DOI:
10.1039/D3NJ03273F
(Paper)
New J. Chem., 2024,
48, 237-243
Synthesis of novel chromeno[1,6]naphthyridine derivatives in PEG-400 via catalyst-free, one-pot, and multicomponent reactions†
Received
13th July 2023
, Accepted 15th November 2023
First published on 15th November 2023
Abstract
An efficient, one-pot, and catalyst-free pathway was reported for the synthesis of novel chromeno[1,6]naphthyridine derivatives via a multicomponent condensation reaction of salicylaldehyde derivatives, malononitrile dimer, and active methylene compounds. All the reactions were performed in polyethylene glycol-400 as a green solvent at 80 °C. The reactions proceeded with a high-atom economy, and new products were synthesized with good yields and a simple work-up procedure, with water as the only by-product.
Introduction
Heterocycles, found in many organic compounds, have attracted much attention because of their use in various fields of science, especially in medicine. Naphthyridines constitute a family of heterocyclic compounds that have been used not only as luminescent materials in molecular recognition but also as anticancer active screening agents in new drug discovery.1 These natural or synthetic heterocyclic compounds exist as six isomers with different locations of nitrogen atoms.2 Among them, the 1,6-naphthyridine nucleus has attracted particular attention in recent years, due to the wide range of biological activities shown by its derivatives.3 A tremendous volume of research on 1,6-naphthyridines has been conducted in the last decade, yielding versatile, simple, eco-friendly, and nonconventional synthetic routes and highlighting the significance of 1,6-naphthyridines in medicinal chemistry, notably in cancer treatment. For example, AMG 337 (1, antagonist to the receptor tyrosine kinase mesenchymal epithelial transition factor in PC3 cells),4 GENZ-644282 (2, inhibits lung cancer, renal carcinoma, colon cancer, and melanoma),5 BAY-94-8862 (3, nonsteroidal antagonist of mineralocorticoid receptor),6 and aaptamine (4, marine natural compound displaying antimicrobial, antifungal, and antiretroviral activities)7 are among the 1,6-naphthyridine derivatives under clinical trials for cancer chemotherapy (Fig. 1).
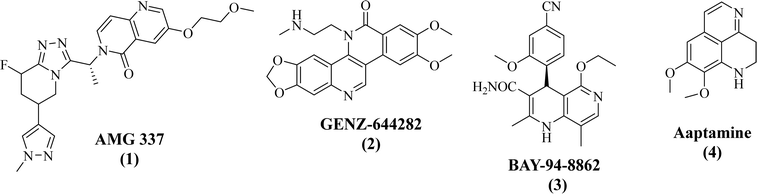 |
| Fig. 1 Examples of essential natural products with a naphthyridine core hat are being tested in clinical trials for cancer chemotherapy. | |
Moreover, coumarin-fused heterocycles are among the most exciting and widely used compounds because of their applications, including activities against tumors,8 HIV9 viruses,10 bacteria,11 and fungi.11 Therefore, it can be predicted that integrating chromene moieties with (1,6)-naphthyridines in a single molecular framework may enhance their biological and fluorescence properties. In 2010, Hui et al. reported, for the first time, a silica-catalyzed synthesis of chromeno[4,3,2-de][1,6]naphthyridine derivatives via a one-pot cascade reaction of malononitrile with substituted 2-hydroxyacetophenone and aromatic aldehydes in aqueous media.12 Subsequently, other researchers reported this reaction under new catalytic and temperature conditions.13 In 2021, Wang et al. reported a novel approach for the synthesis of chromeno[4,3,2-de][1,6]naphthyridine derivatives from a wide range of 2-hydroxybenzaldehydes with alkyl 3-oxo-3-substituted propanoates and malononitrile via a propionic-acid-promoted cascade Knoevenagel condensation/Michael addition/intramolecularly nucleophilic addition accompanied by oxidative aromatization.14
Recently, Elinson et al. reported the synthesis of benzo[b]chromeno[4,3,2-de][1,6]naphthyridines via an intermolecular oxidative cyclization of 5-(2-hydroxy-6-oxocyclohexyl)-5H-chromeno[2,3-b]pyridines in formic acid.15
In this line of research and in continuation of our research on the synthesis of novel heterocycles,16 herein, we report an efficient method for the synthesis of chromeno[1,6]naphthyridine derivatives via a one-pot, three-component condensation reaction between salicylaldehyde derivatives, malononitrile dimer, and active methylene group compounds (5-amino-3-(cyanomethyl)-1H-pyrazole-4-carbonitrile/5-amino-3-(cyanomethyl)isoxazole-4 carbonitrile/2-(cyanomethyl) pyrazolo [1,5-a]pyrimidine-3-carbonitrile derivatives/malononitrile dimer/alkyl malonates) in polyethylene glycol-400 (PEG-400) at 80 °C (Scheme 1). This new nominally catalyst-free pathway provided more than 30 previously (to the best of our knowledge) undescribed chromeno[1,6]naphthyridine derivatives with acceptable yields in PEG-400, which appeared to play a dual role of solvent and catalyst.
