthesizing these derivatives from the readily available starting materials is highly desirable. Though earlier approaches were unique and elegant, some methods had certain demerits: the cumbersome procedure for the preparation of pre-functionalized and aryl acetylene is expensive as compared with that for styrene oxide;16,17 requirement of additives;18a harsh and inert atmospheric reaction conditions; requirement of expensive transition-metal catalysts.17 Consequently, there is scope to develop a new synthetic route, which should be efficient, convenient, reliable, step-economic, and straightforward to produce diverse furocoumarin derivatives. In addition, styrene oxide is a building block for the synthesis of organic molecules.19
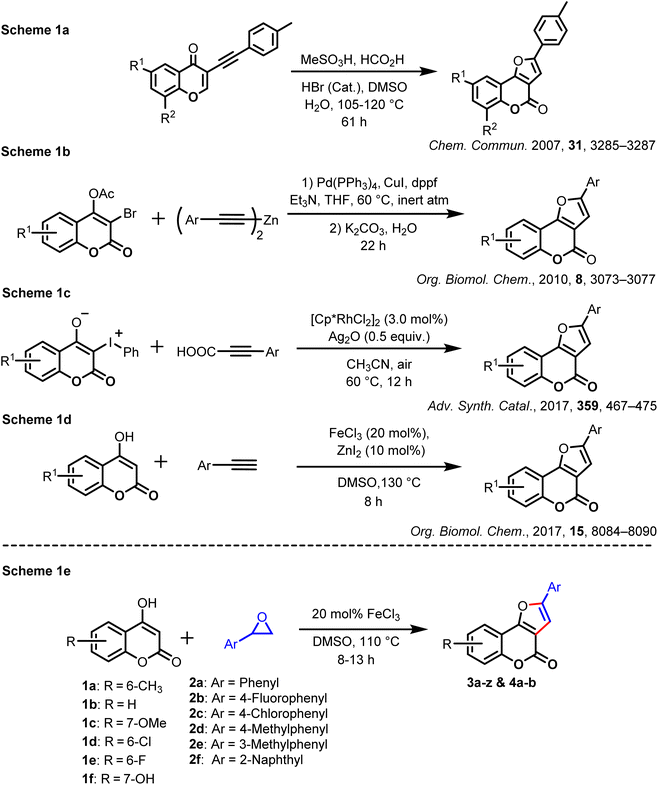 |
| Scheme 1 Previously reported methods and our synthetic strategy via regioselective ring-opening of aryl oxirane with 4-hydroxycoumarin. | |
We report a simple, high-atom-economical, straightforward, and inexpensive method for the synthesis of furocoumarin derivatives from 4-hydroxycoumarin and aryl oxirane via intermolecular regioselective ring-opening of an aryl oxirane followed by dehydration and cyclization using 20 mol% FeCl3 as a Lewis-acid catalyst in DMSO (Scheme 1d). Moreover, the yield of the desired product from this protocol was higher than that achieved from earlier reported methods.
Results and discussion
At the outset of the present study, model substrates were chosen (4-hydroxy-6-methylcoumarin (1a) and styrene oxide (2a)) to discover the most suitable reaction conditions. Initially, the reaction was carried out without a catalyst at room temperature and gradually increasing temperature up to 120 °C in DMSO as a solvent (Table 1, entry 1). No desired product was obtained. To ascertain the role of the catalyst, a similar reaction was carried out in the presence of 5 mol% FeCl3 at room temperature for 24 h (Table 1, entry 2), but there was no progress in the reaction. Then, the same reaction mixture was placed in a pre-heated oil bath at 60 °C and was heated for 15 h (Table 1, entry 3): the desired product (3a) was isolated in 15% yield. The product 3a was confirmed from spectra (IR, 1H, and 13C NMR) and HRMS. In the 1H NMR spectrum, the characteristic peak at δ 5.6 ppm for the H-3 group of compound 1a and the peaks at δ 3.82, δ 3.09, and δ 2.76 for the protons in the epoxide disappeared, and a new peak at δ 7.18 appeared for the H-3 group of the desired product 3a.
Table 1 Optimization of reaction conditionsa,b
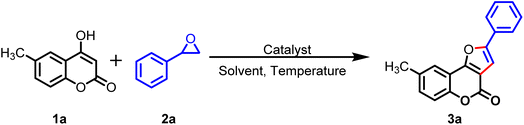
|
Entry |
Catalyst |
Mol% |
Solvent |
Time (h) |
Temperature (°C) |
Yieldb (%) |
Reaction conditions: all reactions were undertaken using 4-hydroxy-6-methylcoumarin (1a, 1.0 mmol) and styrene oxide (2a, 1.0 mmol).
Isolated yield.
Reaction carried out at room temperature. NR: no reaction; ND: no desired product.
|
1c |
— |
— |
DMSO |
24 |
RT → 120 |
ND |
2c |
FeCl3 |
5 |
DMSO |
24 |
RT |
ND |
3 |
FeCl3 |
5 |
DMSO |
15 |
60 |
15 |
4 |
FeCl3 |
5 |
DMSO |
15 |
80 |
32 |
5 |
FeCl3 |
5 |
DMSO |
13 |
100 |
45 |
6 |
FeCl3 |
5 |
DMSO |
10 |
110 |
62 |
7 |
FeCl3 |
5 |
DMSO |
10 |
120 |
59 |
8 |
FeCl3 |
10 |
DMSO |
9 |
110 |
72 |
9
|
FeCl
3
|
20
|
DMSO
|
8
|
110
|
89
|
10 |
FeCl3 |
30 |
DMSO |
8 |
110 |
88 |
11 |
FeCl3 |
20 |
DMF |
8 |
110 |
56 |
12 |
FeCl3 |
20 |
Toluene |
8 |
110 |
22 |
13 |
FeCl3 |
20 |
Methanol |
8 |
110 |
18 |
14 |
CoCl2 |
20 |
DMSO |
10 |
110 |
45 |
15 |
CuCl2 |
20 |
DMSO |
10 |
110 |
23 |
16 |
CSA(±) |
20 |
DMSO |
10 |
110 |
ND |
In the 13C-NMR spectrum, the peaks δ 51.9 and δ 50.8 for the epoxide carbon disappeared. In addition, the HRMS value of product 3a gave 277.0859 instead of the expected value of 277.0860, further indicating the formation of the desired product 3a. The role of temperature was also examined to improve the yield of the desired product. As we increased the temperature, the yield of (3a) increased significantly at 110 °C, and the desired product (3a) was obtained at 62% yield (Table 1, entries 4–7). Further increases in the temperature did not increase the yield of the desired product. The yield was reduced markedly above 140 °C and provided an inseparable mixture of products (which may have been due to decomposition). Next, the amount of catalyst required was examined, and the reaction was carried out using 10%, 20%, and 30 mol%, respectively (Table 1, entries 8–10). The screening result revealed that the reaction proceeded very well at 20 mol% of catalyst, and the desired product was isolated in 89% yield (Table 1, entry 9). To examine the suitability of other solvents, the reactions were carried out in DMF, toluene, and methanol (Table 1, entries 11–13). DMSO was the best solvent in terms of yield and reaction time. Motivated by this result, we carried out a reaction with other metal-chloride catalysts such as CoCl2, and CuCl2, and the yield obtained was 45% and 23%, respectively (Table 1, entries 14 and 15). However, no desired product (ND) was obtained in the presence of (±) CSA (Table 1, entry 16). After scrutinizing all the parameters, it was concluded that 20 mol% FeCl3 was the most effective catalyst at 110 °C in DMSO for this particular transformation.
