DOI:
10.1039/D4FO00917G
(Paper)
Food Funct., 2024,
15, 6502-6511
Anti-inflammatory activity of plant sterols in a co-culture model of intestinal inflammation: focus on food-matrix effect†
Received
26th February 2024
, Accepted 22nd May 2024
First published on 23rd May 2024
Abstract
This study investigates the gut anti-inflammatory activity of a plant sterol (PS) food supplement (PS-FS), alongside PS-enriched milk-based fruit beverage and PS-enriched rye bread. A co-culture model based on a dual-chamber system with differentiated intestinal-like Caco-2 cells (apical) and RAW264.7 macrophages (basolateral) was used. The bioaccessible fractions (BF) of the samples were obtained after INFOGEST 2.0 simulated gastrointestinal digestion. The BF were added to the apical part (diluted 1/20 v/v with culture medium to avoid cytotoxicity) for 90 min, followed by stimulation with lipopolysaccharide (LPS) (1 μg mL−1, 24 h) on the basolateral side. The pharmacological interaction between samples and budesonide (1 μM, 90 min) was evaluated. Results indicate that PS-FS significantly attenuated LPS-induced secretion of IL-8 (28%) by Caco-2 cells, and TNF-α (9%) and IL-6 (54%) by RAW264.7 macrophages, whereas PS-enriched beverage and bread did not exhibit protective effects. Additionally, PS-FS demonstrated an improvement in oxidative status in Caco-2 cells, evidenced by reduced levels of reactive oxygen species (47%), iNOS protein expression (27%), and nitrite/nitrate secretion (27%). Mechanistically, PS-FS inhibited the NF-κB-COX-2-PGE2 signaling pathway in macrophages, resulting in decreased NF-κB p65 nuclear translocation (39%), COX-2 protein expression (32%), and PGE2 production (27%). Co-treatment with budesonide and PS-FS displayed an antagonistic effect (combination index 0.38–0.63). This study demonstrates the potent intestinal anti-inflammatory activity of a PS-FS, positioning it as a promising nutraceutical product for the management of inflammatory bowel diseases. However, the food matrix of the milk-based fruit beverage and rye bread appear to interfere with the anti-inflammatory activity of PS.
1. Introduction
Plant sterols (PS) constitute a group of bioactive compounds naturally found in plant-based foods, demonstrating notable health benefits.1 Among the recognized effects of PS consumption is their capacity to reduce blood cholesterol levels,2 although they may exert other biological effects at the gastrointestinal level due to their low rates of absorption (0.2–2%).3 In murine models, it has been indicated a protective role of PS against inflammatory bowel diseases using a mix of PS,4 individual PS, specifically, β-sitosterol and stigmasterol,5–7 as well as a PS-enriched milk-based fruit beverage.8 Studies in cell models involving macrophages or intestinal cells have reported the ability of a mix of PS, as well as β-sitosterol and guggulsterone individually, to modulate the immune response and reduce pro-inflammatory parameters.9–12 Only in one study in differentiated Caco-2 cells it was demonstrated the anti-inflammatory activity of a PS-enriched milk-based fruit beverage, although the effect observed cannot be attributed only to PS, but also to other compounds present in the beverage such as vitamin C, polyphenol glucosides and carotenoids.13 Although cellular models have been used to evaluate the anti-inflammatory activity of PS, they present limitations, such as the absence of cellular diversity and intracellular interactions typical of complex organisms.14 To overcome these limitations, a model involving a co-culture system was developed, in which Caco-2 intestinal epithelial cells are placed on the apical side of a Transwell® plate, while RAW264.7 macrophages occupy the basolateral side.15 This configuration allows to replicate the natural arrangement of cell types that are part of the intestine, with epithelial cells in the outer layer and underlying immune cells. Likewise, through a porous membrane, this model facilitates intercellular communication between these different types of cells, replicating the intercellular interaction that could be found in living organisms. This co-culture model has been employed to evaluate the anti-inflammatory activity of bioactive compounds,15 extracts from plants,16 and fruit juice enriched with pine bark extract.17 To our knowledge, this co-culture model has not been tested with PS or PS-enriched food matrices.
Given that natural foods may not supply a considerable amount of PS to claim associated health effects, the necessity of food fortification or supplementation is emphasized and approved by the European Union in milk-based fruit beverages and rye bread.18–20 PS-enriched foods represent an integrated approach to incorporating these compounds into the daily diet, while food supplements, available in various forms such as capsules or powders, offer a convenient option for obtaining a concentrated dose of PS.21 Exploring the potential effects of bioactive compounds within a food matrix following gastrointestinal in vitro digestion could provide insights after their uptake in a closer real context under physiological conditions. For this, this study aimed to assess the anti-inflammatory activity of PS food supplement (PS-FS) and PS-enriched foods (milk-based fruit beverage and rye bread) using a co-culture model of intestinal inflammation with Caco-2 intestinal cells and RAW264.7 macrophages. The potential pharmacological interaction between budesonide (a drug widely used in the management of intestinal inflammation) and the samples was also evaluated.
2. Materials and methods
2.1 Reagents
The PS standards used were 5β-cholestan-3α-ol (epicoprostanol) as internal standard (IS) at 99.8% purity, sourced from Merck LifeScience S.L.U. (Madrid, Spain). Additionally, 5,22-cholestadien-24-ethyl-3β-ol (stigmasterol) at 97.4% purity and 24α-ethyl-5α-cholestan-3β-ol (sitostanol) at 67.5% purity were purchased from Merck LifeScience S.L.U. (Madrid, Spain). The 5-cholesten-24β-ethyl-3β-ol (β-sitosterol) at 98.8% purity and 24α-methyl-5-cholesten-3β-ol (campesterol) at 98.6% purity were purchased from Chengdu Biopurify Phytochemicals Ltd (Sichuan, China). Anhydrous pyridine (from Acros Organics, Geel, Belgium) and N,O-bis(trimethylsilyl)-trifluoroacetamide (BSTFA) [1% trimethylchlorosilane (TMCS)] (from Merck LifeScience S.L.U., Madrid, Spain) were used. Potassium hydroxide and hydrogen chloride (purity 37%) were acquired from Merck LifeScience S.L.U. (Madrid, Spain). Ethanol was provided by VWR (Briare, France), while hexane, diethyl ether, and cyclohexane were purchased from Scharlau (Barcelona, Spain). Water purification was conducted using a Milli-Q system (Milford, MA, United States). N-(1-Naphthyl)ethylenediamine (NED), dimethyl sulfoxide (DMSO), 3-(4,5-dimethylthiazol-2-yl)-2,5-diphenyl-tetrazolium bromide (MTT), 2′,7′-dichlorofluorescein diacetate (DCFDA), LPS from Escherichia coli O127, phosphatase inhibitor cocktail (100 mM sodium orthovanadate, 115 mM sodium molybdate, 400 mM sodium tartrate, and 200 mM imidazole), and protease inhibitor cocktail (50 mM benzenesulfonic fluoride, 15 mM aprotinin, 100 mM E-65, 50 mM EDTA, and 100 mM leupeptin) were purchased from Merck Lifescience S.L. (Madrid, Spain). VCl3 (>99%, w/w) was from Cymit Química (Barcelona, Spain).