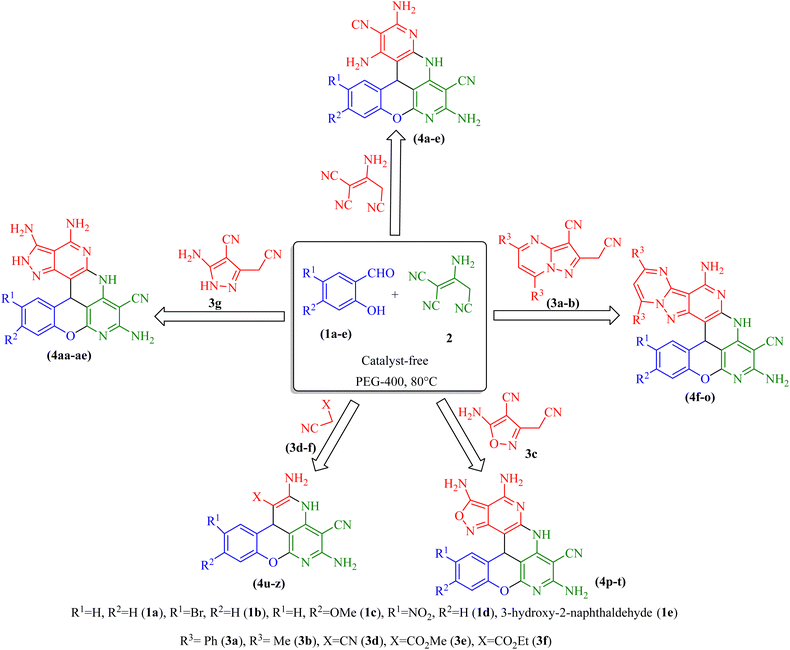 |
| Scheme 1 One-pot, three-component synthesis of chromeno[1,6]naphthyridine derivatives via a condensation reaction between salicylaldehyde derivatives, malononitrile dimer, and compounds containing the active methylene groups 3a–g in PEG-400 at 80 °C. | |
Results and discussion
Pyrazole 3g, pyrazolo[1,5-a]pyrimidines 3a–b and isoxazole 3c were prepared according to the previously reported procedure (Scheme 2).17 The details of the reaction are described in the general procedure section.
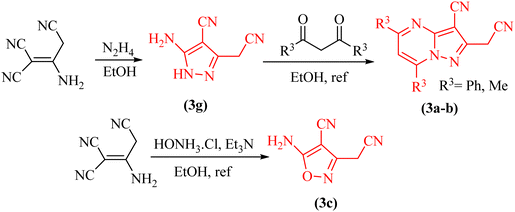 |
| Scheme 2 Preparation of a series of pyrazole, isoxazole and pyrazolo[1,5-a]pyrimidine derivatives in EtOH at reflux conditions. | |
To optimize the reaction conditions for the synthesis of the chromeno[1,6]naphthyridine derivatives, salicylaldehyde (1a, 1 mmol) and malononitrile dimer (2, 2 mmol) were chosen as the substrates for the model reaction to form the corresponding chromeno[1,6]naphthyridine (4a). Various solvents (H2O, EtOH, dimethylformamide [DMF], dimethyl sulfoxide [DMSO], PEG-400, EtOAc, and CHCl3) and temperatures (Table 1) were tested. As shown in Table 1, in the protic solvent H2O at room temperature, the desired product was not formed after 24 hours of the reaction time, whereas under reflux conditions, the product was obtained with 30% efficiency. This result showed temperature to be an influential factor for product formation. Moreover, similar results were obtained in ethanol at room temperature and reflux conditions (Table 1, entries 1–4). When performing the reaction instead in the low-polarity solvents EtOAc, CH3CN, and tetrahydrofuran, under reflux conditions, there was no significant improvement in the reaction efficiency in each case (Table 1, entries 5–7). In the aprotic polar solvents DMSO and DMF, at 80 °C, the yield was improved and the product was obtained with moderate efficiency levels of 60% and 54%, respectively (Table 1, entries 8 and 9). Surprisingly, when the reaction was conducted in PEG-400 at 80 °C, the product was isolated with an efficiency of 92%, whereas the reaction time was reduced to 10 hours. As previously reported in the reactions without a catalyst in polyethylene glycol, this solvent appeared to increase the reaction rate by forming hydrogen bonds with atoms prone to participating in this bond formation in the reaction environment.18 When increasing the temperature to 90 °C and reaction time to 15 hours, the efficiency did not change, although decreasing the temperature to 60 °C afforded o a 7% decrease in the yield (Table 1, entries 10–12).