After optimizing the reaction conditions, the scope and practicability of the reaction procedure stated above were investigated with various substituted 4-hydroxycoumarins and styrene oxides, as shown in Table 2.
Table 2 Substrate scope of furocoumarin scaffoldsa,b
Reaction conditions: all reactions were performed using 4-hydroxycoumarins (1a–c, 1.0 mmol) and styrene oxides (2a–e, 1.0 mmol) in the presence of FeCl3 in 2 mL of DMSO at 110 °C.
Isolated yield.
|
|
Then, the substrate scope of electron-rich 4-hydroxy-6-methylcoumarin (1a) was investigated with readily available 4-fluoro styrene oxide (2b) and 4-chloro styrene oxide (2c), and the expected products (3b) and (3c) were isolated in 78% and 79% yield, respectively. Subsequently, the reactions were examined with 4-hydroxy-6-methylcoumarin (1a) with the freshly prepared styrene oxides 2d and 2e under identical reaction conditions, and the desired products 3d and 3e were isolated in good yields. Notably, the styrene oxides having electron-withdrawing groups at the para-position, such as fluoro (2b) and chloro (2c), gave the cyclized products (3b) and (3c) in good-to-excellent yields. Similarly, the styrene oxide containing an electron-donating methyl group at the para (2d) and ortho (2e) positions provided the desired products 3d and 3e in good yields. To examine the generality of this protocol, similar reactions were examined with 4-hydroxy-coumarin (1b) with the five styrene oxides (2a–e) and afforded the desired products 3f–j in 89–79% yields. Likewise, 4-hydroxy-7-methoxycoumarin (1c) proceeded well with the styrene oxides (2a–e) to furnish the corresponding expected products 3k–3o in 79%–88% yields.
Subsequently, the cyclization reaction was scrutinized with 4-hydoxycoumarin having an electron-withdrawing group at the sixth position, such as chloro (1d) and fluoro (1e), under similar reaction conditions. From these studies, the desired products 3p–3s and 3t–3w were obtained in fairly good yields, as shown in Table 3. To enrich the diversity of products, the reactions were also examined with 4,7-dihydroxy coumarin (1f) and substituted styrene oxides 2b, 2c, and 2d, and the desired products 3x, 3y, and 3z were isolated in 79%, 85%, and 80% yields, respectively. To further check the practicability of the this procedure, the reaction was scrutinized with polyaromatic 2-naphthyl oxirane (2f) and 4-hydroxycoumarin (1b), and the desired product 4a was obtained in 80% yield. Likewise, the reaction between 2f and 6-fluoro-4-hydroxycoumarin (1e) provided the desired product 4b in 78% yield under identical reaction conditions, as depicted in Table 4.
Table 3 Substrate scope of furocoumarin scaffoldsa,b
Reaction conditions: all reactions were performed using substituted 4-hydroxycoumarin (1d–f, 1.0 mmol) and substituted styrene oxide (2a–e, 1.0 mmol) in the presence of FeCl3 in 2 mL of DMSO at 110 °C.
Isolated yield. NR: no reaction.
|
|
Table 4 Substrate scope of furocoumarin scaffoldsa,b
Reaction conditions: all reactions were performed using substituted 4-hydroxycoumarin (1b and 1e, 1.0 mmol) and 2-naphthyl oxirane (2f, 1.0 mmol) in the presence of FeCl3 in 2 mL of DMSO at 110 °C.
Isolated yield.
|
|
All products were characterized using spectroscopy (IR, 1H-NMR, and 13C-NMR) as well as HRMS. To ascertain the regioselective opening of the styrene oxide, the product 3h was also confirmed with a single XRD datum. The ORTEP diagram of compound 3h is depicted in Fig. 2.
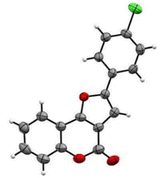 |
| Fig. 2 ORTEP diagram of product 3h. | |
To ascertain if the reaction was going through a radical pathway, a control experiment was performed with 6-methyl-4-hydroxycoumarin (1a) and styrene oxide (2a) in the presence of the radical inhibitor (2,2,6,6-tetramethylpiperidin-1-yl)oxyl (TEMPO, 1 equiv.), which is shown in Scheme 2. Experimental results revealed that the product 3a was isolated in 62% yield. Next, we prepared substrate 5 by another route, and we examined the cyclization reaction under identical reaction conditions in the presence of the catalyst (20 mol% FeCl3). However, we did not obtain the expected cyclized product 3g. From these two observations, we concluded that the reaction did not go through a radical pathway or through intermediate 5.
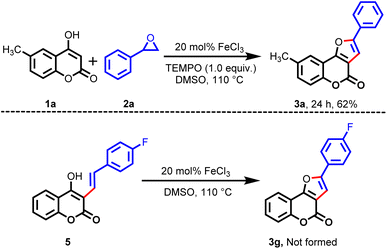 |
| Scheme 2 Control experiment. | |
The formation of the product could be explained. In 1964, Swanson and Laurie reported that FeCl3 could undergo a disproportionate reaction to give reactive Lewis-acid species [FeCl2]+ and [FeCl4]− in polar solvents such as DMF, pyridine, and DMSO.21 In 2018, Matsubara et al. demonstrated that FeCl3 acted as an ion-pairing Lewis-acid catalyst for the aza-Diels–Alder reaction.15d Based on their observations, we postulated a mechanism for formation of the desired product, as shown in Scheme 3. Initially, styrene oxide reacts with the reactive species [FeCl2]+ to give the highly reactive intermediate A. Subsequently, intermediate A reacts with 4-hydroxy-6-methylcoumarin (1a) to provide intermediate B by regioselective epoxide ring-opening at the less hindered site of styrene oxide (2a).13–15 Then, the chloride anion (Cl−), obtained from [FeCl4]−, assists in breaking of the Fe–O bond to provide the intermediate C. Next, Cl− removes the hydrogen atom from intermediate C, which generates the negatively charged oxygen atom, which facilitates the cyclization of intermediate C by eliminating one molecule of water to provide the cyclized intermediate D. Finally, the cyclized intermediate D undergoes oxidation by FeCl3 to form the desired product 3a. Simultaneously, the reduced FeCl2 undergoes aerial oxidation to FeCl3, and participates in the catalytic cycle. The unsuccessful result of the reaction between 1e and 2c may not occur. This is probably because of an electron-withdrawing group on both rings. Cyclization of the intermediate C is not facilitated if both rings contain a strong electron-withdrawing group because a lone pair of oxygen atoms is not readily available to attack the carbon atom to cyclize.
