DOI:
10.1039/D3FO04211A
(Paper)
Food Funct., 2024,
15, 689-703
Age differences in the impact of dietary salt on metabolism, blood pressure and cognitive function in male rats
Received
2nd October 2023
, Accepted 4th December 2023
First published on 6th December 2023
Abstract
The influence of salt consumption on physiological processes, especially blood pressure (BP), metabolism, and cognition, remains a topical concern. While guidelines endorse reduced salt diets, there are gaps in understanding the age-specific implications and challenges in adherence. The present study delved into the differential effects of salt intake on young adult and aged male rats over a 12-week period, using control, low-, and high-salt diets. Key metrics, such as BP, cognition, and general parameters, were monitored. Our findings revealed significant age-dependent effects of salt intake on survival rates, body weight, blood sodium, blood glucose, blood lipids, BP, heart rates, and cognition. Notably, young adult rats did not show significant sodium level changes on a high-salt diet, whereas aged rats experienced increased sodium levels even on a normal salt diet. Blood glucose levels decreased significantly in aged rats on a high-salt diet but remained stable in young adults. Aged rats had the highest survival rates on low-salt diets. Low-salt diets led to reduced BP in both age groups, more significantly in young adults. Young adult rats displayed increased BP variability on both high- and low-salt diets, while a decrease in BP variability was exclusive to aged rats on a low-salt diet. There were significant differences across age groups in short-term memory, but not in long-term memory. The study provides a nuanced understanding of the age-dependent physiological effects of salt intake, suggesting the necessity of age-specific guidelines for public health.
1 Introduction
The effect of salt intake on physiological functions is a topic of major importance, given its role in regulating blood pressure (BP) and potentially influencing cardiovascular diseases and cognitive function. Several existing guidelines recommend a low-salt diet for the entire population, suggesting that reducing salt intake can lower BP and, by extension, the incidence of cardiovascular diseases and cognitive impairments.1,2 Despite the availability of these comprehensive guidelines, the global implementation of sodium reduction strategies has been inconsistent.3 Moreover, the implications of varying salt intakes on metabolism, BP, particularly BP variability (BPV), and both short-term and long-term memory remain inconclusive across different age groups. The existing literature often neglects to account for age-specific variations in these health outcomes. Age is a key factor, with evidence suggesting unique risks and benefits of BP treatments for the elderly population.4 Regarding the impact of dietary salt on BP, older individuals generally are more salt sensitive, and thus at higher risk for developing hypertension.5
In addition, research of salt intake has traditionally been focused on its impact on humans; however, it is difficult to regulate experimentally in humans due to ethical considerations. There are distinct advantages in utilizing animal models, particularly rodents, to manipulate dietary conditions under strict controls and monitor the effects accurately and longitudinally, gaining invaluable insights. However, previous studies fed animals an exceptionally high-salt diet containing 8 to 20 times as much salt than those consumed by control animals, while the average salt intake in humans is usually about double the World Health Organization (WHO)'s suggested limit of 5 g per day.3,6–10 Therefore, rodent studies should employ lower salt concentrations, especially as new evidence emerges that the connection between salt intake and cardiovascular issues is only relevant in countries where the average consumption surpasses 5 g per day.9,10
Given the complex interplay between dietary salt, metabolism, BP, and cognitive function, this study aims to fill the research gap by examining the age-specific impacts of salt intake. Specifically, the research will investigate how dietary salt intake with clinical relevance affects metabolism, BP levels, BPV, and cognitive function across different age groups, and whether a low-salt diet can have beneficial effects. Through this multi-dimensional approach, the study aims to provide a nuanced understanding that can inform targeted public health recommendations.
2 Materials & methods
2.1 Animals and diets
Male Sprague-Dawley (SD) rats (young adult, 3 months old; and aged, 22–24 months old) were purchased from Slac Laboratory Animal (Shanghai, China) and housed in standard cages in a temperature-controlled facility with 12 h light/dark cycle. All animal experiments were approved by the Research Ethics Committee of Hangzhou Normal University. The animal experimental procedures followed the National Institutes of Health Guide for the Care and Use of Laboratory Animals and were conducted in adherence to ARRIVE. Rats received tap water ad libitum and normal control (0.4% NaCl), low-salt (0.1% NaCl), or high-salt (1% NaCl) chow (Xietong Biotechnology Co., Hangzhou, China) for 12 weeks. Animals were assigned randomly to the experimental groups. All animals qualified for inclusion in the study upon successfully completing baseline assessments but were excluded if they did not meet the criteria during baseline training. Data were collected and processed randomly. The individuals conducting the experiment measurements were blinded to group allocation. The person assessing, measuring, or quantifying the experimental outcomes were blinded to the intervention.
2.2 Blood pressure and heart rate measurements
At baseline and then 2, 4, 8, and 12 weeks after the onset of the diet, systolic blood pressure (SBP), diastolic blood pressure (DBP), mean blood pressure (MBP), pulse pressure (PP, SBP minus DBP), and heart rate (HR) were measured repeatedly using a noninvasive pressure device ALC-NIBP (Alcott Biotech, Shanghai, China). Measurements were obtained in conscious rats restrained in a thermal plastic chamber as described.11 Standard deviation (SD) and coefficients of variation (CVs) (SD × 100/mean, %) were calculated for the variability of SBP, DBP, MBP, PP, and HR.
2.3 Behavioral tests
Cognitive functions (T-maze and novel object recognition) were measured repeatedly at baseline, 4, 8, and 12 weeks after the onset of the diet.
2.3.1 Apparatus.
The spontaneous alternation task was carried out in a modifiable T-maze. The maze was made of black plastic, and the floors were 15 cm wide, and the walls were 40 cm high. The stem was 75.5 cm long and the cross piece was 136 cm long. An open black box (100 × 100 × 40 cm, long × wide × high) was used for the novel object recognition test. Two distinct objects were additionally added to the novel object recognition test. All behavioral tests were videotaped.
2.3.2 Procedure.
2.3.2.1 T-maze.
Spontaneous alternation allows a free choice of the goal arm on both the sample and choice trials. Briefly,12 the animal was placed in the start area and allowed to choose a goal arm. Then the animal was removed gently and re-placed in the start area. The animal was allowed to choose between two open goal arms. 5 trials were preformed and a percentage per animal was calculated.
2.3.2.2 Novel object recognition.
Briefly,13 animals were handled for 3 d before the initial test during the acclimation period. In the training trial, animals explored two identical objects (A1 and A2) for a period of 10 min. The animals were returned to the home cage for 1 h. In the testing trial, the animals explored a familiar sample object (A) and a novel object (B) for 5 min. Object exploration includes any direct contact with the mouth, nose, or paw; it should not include contacts that are thought to be accidental, such as bumping the object while passing by. The training and testing trials were videotaped for subsequent measurement and analysis of object exploration by an individual blind to the experimental conditions. A discrimination ratio (novel object interaction/total interaction with both objects) was calculated.
2.4 Physiological parameters
Physiological variables including sodium (Na+), potassium (K+), sodium to potassium ratios, and glucose were measured at the end point using an ISTAT portable clinical analyzer (Abott, IL, USA) and EC8+ cartridge (Abott, IL, USA).