Table 1 Optimization of the reaction conditions in the synthesis of chromeno[1,6]naphthyridine (4a)a
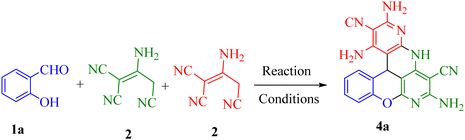
|
Entry |
Solvent |
Temperature (°C) |
Time (h) |
Yieldb (%) |
Reaction conditions: 1a (1 mmol), 2 (2 mmol), solvent (3 mL).
Isolated yield.
Incomplete reaction with several unidentified spots on TLC.
|
1 |
H2O |
Rt |
24 |
—c |
2 |
H2O |
Reflux |
24 |
30 |
3 |
EtOH |
Rt |
24 |
—c |
4 |
EtOH |
Reflux |
24 |
25 |
5 |
EtOAc |
Reflux |
24 |
37 |
6 |
CH3CN |
Reflux |
24 |
25 |
7 |
THF |
Reflux |
24 |
30 |
8 |
DMSO |
80 |
24 |
60 |
9 |
DMF |
80 |
24 |
54 |
10 |
PEG-400 |
80 |
10 |
92 |
11 |
PEG-400 |
90 |
15 |
92 |
12 |
PEG-400 |
60 |
10 |
85 |
With optimal conditions in hand, some salicylaldehyde derivatives (Scheme 3, 1a–e) were allowed to react with 2-aminopropene-1,1,3-tricarbonitrile 2 and 2-(cyanomethyl)pyrazolo[1,5-a]pyrimidine-3-carbonitrile derivatives (Scheme 3, 3a and 3b) (or 5-amino-3-(cyanomethyl)isoxazole-4-carbonitrile 3c/alkyl malonates 3d–f/5-amino-3-(cyanomethyl)-1H-pyrazole-4-carbonitrile 3g/malononitrile dimer) motifs. The corresponding chromeno[1,6]naphthyridine products 4a-ae were afforded in high yields. The structures of the synthesized products are shown in Scheme 4.
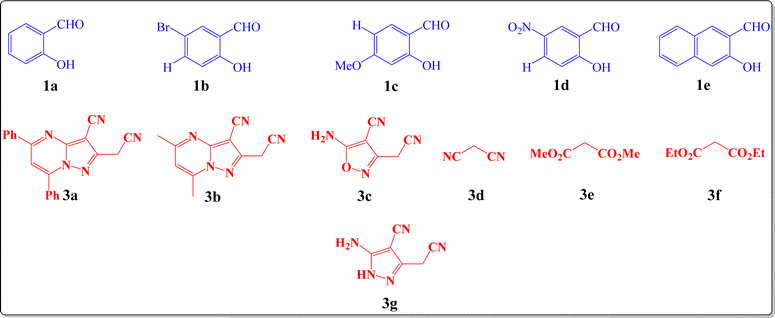 |
| Scheme 3 Depictions of the structures of the used salicylaldehyde derivatives 1a–e and active methylene compounds 3a–g. | |
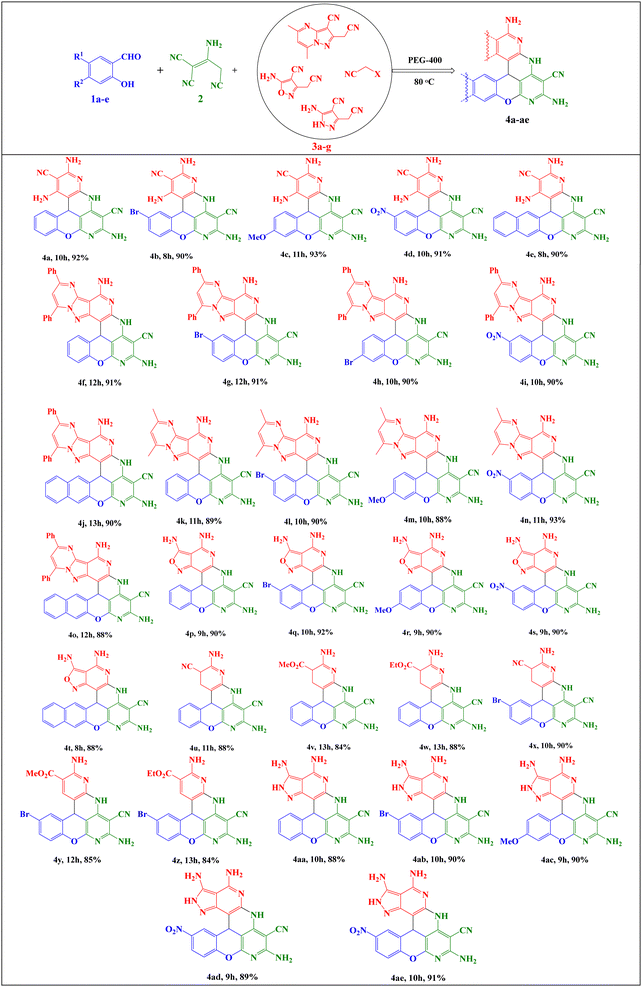 |
| Scheme 4 One-pot, three-component synthesis of chromeno[1,6]naphthyridine derivatives. a Reaction conditions: 1a–e (1 mmol), 2 (1 mmol), 3a–g (1 mmol), PEG-400 (3 mL), 80 °C. The yields refer to the isolated pure products. | |
The results showed that the presence of electron-withdrawing and electron-donating groups on the aromatic ring of salicylaldehyde, as well as the type of active methylene compound, did not have much effect on the reaction efficiency, since the products were obtained with similar efficiencies and reaction times.