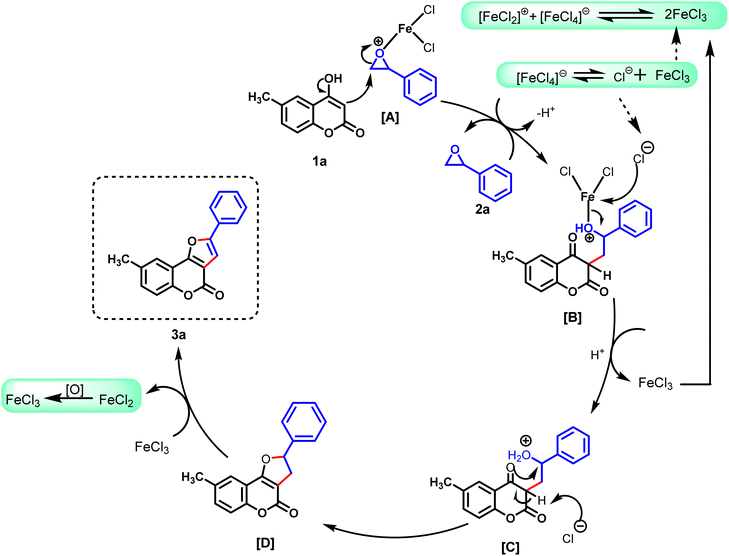 |
| Scheme 3 A plausible mechanism for the synthesis of furocoumarin derivatives. | |
A large-scale reaction (0.810 g, 5 mmol) was examined with 4-hydroxycoumarin (1a) and styrene oxide (2a). The desired product 3f was obtained at 1.012 g (97%) along with the recovery of 0.168 g of 4-hydroxycoumarin (1a). The yield was calculated based on recovery of the starting material (Scheme 4).
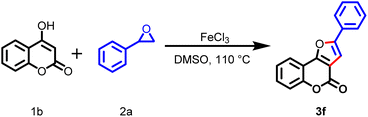 |
| Scheme 4 Large-scale reaction between 4-hydroxycoumarin (1b) with styrene oxide (2a). | |
Conclusions
We demonstrated that the regioselective ring-opening of aryl oxirane with 4-hydroxycoumarins was a useful reaction for synthesizing 2-Aryl-4H-furo[3,2-c]coumarin derivatives. The catalyst FeCl3 had unique features: it acted as a Lewis acid and existed in an ion-pair form in the solvent. The product was created through formation of a C–C bond between the H-3 C of 4-hydroxycoumarin and the less hindered site of the styrene oxide, followed by concomitant cyclization with the elimination of water molecules. Product formation proceeded through a cascade reaction, such as intermolecular coupling–dehydration–cyclization. The advantages of this protocol were: no need for derivatization of 4-hydroxycoumarin required for incorporation of arylacetylene at the C-3 position; non-requirement of any additive; easy handling; mild reaction conditions; high regioselectivity; no need for additives or ligands. Furthermore, diverse furocoumarins were developed using this protocol. This novel synthetic approach might attract attention from pharmaceutical and fine-chemical industries, and may be considered a “greener” approach compared with strategies reported previously.
General procedure for the synthesis of aryl oxirane (2d–2f)20
In a 100 mL dry round-bottomed flask, the corresponding substituted styrene (5 mmol) was taken in 25 mL of dichloromethane. The reaction flask was placed in an ice bath at 0–5 °C. Then, m-chloroperbenzoic acid (m-CPBA) was added in portions for 30 min at the ice-bath temperature. After 2 h of stirring at 0–5 °C, the reaction was brought slowly to room temperature and stirred for an additional 6 h. The resulting mixture was passed through a filter paper. The solvent was concentrated using a rotatory evaporator. The crude residue was separated by column chromatography (silica gel at 60–120 mesh).
General procedure for the synthesis of furocoumarin derivatives 3a–3z and 4a–4b
In a dry 25 mL round-bottomed flask, 4-hydroxycoumarin (1, 1.0 mmol) and styrene oxide (2, 1.0 mmol) were dissolved in 2 mL of DMSO. Then, the catalyst (20 mol% FeCl3) was added to the reaction mixture and placed in a pre-heated oil bath at 110 °C with constant stirring under an air atmosphere. The progress of the reaction was monitored by checking TLC occasionally. After completion of the reaction, the reaction mixture became a Castleton Green color. Then, the reaction was brought to room temperature, and the resulting mixture was diluted with 10 mL of DCM. The organic layer was washed with brine solution (5 mL × 2). The organic extract was dried over anhydrous sodium sulfate, and the solvent was removed in a rotary evaporator. Finally, the crude residue was passed through a silica-gel column (mesh of 60–120) to obtain the pure and desired products.
8-Methyl-2-phenyl-4H-furo[3,2-c]chromen-4-one (3a)
White solid (245.70 mg, 89%) mp 185–186 °C (lit. mp. 187–188 °C).181H NMR (600 MHz, CDCl3) δ 7.82 (d, J = 7.8 Hz, 2H), 7.75 (s, 1H), 7.48 (t, J = 7.6 Hz, 2H), 7.41–7.39 (m, 1H), 7.34 (q, J = 8.6 Hz, 2H), 7.18 (s, 1H), 2.49 (s, 3H); 13C NMR (150 MHz, CDCl3) δ 158.6, 157.1, 156.6, 151.0, 134.6, 131.8, 129.2, 129.1, 129.1, 124.7, 120.6, 117.2, 112.5, 112.5, 102.8, 21.1; IR (KBr) νmax/cm−1 1745 (C
O), 1622 (C
C), 1310 (C–O); HRMS (ESI) calcd for C18H13O3 277.0860 (M + H+); found 277.0859.
2-(4-Fluorophenyl)-8-methyl-4H-furo[3,2-c]chromen-4-one (3b)
White solid (229.37 mg, 78%) mp 215–216 °C. 1H NMR (400 MHz, CDCl3) δ 7.79 (ddd, J = 8.8, 5.0, 2.5 Hz, 2H), 7.72 (s, 1H), 7.33 (d, J = 2.1 Hz, 2H), 7.17 (t, J = 8.6 Hz, 2H), 7.10 (s, 1H), 2.48 (s, 3H); 13C NMR (100 MHz, CDCl3) δ 163.2 (JC–F = 248 Hz), 162.0, 158.5, 157.1, 155.6, 150.9, 134.6, 131.9, 126.6 (JC–F = 8.2 Hz), 125.5 (JC–F = 3.37 Hz), 120.6, 117.3, 116.4, 116.2, 112.5 (JC–F = 8.9 Hz), 102.5 (JC–F = 1.4 Hz), 21.1; 19F NMR (376 MHz, CDCl3) δ −111.12; IR (KBr) νmax/cm−1 1740 (C
O), 1620 (C
C), 1290 (C–O); HRMS (ESI) calcd for C18H12FO3 295.0765 (M + H+); found 295.0765.