2.2 Samples
PS-FS (Lipophytol® P Dispersible, Lipofoods), a food ingredient used for food fortification, was used. The PS content in PS-FS was determined following the methodology described previously (see Table 1S in ESI†).22 Food matrices such as a milk-based fruit beverage and wholemeal rye bread were used, both enriched with PS (0.9 and 2.3% w/w, respectively) to provide the dose of PS for which its hypocholesterolemic effect has been demonstrated (1.5–3 g day−1) in a consumption serving (200 and 80 g, respectively).23 PS content in beverage24 and bread25 are described in previous studies (see Table 1S in ESI†).
2.3 Simulation of gastrointestinal digestion
A simulation of the gastrointestinal digestion of the samples has been carried out, with the purpose of obtaining bioaccessible fraction (BF) that would subsequently be used to evaluate its effects on cell culture. Briefly, an aqueous suspension was prepared by combining 2.3 g of PS-FS (equivalent to the daily dose of 2 g PS, according to its purity) and 200 mL of water. Then, 5 mL of PS-FS suspension or beverage, or 5 g of bread, were digested following the harmonized INFOGEST 2.0 method adapted for PS, either liquid24 and solid matrices.25 The digesta was centrifuged (3100g, 4 °C for 90 min) to obtain the BF, which was subsequently sterilized by filtration with a 0.22 μm filter. To assess the potential harmful effects of digestion reagents on cell culture and following the recommendations for biocompatibility when using food digesta and downstream cell assays, a blank of digestion was performed by digesting 5 g of water as control.26 The PS concentration in the BF of digestion blank, PS-FS, PS-enriched beverage24 and PS-enriched rye bread25 was quantified using a previously established method.
2.4 PS determination in BF
Sterol determination in the BF of beverage and PS-FS was performed as described in Makran et al.24 with modifications. Addition of epicoprostanol as IS (200 μg) to 5 g of BF was carried out before hot saponification (2 mL of 2 M potassium hydroxide in ethanol: water (9
:
1, v
:
v) in a water bath (1 h, 65 °C)). The extraction of the unsaponifiable fraction was performed with 3 × 10 mL of diethyl ether.
For the BF of WRB, the methodology used was as described in Faubel et al.25 Addition of epicoprostanol as IS (200 μg) to 2 mL of the BF and 1 mL of absolute ethanol was carried out. To perform hot saponification, 0.5 mL of saturated aqueous KOH was added, and samples were heated (30 min, 80 °C) in a shaker water bath at 100 rpm. The unsaponifiable fraction was extracted by adding 12 mL of water and 20 mL of cyclohexane and shacking it for 10 min, followed by evaporation of the organic phase to dryness with a rotary evaporator (50 °C), then dissolved in 1 mL of hexane. Solid-phase extraction using silica cartridges (Finisterre SPE tube Si, 500 mgper 6 mL, Teknokroma, Barcelona, Spain) was employed for purification of the organic extract, and sterols were eluted using 5 mL of hexane
:
diethyl ether (1
:
1, v
:
v).
For all samples, the solvent was removed under a stream of nitrogen and the derivatization process was carried out by using 200 μL of pyridine: BSTFA + 1% TMCS (3
:
10, v
:
v) at 65 °C (SBH200D Blockheater, Stuart, Staffordshire, United Kingdom) for 1 h. Subsequently, samples were dissolved in 100 μL (for BF of beverage or PS-FS) or 500 μL (for BF of WRB) of hexane and subjected to analysis (0.7 μL) using FAST gas chromatography-flame ionization detector (Shimadzu GC-2025, Kyoto, Japan) equipped with a capillary column Restek Rxi-5Sil MS (10 m × 0.10 mm × 0.10 μm film thickness, Bellafonte, Pennsylvania, USA). The oven temperature was initially set at 220 °C, rising up to 300 °C at a rate of 15.5 °C min−1, then further increased to 325 °C at a rate of 46.6 °C min−1, and held for 0.65 min. Hydrogen was used as the carrier gas (28.7 mL min−1). The split ratio employed was 1
:
40, and both the injector and detector temperatures were maintained at 325 °C. Quantification was achieved using calibration curves with PS standards. The calibration curves were: cholesterol (range: 12.7–203.1 μg, y = 0.0066x − 0.0234, r = 0.9997), campesterol (range: 53.2–321.4 μg; y = 0.0049x − 0.0594, r = 0.9990), stigmasterol (range: 3.8–18.7 μg; y = 0.0045x − 0.0031, r = 0.9982), β-sitosterol (range: 1067.0–2766.4 μg; y = 0.0073x − 2.0724, r = 0.9995), β-sitosterol (range: 0.3–29.8 μg; y = 0.005x + 0.0008, r = 0.9998), sitostanol (range: 230.3–474.3 μg; y = 0.0041x − 0.3085, r = 0.9999), sitostanol (range: 12.8–127.9 μg; y = 0.0026x + 0.0006, r = 0.9975). For quantifying Δ5-avenasterol, Δ5,24-stigmastadienol, Δ7-stigmastenol, and Δ7-avenasterol in the BF of WRB, the β-sitosterol calibration curve ranged 0.3–29.8 μg was developed since commercial standards were unavailable. Additionally, campestanol was quantified using sitostanol curve ranged 12.8–127.9 μg.
2.5 Cell lines
Human colonic epithelial adenocarcinoma cells (Caco-2) and murine macrophage/monocyte (RAW264.7) cell lines were procured from the American Type Culture Collection (HTB-37 and TIB-71, respectively; Rockville, MD, USA). Both cell lines were cultured in Dulbecco's modified Eagle's Medium High Glucose (4.5 g L−1 glucose), supplemented with 10% (v/v) fetal bovine serum, 100 U mL−1 penicillin, and 100 μg mL−1 streptomycin. The cells were cultured in a controlled environment at 37 °C with 5% (v/v) CO2 at a constant level of 95% relative humidity.
2.6 Cell viability assay
To evaluate the anti-inflammatory properties of BF in the cellular model, the appropriate dilution of BF and incubation time (i.e., no cytotoxic effects) was determined using the MTT assay, according to methodology described previously.27 For this purpose, Caco-2 cells were seeded at a density of 26
500 cells per well in a 96-well plate and incubated for 8 days to promote their differentiation.13 Then, the cells were exposed to BF diluted with culture medium (1/10, 1/20, and 1/30, v/v) during different times (30, 60, and 90 min). Subsequently, the BF was removed, and cells were incubated with MTT solution (0.1 mg mL−1) during 2 h. After discarding MTT, the resulting formazan salts were dissolved in DMSO, and the absorbance of the resulting-coloured solution was measured with a multi-well spectrophotometer at 570 nm (LTEK A-302 plate reader, INNO, Seongnam, Republic of Korea).
