DOI:
10.1039/D4NA00284A
(Paper)
Nanoscale Adv., 2024,
6, 3948-3960
A multidentate copper complex on magnetic biochar nanoparticles as a practical and recoverable nanocatalyst for the selective synthesis of tetrazole derivatives†
Received
4th April 2024
, Accepted 10th June 2024
First published on 12th June 2024
Abstract
Waste recycling, novel and easy methods of recycling catalysts, use of green solvents, use of selective catalysts and preventing the production of by-products are the most important principles of green chemistry and modern technology. Therefore, in this work, biochar nanoparticles (B-NPs) were synthesized by the pyrolysis of chicken manure as a novel method for waste recycling. Subsequently, the B-NPs were magnetized by Fe(0) nanoparticles to improve the easy recovery of biochar. Then, the surface of biochar magnetic nanoparticles (FeB-MNPs) was modified by (3-chloropropyl)trimethoxysilane (3Cl-PTMS). Finally, a multidentate copper complex of 2,2′-(propane-1,3-diylbis(oxy))dianiline (P.bis(OA)) was immobilized on the surface of modified FeB-MNPs, which was labeled as Cu-P.bis(OA)@FeB-MNPs. Cu-P.bis(OA)@FeB-MNPs was investigated as a commercial, homoselective, practical, and recyclable nanocatalyst in the synthesis of 5-substituted-1H-tetrazole compounds through the [3 + 2] cycloaddition of sodium azide (NaN3) and organo-nitriles in polyethylene glycol 400 (PEG-400) as a green solvent. Cu-P.bis(OA)@FeB-MNPs was characterized using wavelength dispersive X-ray (WDX) spectroscopy, scanning electron microscopy (SEM), thermogravimetric analysis (TGA), energy-dispersive X-ray spectroscopy (EDS), vibrating-sample magnetometer (VSM), atomic absorption spectroscopy (AAS) and N2 adsorption–desorption (Brunauer–Emmett–Teller (BET) method) techniques. Cu-P.bis(OA)@FeB-MNPs was recovered and reused for several runs in the synthesis of tetrazoles.
1. Introduction
Chemical sciences is one of the most important fields for development in the world, which provides many applications in the fields of medicine and various industries. However, unfortunately, waste chemical materials have also been introduced into the environment during the growth of chemical science. Therefore, principles of green chemistry were introduced, which minimize the environmental damage from chemical industries and laboratories. One of the principles of green chemistry is the use of recyclable catalysts.1–5 Acids, bases, and transition metals or metal complexes are among the most well-known types of catalysts. In general, catalysis systems are divided into two categories: homogeneous catalysis systems and heterogeneous catalysis systems.6 Homogeneous catalysts are known to exhibit several properties such as high performance, selectivity, instability, difficult to recover, and time-consuming product purification.7,8 On the other side, heterogeneous catalysts are known to exhibit different properties such as good recoverability, high stability, low efficiency, and selectivity.7,8 Recently, nanocatalysts have emerged as a new category of catalyst systems that act as the bridge between homogeneous and heterogeneous catalysts and have advantages over both homogeneous and heterogeneous catalysts.6,8–12 Because decreasing the particle size provides a high surface area, the catalytic activity and selectivity are increased (e.g., homogeneous catalysts). In addition, nanomaterials have high stability and are heterogeneous in nature, enabling them to be easily recovered and reused like heterogeneous catalysts. In this context, various nanoparticles such as boehmite,13–15 mesoporous silica materials,16–18 graphene oxide,19–21 MOF compounds,22–31 carbon nanostructures,32,33 polymers,34 biochar,35,36 and magnetic particles37 have been reported as catalysts or catalyst supports. However, most of these materials are synthesized from mineral and non-renewable chemical compounds, which are against the principles of green and modern chemistry. Raw materials for biochar production include wood chips, tree bark, plant residues, animal manure, and organic wastes.38–40 As is known, the use of renewable materials and waste recycling are other principles of green chemistry.41,42 The synthesis of biochar nanoparticles from natural and renewable sources is very important in expanding its application. Considering the increasing importance of the catalysts in various industries and laboratories, the introduction of the catalysts (e.g. biochar) made from renewable sources is a necessity for the future. On the other hand, the increase in the population has led to the accumulation of a large amount of waste, which has become a challenge for the planet and the future of mankind. Therefore, recently, the technology of recycling waste and turning waste into valuable materials is of special interest, so that one of the principles of green chemistry is dedicated to this challenge. Considering that biochar synthesis is a novel method for waste recycling, it doubles the importance of expanding the use of biochar. Therefore, in this work, biochar nanoparticles were synthesized by the pyrolysis of chicken manure as a method for recycling agricultural waste. In fact, biochar is stable carbon black, and its surface is covered by C
O, COOH, and OH groups that provide a modifiable surface for the immobilization of catalyst species.43–47 Despite the special advantages of biochar, it has rarely been reported as a catalyst or catalyst support. Despite the unique advantages of biochar, the separation and recycling of biochar nanoparticles require time-consuming and difficult methods such as centrifugation and filtration. This limitation can be overcome by biochar magnetic nanocomposites as a new and ideal methodology, which can be easily separated by an external magnet.48–51 For example, in 2024 biochar was magnetized by magnetic Fe3O4 nanoparticles36 and magnetic Ni nanoparticles.48 But, biochar, and Fe(0) particles – with high surface area and stability – have rarely been used for the magnetization of materials.52 Therefore, in this work, a complex of copper on magnetic biochar nanoparticles (Cu-P.bis(OA)@FeB-MNPs) was prepared as a new recyclable nanocatalyst for the homoselective synthesis of 5-substituted-1H-tetrazole compounds through [3 + 2] the cycloaddition of NaN3 and organo-nitriles in PEG-400 as a green solvent. This is the first report on a copper complex of 2,2′-(propane-1,3-diylbis(oxy))dianiline on magnetic biochar nanoparticles and its introduction as a green catalyst in the synthesis of organic compounds.
2. Experimental
2.1. Preparation of biochar magnetic nanoparticles (FeB-MNPs)
Biochar nanoparticles were formed by simple pyrolysis of chicken manure. In this context, 500 g of dried chicken manure was heated at 400 °C as the pyrolysis temperature for 1 h under N2 sweeping. At the end of pyrolysis, the heating was stopped and the Chinese crucibles were cooled with N2 sweep. The resulting black solid was biochar. Then, 4.5 g of the synthesized biochar was mixed with FeCl2·4H2O (5.34 g) in ethanol (25 mL) and H2O (5 mL), and then, it was stirred at room temperature for 15 min. Then, NaBH4 (2.5 g in 70 mL of H2O) as a reduction agent was injected into the mixture dropwise within 20 min. The obtained mixture was stirred at room temperature for 15 min. The magnetic powder (biochar magnetic nanoparticles) was filtered by magnetic decantation, washed with ethanol and dried for 6 h at 90 °C.52
2.2. Modification of FeB-MNPs with (3-chloropropyl)trimethoxysilane (3Cl-PTMS)
FeB-MNPs (1 g) were dispersed in n-hexane (25 mL) for 30 min by an ultrasonic bath. Then, (3-chloropropyl)trimethoxysilane (1.5 mL) was injected into it and was allowed to stir for 24 h under reflux conditions. After 24 h, the mixture was cooled to room temperature, and the modified FeB-MNPs (3Cl-PTMS@FeB-MNPs) were separated by magnetic decantation and washed with ethanol. The modified FeB-MNPs were dried at 60 °C (Scheme 1).