2-(4-Chlorophenyl)-8-methyl-4H-furo[3,2-c]chromen-4-one (3c)
White solid (244.93 mg, 79%) mp 210–212 °C. 1H NMR (600 MHz, CDCl3) δ 7.74 (d, J = 8.9 Hz, 3H), 7.45 (d, J = 8.3 Hz, 2H), 7.36–7.33 (m, 2H), 7.16 (s, 1H), 2.48 (s, 3H); 13C NMR (150 MHz, CDCl3) δ 158.4, 157.2, 155.4, 151.0, 135.1, 134.6, 132.0, 129.4, 127.6, 125.8, 120.6, 117.3, 112.5, 112.4, 103.3, 21.1; IR (KBr) νmax/cm−1 1740 (C
O), 1622 (C
C), 1290 (C–O); HRMS (ESI) calcd for C18H12ClO3 311.0470 (M + H+); found 311.0465.
8-Methyl-2-(p-tolyl)-4H-furo[3,2-c]chromen-4-one (3d)
White solid (246.58 mg, 85%) mp 185–186 °C (lit. mp. 185–187 °C).181H NMR (600 MHz, CDCl3) δ 7.75 (s, 1H), 7.71 (d, J = 8.1 Hz, 2H), 7.35–7.31 (m, 2H), 7.28 (d, J = 8.0 Hz, 2H), 7.11 (s, 1H), 2.48 (s, 3H), 2.42 (s, 3H); 13C NMR (150 MHz, CDCl3) δ 158.7, 156.8, 156.8, 150.9, 139.4, 134.5, 131.6, 129.8, 126.4, 124.6, 120.6, 117.2, 112.6, 112.5, 102.0, 21.5, 21.1; IR (KBr) νmax/cm−1 1735 (C
O), 1600 (C
C), 1280 (C–O); HRMS (ESI) calcd for C18H13O3 291.1016 (M + H+); found 291.1035.
8-Methyl-2-(m-tolyl)-4H-furo[3,2-c]chromen-4-one (3e)
White solid (232.07 mg, 80%) mp 238–240 °C. 1H NMR (600 MHz, CDCl3) δ 7.77 (s, 1H), 7.64–7.62 (m, 2H), 7.38–7.33 (m, 3H), 7.21 (d, J = 7.6 Hz, 1H), 7.16 (s, 1H), 2.49 (s, 3H), 2.45 (s, 3H); 13C NMR (100 MHz, CDCl3) δ 167.3, 158.6, 157.0, 156.8, 151.0, 138.9, 134.5, 131.8, 130.0, 129.0, 125.2, 121.9, 120.7, 117.2, 112.6, 112.6, 102.7, 21.6, 21.1; IR (KBr) νmax/cm−1 1750 (C
O), 1580 (C
C), 1280 (C–O); HRMS (ESI) calcd for C18H13O3 291.1016 (M + H+); found 291.1043.
2-Phenyl-4H-furo[3,2-c]chromen-4-one (3f)
White solid (233.23 mg, 89%) mp 184–185 °C (lit. mp. 186–187 °C).181H NMR (400 MHz, CDCl3) δ 7.98 (dd, J = 7.8, 1.4 Hz, 1H), 7.84–7.82 (m, 2H), 7.56–7.50 (m, 2H), 7.49–7.46 (m, 2H), 7.43–7.37 (m, 2H), 7.19 (s, 1H); 13C NMR (150 MHz, CDCl3) δ 158.4, 157.0, 156.8, 152.7, 130.7, 129.33, 129.2, 129.1, 124.7, 124.7, 120.9, 117.5, 112.9, 112.6, 102.8; IR (KBr) νmax/cm−1 1742 (C
O), 1622 (C
C), 1250 (C–O); HRMS (ESI) calcd for C17H11O3 263.0703 (M + H+); found 263.0681.
2-(4-Fluorophenyl)-4H-furo[3,2-c]chromen-4-one (3g)
White solid (221.24 mg, 79%) mp 192–196 °C (lit. mp. 210–215 °C).181H NMR (600 MHz, CDCl3) δ 7.95 (d, J = 7.7 Hz, 1H), 7.80 (dd, J = 8.6, 5.2 Hz, 2H), 7.55–7.52 (m, 1H), 7.47 (d, J = 8.3 Hz, 1H), 7.39 (t, J = 7.5 Hz, 1H), 7.18 (t, J = 8.6 Hz, 2H), 7.13 (s, 1H); 13C NMR (150 MHz, CDCl3) δ 163.3 (JC–F = 248.7 Hz), 158.3, 157.0, 155.8, 152.7, 130.8, 126.7(JC–F = 8.2 Hz), 125.4 (JC–F = 3.3 Hz), 124.7, 120.9, 117.6, 116.4 (JC–F = 22 Hz), 112.8, 112.6, 102.5 (JC–F = 1.0 Hz); 19F NMR (565 MHz, CDCl3) δ −110.99; IR (KBr) νmax/cm−1 1750 (C
O), 1590 (C
C), 1280 (C–O); HRMS (ESI) calcd for C17H10FO3 281.0609 (M + H+); found 281.0609.
2-(4-Chlorophenyl)-4H-furo[3,2-c]chromen-4-one (3h)
White solid (239.77 mg, 81%) mp 248–250 °C. 1H NMR (600 MHz, CDCl3) δ 7.96 (d, J = 7.8 Hz, 1H), 7.75 (d, J = 8.4 Hz, 2H), 7.54 (t, J = 7.8 Hz, 1H), 7.46 (t, J = 7.6 Hz, 3H), 7.39 (t, J = 7.5 Hz, 1H), 7.18 (s, 1H); 13C NMR (150 MHz, CDCl3) δ 158.2, 157.2, 155.6, 152.8, 135.2, 131.0, 129.5, 127.6, 125.9, 124.8, 120.9, 117.6, 112.8, 112.7, 103.3; IR (KBr) νmax/cm−1 1736 (C
O), 1620 (C
C), 1280 (C–O); HRMS (ESI) calcd for C17H10ClO3 297.0313 (M + H+); found 297.0313.
2-(p-Tolyl)-4H-furo[3,2-c]chromen-4-one (3i)
White solid (237.42 mg, 86%) mp 186–188 °C (lit. mp. 190–192 °C).181H NMR (400 MHz, CDCl3) δ 7.98–7.96 (m, 1H), 7.71 (d, J = 8.2 Hz, 2H), 7.53 (ddd, J = 8.6, 7.2, 1.5 Hz, 1H), 7.48–7.45 (m, 1H), 7.40–7.36 (m, 1H), 7.29 (d, J = 8.0 Hz, 2H), 7.13 (s, 1H), 2.42 (s, 3H); 13C NMR (100 MHz, CDCl3) δ 158.5, 157.0, 156.8, 156.4, 152.7, 139.5, 130.6, 129.8, 126.4, 124.7, 120.9, 117.5, 113.0, 112.6, 102.0, 21.5; IR (KBr) νmax/cm−1 1743 (C
O), 1585 (C
C), 1200 (C–O); HRMS (ESI) calcd for C18H13O3 277.0860 (M + H+); found 277.0860.