2.5 Blood lipid determination by enzyme-linked immunosorbent assay (ELISA)
Blood lipid levels were measured using a lipid ELISA kit (Baiyi Bio, Shanghai, China). Plasma was collected using EDTA anticoagulant tubes and was centrifuged for 30 minutes at 3000g at 4 °C. Briefly, standards and samples were added followed by lipid HRP-conjugate reagent and incubated for 60 minutes at 37 °C. Each well was aspirated and washed five times. Chromogen solutions A and B were added, then gently mixed and incubated for 15 minutes at 37 °C (protected from light). A stop solution was added and absorbance at 450 nm was measured with an automated microplate reader. Lipid concentrations were expressed as mmol L−1. All experiments were performed in triplicate.
2.6 Statistical analysis
All statistical analyses were performed using the software Prism 9 (GraphPad). All values were expressed as mean ± SEM. Data were analyzed by one-way or two-way ANOVA with Tukey's post-hoc tests. Survival rate was analyzed using the Log-rank (Mantel–Cox) test. Alpha was set at 0.05 in all analyses.
3 Results
3.1 General parameters
During the dietary experiment, it was observed that the body weights of young adult rats uniformly exhibited a significant increase. However, rats on a high-salt diet had comparatively lower body weights than those on low-salt and control diets, as indicated in Fig. 1A. In contrast, aged rats on the high-salt diet experienced a decline in body weight, as shown in Fig. 1B. Nevertheless, the final body weights across all age groups and dietary conditions revealed no significant disparities, as illustrated in Fig. 1C. Specifically, rats in the low-salt (0.1% NaCl) group weighed an average of 793 ± 26 and 778 ± 47 g (young vs. aged), in the normal control salt (0.4% NaCl) group an average of 765 ± 21 and 757 ± 21 g (young vs. aged), and in the high-salt (1% NaCl) group an average of 751 ± 23 and 754 ± 25 g (young vs. aged).
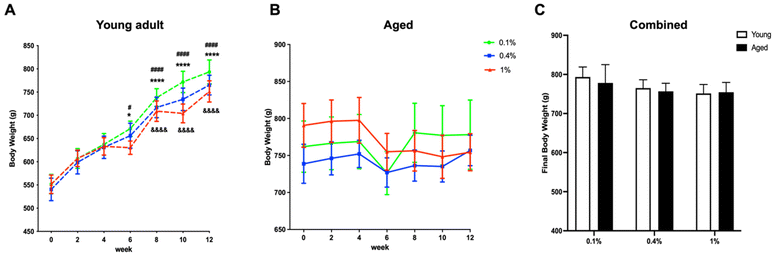 |
| Fig. 1 Age differences in the impact of dietary salt on the body weight in male Sprague-Dawley (SD) rats. Young adult (3 months old) and aged (22–24 months old) male SD rats received normal control (0.4% NaCl), low-salt (0.1% NaCl), or high-salt (1% NaCl) chow for 12 weeks. Body weights were measured bi-weekly. (A) Body weights of young adult rats; (B) body weights of aged rats; (C) final body weights of combined data from young adult and aged rats. Green dashed line, young adult rats on 0.1% NaCl diet; blue dashed line, young adult rats on 0.4% NaCl diet; red dashed line, young adult rats on 1% NaCl diet; green solid line, aged rats on 0.1% NaCl diet; blue solid line, aged rats on 0.4% NaCl diet; red solid line, aged rats on 1% NaCl diet. N = 9–14 per group. The results are presented as mean ± SEM. *, P < 0.05, week 6 compared to baseline; ****, P < 0.0001, week 8, 10, or 12 compared to baseline in 0.1% NaCl group; #, P < 0.05, week 6 compared to baseline; ####, P < 0.0001, week 8, 10, or 12 compared to baseline in 0.4% NaCl group; &&&&, P < 0.0001, week 8, 10, or 12 compared to baseline in 1% NaCl group. Statistical significance was determined using the two-way ANOVA with Tukey's post hoc test. | |
Low-salt intake did not show any observable changes in blood sodium levels between the young adult and aged rat groups. Interestingly, both the normal control diet and the high-salt diet significantly influenced blood sodium levels between the two age groups, as seen in Fig. 2A. The data imply that the ability to regulate sodium levels may be compromised in aged rats even on a normal control salt diet.
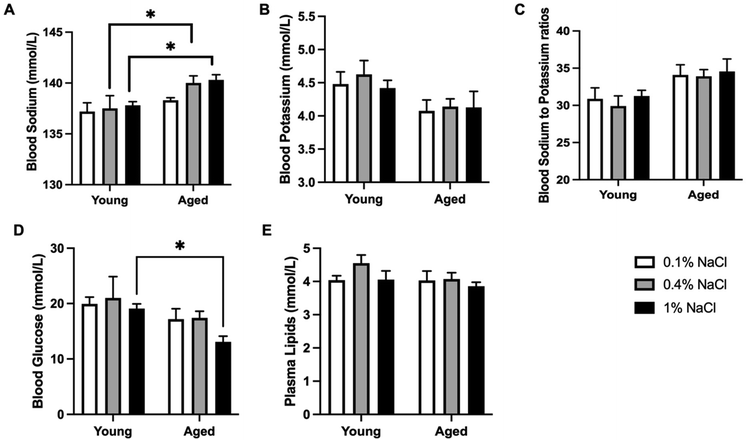 |
| Fig. 2 Age differences in the impact of dietary salt on the blood sodium and glucose levels in male SD rats. Young adult (3 months old) and aged (22–24 months old) male SD rats received normal control (0.4% NaCl), low-salt (0.1% NaCl), or high-salt (1% NaCl) chow for 12 weeks. Blood samples were collected at the end point and physiological variables including (A) sodium (Na+), (B) potassium (K+), (C) sodium to potassium ratios and (D) glucose were measured at the end point using an iSTAT portable clinical analyzer and EC8+ cartridge, as well as (E) plasma lipids were measured using a lipids enzyme-linked immunosorbent assay (ELISA) kit. White block, 0.1% NaCl diet; grey block, 0.4% NaCl diet; black block, 1% NaCl diet. N = 4–10 per group. The results are presented as mean ± SEM. *, P < 0.05, young adult compared to aged rats. Statistical significance was determined using the two-way ANOVA with Tukey's post hoc test. | |
Additionally, we found no marked differences in blood potassium levels (Fig. 2B) or sodium to potassium ratios across the groups (Fig. 2C). For blood glucose levels, aged rats on a high-salt diet showed a significant reduction, whereas this was not significant in young adult rats, as depicted in Fig. 2D. When assessing combined data from both age groups, it is evident that a high-salt diet considerably lowered blood glucose levels in aged rats in comparison with young adult rats, as shown in Fig. 2D. Furthermore, there was no significant difference in plasma lipid levels among the groups (Fig. 2E).
In terms of survival rates, all young adult rats survived during the study period. Among the aged rats, the highest survival rate was observed in those on the low-salt diet, as shown in Fig. 3.