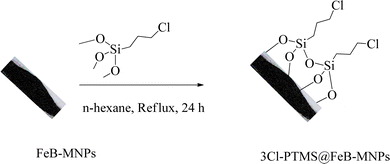 |
| Scheme 1 Synthesis of 3Cl-PTMS@FeB-MNPs. | |
2.3. Functionalization of FeB-MNPs with P.bis(OA) (P.bis(OA)@FeB-MNPs)
FeB-MNPs (1 g) was mixed with P.bis(OA) (1 mmol) and triethylamine (8 mmol) in toluene (10 mL) and it was dispersed for 25 min in an ultrasonic bath. Then, the mixture was stirred for 72 h under reflux conditions. The mixture was allowed to cool to room temperature. After that, the functionalized FeB-MNPs with P.bis(OA) (P.bis(OA)@FeB-MNPs) were filtered by magnetic decantation and then washed 5 times with dimethyl sulfoxide (DMSO) and ethanol. The obtained P.bis(OA)@FeB-MNPs were dried at room temperature (Scheme 2).
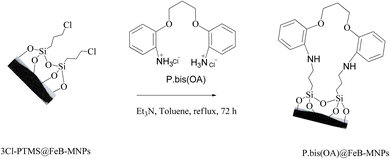 |
| Scheme 2 Synthesis of functionalized FeB-MNPs with P.bis(OA) (P.bis(OA)@FeB-MNPs). | |
2.4. Synthesis of Cu-P.bis(OA)@FeB-MNPs
P.bis(OA)@FeB-MNPs (1 g) was mixed with Cu(NO3)2·9H2O (2 mmol) in 25 mL of ethanol. The mixture was stirred under reflux conditions for 24 h. After that, the mixture was cooled. Then, the prepared final catalyst (Cu-P.bis(OA)@FeB-MNPs) was separated by magnetic decantation and washed several times with water and ethanol to remove excess copper from the mixture. Finally, Cu-P.bis(OA)@FeB-MNPs were kept at a temperature of 50 °C, which was dried (Scheme 3).
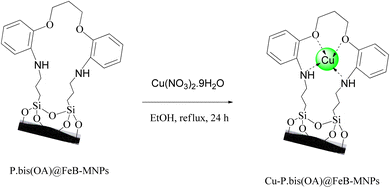 |
| Scheme 3 Synthesis of Cu-P.bis(OA)@FeB-MNPs. | |
2.5. Synthesis of tetrazoles catalyzed by Cu-P.bis(OA)@FeB-MNPs
The catalytic application of Cu-P.bis(OA)@FeB-MNPs was investigated in the synthesis of tetrazoles through a ring addition reaction of nitrile and sodium azide (Scheme 4). A mixture of nitrile (1 mmol), sodium azide (1.4 mmol) in PEG-400 solvent, and 30 mg of Cu-P.bis(OA)@FeB-MNPs was stirred at a temperature of 120 °C. The reaction time was monitored by thin-layer chromatography (TLC) using UV wavelengths of 254 and 356 nm in acetone
:
n-hexane (8
:
2) tank solvent. After completion of the reaction, the reaction mixture was cooled and diluted with ethyl acetate, distilled water, and HCl solution (4 N). Then, the Cu-P.bis(OA)@FeB-MNPs catalyst was isolated from the mixture and washed with ethyl acetate and HCl solution (4 N). After that, tetrazole products were extracted in ethyl acetate solvent. The organic phase was removed by evaporation to obtain 5-substituted 1H-tetrazoles.
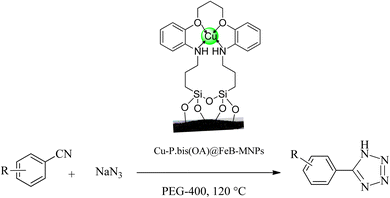 |
| Scheme 4 Synthesis of tetrazoles in the presence of Cu-P.bis(OA)@FeB-MNPs. | |
2.6. NMR spectra of the tetrazoles
2-(1H-Tetrazol-5-yl)benzonitrile.
1H NMR (250 MHz, DMSO): δH = 8.06 (d, J = 5.42 Hz, 2H), 7.92 (t, J = 7.05 Hz, 1H), 7.76 (t, J = 7.12 Hz, 1H) ppm.
4-(1H-Tetrazol-5-yl)benzonitrile.
1H NMR (250 MHz, DMSO): δH = 8.20 (d, J = 8.22 Hz, 2H), 8.08 (d, J = 4.95 Hz, 2H) ppm.
5-(3-Nitrophenyl)-1H-tetrazole.
1H NMR (250 MHz, DMSO): δH = 8.81 (s, 1H), 8.42 (t, J = 8.25 Hz, 2H), 7.88 (t, J = 7.62 Hz, 1H) ppm.
5-Phenyl-1H-tetrazole.
1H NMR (250 MHz, DMSO): δH = 8.04 (m, 2H), 7.59 (m, 3H) ppm.
5-(2-Chlorophenyl)-1H-tetrazole.
1H NMR (250 MHz, DMSO): δH = 7.79 (d, J = 5.90 Hz, 1H), 7.70 (d, J = 6.35 Hz, 1H), 7.58 (m, 2H) ppm.
5-(4-Chlorophenyl)-1H-tetrazole.
1H NMR (250 MHz, DMSO): δH = 8.03 (d, J = 6.65 Hz, 2H), 7.67 (d, J = 6.75 Hz, 2H) ppm.
3. Results and discussion
In this work, a heterogeneous catalyst of copper complex was immobilized on FeB-MNPs. Then, this nanocatalyst was characterized by WDX, SEM, TGA, EDS, VSM, AAS, and BET techniques. Then, its catalytic performance was investigated in the homoselective synthesis of tetrazoles.
3.1. Particle size and morphological identification of Cu-P.bis(OA)@FeB-MNPs by SEM
Fig. 1 shows the SEM images of Cu-P.bis(OA)@FeB-MNPs. As can be seen in SEM images, Cu-P.bis(OA)@FeB-MNPs have similar spherical shapes and uniform diameters of less than 70 nm. Also, the observed agglomeration of its particles in the SEM images is due to the magnetic nature of these nanoparticles.
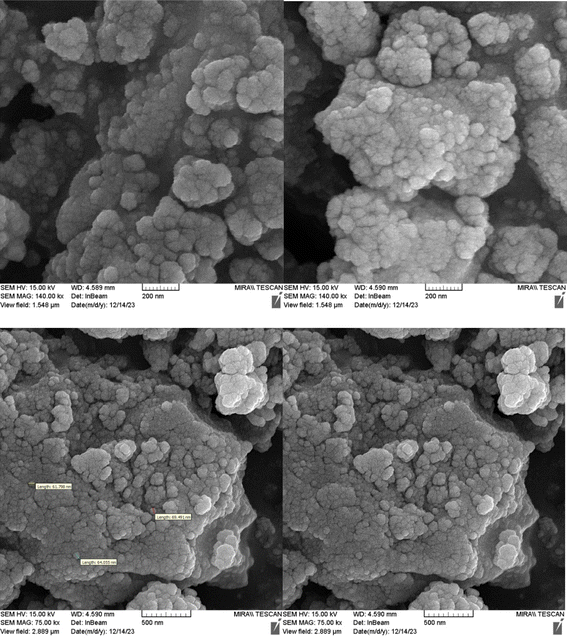 |
| Fig. 1 SEM images of Cu-P.bis(OA)@FeB-MNPs. | |
3.2. Qualitative studying of the elements content of Cu-P.bis(OA)@FeB-MNPs
EDS analysis of Cu-P.bis(OA)@FeB-MNPs is shown in Fig. 2. Magnetic biochar nanoparticles are composed of C, O, and Fe, and after their surface modification, it is functionalized with (3-chloropropyl)trimethoxysilane. Also, the 2,2′-(propane-1,3-diylbis(oxy))dianiline ligand is composed of N, O, and C, which is complexed with Cu metal. Therefore, the composition of Cu-P.bis(OA)@FeB-MNPs must include all these elements. As it is clear in the EDS analysis, all these elements are present in the composition of this catalyst, which indicates the successful synthesis of Cu-P.bis(OA)@FeB-MNPs. All C, O, Si, Cu, and N elements were observed in the catalyst structure. These evidences show that the synthesis of this catalyst was done successfully. As indicated in the EDS diagram, Cu-P.bis(OA)@FeB-MNPs are formed from C, N, O, Si, Fe, and Cu elements. The presence of Si element in this catalyst confirmed that FeB-MNPs were successfully modified by Cl-PTMS. Considering that silicon element was not present in the structure of the magnetic biochar nanoparticles and the elemental composition difference between FeB-MNPs and 3Cl-PTMS@FeB-MNPs is the presence or absence of silicon, therefore, the presence of silicon element in the elemental composition of 3Cl-PTMS@FeB-MNPs shows the successful modification of FeB-MNPs with (3-chloropropyl)trimethoxysilane. The presence of N element in Cu-P.bis(OA)@FeB-MNPs confirmed that the P.bis(OA) ligand was successfully immobilized on the surface of FeB-MNPs. Also, the presence of Cu element confirmed that copper complex was successfully synthesized on the surface of the modified FeB-MNPs.