2-(m-Tolyl)-4H-furo[3,2-c]chromen-4-one (3j)
White solid (226.38 mg, 82%) mp 168–169 °C (lit. mp. 168–170 °C).181H NMR (400 MHz, CDCl3) δ 7.99 (dd, J = 7.8, 1.4 Hz, 1H), 7.63 (d, J = 8.2 Hz, 2H), 7.56–7.51 (m, 1H), 7.48–7.46 (m, 1H), 7.41–7.35 (m, 2H), 7.22 (d, J = 7.3 Hz, 1H), 7.17 (s, 1H), 2.45 (s, 3H); 13C NMR (100 MHz, CDCl3) δ 175.1, 152.7, 138.9, 130.7, 130.7, 130.1, 129.1, 129.0, 128.6, 125.3, 124.7, 121.9, 120.9, 117.5, 112.9, 112.6, 102.7, 21.6; IR (KBr) νmax/cm−1 1743 (C
O), 1585 (C
C), 1200 (C–O); HRMS (ESI) calcd for C18H13O3 277.0860 (M + H+); found 277.0862.
7-Methoxy-2-phenyl-4H-furo[3,2-c]chromen-4-one (3k)
White solid (257.02 mg, 88%) mp 172–173 °C (lit.16 mp. 170–172 °C). 1H NMR (600 MHz, CDCl3) δ 7.86 (d, J = 9.3 Hz, 1H), 7.81–7.79 (m, 2H), 7.47 (t, J = 7.7 Hz, 2H), 7.38 (t, J = 7.4 Hz, 1H), 7.15 (s, 1H), 6.97 (dq, J = 4.0, 2.3 Hz, 2H), 3.91 (s, 3H); 13C NMR (150 MHz, CDCl3) δ 162.1, 158.7, 157.9, 157.8, 155.9, 154.6, 129.3, 129.1, 129.0, 124.5, 121.9, 113.0, 106.3, 102.6, 101.6, 55.9; IR (KBr) νmax/cm−1 1740 (C
O), 1622 (C
C), 1300 (C–O); HRMS (ESI) calcd for C18H13O4 293.0809 (M + H+); found 293.0810.
2-(4-Fluorophenyl)-7-methoxy-4H-furo[3,2-c]chromen-4-one (3l)
White solid (257.35 mg, 83%) mp 220–221 °C. 1H NMR (400 MHz, CDCl3) δ 7.84 (d, J = 9.3 Hz, 1H), 7.79–7.76 (m, 2H), 7.17 (t, J = 8.7 Hz, 2H), 7.08 (s, 1H), 6.98–6.95 (m, 2H), 3.90 (s, 3H); 13C NMR (100 MHz, CDCl3) δ 164.3, 162.1, 158.7, 157.7, 154.9, 154.5, 126.4 (JC–F = 8.26 Hz), 125.6 (JC–F = 1.58 Hz), 121.8, 116.3 (JC–F = 22.1 Hz), 113.1, 110.1, 106.2, 102.3 (JC–F = 1.29 Hz), 101.6, 55.9; 19F NMR (376 MHz, CDCl3) δ −111.47. IR (KBr) νmax/cm−1 1735 (C
O), 1592 (C
C), 1285 (C–O); HRMS (ESI) calcd for C18H12FO4 311.0715 (M + H+); found 311.0716.
2-(4-Chlorophenyl)-7-methoxy-4H-furo[3,2-c]chromen-4-one (3m)
White solid (257.56 mg, 79%) mp 218–220 °C. 1H NMR (400 MHz, CDCl3) δ 7.86–7.84 (m, 1H), 7.74–7.71 (m, 2H), 7.46–7.43 (m, 2H), 7.14 (s, 1H), 6.97 (dd, J = 6.3, 2.4 Hz, 2H), 3.91 (s, 3H); 13C NMR (100 MHz, CDCl3) δ, 162.3, 158.5, 157.9, 154.7, 154.6, 134.8, 129.4, 127.7, 125.7, 121.9, 113.1, 110.1, 106.1, 103.1, 101.6, 55.9; IR (KBr) νmax/cm−1 1735 (C
O), 1592 (C
C), 1285 (C–O); HRMS (ESI) calcd for C18H12ClO4 327.0419 (M + H+); found 327.0419.
7-Methoxy-2-(p-tolyl)-4H-furo[3,2-c]chromen-4-one (3n)
White solid (263.23 mg, 86%) mp 225–226 °C. 1H NMR (600 MHz, CDCl3) δ 7.86–7.85 (m, 1H), 7.68 (d, J = 8.1 Hz, 2H), 7.28–7.26 (m, 2H), 7.08 (s, 1H), 6.96 (dq, J = 4.4, 2.3 Hz, 2H), 3.90 (s, 3H), 2.41 (s, 3H); 13C NMR (150 MHz, CDCl3) δ 162.0, 158.8, 157.5, 156.1, 154.4, 139.1, 129.8, 126.5, 124.5, 121.8, 113.0, 110.2, 106.4, 101.8, 101.6, 55.9, 21.5; IR (KBr) νmax/cm−1 1738 (C
O), 1622 (C
C), 1310 (C–O); HRMS (ESI) calcd for C19H15O4 307.0965 (M + H+); found 307.0965.
7-Methoxy-2-(m-tolyl)-4H-furo[3,2-c]chromen-4-one (3o)
White solid (244.87 mg, 80%) mp 228–230 °C. 1H NMR (600 MHz, CDCl3) δ 7.88–7.87 (m, 1H), 7.60 (d, J = 11.5 Hz, 2H), 7.36 (t, J = 7.6 Hz, 1H), 7.20 (d, J = 7.6 Hz, 1H), 7.13 (s, 1H), 6.97 (dq, J = 4.3, 2.3 Hz, 2H), 3.90 (s, 3H), 2.44 (s, 3H); 13C NMR (150 MHz, CDCl3) δ 162.1, 158.8, 157.7, 156.0, 154.5, 138.8, 129.8, 129.2, 129.0, 125.1, 121.9, 121.78, 113.0, 110.1, 106.4, 102.5, 101.6, 55.9, 21.6; IR (KBr) νmax/cm−1 1738 (C
O), 1622 (C
C), 1310 (C–O); HRMS (ESI) calcd for C19H15O4 307.0965 (M + H+); found 307.0965.
8-Chloro-2-phenyl-4H-furo[3,2-c]chromen-4-one (3p)
White solid (263.46 mg, 89%) mp 215–216 °C (lit. mp. 215–216 °C).161H NMR (600 MHz, CDCl3) δ 7.94 (d, J = 2.4 Hz, 1H), 7.83–7.81 (m, 2H), 7.50–7.46 (m, 3H), 7.43–7.40 (m, 2H), 7.19 (s, 1H); 13C NMR (150 MHz, CDCl3) δ 157.7, 157.4, 155.6, 151.0, 130.6, 130.3, 129.6, 129.2, 128.8, 124.8, 120.4, 118.9, 113.9, 113.4, 102.9; IR (KBr) νmax/cm−1 1745 (C
O), 1583 (C
C), 1296 (C–O); HRMS (ESI) calcd for C17H10ClO3 297.0313 (M + H+); found 297.0313.