2.7 Co-culture model of intestinal inflammation
The co-culture system of Caco-2 cells and RAW264.7 macrophages was carried out as indicated by Tanoue et al.15 with some modifications. The concentration and exposure time of LPS was modified with respect to that indicated by Tanoue et al.15 to achieve significant stimulation in the cellular model (data not shown). Briefly, Caco-2 cells were seeded in the apical compartment of Transwell® 12-well plates (with a membrane area of 1.12 cm2 and a pore size of 0.4 μm; Corning CoStar Corp., Cambridge, MA) at a density of 9 × 104 cells per well. One day before the 8-day differentiation of Caco-2 cells, RAW264.7 cells were seeded in a 12-well plate at a density of 3.4 × 105 cells per well and were maintained for 24 h. Then, Transwell® insert (on which the Caco-2 cells had been grown) were added into the 12-well plate preloaded with RAW264.7 macrophages. Following this, appropriately diluted BF from samples and digestion blank (1/20, v/v), were introduced into the apical compartment and incubated for 90 min. For the evaluation of pharmacological interaction between budesonide and the sample, budesonide (1 μM) was added in the apical compartment, both as a standalone treatment and in combination with the BF. After 90 min-incubation, treatments were replaced with culture medium and RAW264.7 cells were subjected to an inflammatory stimulus by exposing them to LPS at 1 μg mL−1 for a period of 24 h. In addition, cells untreated with BF or LPS were used as a control.
2.8 ELISA
The assessment of inflammatory mediator's secretion by Caco-2 cells (interleukin (IL)-8) and RAW264.7 macrophages (IL-6, tumor necrosis factor (TNF)-α, and prostaglandin E2 (PGE2)) was conducted by quantifying their concentrations in the apical or basolateral media, respectively, using ELISA kits, following the manufacturer's instructions (Invitrogen, Frederick, USA).
2.9 Measurement of reactive oxygen species
Intracellular levels of reactive oxygen species (ROS) were measured in Caco-2 cells using DCFDA reagent.28 After LPS-treatment, 10 μL of whole protein extract of Caco-2 cells (see “2.10 Western Blot” section) were mixed with 10 μL of 10 μM DCFDA and 80 μL of PBS in 96-well microplates. After incubation (30 min, 37 °C), the fluorescence was measured by fluorescence spectroscopy with excitation/emission wavelength at 485/535 nm (Promega GloMax®, Milan, Italy). Data were normalized with protein concentrations.
2.10 Nitrite/nitrate determination
The evaluation of NO released by Caco-2 cells in the medium was carried out by measuring nitrite/nitrate (NOx) level using the VCl3/Griess assay, as previously documented.29 Briefly, 100 μL of the basolateral culture medium were mixed with 100 μL of 0.8% (w/v) VCl3 (prepared in 1M HCl), 50 μL of 2% (w/v) sulphanilamide (prepared in 5% (v/v) H3PO4) and 50 μL of 0.1% (w/v) NED (prepared in ultrapure water) onto 96-well plate. Subsequently, the plate was incubated for 30 min in the dark at 37 °C, and the absorbance was then measured at 540 nm using a plate reader. NOx concentration was determined by interpolation to an external calibration curve of sodium nitrate within the range of 1.56–100 μM.
2.11 Western Blot analysis
Protein extraction and analysis by western blot were carried out according to the methodology described by Restivo et al.28 RAW264.7 macrophages were lysed in hypotonic buffer (10 mM HEPES, 1.5 mM MgCl2, 10 mM KCl, 0.1 mM DTT, 1% (v/v) phosphatase inhibitor and 1% (v/v) protease inhibitor) during a 15 min incubation on ice. The resulting lysate was clarified by centrifugation (12000g, 20 min at 4 °C) to obtain cytosolic fraction (i.e., supernatant). Subsequently, pellet was lysed (20 mM HEPES, 25% glycerol, 0.42 mM NaCl, 1.5 mM MgCl2, 0.2 mM EDTA, 0.1 mM DTT, 1% (v/v) phosphatase inhibitor and 1% (v/v) protease inhibitor) during a 30 min incubation on ice and centrifugated (20
000g, 10 min at 4 °C) to obtain the nuclear fraction. Regarding Caco-2 cells, they were lysed on ice-cold buffer RIPA containing 50 mM Tris-HCl (pH 7.4), 150 mM NaCl, 1% Triton X-100, 0.1% SDS, 1% (v/v) phosphatase inhibitor and 1% (v/v) protease inhibitor. Lysates were centrifuged (12
000g, 4 °C for 10 min) and the supernatants correspond to whole-cell protein extract. After protein quantification (Bradford′s method), 50 μg was subjected to 10% sodium dodecyl sulfate polyacrylamide gel electrophoresis and then electroblotted onto polyvinylidene fluoride membranes. Membranes were blocked in 5% (w/v) skimmed dry milk (1 h, room temperature) and subsequently incubated in the presence of the corresponding primary antibodies diluted 1/500 (v/v) (against cyclooxygenase 2 (COX-2), nuclear factor (NF)-κB p65, inducible nitric oxide synthase (iNOS), β-actin and laminin B) overnight at 4 °C. After incubation for 90 min at room temperature in the presence of HRP-conjugated secondary antibodies (1/2000, v/v), proteins were visualised by using an enhanced chemiluminescent substrate (ECL™ Prime Western Blotting System). Chemiluminescent bands were evaluated with a C-Digit Blot Scanner (LI-COR, Lincoln, NE, USA) and band intensities were analysed using ImageJ.
2.12 Estimation of combination index
The interaction between samples and budesonide to reduce the levels of pro-inflammatory mediators was evaluated by calculating the combination index (CI) based on the methodology described by Chou30 and adapted by Alvarez-Sala et al.31 The CI was determined using the following formula: CI = (%sample + budesonide)/(%sample + %budesonide), where ‘%sample’ and ‘%budesonide’ represent the reduction percentage of the individual treatment with samples and budesonide, respectively. Meanwhile, ‘%sample + budesonide’ represents the reduction percentage achieved with the combined treatment. An CI ratio less than, equal to, and greater than 1 indicates antagonism, addition, and synergy, respectively.
2.13 Statistical analysis
Data normality was assessed using the Shapiro–Wilk test, while the Levene test was used to evaluate homoscedasticity. One-way analysis of variance, followed by post-hoc HSD Tukey test, was applied to determine statistically significant differences (p < 0.05), using Statgraphics Plus 5.1 software (Statpoint Technologies Inc., Warrenton, VA, USA).
3. Results
3.1 Cytotoxicity of samples
To initiate the assessment of the anti-inflammatory potential of the samples, an evaluation of their cytotoxic effects on differentiated Caco-2 cells was conducted to select the optimal dilution and exposure time. Table 1 illustrates that the BF of digestion blank exhibited no cytotoxicity on Caco-2 cells, regardless of the tested dilutions (ranging from 1/10 to 1/30, v/v) and exposure durations (30, 60, and 90 min). Contrastingly, the BF from the PS-FS, at the lowest dilution (1/10, v/v), led to a notable decrease in cell viability across all three exposure times, with reductions of 22.7, 16.1, and 20.0%, respectively, compared to the untreated cell control (p < 0.05). This trend was similarly observed with the PS-enriched beverage, displaying comparable cytotoxicity, albeit additionally at the 1/30 (v/v) dilution after 90 min of treatment (16.5%). Remarkably, the BF derived from PS-enriched rye bread exhibited the highest toxicity. In this sense, the 1/10 (v/v) dilution significantly reduced cell viability at all exposure times (18.6–39.7%) compared to untreated cells (p < 0.05). Likewise, the 1/20 (v/v) dilution slightly reduced cell viability by 12%.