Based on the current results and the mechanistic data on the multicomponent formation of the chromenopyridine moiety from carbonyl compounds and C–H acids,19 a plausible mechanism was derived. With the synthesis of product 4a as an example (Scheme 5), the initial step according to this mechanism is the formation of Knoevenagel adduct A from substrate 1a and 2-aminoprop-1-ene-1,1,3-tricarbonitrile. Next, nucleophilic attack by compound 2 on intermediate A yielded intermediate B,20 followed by several consecutive intramolecular cyclization reactions passing through intermediates C to F21,22 to form the final product 4a. The accelerating role of polyethylene glycol at different stages of the reaction was posited to involve the formation of hydrogen bonds with the nitrogen atom of the cyanide group or the oxygen of the carbonyl group.
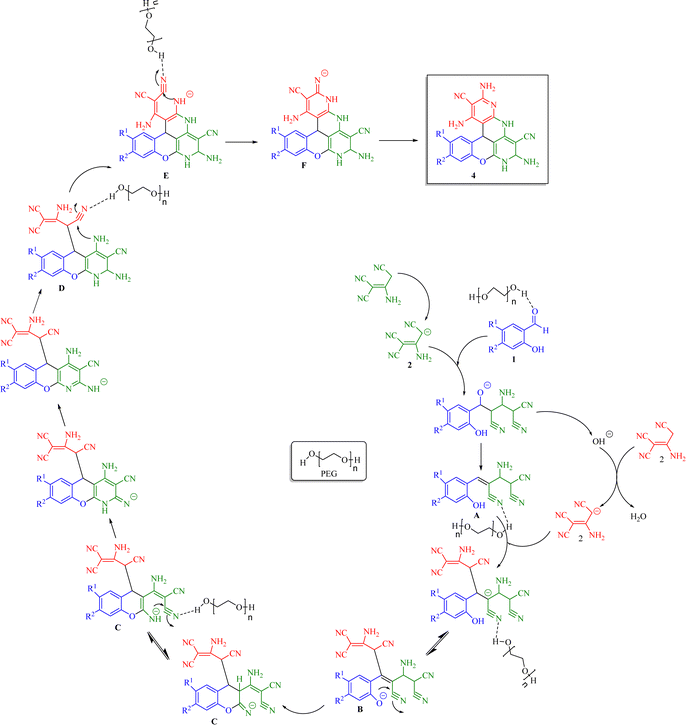 |
| Scheme 5 Proposed mechanism for the synthesis of chromeno[1,6]naphthyridine derivatives. | |
Experimental
Chemicals were purchased from Merck and Aldrich Chemical Companies. Proton nuclear magnetic resonance spectra were recorded using a Bruker (400 MHZ) Avance Ultrashield spectrometer for samples each in pure deuterated DMSO-d6 solvent with tetramethylsilane as an internal standard. Mass spectra were recorded using a FINNIGANMAT 8430 mass spectrometer operating at 70 eV. FTIR spectroscopy (Shimadzu FTIR 8300 spectrophotometer) was employed for the characterization of the products. Melting points were determined in open capillary tubes using a Barnstead Electrothermal 9100 BZ circulating oil melting point apparatus. Reaction monitoring was accomplished using thin layer chromatography (TLC) on silica gel PolyGram SILG/UV254 plates.