8-Chloro-2-(4-fluorophenyl)-4H-furo[3,2-c]chromen-4-one (3q)
White solid (257.49 mg, 82%) mp 234–235 °C (lit. mp. 234 °C).181H NMR (400 MHz, CDCl3) δ 7.92 (d, J = 2.4 Hz, 1H), 7.82–7.79 (m, 2H), 7.48 (dd, J = 8.9, 2.4 Hz, 1H), 7.41 (d, J = 8.9 Hz, 1H), 7.19 (t, J = 8.7 Hz, 2H), 7.13 (s, 1H). 13C NMR (100 MHz, CDCl3) δ 163.4 (JC–F = 249.09 Hz), 157.7, 156.5, 155.6, 151.0, 130.7, 130.3, 126.8 (JC–F = 8.34 Hz), 125.1 (JC–F = 3.37 Hz), 120.4, 119.0, 116.5 (JC–F = 33.12 Hz), 113.8, 113.4, 102.6 (JC–F = 1.44 Hz). 19F NMR (376 MHz, CDCl3) δ −110.45; IR (KBr) νmax/cm−1 1732 (C
O), 1580 (C
C), 1256 (C–O); HRMS (ESI) calcd for C17H9ClFO3 315.0219 (M + H+); found 315.0213.
8-Chloro-2-(4-chlorophenyl)-4H-furo[3,2-c]chromen-4-one (3r)
White solid (263.98 mg, 80%) mp 262–263 °C. 1H NMR (600 MHz, CDCl3) δ 7.93 (d, J = 2.4 Hz, 1H), 7.76–7.74 (m, 2H), 7.49–7.46 (m, 3H), 7.41 (d, J = 8.8 Hz, 1H), 7.18 (s, 1H); 13C NMR (150 MHz, CDCl3) δ 157.6, 156.3, 155.8, 151.1, 135.5, 130.8, 130.4, 129.5, 127.3, 126.0, 120.5, 119.0, 113.8, 113.4, 103.3; IR (KBr) νmax/cm−1 1732 (C
O), 1582 (C
C), 1256 (C–O); HHRMS (ESI) calcd for C17H9Cl2O3 330.9924 (M + H+); found 331.0257.
8-Chloro-2-(p-tolyl)-4H-furo[3,2-c]chromen-4-one (3s)
White solid (244.93 mg, 79%) mp 212–213 °C. 1H NMR (600 MHz, DMSO-d6) δ 7.99 (dd, J = 8.5, 3.0 Hz, 1H), 7.92–7.90 (m, 2H), 7.57 (d, J = 3.2 Hz, 1H), 7.41 (d, J = 7.9 Hz, 2H), 7.01 (d, J = 8.6 Hz, 1H), 6.96 (s, 1H), 2.44 (s, 3H). 13C NMR (100 MHz, DMSO-d6) δ 160.5, 157.7, 157.2, 155.0, 154.0, 138.6, 129.7, 126.1, 124.2, 122.2, 113.6, 108.8, 104.3, 103.0, 102.2, 21.0. IR (KBr) νmax/cm−1 1745 (C
O), 1622 (C
C), 1310 (C–O); HRMS (ESI) calcd for C18H12ClO3 311.0470 (M + H+); found 311.0708.
8-Chloro-2-(m-tolyl)-4H-furo[3,2-c]chromen-4-one (3t)
White solid (241.83 mg, 78%) mp 212–213 °C. 1H NMR (600 MHz, CDCl3) δ 7.95 (d, J = 2.3 Hz, 1H), 7.64–7.62 (m, 2H), 7.47 (dd, J = 8.8, 2.4 Hz, 1H), 7.41–7.36 (m, 2H), 7.24 (d, J = 7.8 Hz, 1H), 7.17 (s, 1H), 2.45 (s, 3H). 13C NMR (150 MHz, CDCl3) δ 159.6, 157.8, 157.6, 155.6, 151.0, 139.0, 130.6, 130.4, 130.3, 129.1, 128.7, 125.4, 122.0, 120.6, 120.4, 118.9, 102.7, 29.8; IR (KBr) νmax/cm−1 1745 (C
O), 1610 (C
C), 1265 (C–O); HRMS (ESI) calcd for C18H12ClO3 311.0470 (M + H+); found 311.0595.
8-Fluoro-2-phenyl-4H-furo[3,2-c]chromen-4-one (3u)
White solid (212.84 mg, 76%) mp 179–180 °C (lit. mp. 178.3–179.1 °C).171H NMR (600 MHz, CDCl3) δ 7.83–7.82 (m, 2H), 7.65–7.63 (m, 1H), 7.50 (t, J = 7.6 Hz, 2H), 7.46–7.41 (m, 2H), 7.25–7.23 (m, 1H), 7.20 (s, 1H); 13C NMR (100 MHz, CDCl3) δ 159.2 (JC–F = 243.91 Hz), 157.8, 156.3 (JC–F = 3.9 Hz), 156.2, 148.9, 135.5, 129.5, 127.3, 126.0, 119.3 (JC–F = 8.58 Hz), 118.3 (JC–F = 24.38 Hz), 113.4, 109.9, 106.8 (JC–F = 25.81) 103.4; 19F NMR (377 MHz, CDCl3) δ −116.24; IR (KBr) νmax/cm−1 1732 (C
O), 1580 (C
C), 1260 (C–O); HRMS (ESI) calcd for C17H10FO3 281.0609 (M + H+); found 281.0652.
2-(4-Chlorophenyl)-8-fluoro-4H-furo[3,2-c]chromen-4-one (3v)
White solid (226.09 mg, 72%) mp 181–183 °C. 1H NMR (600 MHz, CDCl3) δ 7.76–7.74 (m, 2H), 7.62 (dd, J = 7.7, 3.0 Hz, 1H), 7.48–7.46 (m, 2H), 7.45–7.44 (m, 1H), 7.25–7.23 (m, 1H), 7.19 (s, 1H); 13C NMR (150 MHz, CDCl3) δ 159.3 (JC–F = 243.72 Hz), 157.9, 156.3 (JC–F = 2.91 Hz), 156.3, 149.0, 135.6, 129.6, 127.4, 126.1, 119.4 (JC–F = 8.55 Hz), 118.4 (JC–F = 24.42 Hz), 113.6, 113.5, 106.9 (JC–F = 25.69 Hz), 103.5; 19F NMR (377 MHz, CDCl3) δ −116.08; IR (KBr) νmax/cm−1 1736 (C
O), 1595 (C
C), 1293 (C–O); HRMS (ESI) calcd for C17H9ClFO3 315.0219 (M + H+); found 315.0213.