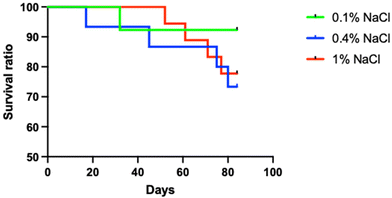 |
| Fig. 3 The impact of dietary salt on survival ratio in aged male SD rats. Young adult (3 months old) and aged (22–24 months old) male SD rats received normal control (0.4% NaCl), low-salt (0.1% NaCl), or high-salt (1% NaCl) chow for 12 weeks. All young adult rats survived during the study period (data not shown here). Among aged rats, the highest survival ratio was observed in those on the low-salt diet, although no significance was detected. Green solid line, aged rats on 0.1% NaCl diet; blue solid line, aged rats on 0.4% NaCl diet; red solid line, aged rats on 1% NaCl diet. N = 13–18 per group. Data was analyzed using the Log-rank (Mantel–Cox) test. | |
3.2 Conscious BP and HR levels and variability
In young adult rats, a low-salt diet led to significant reductions in SBP (Fig. 4A), DBP (Fig. 5A), and MBP (Fig. 6A) when compared to both a high-salt diet from the eighth week and a normal control diet starting from the twelfth week. Conversely, in aged rats (Fig. 4B, 5B and 6B), the low-salt diet only led to a significant decrease in SBP (Fig. 4B), when compared to the high-salt diet at the twelfth week.
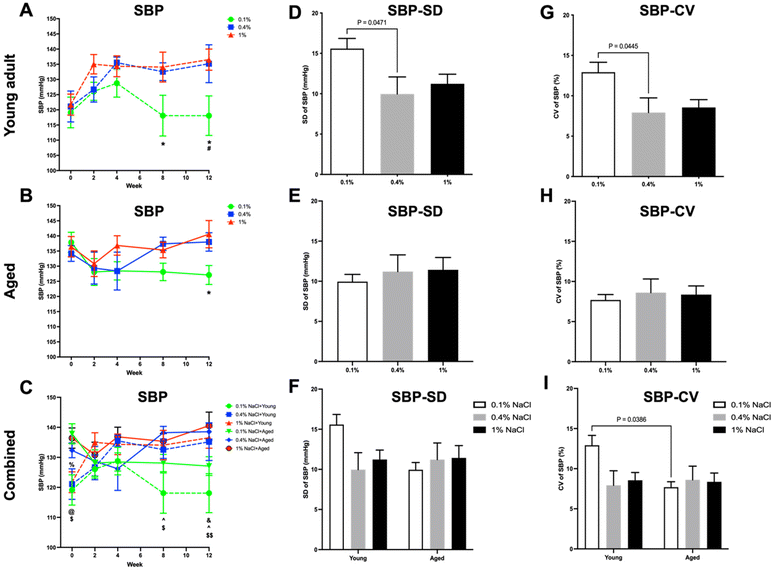 |
| Fig. 4 Age differences in the impact of dietary salt on systolic blood pressure (SBP) level and variability in male SD rats. Young adult (3 months old) and aged (22–24 months old) male SD rats received normal control (0.4% NaCl), low-salt (0.1% NaCl), or high-salt (1% NaCl) chow for 12 weeks. BP was measured repeatedly at baseline and then 2, 4, 8, and 12 weeks after the onset of the diet. SBP of (A) young adult rats, (B) aged rats, (C) both young adult and aged rats; SBP variability (standard deviation, SD) of (D) young adult rats, (E) aged rats, (F) both young adult and aged rats; SBP variability (coefficients of variation, CV) of (G) young adult rats, (H) aged rats, (I) both young adult and aged rats. Green dashed line, young adult rats on 0.1% NaCl diet; blue dashed line, young adult rats on 0.4% NaCl diet; red dashed line, young adult rats on 1% NaCl diet; green solid line, aged rats on 0.1% NaCl diet; blue solid line, aged rats on 0.4% NaCl diet; red solid line, aged rats on 1% NaCl diet. N = 8–12 per group. The results are presented as mean ± SEM. *, P < 0.05, 0.1% NaCl compared to 1% NaCl; #, P < 0.05, 0.1% NaCl compared to 0.4% NaCl. When data from both young adult and aged rats were combined, @, P < 0.05, young + 0.1% NaCl vs. aged + 0.1% NaCl; $, P < 0.05, $$, P < 0.01, young + 0.1% NaCl vs. aged + 1% NaCl; %, P < 0.05, young + 0.4% NaCl vs. aged + 0.1% NaCl; ^, P < 0.05, young + 0.1% NaCl vs. aged + 0.4% NaCl; &, P < 0.05, young + 0.1% NaCl vs. young + 1% NaCl; ^, P < 0.05, young + 0.1% NaCl vs. aged + 0.4% NaCl. Statistical significance was determined using the two-way ANOVA with Tukey's post hoc test. | |
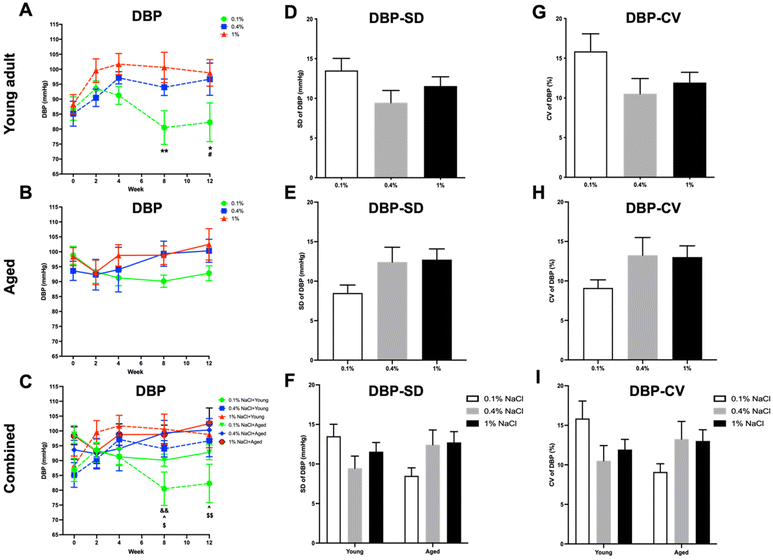 |
| Fig. 5 Age differences in the impact of dietary salt on diastolic blood pressure (DBP) level and variability in male SD rats. Young adult (3 months old) and aged (22–24 months old) male SD rats received normal control (0.4% NaCl), low-salt (0.1% NaCl), or high-salt (1% NaCl) chow for 12 weeks. BP was measured repeatedly at baseline and then 2, 4, 8, and 12 weeks after the onset of the diet. DBP of (A) young adult rats, (B) aged rats, (C) both young adult and aged rats; DBP variability (SD) of (D) young adult rats, (E) aged rats, (F) both young adult and aged rats; DBP variability (CV) of (G) young adult rats, (H) aged rats, (I) both young adult and aged rats. Green dashed line, young adult rats on 0.1% NaCl diet; blue dashed line, young adult rats on 0.4% NaCl diet; red dashed line, young adult rats on 1% NaCl diet; green solid line, aged rats on 0.1% NaCl diet; blue solid line, aged rats on 0.4% NaCl diet; red solid line, aged rats on 1% NaCl diet. N = 8–12 per group. The results are presented as mean ± SEM. *, P < 0.05, 0.1% NaCl compared to 1% NaCl; #, P < 0.05, 0.1% NaCl compared to 0.4% NaCl. When data from both young adult and aged rats were combined, &&, P < 0.01, young + 0.1% NaCl vs. young + 1% NaCl; ^, P < 0.05, young + 0.1% NaCl vs. aged + 0.4% NaCl; $, P < 0.05, $$, P < 0.01, young + 0.1% NaCl vs. aged + 1% NaCl. Statistical significance was determined using the two-way ANOVA with Tukey's post hoc test. | |
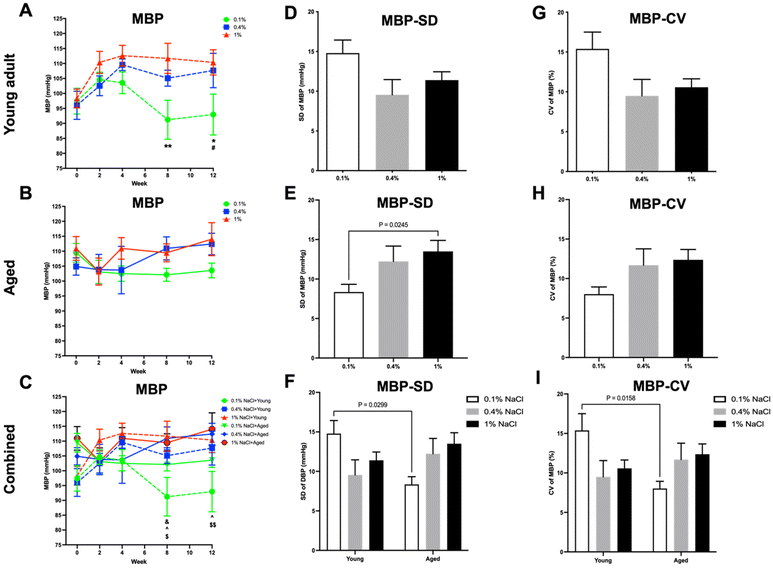 |