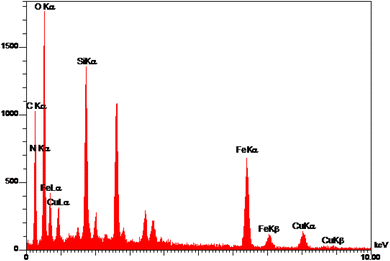 |
| Fig. 2 The EDS diagram of Cu-P.bis(OA)@FeB-MNPs. | |
WDX analysis is another method for the qualitative study of the element content and their distribution in a sample. The WDX images of Cu-P.bis(OA)@FeB-MNPs are shown in Fig. 3. The WDX images confirmed the presence of C, N, O, Si, Fe, and Cu elements, which is in agreement with the obtained results from EDS analysis. In addition, the WDX images show a quite homogeneous distribution of C, N, O, Si, Fe, and Cu elements in the structure of Cu-P.bis(OA)@FeB-MNPs.
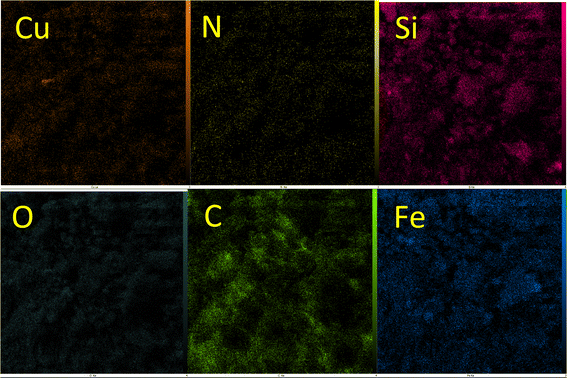 |
| Fig. 3 WDX images of Cu-P.bis(OA)@FeB-MNPs. | |
Because the copper element is the main catalytic active site of Cu-P.bis(OA)@FeB-MNPs, the exact concentration of Cu-metal was determined by AAS analysis, which was found to be 0.72 × 10−3 mol g−1.
3.3. Studying the amount of organic ligand on FeB-MNPs using TGA
The content of Cl-PTMS and P.bis(OA) as organic layers that immobilized on FeB-MNPs was studied by TGA analysis. TGA and differential thermogravimetry (DTG) diagrams of Cu-P.bis(OA)@FeB-MNPs are outlined in Fig. 4. The TGA diagram of Cu-P.bis(OA)@FeB-MNPs indicated three steps of weight loss. The first of them is due to the evaporation of the absorbent solvents, which happened below 200 °C (about 3% of weight). It is very important that no weight loss happens up to 200 °C, except for the evaporation of the absorbent solvents, which means that Cu-P.bis(OA)@FeB-MNPs is stable and applicable up to 200 °C. The immobilized organic ligands on FeB-MNPs were decomposed after 200 °C, that indicated as the second step of weight loss in the TGA diagram. Therefore, P.bis(OA) ligand was successfully immobilized on the surface of FeB-MNPs. Finally, a small weight loss above 700 °C may be due to the continuation of biochar pyrolysis.
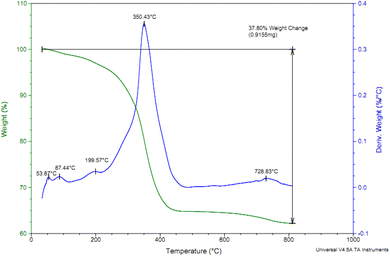 |
| Fig. 4 TGA/DTG diagrams for Cu-P.bis(OA)@FeB-MNPs. | |
3.4. N2 adsorption–desorption isotherms of Cu-P.bis(OA)@FeB-MNPs
The nitrogen adsorption/desorption technique is commonly used for the determination of the pore volume, pore diameter, and surface area of the materials. The resulting isotherms of nitrogen adsorption/desorption for Cu-P.bis(OA)@FeB-MNPs are investigated in Fig. 5. These isotherms display type H3 based on the IUPAC classification, which shows the pores structure of Cu-P.bis(OA)@FeB-MNPs.52 Based on BET results, the specific surface area of Cu-P.bis(OA)@FeB-MNPs is 55.23 m2 g−1. Also, the total pore volume of Cu-P.bis(OA)@FeB-MNPs is 0.09 cm3 g−1, and the average pore diameter of Cu-P.bis(OA)@FeB-MNPs is 6.81 nm.
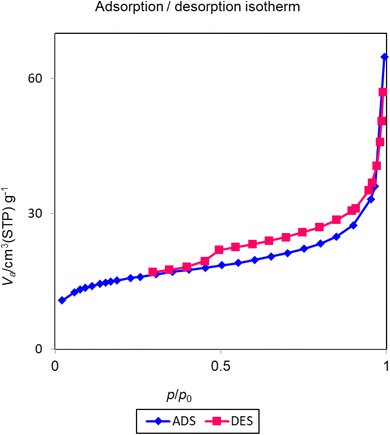 |
| Fig. 5 N2 adsorption–desorption isotherms of Cu-P.bis(OA)@FeB-MNPs. | |
3.5. VSM curve of Cu-P.bis(OA)@FeB-MNPs
The magnetic property of Cu-P.bis(OA)@FeB-MNPs was studied with VSM by the “Magnetic Kavir Kashan” device, which is outlined in Fig. 6. As shown, Cu-P.bis(OA)@FeB-MNPs showed 0.8 emu g−1, which is lower than the reported saturation magnetization for FeB-MNPs.52 The decrease in the saturation magnetic property of Cu-P.bis(OA)@FeB-MNPs is due to the grafting of Cu-complex, P.bis(OA) ligand and silica layer on FeB-MNPs.