Table 1 Evaluation of the viability of 8-day differentiated Caco-2 cells after treatment with bioaccessible fractions of digestion blank, plant sterol (PS) food supplement, beverage or rye bread enriched with PS
Cell viability (% vs. control) |
Dilution (v/v) |
30 min |
60 min |
90 min |
Data are expressed as mean ± SD (n = 3). Asterisk (*) indicates statistically significant difference (p < 0.05) compared to control cells (i.e., untreated cells). |
Control cells |
100 ± 4.6 |
100 ± 4.0 |
100 ± 4.4 |
Digestion blank |
1/10 |
99.5 ± 1.4 |
97.9 ± 8.1 |
91.6 ± 2.8 |
1/20 |
103.7 ± 0.8 |
94.7 ± 2.5 |
98.6 ± 1.2 |
1/30 |
105.6 ± 1.2 |
96.8 ± 3.9 |
97.1 ± 2.0 |
PS food supplement |
1/10 |
77.3 ± 8.1* |
83.9 ± 4.2* |
80.0 ± 0.5* |
1/20 |
104.8 ± 2.9 |
97.7 ± 2.6 |
98.6 ± 0.3 |
1/30 |
96.5 ± 6.1 |
98.6 ± 3.9 |
97.1 ± 0.8 |
PS-enriched beverage |
1/10 |
70.3 ± 1.8* |
74.3 ± 19.0* |
93.5 ± 0.1* |
1/20 |
90.4 ± 14.5 |
94.2 ± 2.6 |
91.7 ± 8.4 |
1/30 |
96.8 ± 10.1 |
98.9 ± 0.6 |
83.5 ± 0.6* |
PS-enriched rye bread |
1/10 |
81.4 ± 1.5* |
78.2 ± 14.2* |
60.3 ± 1.1* |
1/20 |
98.0 ± 1.0 |
97.3 ± 2.4 |
88.0 ± 2.2* |
1/30 |
97.6 ± 1.2 |
94.3 ± 10.1 |
95.8 ± 2.0 |
In view of the results obtained, the 1/20 (v/v) dilution and exposure time of 90 min were selected for subsequent tests to evaluate the anti-inflammatory activity. This condition allows the maximum possible concentration of PS to be reached and for the longest possible time, without a general overtly cytotoxic effect of samples. The amount of cholesterol and PS present in each sample applying the corresponding 1/20 (v/v) dilution is shown in Table 2, highlighting the greatest concentration of these in the BF of rye bread (67.8 μM) compared to the PS-FS (13.0 μM) and beverage (20.5 μM).
Table 2 Sterol concentration (μM) in bioaccessible fractions of digestion blank, plant sterol (PS) food supplement, beverage and rye bread enriched with PS (applying dilution 1/20 v/v with culture medium)
|
Digestion blank |
PS food supplement |
PS-enriched beverage |
PS-enriched rye bread |
Data are expressed as mean ± SD (n = 3). Different letters (a–c) indicate statistically significant difference (p < 0.05) in each sterol concentration between samples. |
Cholesterol |
2.63 ± 0.02b |
1.91 ± 0.05a |
2.86 ± 0.17b |
2.31 ± 0.04c |
Campesterol |
— |
0.63 ± 0,03a |
1.32 ± 0.03b |
4.89 ± 0.10c |
Campestanol |
— |
0.38 ± 0.00a |
1.09 ± 0.09b |
1.81 ± 0.08c |
Stigmasterol |
— |
0.05 ± 0.00a |
0.11 ± 0.01b |
0.42 ± 0.03c |
β-Sitosterol |
— |
9.27 ± 0.23a |
13.97 ± 0.21b |
47.16 ± 0.82c |
Sitostanol |
— |
2.58 ± 0.06a |
3.96 ± 0.07b |
12.66 ± 0.32c |
Δ5-Avenasterol |
— |
0.02 ± 0.00a |
— |
0.05 ± 0.00b |
Δ5,24-Stigmastadienol |
— |
0.03 ± 0.00a |
— |
0.18 ± 0.00b |
Δ7-Stigmastenol |
— |
0.03 ± 0.01a |
— |
0.41 ± 0.01b |
Δ7-Avenasterol |
— |
0.03 ± 0.00a |
— |
0.27 ± 0.01b |
Total PS |
— |
13.02 ± 0.34a |
20.46 ± 0.22b |
67.84 ± 1.35c |
3.2 Regulation of the pro-inflammatory cytokine secretion
The secretion of pro-inflammatory cytokines by cells represents a crucial biomarker of response to an inflammatory stimulus. In the current study, it was employed species-specific antibodies to elucidate the cell responsible (Caco-2 or RAW264.7) for cytokine secretion. In this regard, exposure to LPS (1 μg mL−1) for 24 h in the basolateral compartment of Transwell®-like system induced the secretion of TNF-α and IL-6 by RAW264.7 macrophages. This led to a significant increase (p < 0.05) in the concentration of these mediators in the basolateral medium compared to the control (3 and 164-fold change over control, respectively) (Fig. 1). Additionally, the pro-inflammatory response exposure was extended to Caco-2 cells, as evidenced by an increase (p < 0.05) in the concentration of IL-8 in apical medium (1.9-fold change over control). As expected, pre-treatment with digestion blank did not modify the LPS-induced secretion of pro-inflammatory cytokines in both cell lines, compared to cells exposed only to LPS. Nevertheless, the PS-FS exhibited protective effects against secretion, showing a significant reduction (p < 0.05) on levels of TNF-α (9%), IL-6 (54%), and IL-8 (28%), compared to digestion blank + LPS. The efficacy of PS-FS resembled that observed with pre-treatment using budesonide (1 μM), highlighting its potent activity in mitigating intestinal inflammation. In contrast, the BF of beverage and bread enriched with PS did not demonstrate a reduction in these inflammation parameters. Consequently, the analysis of the following parameters was carried out only for the samples with a potential anti-inflammatory effect, PS-FS and/or budesonide. In addition, due to the absence of effect of the digestion blank, its use was not considered in the analyses of the following parameters.