| Fig. 6 Age differences in the impact of dietary salt on mean blood pressure (MBP) level and variability in male SD rats. Young adult (3 months old) and aged (22–24 months old) male SD rats received normal control (0.4% NaCl), low-salt (0.1% NaCl), or high-salt (1% NaCl) chow for 12 weeks. BP was measured repeatedly at baseline and then 2, 4, 8, and 12 weeks after the onset of the diet. MBP of (A) young adult rats, (B) aged rats, (C) both young adult and aged rats; MBP variability (SD) of (D) young adult rats, (E) aged rats, (F) both young adult and aged rats; MBP variability (CV) of (G) young adult rats, (H) aged rats, (I) both young adult and aged rats. Green dashed line, young adult rats on 0.1% NaCl diet; blue dashed line, young adult rats on 0.4% NaCl diet; red dashed line, young adult rats on 1% NaCl diet; green solid line, aged rats on 0.1% NaCl diet; blue solid line, aged rats on 0.4% NaCl diet; red solid line, aged rats on 1% NaCl diet. N = 8–12 per group. The results are presented as mean ± SEM. *, P < 0.05, **, P < 0.01, 0.1% NaCl compared to 1% NaCl; #, P < 0.05, 0.1% NaCl compared to 0.4% NaCl. When data from both young adult and aged rats were combined, &&, P < 0.01, young + 0.1% NaCl vs. young + 1% NaCl; ^, P < 0.05, young + 0.1% NaCl vs. aged + 0.4% NaCl; $, P < 0.05, $$, P < 0.01, young + 0.1% NaCl vs. aged + 1% NaCl. Statistical significance was determined using the two-way ANOVA with Tukey's post hoc test. | |
When data from both young adult and aged rats were aggregated, baseline BP levels (SBP, DBP, and MBP; Fig. 4C, 5C and 6C) were found to be higher in the aged rats, although statistical significance was achieved only in SBP levels (Fig. 4C). Furthermore, young adult rats on a low-salt diet exhibited significantly lower levels of SBP (Fig. 4C), DBP (Fig. 5C), and MBP (Fig. 6C) compared to aged rats on either a normal control diet or a high-salt diet, starting from the eighth week.
No statistical significance was noted in PP levels across any of the groups (Fig. 7). Regarding HR (Fig. 8), aged rats displayed significantly lower HR at baseline when compared to young adult rats (Fig. 8C). Moreover, young adult rats on a normal control diet had a higher HR compared to aged rats on all three diets at the second week and compared to aged rats on a high-salt diet at the twelfth week (Fig. 8C).
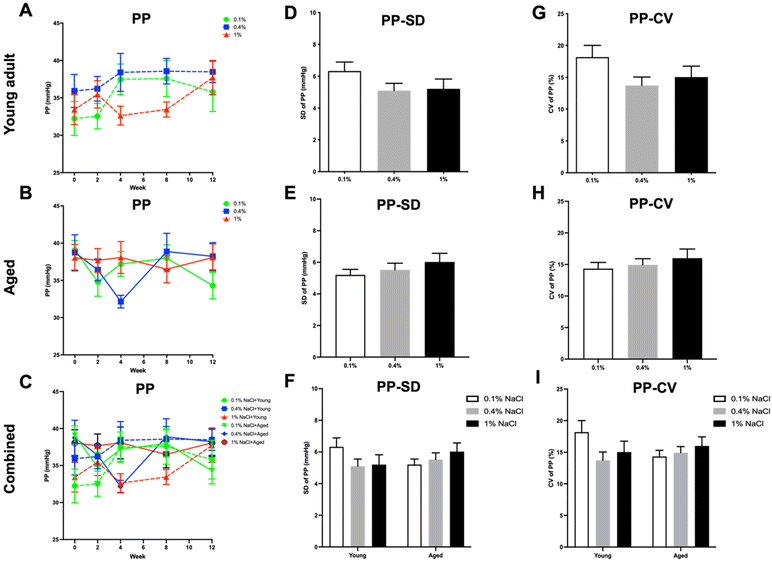 |
| Fig. 7 The impact of dietary salt on pulse pressure (PP) level and variability in young adult and aged male SD rats. Young adult (3 months old) and aged (22–24 months old) male SD rats received normal control (0.4% NaCl), low-salt (0.1% NaCl), or high-salt (1% NaCl) chow for 12 weeks. BP was measured repeatedly at baseline and then 2, 4, 8, and 12 weeks after the onset of the diet. PP of (A) young adult rats, (B) aged rats, (C) both young adult and aged rats; PP variability (SD) of (D) young adult rats, (E) aged rats, (F) both young adult and aged rats; PP variability (CV) of (G) young adult rats, (H) aged rats, (I) both young adult and aged rats. Green dashed line, young adult rats on 0.1% NaCl diet; blue dashed line, young adult rats on 0.4% NaCl diet; red dashed line, young adult rats on 1% NaCl diet; green solid line, aged rats on 0.1% NaCl diet; blue solid line, aged rats on 0.4% NaCl diet; red solid line, aged rats on 1% NaCl diet. N = 8–12 per group. The results are presented as mean ± SEM. Data was analyzed using the two-way ANOVA with Tukey's post hoc test. | |
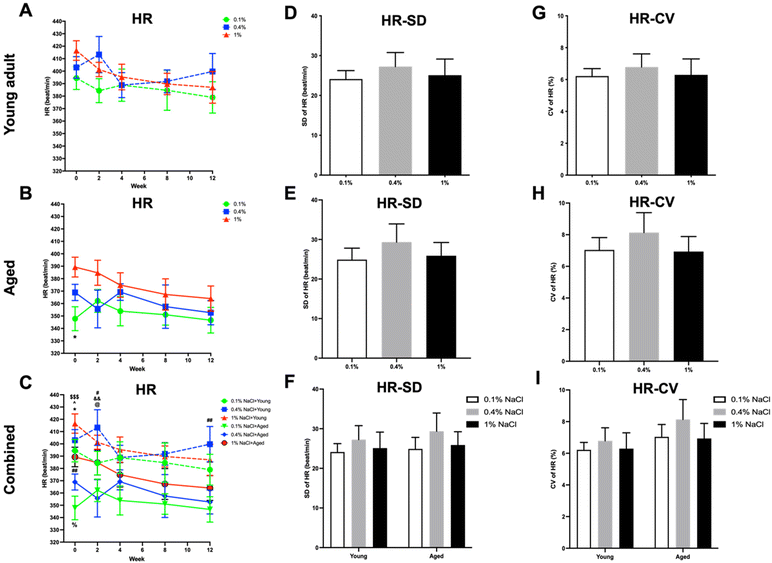 |
| Fig. 8 Age differences in the impact of dietary salt on heart rate (HR) level and variability in male SD rats. Young adult (3 months old) and aged (22–24 months old) male SD rats received normal control (0.4% NaCl), low-salt (0.1% NaCl), or high-salt (1% NaCl) chow for 12 weeks. HR was measured repeatedly at baseline and then 2, 4, 8, and 12 weeks after the onset of the diet. HR of (A) young adult rats, (B) aged rats, (C) both young adult and aged rats; HR variability (SD) of (D) young adult rats, (E) aged rats, (F) both young adult and aged rats; HR variability (CV) of (G) young adult rats, (H) aged rats, (I) both young adult and aged rats. Green dashed line, young adult rats on 0.1% NaCl diet; blue dashed line, young adult rats on 0.4% NaCl diet; red dashed line, young adult rats on 1% NaCl diet; green solid