8-Fluoro-2-(p-tolyl)-4H-furo[3,2-c]chromen-4-one (3w)
White solid (235.25 mg, 80%) mp 185–186 °C. 1H NMR (600 MHz, CDCl3) δ 7.71 (d, J = 8.1 Hz, 2H), 7.62 (dd, J = 7.7, 2.9 Hz, 1H), 7.44 (dd, J = 9.1, 4.2 Hz, 1H), 7.29 (d, J = 8.0 Hz, 2H), 7.23 (td, J = 8.7, 2.9 Hz, 1H), 7.13 (s, 1H), 2.42 (s, 3H); 13C NMR (150 MHz, CDCl3) δ 159.1 (JC–F = 243.48 Hz), 158.1, 157.6, 155.8 (JC–F = 2.76 Hz), 148.7 (JC–F = 2.05 Hz), 139.8, 129.9, 126.1, 124.7, 119.2 (JC–F = 8.62 Hz), 117.9 (JC–F = 24.48 Hz), 113.7 (JC–F = 9.87 Hz), 113.4, 106.7 (JC–F = 25.75 Hz), 102.1, 21.5; 19F NMR (565 MHz, CDCl3) δ −116.33. IR (KBr) νmax/cm−1 1745 (C
O), 1622 (C
C), 1310 (C–O); HRMS (ESI) calcd for C18H12FO3 295.0765 (M + H+); found 295.0765.
2-(4-Fluorophenyl)-7-hydroxy-4H-furo[3,2-c]chromen-4-one (3x)
White solid (233.87 mg, 79%); mp 198–199 °C. 1H NMR (600 MHz, DMSO-d6) δ 7.99–7.96 (m, 2H), 7.89 (d, J = 8.3 Hz, 1H), 7.54 (s, 1H), 7.35 (t, J = 9.0 Hz, 2H), 6.92 (dd, J = 8.5, 2.0 Hz, 1H), 6.87 (d, J = 2.3 Hz, 1H); 13C NMR (150 MHz, DMSO-d6) δ 162.2 (JC–F = 246.06 Hz), 160.7, 157.6, 157.4, 154.0, 153.8, 126.5 (JC–F = 8.31 Hz), 125.4 (JC–F = 3.03 Hz), 122.3, 116.2 (JC–F = 21.96 Hz), 113.6, 108.8, 104.2, 103.0, 102.9; 19F NMR (565 MHz, DMSO-d6) δ −112.06; IR (KBr) νmax/cm−1 3510 (O–H), 1732 (C
O), 1615 (C
C), 1265 (C–O); HRMS (ESI) calcd for C17H10FO4 297.0558 (M + H+); found 297.0743.
2-(4-Chlorophenyl)-7-hydroxy-4H-furo[3,2-c]chromen-4-one (3y)
White solid (265.21 mg, 85%); mp 288–290 °C. 1H NMR (600 MHz, DMSO-d6) δ 7.96 (d, J = 8.5 Hz, 2H), 7.91 (d, J = 8.6 Hz, 1H), 7.65 (s, 1H), 7.58 (d, J = 8.5 Hz, 2H), 6.92 (dd, J = 8.6, 2.1 Hz, 1H), 6.88 (d, J = 2.0 Hz, 1H); 13C NMR (150 MHz, DMSO-d6) δ 160.7, 157.5, 157.5, 154.1, 153.5, 133.2, 129.1, 127.6, 125.8, 122.3, 113.6, 108.8, 104.1, 103.9, 103.0; IR (KBr) νmax/cm−1 3500 (O–H), 1730 (C
O), 1615 (C
C), 1250 (C–O); HRMS (ESI) calcd for C17H10ClO4 313.0263 (M + H+); found 313.0261.
7-Hydroxy-2-(p-tolyl)-4H-furo[3,2-c]chromen-4-one (3z)
White solid (233.65 mg, 80%); mp 265–266 °C (lit. mp. >250 °C). 1H NMR (600 MHz, DMSO-d6) δ 7.99 (dd, J = 8.5, 3.0 Hz, 1H), 7.92–7.90 (m, 2H), 7.57 (d, J = 3.2 Hz, 1H), 7.41 (d, J = 7.9 Hz, 2H), 7.01 (d, J = 8.6 Hz, 1H), 6.97–6.96 (s, 1H), 2.44 (s, 3H); 13C NMR (100 MHz, DMSO-d6) δ 160.5, 157.7, 157.2, 155.0, 154.0, 138.6, 129.7, 126.1, 124.2, 122.2, 113.6, 108.8, 104.3, 103.0, 102.2, 21.0; IR (KBr) νmax/cm−1 3500 (O–H), 1740 (C
O), 1622 (C
C), 1280 (C–O); HRMS (ESI) calcd for C18H13O4 293.0809 (M + H+); found 293.0810.
2-(Naphthalen-2-yl)-4H-furo[3,2-c]chromen-4-one (4a)
White solid (249.66 mg, 80%); mp 230–232 °C. 1H NMR (600 MHz, CDCl3) δ 8.32 (s, 1H), 8.05 (dd, J = 7.8, 1.4 Hz, 1H), 7.94 (dd, J = 8.0, 5.0 Hz, 2H), 7.88–7.85 (m, 2H), 7.57–7.52 (m, 3H), 7.48 (d, J = 8.2 Hz, 1H), 7.43–7.40 (m, 1H), 7.30 (s, 1H). 13C NMR (150 MHz, CDCl3) δ 158.4, 157.2, 156.8, 152.8, 133.5, 133.4, 130.8, 129.1, 128.5, 128.0, 127.1, 127.0, 126.3, 124.7, 123.8, 122.3, 121.0, 117.6, 112.9, 112.7, 103.3. IR (KBr) νmax/cm−1 1745 (C
O), 1621 (C
C), 1282 (C–O); HRMS (ESI) calcd for C21H13O3 313.0860 (M + H+); found 313.0870.
8-Fluoro-2-(naphthalen-2-yl)-4H-furo[3,2-c]chromen-4-one (4b)
White solid (257.45 mg, 78%); mp 238–239 °C. 1H NMR (600 MHz, CDCl3) δ 8.32 (s, 1H), 7.94 (dd, J = 8.0, 3.7 Hz, 2H), 7.87 (d, J = 7.6 Hz, 1H), 7.85 (dd, J = 8.6, 1.7 Hz, 1H), 7.71 (dd, J = 7.7, 2.9 Hz, 1H), 7.55 (pd, J = 6.8, 1.4 Hz, 2H), 7.46 (dd, J = 9.1, 4.3 Hz, 1H), 7.31 (s, 1H), 7.27–7.24 (m, 1H). 13C NMR (100 MHz, CDCl3) δ 160.4, 157.9 (JC–F = 0.93 Hz), 157.4, 156.2 (JC–F = 2.75 Hz), 148.9 (JC–F = 2.05 Hz), 133.6, 133.4, 129.1, 128.6, 128.0, 127.2, 127.2, 126.1, 124.0, 122.2, 119.2 (JC–F = 8.56 Hz), 118.2 (JC–F = 24.47 Hz), 113.6, (JC–F = 9.66 Hz), 113.5, 106.9 (JC–F = 25.64 Hz), 103.43. 19F NMR (376 MHz, CDCl3) δ −116.22. IR (KBr) νmax/cm−1 1740 (C
O), 1615 (C
C), 1280 (C–O); HRMS (ESI) calcd for C21H12FO3 331.0765 (M + H+); found 331.0765.
Author contributions
Simra Faraz contributed 80% of this research work and the remaining 20% was done by Ahmad Ali.
Conflicts of interest
There are no conflicts of interest to declare.