General procedure for the synthesis of chromeno[1,6]naphthyridine
Compounds 3a–e (1 mmol) were each added to a stirred mixture of salicylaldehyde derivatives (1 mmol) and malononitrile dimer (1 mmol) in PEG-400 (3 mL), and each reaction mixture was stirred at 80 °C to complete the formation of the corresponding chromeno[1,6]naphthyridine (monitored using TLC). After the completion of each reaction, water (3 mL) was added to the reaction mixture; the precipitate was filtrated and recrystallized from hot ethanol to afford the pure product.
Conclusions
We reported a highly efficient method for the synthesis of chromeno[1,6]naphthyridine derivatives each via a one-pot, three-component condensation reaction in PEG-400 as a green and biodegradable polymeric solvent at 80 °C. The reactions displayed attractive features, including a simple and practical operation, and their furnishing products with excellent yields.
Conflicts of interest
There are no conflicts to declare.
Acknowledgements
The authors thank the Persian Gulf University Research Councils for their financial support to this work.
Notes and references
-
(a) W. B. Lu, L. H. Zhang, X. S. Ye, J. C. Su and Z. X. Yu, Tetrahedron, 2006, 62, 1806–1816 CrossRef CAS;
(b) A. L. Ruchelamn, S. K. Singh, A. Ray, X. H. Wu, J. M. Yang, A. Liu, L. F. Liu and E. J. Lavoie, Bioorg. Med. Chem., 2003, 11, 2061 CrossRef PubMed;
(c) J. Bedard, S. May, L. L’Heureux, T. Stamminger, A. Copsey, J. Drach, J. Huffman, L. Chan, H. Jin and R. F. Rando, Antimicrob. Agents Chemother., 2000, 44, 929 CrossRef CAS.
-
P. A. Lowe, Comprehensive Heterocyclic Chemistry, Pergamon Press, Oxford, UK, 1984, vol. 1 Search PubMed.
-
(a) T. Devadoss, V. Sowmya and R. Bastati, ChemistrySelect, 2021, 15, 3610–3641 CrossRef;
(b) N. Ghobadi, N. Nazari and P. Gholamzadeh, Adv. Heterocycl. Chem., 2020, 132, 85–134 CrossRef CAS.
- P. E. Hughes, K. Rex, S. Caenepeel, Y. Yang, Y. Zhang, M. A. Broome and A. Coxon, Mol. Cancer Ther., 2016, 15, 1568–1579 CrossRef CAS PubMed.
- L. S. Kurtzberg, S. Roth, R. Krumbholz, J. Crawford, C. Bormann, S. Dunham and B. A. Teicher, Clin. Cancer Res., 2011, 17, 2777–2787 CrossRef CAS PubMed.
- A. Ouvrard-Pascaud, A. Gueret, J. Thireau, L. Nicol, L. Fazal, C. Delcayre and P. Mulder, Eur. Heart J., 2016, 37, 111 Search PubMed.
- E. L. Larghi, M. L. Bohn and T. S. Kaufman, Tetrahedron, 2009, 65, 4257–4282 CrossRef CAS.
- M. Suzuki, K. Nakagawa-Goto, S. Nakamura, H. Tokuda, S. L. Morris-Natschke, M. Kozuka, H. Nishino and K.-H. Lee, Pharm. Biol., 2006, 44, 178 CrossRef CAS.
- A. M. Almerico, P. Diana, P. Barraja, G. Dattolo, F. Mingoia, A. G. Loi, F. Scintu, C. Milia, I. Puddu and P. La Colla, Il Farmaco, 1998, 53, 33–40 CrossRef CAS.