line, aged rats on 0.1% NaCl diet; blue solid line, aged rats on 0.4% NaCl diet; red solid line, aged rats on 1% NaCl diet. N = 8–12 per group. The results are presented as mean ± SEM. *, P < 0.05, 0.1% NaCl compared to 1% NaCl. When data from both young adult and aged rats were combined, %, P < 0.05, young + 0.1% NaCl vs. aged + 0.1% NaCl; #, P < 0.05, ##, P < 0.01, young + 0.4% NaCl vs. aged + 0.1% NaCl; &&, P < 0.05, young + 0.4% NaCl vs. aged + 0.4% NaCl; @, P < 0.05, young + 0.4% NaCl vs. aged + 1% NaCl; $$$, P < 0.001, young + 1% NaCl vs. aged + 0.1% NaCl; ^, P < 0.05, young + 1% NaCl vs. aged + 0.4% NaCl. Statistical significance was determined using the two-way ANOVA with Tukey's post hoc test. | |
In the context of BPV, both high-salt and low-salt diets led to an increase in the SD and CV of SBP (Fig. 4D and G), DBP (Fig. 5D and G), and MBP (Fig. 6D and G) in young adult rats, relative to those on a normal control diet. However, only the low-salt diet exhibited a statistically significant increase in both SD and CV for SBP (Fig. 4D and G). In contrast, aged rats on a low-salt diet exhibited a decrease in SD and CV for SBP (Fig. 4E and H), DBP (Fig. 5E and H), and MBP (Fig. 6E and H). Notably, only the SD of MBP (Fig. 6E) reached statistical significance in these reductions.
Upon integrating the data from both young adult and aged rats, it was observed that there were pronounced differences in CV for SBP (Fig. 4I) as well as SD and CV for MBP (Fig. 6F and I) between the young adult rats on a low-salt diet and the aged rats on the same diet. For PP (Fig. 7D–I) and HR (Fig. 8D–I) variability, no statistically significant differences were discerned across the groups.
3.3 Cognitive function
The novel object recognition test (Fig. 9A–C) was utilized to evaluate short-term memory, and a preference index—defined as the ratio of time spent with the novel object to total interaction time—was computed. It was observed that, in young adult rats (Fig. 9A) subjected to control diets, the preference index experienced a decline at the second week before reverting to the baseline level by the twelfth week. No discernible differences were noted in young adult rats on both low-salt and high-salt diets. Conversely, in the aged rats (Fig. 9B), the preference index remained consistent in the high-salt diet group but exhibited a decrease in the low-salt and control diet groups. Upon aggregating the data from both young adult and aged rats (Fig. 9C), pronounced disparities became apparent between young adult rats on a high-salt diet and aged rats on a control diet. The findings imply age differences in the influence of varying salt intakes on short-term memory.
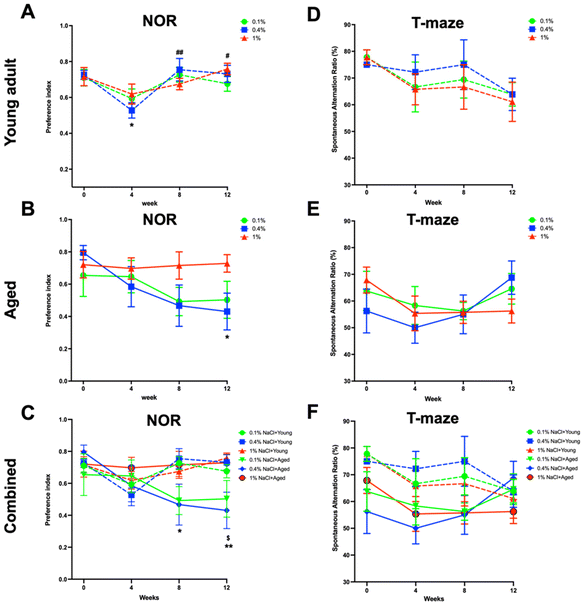 |
| Fig. 9 Age differences in the impact of dietary salt on memory function in male SD rats. Young adult (3 months old) and aged (22–24 months old) male SD rats received normal control (0.4% NaCl), low-salt (0.1% NaCl), or high-salt (1% NaCl) chow for 12 weeks. The novel object recognition (NOR, short-term memory) of (A) young adult rats, (B) aged rats, and (C) both young adult and aged rats; as well as T-maze (long-term memory) of (D) young adult rats, € aged rats, and (F) both young adult and aged rats, were measured repeatedly at baseline and then 4, 8 and 12 weeks after the onset of the diet. Green dashed line, young adult rats on 0.1% NaCl diet; blue dashed line, young adult rats on 0.4% NaCl diet; red dashed line, young adult rats on 1% NaCl diet; green solid line, aged rats on 0.1% NaCl diet; blue solid line, aged rats on 0.4% NaCl diet; red solid line, aged rats on 1% NaCl diet. N = 8–12 per group. The results are presented as mean ± SEM. *, P < 0.05, **, P < 0.01, rats on 0.4% NaCl diet, week 4, week 8, or week 12 compared to baseline; #, P < 0.05, ##, P < 0.01, rats on 0.4% NaCl diet, week 8 or week 12 compared to week 4; $, P < 0.05, young + 1% NaCl vs. aged + 0.4% NaCl. Statistical significance was determined using the two-way ANOVA with Tukey's post hoc test. | |
For the assessment of long-term memory and learning capabilities, the spontaneous alternation ratio in a T-maze (Fig. 9D–F) was measured. Despite an observed decline in T-maze performance associated with a high-salt diet, compared to both low-salt and control diets in both age groups, these deviations were not statistically significant.