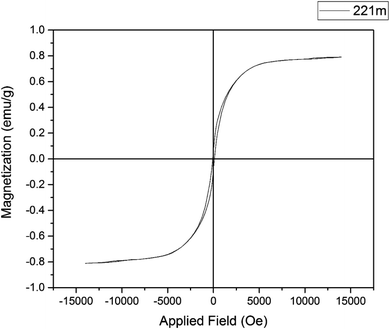 |
| Fig. 6 Magnetization curves for Cu-P.bis(OA)@FeB-MNPs. | |
3.6. Catalytic performance of Cu-P.bis(OA)@FeB-MNPs in the synthesis of tetrazoles
The catalytic application of Cu-P.bis(OA)@FeB-MNPs was examined for the synthesis of tetrazoles. This catalyst showed a high efficiency and good selectivity in the cyclization reaction of [2 + 3] nitriles with sodium azide in the synthesis of tetrazoles (Scheme 5).
 |
| Scheme 5 The general progress for tetrazoles synthesis catalyzed by Cu-P.bis(OA)@FeB-MNPs. | |
The catalytic performance of Cu-P.bis(OA)@FeB-MNPs is described in the homoselective production of nitrogen-rich heterocyclic 5-substituted tetrazoles via [3 + 2]cycloaddition reaction of NaN3 with monofunctionalyzed benzonitriles. At first, the minimum required amount of Cu-P.bis(OA)@FeB-MNPs was checked in the [3 + 2]cycloaddition reaction of NaN3 with unsubstituted benzonitrile (Ph–CN) for the formation of 5-phenyl-1H-tetrazole. No product was formed when zero amount of Cu-P.bis(OA)@FeB-MNPs was used (Table 1, entry 1). But, when the amount of Cu-P.bis(OA)@FeB-MNPs increases, the yield of synthesized 5-phenyl-1H-tetrazole product increases, and the reaction time decreases. Finally, the minimum required amount of Cu-P.bis(OA)@FeB-MNPs was optimized at 30 mg (Table 1, entry 3). Next, the effect of the solvent in the synthesis of 5-phenyl-1H-tetrazole was checked. Volatile solvents e.g. n-hexane, aprotic solvents e.g. DMSO and protic solvents were investigated in the production of 5-phenyl-1H-tetrazole using 30 mg of Cu-P.bis(OA)@FeB-MNPs to obtain the optimal conditions. As clearly shown in Table 1, the production of 5-phenyl-1H-tetrazole increased in polar solvents with high boiling point. Finally, the best results were obtained in the PEG-400 solvent. In addition, in the study of the effect of temperature, the best results were indicated at 120 °C. The model reaction was tested on a large scale of the reactants (NaN3 (14 mmol, 0.9101 g) with benzonitriles (10 mmol, 1.0304 g)). The reaction yield was approximately 69% after 10 h.
Table 1 Optimization of reaction conditions for the production of tetrazoles in the presence of Cu-P.bis(OA)@FeB-MNPs
Entry |
Catalyst (mg) |
Solvent |
Temp. (°C) |
Time (min) |
Yielda (%) |
The products were separated using thin layer chromatography.
|
1 |
— |
PEG |
120 |
720 |
NR |
2 |
20 |
PEG |
120 |
220 |
81 |
3 |
30 |
PEG |
120 |
120 |
97 |
4 |
40 |
PEG |
120 |
110 |
97 |
5 |
30 |
H2O |
Reflux |
360 |
51 |
6 |
30 |
EtOH |
Reflux |
360 |
58 |
7 |
30 |
Dioxane |
Reflux |
360 |
53 |
8 |
30 |
DMSO |
120 |
360 |
73 |
9 |
30 |
n-Hexane |
Reflux |
360 |
20 |
10 |
30 |
PEG |
100 |
180 |
75 |
In continuation, various 5-substituted tetrazole compounds were synthesized under the above-defined conditions in the presence of Cu-P.bis(OA)@FeB-MNPs as a catalyst. The details of the experimental results such as reaction time, efficiency, and TOF values are shown in Table 2. In which, the starting material of benzonitriles having an electron-donating or withdrawing groups on meta, ortho, or para position of the aromatic ring were tested and successfully converted to tetrazole products. As displayed in Table 2, the final 5-substituted tetrazoles were formed in excellent yields within a fast reaction rate in the presence of Cu-P.bis(OA)@FeB-MNPs.
Table 2 Synthesis of 5-substituted tetrazoles catalyzed by Cu-P.bis(OA)@FeB-MNPs
Entry |
Nitrile |
Product |
Time (min) |
Yield (%) |
Melting point (°C) |
1 |
|
|
120 |
97 |
211–214 [ref. 14] |
2 |
|
|
35 |
94 |
209–211 [ref. 53] |
3 |
|
|
210 |
90 |
179–182 [ref. 37] |
4 |
|
|
45 |
95 |
221–224 [ref. 54] |
5 |
|
|
110 |
87 |
216–219 [ref. 55] |
6 |
|
|
300 |
86 |
260–263 [ref. 56] |
7 |
|
|
320 |
89 |
259–261 [ref. 14] |
8 |
|
|
100 |
93 |
249–252 [ref. 55] |
9 |
|
|
80 |
89 |
150–152 [ref. 37] |
Importantly, Cu-P.bis(OA)@FeB-MNPs exhibit homoselectivity in the formation of 5-substituted tetrazoles. Because, in the reaction of NaN3 with dicyano-substituted benzonitriles (e.g. terephthalonitrile and phthalonitrile), only mono-addition happened in the presence of Cu-P.bis(OA)@FeB-MNPs (Scheme 6). These starting materials have two quite similar C
N groups in their structure. The homoselectivity of Cu-P.bis(OA)@FeB-MNPs in the formation of 5-substituted tetrazoles through [3 + 2]cycloaddition of NaN3 with terephthalonitrile and phthalonitrile were investigated using 1H NMR. When phthalonitrile was used as the starting material, two products – 2-(1H-tetrazol-5-yl)benzonitrile (A) or 1,2-di(1H-tetrazol-5-yl)benzene (B) – were formed. As mentioned, one of the C
N groups selectively reacted with NaN3 for the synthesis of 2-(1H-tetrazol-5-yl)benzonitrile, and the other C
N group did not react, and the 1,2-di(1H-tetrazol-5-yl)benzene product was not formed. As expected, when phthalonitrile was used, if product A was synthesized, more than two peaks should be observed in the 1H NMR spectrum for aromatic hydrogens. But if B was synthesized, only two peaks should be observed in the 1H NMR spectrum for aromatic hydrogens. As shown in the 1H NMR spectrum of the final product from the [3 + 2] cycloaddition reaction of NaN3 with phthalonitrile in the presence of Cu-P.bis(OA)@FeB-MNPs, three peaks were indicated for aromatic hydrogens (Fig. 7). Therefore, only product A was certainly formed, which indicates the homoselectively of Cu-P.bis(OA)@FeB-MNPs in the synthesis of tetrazoles.