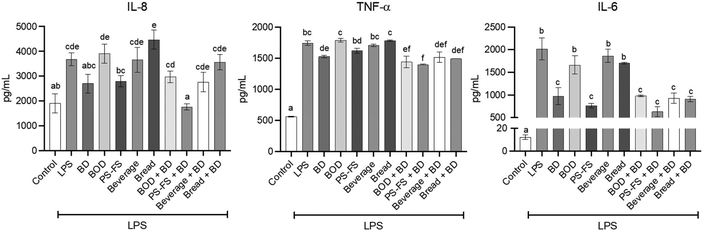 |
| Fig. 1 Levels of pro-inflammatory cytokines in apical (IL-8) and basolateral medium (TNF-α and IL-6) in a bi-compartmental model comprising differentiated Caco-2 cells (apical) and RAW264.7 macrophages (basolateral). The Caco-2 cells were pre-treated for 90 min with the bioaccessible fraction of a blank of digestion (BOD), plant sterol (PS) food supplement (PS-FS), as well as PS-enriched beverage and bread, followed by treatment with lipopolysaccharide (LPS) at a concentration of 1 μg mL−1 for 24 h (basolateral). Budesonide (BD) at a concentration of 1 μM served as a positive control. Data are expressed as mean ± standard deviation (n = 3). Statistically significant differences (p < 0.05) between conditions for the same cytokine are indicated by different letters (a–f). | |
3.3 Improvement of cellular oxidative/nitrogen balance
Oxidative stress is an important factor in inflammation, evidenced through the generation of reactive oxygen and nitrogen species. Due to its importance, an evaluation of intracellular ROS levels in Caco-2 cells was performed. In addition, iNOS protein expression in Caco-2 cells and NOx concentration in the apical medium were measured. The results showed a 3.7, 1.3, and 1.4-fold increase in the levels of ROS, iNOS, and NOx (p < 0.05), respectively, after LPS exposition, compared to control cells (Fig. 2). The exposition to PS-FS or budesonide as a pre-treatment inhibited the intracellular production of ROS in Caco-2 cells (47 and 28%, respectively). Additionally, PS-FS and budesonide attenuated the LPS-induced iNOS protein expression (48 and 27%, respectively) and totally decreased the NOx values, reaching control value, compared to LPS treated cells.
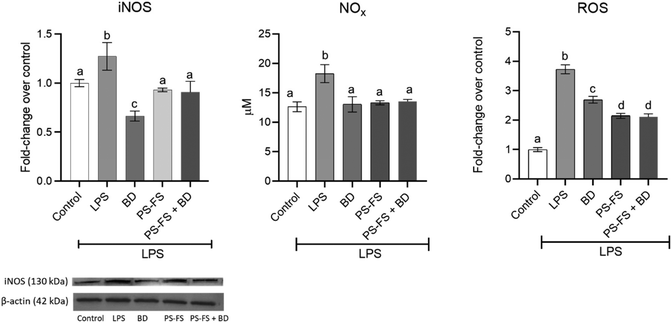 |
| Fig. 2 Protein expression of inducible nitric oxide synthase (iNOS) (normalized with β-actin) in Caco-2 cells, levels of nitrite/nitrate (NOx) in the apical medium and intracellular levels of reactive species oxygen (ROS) in Caco-2 cells in a bi-compartmental model comprising differentiated Caco-2 cells (apical) and RAW264.7 macrophages (basolateral). The Caco-2 cells were pre-treated for 90 min with the bioaccessible fraction of a plant sterol (PS) food supplement (PS-FS), followed by treatment with lipopolysaccharide (LPS) at a concentration of 1 μg mL−1 for 24 h (basolateral). Budesonide (BD) at a concentration of 1 μM served as a positive control. Data are expressed as mean ± standard deviation (n = 3). Statistically significant differences (p < 0.05) between conditions for the same parameter are indicated by different letters (a–c). | |
3.4 Regulation of inflammation-related pathway
Due to its importance in the regulation of the inflammatory response, the involvement of the NF-κB-COX-2-PGE2 signalling pathway was measured. The treatment with LPS induced a significant (p < 0.05) increases (vs. control) in the nuclear translocation of NF-κB p65 (2.8-fold change) in RAW264.7 macrophages, compared to control cells (Fig. 3). An increase in the expression of the COX-2 enzyme (1.8-fold change) and its product PGE2 (2.3-fold change) was also observed. A significant reduction (p < 0.05, vs. LPS) in NF-κB p65, COX-2, and PGE2 was observed in the pre-treatment with budesonide (65, 46, and 36%, respectively) and BF of PS-FS (39, 32, and 27%, respectively).
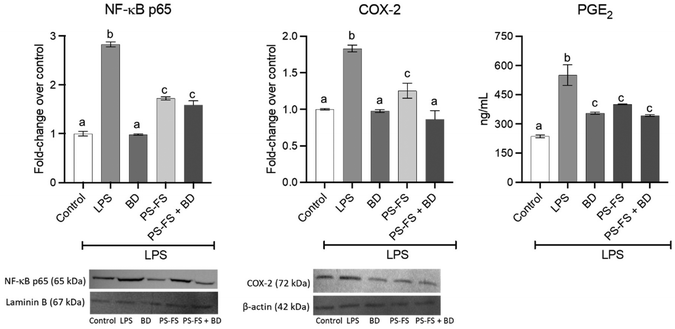 |
| Fig. 3 Protein expression of nuclear factor κB (NF-κB) p65 (normalized with laminin B) and cyclooxygenase 2 (COX-2) (normalized with β-actin) in nuclear and cytosolic fraction, respectively, in RAW264.7 macrophages, as well as levels of prostaglandin E2 (PGE2) in basolateral medium. The Caco-2 cells were pre-treated for 90 min with the bioaccessible fraction of a plant sterol (PS) food supplement (PS-FS), followed by treatment of RAW264.7 macrophages with lipopolysaccharide (LPS) at a concentration of 1 μg mL−1 for 24 h. Budesonide (BD) at a concentration of 1 μM served as a positive control. Data are expressed as mean ± standard deviation (n = 3). Statistically significant differences (p < 0.05) between conditions for the same parameter are indicated by different letters (a–c). | |
3.5 Pharmacological interaction between PS and budesonide
As described so far, budesonide and PS-FS have demonstrated similar effects, in terms of reduction of pro-inflammatory parameters such as TNF-α, IL-8, IL-6, NOx, and PGE2. The PS-FS even showed a greater reduction in intracellular levels of ROS.
However, it is necessary to highlight that in some parameters (COX-2, NF-κB p65 and iNOS) budesonide has been more effective, although it is true that these parameters are involved in the initial stages of the inflammatory response regulation pathway. The evaluation of the pharmacological interaction between budesonide and PS-FS has shown an additive interaction was observed in the effect to reduce TNF-α (CI, 1.13 ± 0.25) and IL-8 (CI, 1.07 ± 0.19) (Fig. 4). However, an antagonistic effect was observed for the rest of the pro-inflammatory markers, with CI values that ranged between 0.38 ± 0.10 and 0.63 ± 0.13.