4 Discussion
The current research represents a pioneering effort in elucidating the age-related differences in the effects of salt intake on metabolism, BP, and cognition in male rats. Notably, the study employed a clinically pertinent high-salt dosage—approximately twice the standard amount—to investigate its impact. We observed age differences in the impact of varying salt diets on survival rate, body weights, blood glucose, blood sodium, BP levels and variability, HR, and cognition. To be more specific, a high-salt diet did not notably impact sodium levels in young adult rats, but even a normal salt diet significantly elevated sodium levels in aged rats. However, no discernible changes were identified in blood potassium levels or sodium-to-potassium ratios across the various groups. Body weights of young adult rats uniformly exhibited a significant increase. However, rats on a high-salt diet had comparatively lower body weights than those on low-salt and control diets. In contrast, body weight declined in aged rats when subjected to a high-salt diet, although these changes were not statistically significant. Blood glucose levels significantly decreased in aged rats following a high-salt diet but remained stable in young adult rats. Remarkably, survival rate was highest among aged rats on a low-salt diet. We also found that baseline BP levels were elevated in aged rats compared to their younger counterparts. Intake of a low-salt diet led to a significant reduction in BP levels across both age groups, with greater reduction effects in young adult rats. Additionally, in young adult rats, both high-salt and low-salt diets increased BPV when compared to a normal control diet, whereas only the low-salt diet led to a decrease in BPV among aged rats. HR at baseline and throughout the dietary intervention was notably lower in the aged rats. Moreover, there were significant differences across age groups in short-term memory, but not in long-term memory.
WHO has released a rigorously substantiated technical package, delineating the steps countries should follow to decrease dietary sodium consumption among their populations.14 However, only a limited number of nations have formally enacted mandatory sodium reduction targets. A recent review has further indicated slow progress in achieving the WHO's goal of a 30% global reduction in sodium intake.15 Alarmingly, the majority of countries continue to report average sodium consumption levels that significantly surpass the WHO's recommended limit of less than 2000 mg per day for adults.14 It is important to note that one significant challenge in creating individual-level recommendations for salt intake is the absence of a reliable method to accurately measure individual salt intake, and the lack of feasible interventions to effectively sustain low salt intake in free-living individuals with ethnical concerns.16 Crucially, this variability stems not only from fluctuations in dietary salt intake but also from large intraindividual variability in urinary excretion, likely due to physiological factors regulating sodium excretion, even in studies where salt intake is tightly controlled.17
Moreover, it is difficult to regulate experimentally in humans due to ethical considerations, highlighting the urgent need for research testing the effects of salt intake under rigorously controlled conditions. In this context, animal models provide an advantageous environment for the exploration of the effects of dietary salt intake, with strict control over other aspects of the diet. However, when designing animal studies, several aspects necessitate caution. The first aspect to consider is the concentration of salt exposure in rodent studies. Previous studies administered to animals an exceptionally high-salt diet containing 8 to 20 times more salt compared to what control animals consumed. Conversely, the typical salt intake in humans ordinarily surpasses the WHO's recommended limit of 5 grams per day by approximately double.3,6–8 Thus, it is advisable for rodent studies to utilize lower salt concentrations to reflect more accurately the conditions for humans. Furthermore, the impact of dietary salt on metabolism, BP (particularly BPV), and cognitive function across different age groups, remains poorly understood. In our experimental conditions, the dietary salt and other nutrients for the rats were strictly controlled and could be accurately measured. In addition, our study utilized salt concentrations to examine the age differences in its impact on metabolism, BP levels and variability, and cognitive functions in a manner that parallels human daily salt consumption.
It is also noteworthy to mention that salt consumption in humans has been correlated with overall caloric intake. Increased caloric intake may, in turn, be associated with higher consumption of saturated fats and refined carbohydrates—substances implicated in cognitive decline.10 Most rodent studies reported no effects of salt on weight gain,3,8,18,19 with a couple of exceptions noting increased20 and decreased weight6 in high-salt groups compared to controls. For instance, Lanaspa and colleagues20 found that young mice on a 1% NaCl solution (containing sweetener sucralose) for 30 weeks exhibited increased body weight, fat mass, and liver triglycerides. In addition, a large-scale prospective study with a follow-up duration of 52 months found that a significant association between high salt consumption (average 8.72 ± 1.93 g day−1) and the onset of metabolic syndrome.21
In our investigation, we noted a universal escalation in body weight among young adult rats over the dietary intervention period. However, the cohort fed a high-salt diet demonstrated the most minimal weight gain. In contrast, aged rats subjected to the same high-salt regimen experienced a decline in body weight. Nevertheless, the final body weights among all experimental groups exhibited no significant variances. Furthermore, our study found age-dependent responses to high-salt intake with respect to metabolic effects. Specifically, blood glucose levels decreased significantly in aged rats following a high-salt diet but remained relatively stable in young adult rats. Additionally, all young adult rats demonstrated resilience to the impact of varying salt levels in their diets, experiencing no adverse effects on survival. Conversely, aged rats responded differently to the dietary interventions. Specifically, aged rats that were given a low-salt diet exhibited the most favorable survival outcomes. These findings imply that high salt intake may exert broader systemic impacts on aged rats, affecting their metabolism, and their likelihood of survival.