- C. A. Kontogiorgis and D. J. Hadjipavlou-Litina, J. Med. Chem., 2005, 48, 6400–6408 CrossRef CAS PubMed.
- O. Kayser and H. Kolodziej, Z. Naturforschung C, 1999, 54, 169–174 CrossRef CAS PubMed.
- W. Hui, L. Wei, W. Yu, X. Hai-Qiang, S. Da-Qing, S. Yan-Hui, Y. Rui, B. Rong-Cheng and Y. Wei, J. Comb. Chem., 2010, 12, 31–34 CrossRef.
-
(a) D. Anshu, P. Vijay, L. G. Shyam, S. Amit, S. R. Kuldeep, S. Amit and J. Astha, Catal. Commun., 2015, 61, 88–91 CrossRef;
(b) Z. Khosravi, H. Mohammadi and H. R. Shaterian, J. Chin. Chem. Soc., 2019, 66, 1641–1648 CrossRef CAS;
(c) F. Shirzaei and H. R. Shaterian, ChemistrySelect, 2021, 6, e20210309112 CrossRef.
- J. Gan, N. Luo, C. Wu, X. Wan and C. Wang, ChemistrySelect, 2021, 6, 9032–9037 CrossRef CAS.
- Y. E. Ryzhkova, F. V. Ryzhkov, A. N. Fakhrutdinov and M. N. Elinson, Molecules, 2022, 27, 4156 CrossRef CAS.
-
(a) A. Hasaninejad and M. Beyrati, RSC Adv., 2018, 8, 1934–1939 RSC;
(b) M. Karami, A. Hasaninejad, H. Mahdavi, A. Iraji, S. Mojtabavi, M. A. Faramarzi and M. Mahdavi, Mol. Divers., 2021, 1–13 Search PubMed;
(c) Z. Rafiee Samani, A. Mehranpour and A. Hasaninejad, J. Heterocycl. Chem., 2020, 57, 2150–2156 CrossRef CAS.
-
(a) E. C. Taylor and K. S. Hartke, J. Am. Chem. Soc., 1959, 81, 2456–2464 CrossRef CAS;
(b) R. Aggarwal, V. Kumar, R. Kumar and S. P. Singh, Beilstein J. Org. Chem., 2011, 7, 179–197 CrossRef CAS;
(c) A. M. Semenova, R. V. Oganesyan, V. V. Dotsenko, E. A. Chigorina, N. A. Aksenov, I. V. Aksenova and E. E. Netrebae, Russ. J. Gen. Chem., 2019, 89, 19–24 CrossRef CAS.
-
(a) A. Maryamabadi, A. Hasaninejad, N. Nowrouzi, G. Mohebbi and B. Asghari, Bioorg. Med. Chem., 2016, 24, 1408 CrossRef CAS PubMed;
(b) N. V. Shitole, S. B. Sapkal, B. B. Shingate and M. S. Shingare, Bull. Korean Chem. Soc., 2011, 32, 35 CrossRef CAS.
-
(a) M. N. Elinson, S. V. Gorbunov, A. N. Vereshchagin, R. F. Nasybullin, A. S. Goloveshkin, I. S. Bushmarinov and M. P. Egorov, Tetrahedron, 2014, 70, 8559 CrossRef CAS;
(b) M. N. Elinson, F. V. Ryzhkov, A. N. Vereshchagin, T. A. Zaimovskaya, V. A. Korolev and M. P. Egorov, Mendeleev Commun., 2016, 26, 399 CrossRef CAS.
- M. N. Elinson, A. N. Vereshchagin, Y. E. Anisina, A. N. Fakhrutdinov, A. S. Goloveshkin and M. P. Egorov, J. Fluorine Chem., 2018, 213, 31–36 CrossRef CAS.
- M. N. Elinson, A. N. Vereshchagin, Y. E. Anisina, A. S. Goloveshkin, I. E. Ushakov and M. P. Egorov, Russ. Chem. Bull., 2018, 67, 1695–1703 CrossRef CAS.
- S. Oliveira-Pinto, O. Pontes, D. Lopes, B. Sampaio-Marques, M. D. Costa, L. Carvalho, C. S. Goncalves, B. M. Casta, P. Maciel, P. Ludovico, F. Baltazar, F. Proenca and M. Costa, Bioorg. Chem., 2020, 100, 103942 CrossRef CAS PubMed.
|
This journal is © The Royal Society of Chemistry and the Centre National de la Recherche Scientifique 2024 |