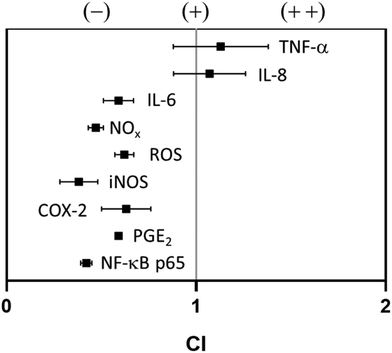 |
| Fig. 4 Pharmacological interaction between budesonide and a plant sterol food supplement in the reduction of different inflammation parameters, evaluated by calculating the combination index (CI). An CI ratio less than, equal to, and greater than 1 indicates antagonism (–), addition (+), and synergy (++), respectively. Data are expressed as mean ± standard deviation (n = 3). COX-2, cyclooxygenase 2; IL-8/6, interleukin 8/6; iNOS, inducible nitric oxide synthase; NF-κB p65, nuclear factor κB p65; NOx, nitrite/nitrate; PGE2, prostaglandin E2; ROS, reactive oxygen species; TNF-α, tumoral necrosis factor α. | |
4. Discussion
Inflammatory bowel diseases are a group of pathologies characterised by an excessive inflammatory response in the gastrointestinal tract.32 Two main clinical phenotypes stand out, ulcerative colitis and Chron's disease. Due to the growing prevalence of these diseases,33 it is of great interest to identify new possible alternatives to conventional treatments, such as dietary bioactive compounds. Previous in vitro studies showed that β-sitosterol standard (0.01–16 μM)10,11 or BF of a PS-enriched milk-based fruit beverage13 reduced the levels of pro-inflammatory cytokines such as IL-6, IL-8, and TNF-α in macrophages (RAW264.7 or J774A.1) (6–64%) or Caco-2 intestinal cells (40–50%) stimulated with LPS, phorbol 12-myristate 13-acetate (PMA) or IL-1β. Additionally, it has been demonstrated that β-sitosterol (50–250 μM) reduce the production of NO (51–70%) and ROS (28–49%) in RAW264.7 macrophages stimulated with PMA.9 These anti-inflammatory effects were associated with an inhibition in activation (33%) and nuclear translocation (16–45%) of NF-κB p65.9,11–13
The gut anti-inflamatory activity of PS was also described in studies with experimental animals. In this sense, a downregulation of cytokines such as IL-6 and TNF-α (22–94%) was reported in murine models of intestinal inflammation induced by DSS, 2,4,6-trinitrobenzene sulfonic acid, or high fat diet after treatment with β-sitosterol (10–20 mg kg−1),5–7 stigmasterol (enriched diet at 0.4%, w/w),7 or γ-oryzanol (50–100 mg kg−1).4 Kim et al.6 observed a reducing effect on iNOS expression after treatment with β-sitosterol (20 mg kg−1) in a murine model of high fat diet-induced colitis. In addition, these studies confirmed the inhibitory effect of PS on the activation (17%) or nuclear translocation of NF-κB (8%) and COX-2 expression (25–34%).4–7 A research conducted by our research group revealed that a milk-based beverage enriched with PS notably alleviated symptoms (24–67%), enhanced colon length (18%) and mitigating colonic mucosal damage (10–34%) in a murine model of DSS-induced colitis.8 However, galactooligosaccharides could interfere with the anti-inflammatory activity of a PS-enriched milk-based fruit beverage, resulting in increased occurrences of bloody stools, elevated immune cell infiltration into colon tissue, and increased myeloperoxidase activity.
The present study reported a reduction of LPS-induced pro-inflammatory cytokine secretion (IL-6, IL-8, and TNF-α) in a co-culture model of Caco-2/RAW264.7 cells. Despite observing an anti-inflammatory effect of PS-FS, PS-enriched beverage and bread did not show a protective effect. It is noteworthy that, considering the 1/20 (v/v) dilution of the BF, the PS concentration in the PS-FS (13.0 μM) is lower than that in the beverage (20.5 μM) and bread (67.8 μM). This suggests that the lack of activity observed is not attributed to a failure in the release of PS from the food matrix during digestion, but rather to a possible negative interaction between PS and food components of the matrix once released. Regarding beverage, the milk could be a possible interferent. According to Llewellyn et al.,34 a high casein (41%, w/w) diet caused intestinal barrier disruption and elevated colonic cytokine levels (IL-6 and TNF-α) in a murine model of intestinal inflammation induced by 3% (w/v) DSS. Additionally, an in vitro study observed an increase in IL-6 (238%), IL-8 (115%), and TNF-α (205%) production by Caco-2 cells and RAW264.7 macrophages (115–308%) after treatment with digested UHT cow milk obtained with INFOGEST digestion method.35 This pro-inflammatory effect was also observed with digested raw milk, which increase in RAW264.7 macrophages the production of TNF-α (150%) and IL-6 (321%). The authors suggested that the pro-inflammatory effects of milk are associated with the action of milk proteins and the products of the Maillard reaction. The same trend has been described for rye bread. A study in mice with genetically induced colitis (Casp8ΔIEC) observed that diets containing 50% (w/w) of different types of rye bread led to harmful effects (loss of body weight and increased mortality) compared to a diet without rye bread.36 The authors could not determine which components of bread were responsible for these self-defeating effects, although they suggest that unknown inflammatory molecules in bread could be behind this effect. Additionally, the presence of gliadins could explain the lack of anti-inflammatory effect of PS-enriched rye bread. In this context, a study using a cell co-culture with Caco-2/HT29-MTX cells found that gliadin treatment reduced antioxidant enzyme activity (glutathione reductase and glutathione-S-transferase), levels of reduced glutathione and increased IL-8 production.37 According to published studies that report counterproductive effects of milk and rye bread on the intestinal inflammatory response, we hypothesize that the food components of the milk-based fruit beverage and rye bread could interfere with the anti-inflammatory activity of PS, therefore being considered unsuitable vehicles for the management of inflammatory bowel disorders.
Since an anti-inflammatory effect was only seen with the use of PS-FS, it was decided to further investigate its mechanism of action. The protective effect of PS-FS was correlated with a reduction in the production of reactive oxygen and nitrogen species. Lower NOx levels were associated with an inhibition in the expression of the enzyme involved in its production (iNOS).
On the other hand, the results of this study show that NF-κB-COX-2-PGE2 signalling pathway could be a therapeutic target of PS. This pathway starts with the activation of NF-κB by different pathways, such as increased ROS production.38 In this activation, NF-κB subunits, such as p65, are translocated to the nucleus, where they increase the expression of cytokines and pro-inflammatory enzymes such as COX-2. This enzyme is involved in the production of PGE2 which acts as a positive regulator of the NF-κB pathway. Our study show that PS-FS gave rise to a lower NF-κB p65 nuclear translocation, lower COX-2 protein expression, and reduced PGE2 production.
The present study confirms the adequate anti-inflammatory efficacy of PS-FS, which in most parameters was equivalent to that observed with the positive control budesonide. This suggests that PS-FS may be a suitable substitute in patients who do not respond to budesonide or who show side effects to the drug, as well as in early stages of the disease where pharmacological intervention is not required. However, an antagonistic effect was reported when PS-FS and budesonide were combined as co-treatment. This suggests an opposite influence when administered simultaneously, which could be due to possible competition for binding sites or interference with signaling pathways crucial for their respective mechanisms of action. These antagonistic interactions underscore the need for cautious consideration when combining these agents in therapeutic regimens, as their simultaneous administration may compromise overall efficacy.
5. Conclusions
The present study elucidates the anti-inflammatory potential of PS when provided in the form of a food supplement. On the contrary, when PS are incorporated into milk-based fruit beverages and rye bread, this beneficial effect disappears, suggesting interference of the food matrix with the anti-inflammatory properties of PS. These findings underline the need for isolated formats that preserve the biological activity of PS, especially in the context of inflammatory bowel diseases. While the observed efficacy of PS-FS is promising, further research, particularly in humans, is essential to validate these findings and determine the translational relevance of PS-FS as a possible dietary alternative for inflammatory bowel disorders.