Less disputed is the correlation between elevated potassium concentrations with the lower rates of stroke, cardiovascular diseases, and overall mortality.9 While some propose that sodium to potassium ratio is a better predictor of BP thresholds than sodium or potassium alone,22 other research has found no such association, particularly in adolescent populations.23 For example, within the context of the Healthy Brain substudy, neither a nutrient nor their ratio was found to be significantly associated with indices of micro- or macro-structural brain MRI in the participants (74 ± 3 years old) with a mean sodium intake of 2677 ± 1060 mg per day.24 In our investigation, we collected blood samples at the endpoint and employed an iSTAT blood-gas analyzer to measure blood sodium, potassium, and their ratios. Our research showed that blood sodium levels were significantly impacted by age. In young adult rats, a high-salt diet did not lead to a notable rise in blood sodium levels. However, in aged rats, even a normal salt diet was sufficient to significantly elevate blood sodium. This implies that sodium regulation mechanisms may be compromised in aged rats. Furthermore, in our study, the absence of significant variations in blood potassium levels or sodium-to-potassium ratios across different age groups suggests that potassium regulation might be less sensitive to dietary salt.
Moreover, a strong correlation has been observed between elevated levels of BP and excessive intake of dietary salt, as demonstrated in human studies. For example, a dose–response analysis, which included 85 trials with sodium intake levels ranging from 0.4 to 7.6 g per day and a follow-up duration between 4 weeks to 36 months, revealed a nearly linear relationship between sodium consumption and reductions in both SBP and DBP.25 Notably, although this effect was consistent regardless of initial BP levels, it was more pronounced among participants who had elevated BP levels to begin with.25
In contrast, other studies indicate that while high salt intake may lead to a short-term increase in BP levels, it does not significantly impact long-term BP levels. In a large-scale study involving 355
134 participants at baseline and 33
915 after a 9-year follow-up, an increase of 100 mmol L−1 in average urinary sodium was linked to a 3.09 mm Hg increase in SBP at baseline, but showed no correlation after 9 years.26 Similarly, there was no observed relationship between urinary sodium levels and the risk of cardiovascular disease over the same follow-up period.26 In a comprehensive meta-analysis encompassing 36 clinical trials, a discernable correlation was identified between reduced sodium intake and BP levels. Specifically, the reduction in BP was more pronounced in hypertensive participants as compared to those without hypertension.27 Subsequent subgroup analyses, sorted by the duration of clinical trial follow-up, revealed nuanced findings. For trials with a follow-up period of less than 3 months, the between-group difference in BP was −4.07/–1.67 mmHg (participants with hypertension vs. without hypertension). For trials lasting 3 to 6 months, the difference was −1.91/1.33 mmHg. Finally, for trials with a follow-up period exceeding 6 months, the difference reduced to −0.88/0.45 mmHg.27 These results suggest the existence of potential long-term compensatory mechanisms that may negate the initial benefits in BP reduction achieved through lowering sodium intake.16
In accordance with existing research, our study revealed that although a high-salt diet initially elevated the BP in young adult rats, this elevation was not statistically significant and plateaued over an extended period. Interestingly, aged rats did not exhibit a significant BP increase with a high-salt diet. These results suggest that the hypertensive effect of a high-salt diet may be age dependent or possibly mitigated by other factors in the long term. Furthermore, a low-salt diet led to a statistically significant reduction in BP across different age groups of rats. This aligns with prior research that also demonstrated a reduction in BP levels due to decreased salt intake, both in control diets and in specific dietary approaches like the dietary approaches to stop hypertension (DASH) diet.25 Additionally, upon aggregating the data for both age groups, aged rats had higher baseline BP levels compared to young adult rats, although only SBP differences reached statistical significance. Furthermore, the BP-lowering effect was particularly notable among young adult rats when compared to aged rats from the eighth week onward. This observation could suggest age-dependent physiological adaptations or differing metabolic rates affecting salt retention and its long-term impact on BP. PP, which tends to increase with age and can be an indicator of arterial stiffness,28 showed no statistically significant differences between the groups of rats. This suggests that the salt diet variations did not have a discernible impact on the PP across the groups. This absence of change might indicate that dietary salt levels do not significantly influence the components that determine PP, such as stroke volume and arterial elasticity.29 Moreover, aged rats exhibited lower HR compared to young adult rats at baseline. However, young adult rats showed a more noticeable elevation in HR at specific time points—after two weeks on a control salt diet and at the twelfth week when compared to aged rats on a high-salt diet. This could imply that younger subjects are more responsive to changes in dietary salt. While these findings support general health guidelines advocating for reduced salt intake to manage BP, they add nuance by highlighting the variable effectiveness of this intervention across age groups. This could be an important consideration for developing age-specific dietary guidelines or treatments for hypertension. However, it is worth noting that the efficacy of low-salt diets in broader populations has been subject to debate, with some limitations pointed out in previous studies.30
In addition, elevated BPV, both short term (24-hour) and long term (visit-to-visit), are known to be significant determinants of hypertensive organ damage and cardiovascular outcomes, contributing to individual risk stratification.31,32 Nonetheless, limited studies have evaluated the impact of dietary salt intake on dynamic BP fluctuations under conditions of daily living. Studies showed mixed results with a general trend towards altered BPV after a high salt diet.31 The main reasons for these conflicting results are due to differences in study designs, subject characteristics, observation duration, and salt intake amount.31 For instance, Simmonds et al. reported that high salt intake (4% NaCl, 14 to 17 days) increased nocturnal BPV in normotensive young male rats without affecting diurnal BP.33 In a study involving 235 patients with essential hypertension, measurements of 24-hour ambulatory BP indicated that nocturnal SBP variability (SD and CV) and DBP variability (SD) were significantly elevated in the group with high urine sodium excretion.34 However, there was no significant difference for diurnal BPV.34 Another study conducted on aged hypertensive patients, which showed increased 24-hour BPV at higher dietary salt intakes.35 In contrast, Migdal et al. found that ten days of high salt intake (18 g day−1) did not increase 24-hour ambulatory BPV in twenty-one healthy young adults.36 Moreover, a separate study observed BP readings taken every six months for three years from 1820 participants (mean age of 43.0 ± 6.1 years) with high-normal DBP, suggesting that sodium reduction may not effectively lower BPV.37 Additional data were sourced from two studies: the cross-sectional Maastricht Study (with 2652 participants, average age of 60) and a randomized crossover trial (with 40 participants, average age of 49).38 The results revealed that urinary sodium excretion and salt intake did not independently correlate with 24-hour BPV.
Furthermore, research has established a connection between salt intake and HR variability. For instance, a study involving healthy, normotensive, middle-aged women revealed that a high-salt diet led to an increase in SBP, a decrease in HR, and an elevation in high-frequency HR variability, indicating enhanced cardiac vagal tone.39 In contrast, a study by Linder et al. (2023)40 examined 20 participants (eight females; average age 24, with a BP reading of 110/64 mmHg) who consumed either salt or placebo capsules over a period of 10 days, separated by at least two weeks. No significant effects on HRV were observed due to high-sodium consumption. However, an increase in the circulating inflammatory biomarker was noted, which could potentially impact BP in young adults.40 Moreover, another investigation found that a low-sodium diet did not significantly alter HR variability in normotensive men and women with asthma.41
Our data show that both high and low salt diets increased BPV in young adult rats when compared to a control diet. This suggests that salt levels, both high and low, may contribute to BP fluctuations in younger rats, though the exact mechanisms are unclear. Interestingly, a low-salt diet reduced BPV in aged rats. This implies that dietary salt restriction may benefit older rats in maintaining stable BP. Moreover, significant differences were found in the CV of SBP variability and the SD and CV of MBP variability between young adult rats on a low-salt diet and aged rats on the same diet. The results suggest that salt restriction could potentially be beneficial for reducing BPV in aged rats but not in young adult rats, indicating age-dependent responses to salt restriction. Furthermore, no significant differences in PP or HR variability were observed across groups, suggesting that these metrics were not influenced by salt intake in this study. Although no citations were found regarding the age-specific effects of salt on BP and HR variability in rats, our data do hint at age-dependent mechanisms by which salt affects BPV, offering a foundation for future studies.