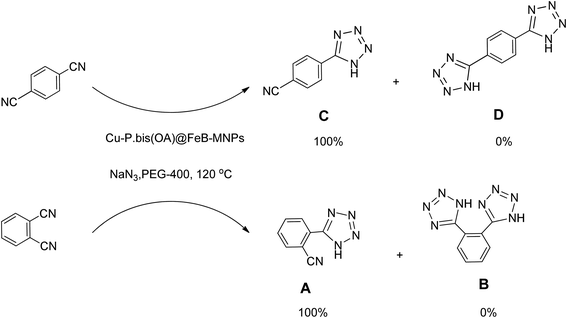 |
| Scheme 6 Homoselectivity of Cu-P.bis(OA)@FeB-MNPs in the [3 + 2] cycloaddition of NaN3 with terephthalonitrile and phthalonitrile. | |
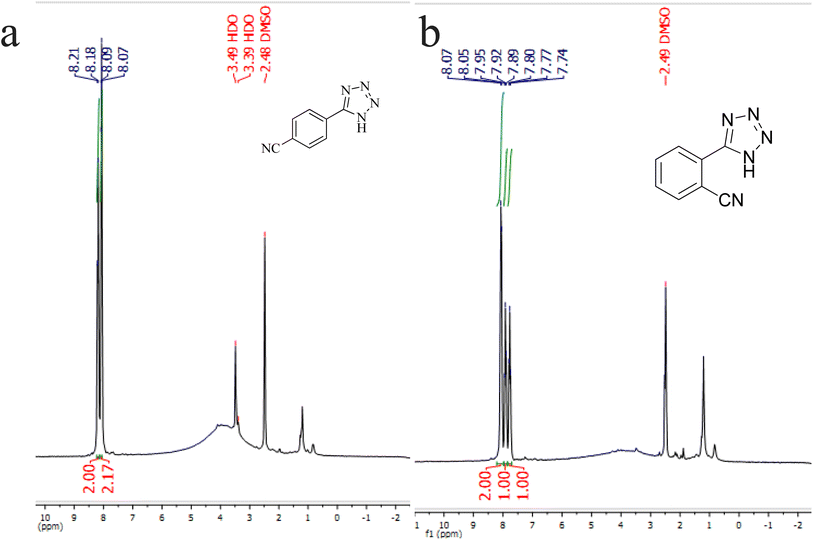 |
| Fig. 7
1H NMR spectrum of 4-(1H-tetrazol-5-yl)benzonitrile (a) and 2-(1H-tetrazol-5-yl)benzonitrile (b). | |
When terephthalonitrile was used as the starting material, two products – 4-(1H-tetrazol-5-yl)benzonitrile (C) or 1,4-di(1H-tetrazol-5-yl)benzene (D) were formed. As mentioned, one of the C
N groups selectively reacted with NaN3 for the synthesis of C, the other C
N group did not react, and the D product was not formed. As expected, when terephthalonitrile was used, if product C was synthesized, two peaks should have been observed in the 1H NMR spectrum for aromatic hydrogens. But if D was synthesized, only one peak should have been observed in the 1H NMR spectrum for aromatic hydrogens. As shown in the 1H NMR spectrum of the final product from the [3 + 2] cycloaddition reaction of NaN3 with terephthalonitrile in the presence of Cu-P.bis(OA)@FeB-MNPs, two peaks indicated the aromatic hydrogens (Fig. 7). Therefore, only product C was certainly formed, which indicates the homoselectively of Cu-P.bis(OA)@FeB-MNPs in the synthesis of tetrazoles.
Based on the literature,53,54,57 an expected mechanism is illustrated in Scheme 7 for the synthesizing of 5-substituted tetrazoles through [3 + 2] cycloaddition reaction of NaN3 and nitriles in the presence of Cu-P.bis(OA)@FeB-MNPs. In this suggested mechanism, the interaction of the C
N functional group with the active site of the catalyst causes the C
N group to become susceptible to attack by azide ions, and the intermediate II is formed as sodium salt forms. The addition of HCl during workup converts the salt form of intermediate II to final tetrazole, which forms tetrazoles extracted in ethyl acetate.
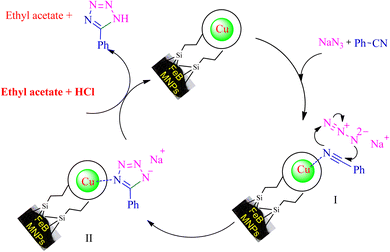 |
| Scheme 7 The expected catalytic mechanism for the synthesis of 5-substituted tetrazoles using Cu-P.bis(OA)@FeB-MNPs. | |
3.7. Reusability of Cu-P.bis(OA)@FeB-MNPs
Practicability, reusability, stability, and availability are the determinative factors for catalysts. According to the emphasis of green chemistry on the reusability of catalysts, the reusability of Cu-P.bis(OA)@FeB-MNPs was investigated in the [3 + 2]cycloaddition reaction of NaN3 with Ph–CN toward the formation of 5-phenyl-1H-tetrazole. In this regard, Cu-P.bis(OA)@FeB-MNPs were isolated after the completion of the reaction and then reused again without double activation. As indicated in Fig. 8, Cu-P.bis(OA)@FeB-MNPs can be recycled up to 6 times.
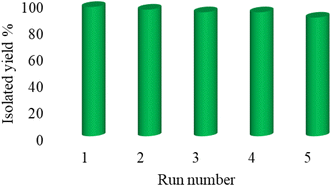 |
| Fig. 8 The reusability of Cu-P.bis(OA)@FeB-MNPs. | |
Also, the recycled Cu-P.bis(OA)@FeB-MNPs nanocatalyst was characterized by SEM (Fig. 9). The SEM images of recycled Cu-P.bis(OA)@FeB-MNPs catalyst showed that the morphology and size of this catalyst did not significantly change after reusing, therefore Cu-P.bis(OA)@FeB-MNPs catalyst was stable after reusing.
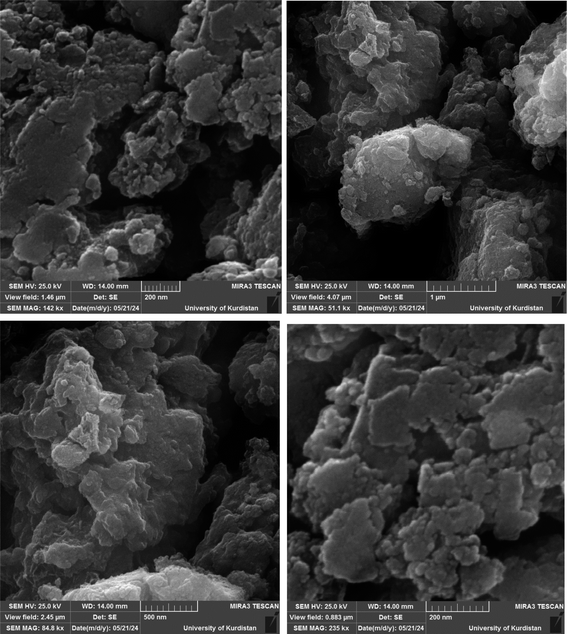 |
| Fig. 9 SEM images of recycled Cu-P.bis(OA)@FeB-MNPs catalyst. | |
3.8. Comparison of Cu-P.bis(OA)@FeB-MNPs with other catalysts
The practicability of Cu-P.bis(OA)@FeB-MNPs was compared with other reported catalysts (Table 3). The [3 + 2] cycloaddition reaction of NaN3 and Ph–CN in the presence of Cu-P.bis(OA)@FeB-MNPs was compared with other catalysts. As indicated, Cu-P.bis(OA)@FeB-MNPs exhibit 98% of the product within only 2 h, which indicates a better yield and reaction time than the other catalysts. Besides, several reports in literature formed from unrenewable materials or used hazard solvents or limited by time-consuming or difficult catalyst recovery. While FeB-MNPs are formed from renewable materials as an ideal waste recycling and Cu-P.bis(OA)@FeB-MNPs can easily be recovered and reused. More addition, 5-substituted tetrazoles were synthesized in the green solvent (PEG-400) in the presence of Cu-P.bis(OA)@FeB-MNPs.