Author contributions
Nerea Faubel and Mussa Makran: investigation, formal analysis, and writing original draft; Ilenia Concetta Giardina: investigation; Reyes Barberá, Guadalupe Garcia-Llatas, Luisa Tesoriere, Alessandro Attanzio and Antonio Cilla: conceptualization, funding acquisition, project administration, supervision, and review & editing.
Conflicts of interest
There are no conflicts to declare.
Acknowledgements
This research is part of the project PID2019-104167RB-I00 funded by MCIN/AEI/10.13039/501100011033 and partially by Generalitat Valenciana (CIAICO/2021/076). Nerea Faubel holds an CPI-22-458 contract from Investigo Program (Generalitat Valenciana, Spain). Mussa Makran holds an FPU19/00156 grant from the Ministry of Sciences, Innovation and Universities (Spain).
References
- B. Salehi, C. Quispe, J. Sharifi-Rad, N. Cruz-Martins, M. Nigam, A. P. Mishra, D. A. Konovalov, V. Orobinskaya, I. M. Abu-Reidah, W. Zam, F. Sharopov, T. Venneri, R. Capasso, W. Kukula-Koch, A. Wawruszak and W. Koch, Phytosterols: From Preclinical Evidence to Potential Clinical Applications, Front. Pharmacol., 2021, 11, 599959 CrossRef PubMed.
- F. Marangoni and A. Poli, Phytosterols and cardiovascular health, Pharmacol. Res., 2010, 61, 193–199 CrossRef CAS PubMed.
- L. Fontané, J. Pedro-Botet, S. Garcia-Ribera, E. Climent, M. D. Muns, S. Ballesta, P. Satorra, J. A. Flores-Le Roux and D. Benaiges, Use of phytosterol-fortified foods to improve LDL cholesterol levels: A systematic review and meta-analysis, Nutr., Metab. Cardiovasc. Dis., 2023, 33, 1472–1480 CrossRef PubMed.
- M. S. Islam, T. Murata, M. Fujisawa, R. Nagasaka, H. Ushio, A. M. Bari, M. Hori and H. Ozaki, Anti-inflammatory effects of phytosteryl ferulates in colitis induced by dextran sulphate sodium in mice, Br. J. Pharmacol., 2008, 154, 812–824 CrossRef CAS PubMed.
- I. A. Lee, E. J. Kim and D. H. Kim, Inhibitory effect of β-sitosterol on TNBS-induced colitis in mice, Planta Med., 2012, 78, 896–898 CrossRef CAS PubMed.
- K. A. Kim, I. A. Lee, W. Gu, S. R. Hyam and D. H. Kim, β-Sitosterol attenuates high-fat diet-induced intestinal inflammation in mice by inhibiting the binding of lipopolysaccharide to toll-like receptor 4 in the NF-κB pathway, Mol. Nutr. Food Res., 2014, 58, 963–972 CrossRef CAS PubMed.
- S. Feng, Z. Dai, A. Liu, H. Wang, J. Chen, Z. Luo and C. S. Yang, β-Sitosterol and stigmasterol ameliorate dextran sulfate sodium-induced colitis in mice fed a high fat Western-style diet, Food Funct., 2017, 8, 4179–4186 RSC.
- G. López-García, A. Cilla, R. Barberá, A. Alegría and M. C. Recio, Effect of a milk-based fruit beverage enriched with plant sterols and/or galactooligosaccharides in a murine chronic colitis model, Foods, 2019, 8, 114 CrossRef PubMed.
- J. J. Moreno, Effect of olive oil minor components on oxidative stress and arachidonic acid mobilization and metabolism by macrophages RAW 264.7, Free Radical Biol. Med., 2003, 35, 1073 CrossRef CAS PubMed.
- Y. Ding, H. T. Nguyen, S. I. Kim, H. W. Kim and Y. H. Kim, The regulation of inflammatory cytokine secretion in macrophage cell line by the chemical constituents of Rhus sylvestris, Bioorg. Med. Chem. Lett., 2009, 19, 3607 CrossRef CAS PubMed.
- M. Valerio and A. B. Awad, β-Sitosterol down-regulates some pro-inflammatory signal transduction pathways by increasing the activity of tyrosine phosphatase SHP-1 in J774A.1 murine macrophages, Int. Immunopharmacol., 2011, 11, 1012 CrossRef CAS PubMed.
- J. H. Cheon, J. S. Kim, J. M. Kim, N. Kim, H. C. Jung and I. S. Song, Plant sterol guggulsterone inhibits nuclear factor-kappaB signaling in intestinal epithelial cells by blocking IkappaB kinase and ameliorates acute murine colitis, Inflamm. Bowel Dis., 2006, 12, 1152 CrossRef PubMed.
- G. López-García, A. Cilla, R. Barberá and A. Alegría, Anti-inflammatory and cytoprotective effect of plant sterol and galactooligosaccharides-enriched beverages in Caco-2 Cells, J. Agric. Food Chem., 2020, 68, 1862–1870 CrossRef PubMed.
- M. D. C. Ponce de León-Rodríguez, J. P. Guyot and C. Laurent-Babot, Intestinal in vitro cell culture models and their potential to study the effect of food components on intestinal inflammation, Crit. Rev. Food Sci. Nutr., 2019, 59, 3648–3666 CrossRef PubMed.
- T. Tanoue, Y. Nishitani, K. Kanazawa, T. Hashimoto and M. Mizuno,
In vitro model to estimate gut inflammation using co-cultured Caco-2 and RAW264.7 cells, Biochem. Biophys. Res. Commun., 2008, 374, 565–569 CrossRef CAS PubMed.
- A. Olejnik, K. Kowalska, M. Olkowicz, J. Rychlik, W. Juzwa, K. Myszka, R. Dembczyński and W. Białas, Anti-inflammatory effects of gastrointestinal digested Sambucus nigra L. fruit extract analysed in co-cultured intestinal epithelial cells and lipopolysaccharide-stimulated macrophages, J. Funct. Foods, 2015, 19, 649–660 CrossRef CAS.
- C. Frontela-Saseta, R. López-Nicolás, C. A. González-Bermúdez, C. Martínez-Graciá and G. Ros-Berruezo G, Anti-inflammatory properties of fruit juices enriched with pine bark extract in an in vitro model of inflamed human intestinal epithelium: the effect of gastrointestinal digestion, Food Chem. Toxicol., 2013, 53, 94–99 CrossRef CAS PubMed.
- Commission Decision 2004/336/EC of 31 March 2004 authorizing the placing on the market of yellow fat spreads, milk based fruit drinks, yoghurt type products and cheese type products with added phytosterols/phytostanols as novel foods or novel food ingredients under Regulation (EC) No 258/97 of the European Parliament and of the Council, Off. J. Eur. Communities, 2004, L105, 49–51 Search PubMed.