Acknowledgements
Simra Faraz thanks CSIR, New Delhi, for her research fellowship [sanction number: 09/0731(13234)/2022-EMR-I]. Ahmad Ali is grateful to UGC, New Delhi, for research fellowship [student ID; 201610191889]. We are thankful to the Department of Chemistry, IIT Guwahati, for research facilities. We sincerely thank the Department of Science and Technology, New Delhi, for financial support (grant number: CRG/2022/002751/OC) to ATK. We acknowledge the North East Centre for Biological Sciences and Healthcare Engineering, IIT Guwahati, Department of Biotechnology, Government of India, for project number BT/COE/34/SP28408/2018 for use of the NMR-instrumentation facility.
References
-
(a) V. L. M. Silva, R. Silva-Reis, A. Moreira-Pais, T. Ferreira, P. A. Oliveira, R. Ferreira, S. M. Cardoso, J. Sharifi-Rad, M. Butnariu, M. A. Costea and I. Grozea, Chin. Med., 2022, 17, 145 CrossRef CAS;
(b) J.-C. Jung and O.-S. Park, Molecules, 2009, 14, 4790–4803 CrossRef CAS;
(c) M. M. Abdou, R. A. El-Saeed and S. Bondock, Arabian J. Chem., 2019, 12, 88–121 CrossRef CAS;
(d) W. M. Barker, M. A. Hermodson and K. P. Link, J. Med. Chem., 1971, 14, 167–169 CrossRef CAS;
(e) S. Emami and S. Dadashpour, Eur. J. Med. Chem., 2015, 102, 611–630 CrossRef CAS.
- J. C. Capra, M. P. Cunha, D. G. Machado, A. D. E. Zomkowski, B. G. Mendes, A. R. S. Santos, M. G. Pizzolatti and A. L. S. Rodrigues, Eur. J. Pharmacol., 2010, 643, 232–238 CrossRef CAS PubMed.
- G. Melagraki, A. Afantitis, O. Igglessi-Markopoulou, A. Detsi, M. Koufaki, C. Kontogiorgis and D. J. Hadjipavlou-Litina, Eur. J. Med. Chem., 2009, 44, 3020–3026 CrossRef CAS PubMed.
- J.-C. Jung, Y.-J. Jung and O.-S. Park, Synth. Commun., 2001, 31, 1195–1200 CrossRef CAS.
- C.-M. Lin, S.-T. Huang, F.-W. Lee, H.-S. Kuo and M.-H. Lin, Bioorg. Med. Chem., 2006, 14, 4402–4409 CrossRef CAS PubMed.
- S. K. Konidala, V. Kotra, R. C. S. R. Danduga and P. K. Kola, Bioorg. Chem., 2020, 104, 104207 CrossRef CAS.
-
R. O′. Kennedy and R. D. Thornes, Coumarins: biology, applications, and mode of action, John Wiley & Sons, Chichester, New York, 1997 Search PubMed.
- X. Sun, T. Liu, J. Sun and X. Wang, RSC Adv., 2020, 10, 10826–10847 RSC.
- F. Annunziata, C. Pinna, S. Dallavalle, L. Tamborini and A. Pinto, Int. J. Mol. Sci., 2020, 21, 4618 CrossRef CAS.
- B. H. Lipshutz, Chem. Rev., 1986, 86, 795–819 CrossRef CAS.
- Z.-Z. Wu, M. Rao, S. Xu, H.-Y. Hu and Q.-Z. Tang, Free Radical Res., 2020, 54, 629–639 CrossRef CAS PubMed.
- B. Banerji, S. K. Killi, A. Katarkar, S. Chatterjee, Y. Tangella, C. Prodhan and K. Chaudhuri, Bioorg. Med. Chem., 2017, 25, 202–212 CrossRef CAS PubMed.
-
(a) N. Hegmann, L. Prusko, N. Diesendorf and M. R. Heinrich, Org. Lett., 2018, 20, 7825–7829 CrossRef CAS;
(b) F. Taghavi, A. Khojastehnezhad, R. Khalifeh, M. Rajabzadeh, F. Rezaei, K. Abnous and S. M. Taghdisi, New J. Chem., 2021, 45, 15405–15414 RSC.
-
(a) S. Faraz, M. Kumar, A. Taleb Khan, S. Ponneganti and P. Radhakrishnanand, Tetrahedron Lett., 2023, 115, 154283 CrossRef CAS;
(b) S. Faraz and A. T. Khan, Synthesis, 2023, 55, 3195–3203 CrossRef CAS;
(c) S. Mondal, S. Sarkar, S. S. Ghosh and A. T. Khan, Eur. J. Org. Chem., 2022, 2022, e202200355 CrossRef CAS.
-
(a) V. B. Pendem and M. Nannapaneni, Phosphorus, Sulfur Silicon Relat. Elem., 2020, 195, 485–490 CrossRef CAS;
(b) M. A. Chari, D. Shobha and K. Mukkanti, Catal. Commun., 2006, 7, 787–790 CrossRef CAS;
(c) W. H. Miles, C. F. Nutaitis and C. A. Anderton, J. Chem. Educ., 1996, 73, 272 CrossRef CAS;
(d) R. Tomifuji, K. Maeda, T. Takahashi, T. Kurahashi and S. Matsubara, Org. Lett., 2018, 20, 7474–7477 CrossRef CAS PubMed.
-
(a) G. Cheng and Y. Hu, Chem. Commun., 2007, 3285–3287 RSC;
(b) L. Chen, Y. Li and M.-H. Xu, Org. Biomol. Chem., 2010, 8, 3073–3077 RSC.
- D. Zha, H. Li, S. Li and L. Wang, Adv. Synth. Catal., 2017, 359, 467–475 CrossRef CAS.
-
(a) A. Dey and A. Hajra, Org. Biomol. Chem., 2017, 15, 8084–8090 RSC;
(b) X. Chang, P. Zeng and Z. Chen, Eur. J. Org. Chem., 2019, 6478–6485 CrossRef CAS;
(c) A. Jana, D. Ali, P. Bhaumick and L. H. Choudhury, J. Org. Chem., 2022, 87, 7763–7777 CrossRef CAS PubMed.
- G. Westphal, M. Wallrabe and T. Schäfer, J. Phys. Chem. C, 2020, 124, 799–804 CrossRef CAS.
-
A. I. Vogel and B. S. Furniss, Vogel, A. I. Vogel's Textbook of Practical Organic Chemistry, Longman Scientific & Technical, Wiley, London : New York, 5th edn., 1989 Search PubMed.
- B. Swanson and V. W. Laurie, J. Phys. Chem., 1965, 69, 244–250 CrossRef.
Footnotes |
† This research paper is dedicated to Professor Sukh Dev on his 100th birthday for his valuable and immense scientific contribution to terpenoids chemistry and their structure elucidation, in addition to being an excellent and inspirational teacher. |
‡ Electronic supplementary information (ESI) available. CCDC 2286699. For ESI and crystallographic data in CIF or other electronic format see DOI: https://doi.org/10.1039/d3ob01721d |
|
This journal is © The Royal Society of Chemistry 2024 |