Table 3 Comparison results of Cu-P.bis(OA)@FeB-MNPs with other catalysts in the formation of 5-phenyl-1H-tetrazole
Entry |
Catalyst |
Time (h) |
Yield (%) |
Ref. |
1 |
CoY zeolite |
14 |
90 |
58
|
2 |
Cu–Zn alloy nanopowder |
10 |
95 |
59
|
3 |
B(C6F5)3 |
8 |
94 |
60
|
4 |
Fe3O4@SiO2/salen Cu(II) |
7 |
90 |
61
|
5 |
Fe3O4/ZnS HNSs |
24 |
81.1 |
62
|
6 |
Mesoporous ZnS |
36 |
86 |
63
|
7 |
AgNO3 |
5 |
83 |
64
|
8 |
CuFe2O4 |
12 |
82 |
65
|
9 |
Nano ZnO/Co3O4 |
12 |
90 |
66
|
10 |
Ni-MP(AMP)2@Fe-biochar |
3.8 |
92 |
52
|
12 |
Fe3O4@boehmite NPs |
4 |
97 |
67
|
13 |
Cu(II)[Sal(PMeOSi)DETA]@KIT-6 |
3 |
94 |
68
|
14 |
KIT-6@DABP@Cu |
5 |
91 |
69
|
15 |
KIT-6@SMTU@Ni |
3 |
95 |
70
|
16 |
Cu-TDBB@MCM-41/Fe3O4 |
7 |
90 |
71
|
17 |
Nd-Schiff-base@BMNPs |
3 |
98 |
48
|
18 |
Nd-bis(PYT)@boehmite NPs |
2.5 |
97 |
53
|
19 |
Pd–SMTU@boehmite |
2.5 |
95 |
72
|
20 |
Cu-P.bis(OA)@FeB-MNPs |
2 |
97 |
This work |
4. Conclusions
In this work, magnetic biochar NPs (FeB-MNPs) were synthesized via pyrolysis of chicken manure, which is a new process for recycling waste. Then, the performed biochar was magnetized by magnetic Fe(0) nanoparticles to improve its recovery. Then, a new copper complex was immobilized on its surface and used as a selective, inexpensive, stable, recoverable, practicable, and available catalyst for the synthesis of 5-substituted tetrazoles. This catalyst (Cu-P.bis(OA)@FeB-MNPs) was characterized by WDX, SEM, TGA, EDS, VSM, AAS, and N2 adsorption–desorption (BET method) techniques. SEM images indicated that the particles of this catalyst are less than 70 nm in size. TGA analysis confirmed that the P.bis(OA) ligand was successfully supported on FeB-MNPs and Cu-P.bis(OA)@FeB-MNPs is stable up to 200 °C. EDS and WDX confirmed that the surface of FeB-MNPs was successfully modified by Cl-PTMS and then functionalized by the P.bis(OA) ligand and the copper complex was formed on the surface of functionalized FeB-MNPs. VSM analysis indicated that this catalyst can be recovered by an external magnet. Therefore, it was recovered and reused for several runs. The BET method showed good surface area for Cu-P.bis(OA)@FeB-MNPs, therefore, this catalyst has high efficiency and activity in the synthesis of 5-substituted tetrazoles.
Author contributions
Marwan Majeed Maseer: methodology. Tavan Kikhavani: supervision, project administration, formal analysis. Bahman Tahmasbi: supervision, conceptualization, formal analysis, resources, project administration, writing – original draft, writing review and editing.
Conflicts of interest
The authors declare no conflict of interest and competing interests.
Acknowledgements
The authors thank the research facilities of Ilam University, Ilam, Iran, for financial support of this research project.
References
- S. L. Tang, R. L. Smith and M. Poliakoff, Principles of green chemistry: productively, Green Chem., 2005, 7, 761–762 RSC.
-
P. T. Anastas and J. C. Warner, Principles of green chemistry, Green Chemistry: Theory and Practice, 1998, vol. 29, pp. 14821–14842 Search PubMed.
-
V. Ahluwalia, M. Kidwai, V. Ahluwalia and M. Kidwai, Basic principles of green chemistry, New trends in green chemistry, 2004, pp. 5–14 Search PubMed.
- P. Anastas and N. Eghbali, Green chemistry: principles and practice, Chem. Soc. Rev., 2010, 39, 301–312 RSC.
- W. Abdussalam-Mohammed, A. Q. Ali and A. Errayes, Green chemistry: principles, applications, and disadvantages, Chem. Methodol., 2020, 4, 408–423 CrossRef CAS.
- D. Astruc, F. Lu and J. R. Aranzaes, Nanoparticles as recyclable catalysts: the frontier between homogeneous and heterogeneous catalysis, Angew. Chem., Int. Ed. Engl., 2005, 44, 7852–7872 CrossRef CAS PubMed.
- A. Corma and H. Garcia, Crossing the borders between homogeneous and heterogeneous catalysis: developing recoverable and reusable catalytic systems, Top. Catal., 2008, 48, 8–31 CrossRef CAS.
- F. Poovan, V. G. Chandrashekhar, K. Natte and R. V. Jagadeesh, Synergy between homogeneous and heterogeneous catalysis, Catal. Sci. Technol., 2022, 12, 6623–6649 RSC.
- S. Shylesh, V. Schünemann and W. R. Thiel, Magnetically separable nanocatalysts: bridges between homogeneous and heterogeneous catalysis, Angew. Chem., Int. Ed. Engl., 2010, 49, 3428–3459 CrossRef CAS PubMed.
- V. Polshettiwar and R. S. Varma, Green chemistry by nano-catalysis, Green Chem., 2010, 12, 743–754 RSC.
- S. B. Somwanshi, S. B. Somvanshi and P. B. Kharat, Nanocatalyst: A Brief Review on Synthesis to Applications, J. Phys.: Conf. Ser., 2020, 012046 CrossRef.
-
P. Saini, S. Meena, D. K. Mahawar, A. Dandia and V. Parewa, Principles and Concepts of Nanocatalysis, Advanced Nanocatalysis for Organic Synthesis and Electroanalysis, 2022, p. 1 Search PubMed.
- B. Tahmasbi, M. Darabi and M. Nikoorazm, A new Schiff-base complex of palladium nanoparticles on modified boehmite with di(pyridin-2-yl)methanone as a robust, reusable, and selective nanocatalyst in the C–C coupling reaction, Appl. Organomet. Chem., 2024, 38, e7348 CrossRef CAS.
- P. Moradi, T. Kikhavani and Y. Abbasi Tyula, A new samarium complex of 1,3-bis(pyridin-3-ylmethyl)thiourea on boehmite nanoparticles as a practical and recyclable nanocatalyst for the selective synthesis of tetrazoles, Sci. Rep., 2023, 13, 5902 CrossRef CAS PubMed.
- A. Jabbari, P. Moradi, M. Hajjami and B. Tahmasbi, Tetradentate copper complex supported on boehmite nanoparticles as an efficient and heterogeneous reusable nanocatalyst for the synthesis of diaryl ethers, Sci. Rep., 2022, 12, 11660 CrossRef CAS PubMed.
- A. Jabbari, M. Nikoorazm and P. Moradi, Two Schiff-base complexes of cadmium and manganese on modified MCM-41 as practical, recyclable and selective nanocatalysts for the synthesis of sulfoxides, J. Porous Mater., 2023, 30, 1395–1402 CrossRef CAS.
- A. Jabbari, M. Nikoorazm and P. Moradi, AV (O)-Schiff-base complex on MCM-41 as an efficient, reusable, and chemoselective nanocatalyst for the oxidative coupling of thiols and oxidation of sulfides, Res. Chem. Intermed., 2023, 49, 1485–1505 CrossRef CAS.
- R. S. Salama, S. M. El-Bahy and M. A. Mannaa, Sulfamic acid supported on mesoporous MCM-41 as a novel, efficient and reusable heterogenous solid acid catalyst for synthesis of xanthene, dihydropyrimidinone and coumarin derivatives, Colloids Surf., A, 2021, 628, 127261 CrossRef CAS.
- P. Moradi and M. Hajjami, Magnetization of graphene oxide nanosheets using nickel magnetic nanoparticles as a novel support for the fabrication of copper as a practical, selective, and reusable nanocatalyst in C–C and C–O coupling reactions, RSC Adv., 2021, 11, 25867–25879 RSC.
- H. M. Altass, M. Morad, A. E.-R. S. Khder, M. A. Mannaa, R. S. Jassas and A. A. Alsimaree,
et al., Enhanced catalytic activity for CO oxidation by highly active Pd nanoparticles supported on reduced graphene oxide/copper metal organic framework, J. Taiwan Inst. Chem. Eng., 2021, 128, 194–208 CrossRef CAS.
- H. M. Altass, S. A. Ahmed, R. S. Salama, Z. Moussa, R. S. Jassas and R. I. Alsantali,
et al., Low temperature CO oxidation over highly active gold nanoparticles supported on reduced graphene oxide@Mg-BTC nanocomposite, Catal. Lett., 2023, 153, 876–886 CrossRef CAS.