- Commission Decision 2006/58/EC of 24 January 2006 authorizing the placing on the market of rye bread with added phytosterols/phytostanols as novel foods or novel food ingredients under Regulation (EC) No 258/97 of the European Parliament and of the Council, Off. J. Eur. Communities, 2006, L31, 18–20 Search PubMed.
- Commission Decision 2006/59/EC of 24 January 2006 authorizing the placing on the market of rye bread with added phytosterols/phytostanols as novel foods or novel food ingredients under Regulation (EC) No 258/97 of the European Parliament and of the Council, Off. J. Eur. Communities, 2006, L31, 21–23 Search PubMed.
- D. S. MacKay and P. J. Jones, Phytosterols in human nutrition: Type, formulation, delivery, and physiological function, Eur. J. Lipid Sci. Technol., 2011, 113, 1427–1432 CrossRef CAS.
- M. González-Larena, G. García-Llatas, M. C. Vidal, L. M. Sánchez-Siles, R. Barberá and M. J. Lagarda, Stability of plant sterols in ingredients used in functional foods, J. Agric. Food Chem., 2011, 59, 3624–3631 CrossRef PubMed.
- Commission Regulation (EU) No 686/2014 of 20 June 2014 amending Regulations (EC) No 983/2009 and (EU) No 384/2010 as regards the conditions of use of certain health claims related to the lowering effect of plant sterols and plant stanols on blood LDL-cholesterol, Off. J. Eur. Communities, 2014, L182, 27–30 Search PubMed.
- M. Makran, N. Faubel, G. López-García, A. Cilla, R. Barberá, A. Alegría and G. Garcia-Llatas, Sterol bioaccessibility in a plant sterol-enriched beverage using the INFOGEST digestion method: Influence of gastric lipase, bile salts and cholesterol esterase, Food Chem., 2022, 382, 132305 CrossRef CAS PubMed.
- N. Faubel, M. Makran, A. Cilla, A. Alegría, R. Barberá and G. Garcia-Llatas, Bioaccessibility of Plant Sterols in Wholemeal Rye Bread Using the INFOGEST Protocol: Influence of Oral Phase and Enzymes of Lipid Metabolism, J. Agric. Food Chem., 2022, 70, 13223–13232 CrossRef CAS PubMed.
- A. Kondrashina, E. Arranz, A. Cilla, M. A. Faria, M. Santos-Hernández, B. Miralles, N. Hashemi, M. K. Rasmussen, J. F. Young, R. Barberá, G. Mamone, L. Tomás-Cobos, S. Bastiaan-Net, M. Corredig and L. Giblin, Coupling in vitro food digestion with in vitro epithelial absorption; recommendations for biocompatibility, Crit. Rev. Food Sci. Nutr., 2023, 1–19 CrossRef PubMed.
- M. Makran, G. Garcia-Llatas, A. Alegría and A. Cilla, Ethylcoprostanol modulates colorectal cancer cell proliferation and mitigates cytotoxicity of cholesterol metabolites in non-tumor colon cells, Food Funct., 2023, 14, 10829–10840 RSC.
- I. Restivo, M. G. Basilicata, I. C. Giardina, A. Massaro, G. Pepe, E. Salviati, C. Pecoraro, D. Carbone, S. Cascioferro, B. Parrino, P. Diana, C. Ostacolo, P. Campiglia, A. Attanzio, A. D'Anneo, F. Pojero, M. Allegra and L. Tesoriere, A Combination of Polymethoxyflavones from Citrus sinensis and Prenylflavonoids from Humulus lupulus Counteracts IL-1β-Induced Differentiated Caco-2 Cells Dysfunction via a Modulation of NF-κB/Nrf2 Activation, Antioxidants, 2023, 12, 1621 CrossRef CAS PubMed.
- K. M. Miranda, M. G. Espey and D. A. Wink, A rapid, simple spectrophotometric method for simultaneous detection of nitrate and nitrite, Nitric Oxide, 2001, 5, 62–71 CrossRef CAS PubMed.
- T. C. Chou, Drug combination studies and their synergy quantification using the Chou-Talalay method, Cancer Res., 2010, 70, 440–446 CrossRef CAS PubMed.
- A. Alvarez-Sala, M. A. Ávila-Gálvez, A. Cilla, R. Barberá, G. Garcia-Llatas, J. C. Espín and A. González-Sarrias, Physiological concentrations of phytosterols enhance the apoptotic effects of 5-fluorouracil in colon cancer cells, J. Funct. Foods, 2018, 49, 5209–5219 RSC.
- B. Gros, N. Plevris, N. Constantine-Cooke, M. Lyons, C. O'Hare, C. Noble, I. D. Arnott, G. R. Jones, C. W. Lees and L. A. A. P. Derikx, Multiple infliximab biosimilar switches appear to be safe and effective in a real-world inflammatory bowel disease cohort, United Eur. Gastroenterol. J., 2023, 11, 179–188 CrossRef CAS PubMed.
- S. C. Ng, H. Y. Shi, N. Hamidi, F. E. Underwood, W. Tang, E. I. Benchimol, R. Panaccione, S. Ghosh, J. C. Y. Wu, F. K. L. Chan, J. J. Y. Sung and G. G. Kaplan, Worldwide incidence and prevalence of inflammatory bowel disease in the 21st century: a systematic review of population-based studies, Lancet, 2017, 390, 2769–2778 CrossRef PubMed.
- S. R. Llewellyn, G. J. Britton, E. J. Contijoch, O. H. Vennaro, A. Mortha, J. F. Colombel, A. Grinspan, J. C. Clemente, M. Merad and J. J. Faith, Interactions Between Diet and the Intestinal Microbiota Alter Intestinal Permeability and Colitis Severity in Mice, Gastroenterology, 2018, 154, 1037–1046 CrossRef PubMed.
- E. I. P. Perceguetti, G. de Matuoka e Chiocchetti, D. P. Baptista, M. L. Gigante and J. A. Macedo, Does milk prevent or promote inflammation? Insights from in vitro assays, Food Biosci., 2024, 57, 103572 CrossRef.
- J. Zimmermann, L. De Fazio, V. Kaden-Volynets, B. Hitzmann and S. C. Bischoff, Consumption of Yeast-Fermented Wheat and Rye Breads Increases Colitis and Mortality in a Mouse Model of Colitis, Dig. Dis Sci., 2022, 67, 4422–4433 CrossRef CAS PubMed.
- S. da Silva, R. Pérez-Gregorio, N. Mateus, V. Freitas and R. Dias, Evidence of increased gluten-induced perturbations in the nucleophilic tone and detoxifying defences of intestinal epithelial cells impaired by gastric disfunction, Food Res. Int., 2023, 173, 113317 CrossRef CAS PubMed.
- M. J. Morgan and Z. G. Liu, Crosstalk of reactive oxygen species and NF-κB signaling, Cell Res., 2011, 21, 103–115 CrossRef CAS PubMed.
Footnotes |
† Electronic supplementary information (ESI) available. See DOI: https://doi.org/10.1039/d4fo00917g |
‡ These two authors should be considered joint first authors of the paper due to their equivalent contribution to the paper. |
|
This journal is © The Royal Society of Chemistry 2024 |
Click here to see how this site uses Cookies. View our privacy policy here.