- A. Rezaei, A. Ghorbani-Choghamarani and B. Tahmasbi, Synthesis and characterization of nickel metal-organic framework including 4,6-diamino-2-mercaptopyrimidine and its catalytic application in organic reactions, Catal. Lett., 2023, 153, 2005–2017 CrossRef CAS.
- S. A. El-Hakam, S. E. Samra, S. M. El-Dafrawy, A. A. Ibrahim, R. S. Salama and A. I. Ahmed, Synthesis of sulfamic acid supported on Cr-MIL-101 as a heterogeneous acid catalyst and efficient adsorbent for methyl orange dye, RSC Adv., 2018, 8, 20517–20533 RSC.
- R. S. Salama, S. El-Hakam, S. Samra, S. El-Dafrawy and A. Ahmed, Cu-BDC as a Novel and Efficient Catalyst for the Synthesis of 3,4-Dihydropyrimidin-2(1H)-ones and Aryl-14H-dibenzo[a,j]Xanthenes under Conventional Heating, Int. J. Nano Mater. Sci., 2018, 7, 31–42 CAS.
- R. S. Salama, S. El-Hakam, S. Samra, S. El-Dafrawy and A. Ahmed, Adsorption, equilibrium and kinetic studies on the removal of methyl orange dye from aqueous solution by using of copper metal organic framework (Cu-BDC), Int. J. Mod. Chem., 2018, 10, 195–207 CAS.
- S. M. El-Dafrawy, R. S. Salama, S. A. El-Hakam and S. E. Samra, Bimetal-organic frameworks (Cux–Cr100−x–MOF) as a stable and efficient catalyst for synthesis of 3,4-dihydropyrimidin-2-one and 14-phenyl-14H-dibenzo[a,j]xanthene, J. Mater. Res. Technol., 2020, 9, 1998–2008 CrossRef CAS.
- R. S. Salama, A. El-Hakam, S. Samra, S. El-Dafrawy, A. A. Ibrahim and A. I. Ahmed, Removal of methyl orange (MO) from aqueous solution by bimetal-organic frameworks (Cux–Cr100−x–MOF): kinetics and isotherms studies, Delta University Scientific Journal, 2023, 6, 266–277 CrossRef.
- R. S. Salama, S. M. Hassan, A. I. Ahmed, W. A. El-Yazeed and M. A. Mannaa, The role of PMA in enhancing the surface acidity and catalytic activity of a bimetallic Cr–Mg-MOF and its applications for synthesis of coumarin and dihydropyrimidinone derivatives, RSC Adv., 2020, 10, 21115–21128 RSC.
- R. S. Salama, M. A. Mannaa, H. M. Altass, A. A. Ibrahim and A. E.-R. S. Khder, Palladium supported on mixed-metal–organic framework (Co–Mn-MOF-74) for efficient catalytic oxidation of CO, RSC Adv., 2021, 11, 4318–4326 RSC.
- R. Salama, Synthesis, characterization and catalytic activities of sulfuric acid loaded on copper metal organic frameworks (Cu-BDC), Delta University Scientific Journal, 2019, 2, 10–15 CrossRef.
- F. T. Alshorifi, D. E. Tobbala, S. M. El-Bahy, M. A. Nassan and R. S. Salama, The role of phosphotungstic acid in enhancing the catalytic performance of UiO-66 (Zr) and its applications as an efficient solid acid catalyst for coumarins and dihydropyrimidinones synthesis, Catal. Commun., 2022, 169, 106479 CrossRef CAS.
- Y. Zhai, Z. Zhu and S. Dong, Carbon-based nanostructures for advanced catalysis, ChemCatChem, 2015, 7, 2806–2815 CrossRef CAS.
- P. Wang, Q. Dong, C. Gao, W. Bai, D. Chu and Y. He, A comprehensive review of carbon nanotubes: growth mechanisms, preparation and applications, Fullerenes, Nanotubes Carbon Nanostruct., 2024, 32, 415–429 CrossRef CAS.
- H. Matsumoto, T. Iwai, M. Sawamura and Y. Miura, Continuous-Flow Catalysis Using Phosphine-Metal Complexes on Porous Polymers: Designing Ligands, Pores, and Reactors, ChemPlusChem, 2024, e202400039 CrossRef PubMed.
- S. Jaiswal, J. Dwivedi, D. Kishore and S. Sharma, Green Methodologies for Tetrazole Synthesis from Different Starting Materials: A Recent Update, Curr. Org. Chem., 2024, 28, 134–160 CrossRef CAS.
- M. Norouzi and P. Moradi, A new copper complex on functionalized magnetic biochar nano-sized materials as sustainable heterogeneous catalysts for C–O bond formation in the natural deep eutectic solvent, Biomass Convers. Biorefin., 2023, 1–13, DOI:10.1007/s13399-023-05163-z.
- P. Moradi, B. Zarei, Y. Abbasi Tyula and M. Nikoorazm, Novel neodymium complex on MCM-41 magnetic nanocomposite as a practical, selective, and returnable nanocatalyst in the synthesis of tetrazoles with antifungal properties in agricultural, Appl. Organomet. Chem., 2023, 37, e7020 CrossRef CAS.
- M. Alekasir, S. Heydarian and B. Tahmasbi, The synthesis of biochar from biomass waste recycling and its surface modification for immobilization of a new Cu complex as a reusable nanocatalyst in the homoselective synthesis of tetrazoles, Res. Chem. Intermed., 2024, 50, 2031–2049 CrossRef CAS.
- S.-Z. Zhang, Z.-S. Cui, M. Zhang and Z.-H. Zhang, Biochar-based functional materials as heterogeneous catalysts for organic reactions, Curr. Opin. Green Sustainable Chem., 2022, 38, 100713 CrossRef CAS.
- L.-N. Dong, S.-Z. Zhang, W.-L. Zhang, Y. Dong, L.-P. Mo and Z.-H. Zhang, Synthesis, characterization and application of magnetic biochar sulfonic acid as a highly efficient recyclable catalyst for preparation of spiro-pyrazolo[3,4-b]pyridines, Res. Chem. Intermed., 2022, 48, 1249–1272 CrossRef CAS.
- A. Ivanković, A. Dronjić, A. M. Bevanda and S. Talić, Review of 12 principles of green chemistry in practice, Int. J. Sustainable Green Energy, 2017, 6, 39–48 CrossRef.
- R. A. Sheldon, Fundamentals of green chemistry: efficiency in reaction design, Chem. Soc. Rev., 2012, 41, 1437–1451 RSC.
- K. Weber and P. Quicker, Properties of biochar, Fuel, 2018, 217, 240–261 CrossRef CAS.
- J. Lee, K.-H. Kim and E. E. Kwon, Biochar as a catalyst, Renewable Sustainable Energy Rev., 2017, 77, 70–79 CrossRef CAS.
- X. Cao, S. Sun and R. Sun, Application of biochar-based catalysts in biomass upgrading: a review, RSC Adv., 2017, 7, 48793–48805 RSC.
- R. Shan, J. Han, J. Gu, H. Yuan, B. Luo and Y. Chen, A review of recent developments in catalytic applications of biochar-based materials, Resour., Conserv. Recycl., 2020, 162, 105036 CrossRef.
- H. Lyu, Q. Zhang and B. Shen, Application of biochar and its composites in catalysis, Chemosphere, 2020, 240, 124842 CrossRef CAS PubMed.
- B. Tahmasbi, P. Moradi and M. Darabi, A new neodymium complex on renewable magnetic biochar nanoparticles as an environmentally friendly, recyclable and efficient nanocatalyst in the homoselective synthesis of tetrazoles, Nanoscale Adv., 2024, 6, 1932–1944 RSC.
- M.-N. Chen, L.-P. Mo, Z.-S. Cui and Z.-H. Zhang, Magnetic nanocatalysts: synthesis and application in multicomponent reactions, Curr. Opin. Green Sustainable Chem., 2019, 15, 27–37 CrossRef.
- S. Z. Zhang, L. N. Dong, L. P. Mo and Z. H. Zhang, Magnetic biochar-supported ZrO2 as an efficient catalyst for one-pot synthesis of 1′, 4′-dihydro-3H,3′H-spiro[furo[3,4-b]quinoline-9,2′-quinoxaline]-1,3′(4H)-diones, Appl. Organomet. Chem., 2023, 37, e6949 CrossRef CAS.
- L. N. Dong, Y. M. Wang, W. L. Zhang, L. P. Mo and Z. H. Zhang, Nickel supported on magnetic biochar as a highly efficient and recyclable heterogeneous catalyst for the one-pot synthesis of spirooxindole-dihydropyridines, Appl. Organomet. Chem., 2022, 36, e6667 CrossRef CAS.
- P. Moradi and M. Hajjami, Magnetization of biochar nanoparticles as a novel support for fabrication of organo nickel as a selective, reusable and magnetic nanocatalyst in organic reactions, New J. Chem., 2021, 45, 2981–2994 RSC.
- Y. Abbasi Tyula, P. Moradi and M. Nikoorazm, A New Neodymium Complex on Boehmite Nanoparticles with 1,3-Bis(pyridine-3-ylmethyl)thiourea as a Practical and Reusable Nanocatalyst for the Chemoselective Synthesis of Tetrazoles, ChemistrySelect, 2023, 8, e202301674 CrossRef.
- A. Jabbari, P. Moradi and B. Tahmasbi, Synthesis of tetrazoles catalyzed by a new and recoverable nanocatalyst of cobalt on modified boehmite NPs with 1,3-bis(pyridin-3-ylmethyl)thiourea, RSC Adv., 2023, 13, 8890–8900 RSC.
- M. Akbari, M. Nikoorazm, B. Tahmasbi and A. Ghorbani-Choghamarani, Homoselective synthesis of tetrazoles and chemoselective oxidation of sulfides using Ni(II)-Schiff base complex stabilized on 3-dimensional mesoporous KIT-6 surface as a recyclable nanocatalyst, Inorg. Chem. Commun., 2024, 160, 111852 CrossRef CAS.
- B. Tahmasbi, M. Nikoorazm, P. Moradi and Y. A. Tyula, A Schiff base complex of lanthanum on modified MCM-41 as a reusable nanocatalyst in the homoselective synthesis of 5-substituted 1H-tetrazoles, RSC Adv., 2022, 12, 34303–34317 RSC.
- B. Tahmasbi, M. Nikoorazm, P. Moradi and Y. Abbasi Tyula, A Schiff base complex of lanthanum on modified MCM-41 as a reusable nanocatalyst in the homoselective synthesis of 5-substituted 1H-tetrazoles, RSC Adv., 2022, 12, 34303–34317 RSC.
- V. Rama, K. Kanagaraj and K. Pitchumani, Syntheses of 5-substituted 1H-tetrazoles catalyzed by reusable CoY zeolite, J. Org. Chem., 2011, 76, 9090–9095 CrossRef CAS PubMed.
- G. Aridoss and K. K. Laali, Highly efficient synthesis of 5-substituted 1H-tetrazoles catalyzed by Cu–Zn alloy nanopowder, conversion into 1,5-and 2,5-disubstituted tetrazoles, and synthesis and NMR studies of new tetrazolium ionic liquids, Eur. J. Org Chem., 2011, 2011, 6343–6355 CrossRef CAS.
- S. K. Prajapti, A. Nagarsenkar and B. N. Babu, An efficient synthesis of 5-substituted 1H-tetrazoles via B (C6F5)3 catalyzed [3 + 2] cycloaddition of nitriles and sodium azide, Tetrahedron Lett., 2014, 55, 3507–3510 CrossRef CAS.
- F. Dehghani, A. R. Sardarian and M. Esmaeilpour, Salen complex of Cu (II) supported on superparamagnetic Fe3O4@SiO2 nanoparticles: an efficient and recyclable catalyst for synthesis of 1-and 5-substituted 1H-tetrazoles, J. Organomet. Chem., 2013, 743, 87–96 CrossRef CAS.
- G. Qi, W. Liu and Z. Bei, Fe3O4/ZnS Hollow Nanospheres: A Highly Efficient Magnetic Heterogeneous Catalyst for Synthesis of 5-Substituted 1H-Tetrazoles from Nitriles and Sodium Azide, Chin. J. Chem., 2011, 29, 131–134 CrossRef CAS.
- L. Lang, H. Zhou, M. Xue, X. Wang and Z. Xu, Mesoporous ZnS hollow spheres-catalyzed synthesis of 5-substituted 1H-tetrazoles, Mater. Lett., 2013, 106, 443–446 CrossRef CAS.
- P. Mani, A. K. Singh and S. K. Awasthi, AgNO3 catalyzed synthesis of 5-substituted-1H-tetrazole via [3 + 2] cycloaddition of nitriles and sodium azide, Tetrahedron Lett., 2014, 55, 1879–1882 CrossRef CAS.
- B. Sreedhar, A. S. Kumar and D. Yada, CuFe2O4 nanoparticles: a magnetically recoverable and reusable catalyst for the synthesis of 5-substituted 1H-tetrazoles, Tetrahedron Lett., 2011, 52, 3565–3569 CrossRef CAS.
- S. M. Agawane and J. M. Nagarkar, Synthesis of 5-substituted 1H-tetrazoles using a nano ZnO/Co3O4 catalyst, Catal. Sci. Technol., 2012, 2, 1324–1327 RSC.
- P. Moradi, Investigation of Fe3O4@boehmite NPs as efficient and magnetically recoverable nanocatalyst in the homoselective synthesis of tetrazoles, RSC Adv., 2022, 12, 33459–33468 RSC.
- M. Akbari, M. Nikoorazm, B. Tahmasbi and A. Ghorbani-Choghamarani, The new Schiff-base complex of copper (II) grafted on mesoporous KIT-6 as an effective nanostructure catalyst for the homoselective synthesis of various tetrazoles, Appl. Organomet. Chem., 2024, 38, e7317 CrossRef CAS.
- M. Darabi, M. Nikoorazm, B. Tahmasbi and A. Ghorbani-Choghamarani, Homoselective synthesis of tetrazole derivatives using copper complex anchored on mesoporous KIT-6 as a reusable, highly efficient, and environmentally green nanocatalyst, Appl. Organomet. Chem., 2024, 38, e7392 CrossRef CAS.
- M. Darabi, M. Nikoorazm, B. Tahmasbi and A. Ghorbani-Choghamarani, Immobilization of Ni (ii) complex on the surface of mesoporous modified-KIT-6 as a new, reusable and highly efficient nanocatalyst for the synthesis of tetrazole and pyranopyrazole derivatives, RSC Adv., 2023, 13, 12572–12588 RSC.
- M. Nikoorazm, B. Tahmasbi, S. Gholami, M. Khanmoradi, Y. A. Tyula and M. Darabi,
et al., Synthesis and characterization of a new Schiff-base complex of copper on magnetic MCM-41 nanoparticles as efficient and reusable nanocatalyst in the synthesis of tetrazoles, Polyhedron, 2023, 244, 116587 CrossRef CAS.
- P. Moradi and A. Ghorbani-Choghamarani, Efficient synthesis of 5-substituted tetrazoles catalysed by palladium–S-methylisothiourea complex supported on boehmite nanoparticles, Appl. Organomet. Chem., 2017, 31, e3602 CrossRef.
|
This journal is © The Royal Society of Chemistry 2024 |