The impact of dietary salt intake on cognitive functions in humans was largely derived from longitudinal studies in aging populations. Results from these investigations, however, are varied. For instance, a three-year prospective study of 1262 adults aged between 67 and 84 revealed that reduced salt consumption at baseline was linked with enhanced cognitive function, as measured by the mini-mental state examination (MMSE).42 It is noteworthy, though, that this relationship was only present in participants with lower levels of physical activity at baseline, and there was no correlation between salt intake and cognitive function in more active individuals. Conversely, two prospective studies involving older adults found no link between dietary salt intake and cognitive decline. These studies included a study by Nowak et al.24 involving 1194 adults with a mean age of 74 ± 3, and a 6.9-year follow-up, and another study by Haring et al.43 involving 6426 women aged between 65 and 79, with a median follow-up of 9.1 years. The latter study notably found that salt intake did not affect the risk for cognitive impairment in hypertensive women or with antihypertensive medication, implying a possible indirect effect of salt on cognition via vascular function changes.43
Cross-sectional studies have also evaluated the relationship between salt intake and cognitive function. A study by Rush and colleagues reported that a low salt diet was linked with poorer performance on the MMSE and impaired executive functioning, as assessed by the Trails-B task, in a sample of 925 adults aged 50–96.44 This effect was more pronounced in the oldest participants, aged over 80.44 In contrast, two smaller studies that estimated salt intake using biomarkers instead of food frequency questionnaires found associations between high salt intake and cognitive impairment as measured by the MMSE. One study found that 24-hour urinary sodium excretion was negatively associated with MMSE scores in 119 adults with a mean age of 54.2 ± 16.1,45 and the other study reported a negative correlation between serum sodium levels and MMSE scores in 82 older adults with a mean age of 87 ± 6.46 On the other hand, in another larger-scale study involving 989 participants aged 50 years and older, no significant correlation was observed between sodium consumption and cognitive impairment.47 These studies reveal a complex relationship between dietary salt intake and cognitive function, with many variables at play.
While the findings from human studies have been varied, the majority of animal studies have consistently shown negative cognitive impacts from a high salt diet. For instance, Faraco et al. found that twelve weeks of a high salt diet (4% salt diet) induced deficits in both nonspatial and spatial memory, which were reversible by normalization of salt intake in young mice.3 A parallel study revealed deteriorations in place recognition memory and long-term contextual fear memory in young male mice following seven weeks on a high salt diet (8% NaCl compared to 0.4% in the control diet).6 Similar results were found in young male rats after consuming a high salt diet (8% NaCl compared to 0.26% in the control diet) for nine weeks, with detectable impairments in spatial memory and in a contextual fear condition.7 Furthermore, Chugh et al. observed age differences in the impact of a high salt diet (8% NaCl compared to 0.4% control diet) for four weeks on memory function, with impairment in aged rats and no effect in younger ones.8 Nonetheless, it is important to underscore that these animal studies implemented extremely high levels of salt intake, ranging from 8 to 16 times higher than a normal salt diet, limiting the translatability of these findings to clinical settings. Moreover, one study found that a restriction in salt intake notably impairs the ability of Dahl S (salt-sensitive) rats in the social transmission of food preference and social recognition memory, however, it does not affect their spatial learning capabilities.48 Conversely, for Dahl R (salt-resistant) rats, the salt restriction did not interfere with their social learning abilities but enhanced their navigation performance significantly.48 Furthermore, sodium restriction did not have any discernible influence on object recognition memory in either subgroup of rats.48
The findings of our investigation are consistent with extensive research, illustrating that the intake of dietary salt can yield intricate impacts on cognition. Within this specific study, a decrement in short-term memory was manifested at the second week, subsequently reverting to the baseline level by the twelfth week in young adult rats subjected to control diets. It is noteworthy that variations in salt content, whether high or low, did not exhibit any discernible influence on both short-term and long-term memory in young adult rats. In contrast, aged rats demonstrated a decrease in short-term memory yet exhibited enhancement in long-term memory when subjected to control or low-salt diets. However, the high-salt diet had no discernible impact on short-term memory but did precipitate impairment in long-term memory in aged rats. A synthesis of data from both young adult and aged rats revealed pronounced disparities in short-term memory between young adult rats on a high-salt diet and aged rats on a control diet, yet no notable variances were identified in long-term memory. The mechanisms elucidating the age-related disparities in the effects of diverse salt intake levels on both memory types are unclear and necessitate further exploration.
To the best of our understanding, our research is pioneering in exploring the age differences in how varying levels of dietary salt affect metabolism, BP and its variability, and cognitive function. Our study adhered to rigorous methodological standards, featuring randomized assignment, double-blind procedures, and video-recorded behavioral assessments. Nevertheless, the findings of our study should be interpreted within the scope of its limitations. A notable limitation is the absence of insights into the molecular mechanisms that may explain the observed age differences in the effects of salt intake on the relationship between metabolism, BP, and cognitive function. The other limitation is that we only used male rats instead of both sexes in this study. Future research should aim to examine the prospective mechanisms and sex differences.
5 Conclusions
This study marks a significant advancement in our understanding of the age differences in the complex interplay between dietary salt intake, metabolism, BP, and cognitive function. Prior research only probed individual components of this dynamic system, leaving a comprehensive understanding elusive. Our results suggest the necessity of age-specific guidelines and underline the importance of employing clinically relevant salt concentrations in future studies.
Author contributions
F. S.: conceptualization, methodology, investigation, data curation, formal analysis, project administration, supervision, writing – original draft, writing – review and editing, and funding acquisition. J. Y. Z., Q. J., L. P. Z., C. J., Q. X. W., J. T. X. and M. J. Y.: methodology, investigation, data curation, and formal analysis.
Conflicts of interest
There are no conflicts of interest to declare.
Acknowledgements
The current work was supported by the Start-up Funding of Hangzhou Normal University, grant number 4255C50221204123. We extend our sincere gratitude to the entire staff of the Laboratory Animal Facility at Hangzhou Normal University for their invaluable support and assistance.
References
- D. Mohan, K. H. Yap, D. Reidpath, Y. C. Soh, A. McGrattan, B. C. M. Stephan, L. Robinson, N. Chaiyakunapruk and M. Siervo, Link Between Dietary Sodium Intake, Cognitive Function, and Dementia Risk in Middle-Aged and Older Adults: A Systematic Review, J. Alzheimer's Dis., 2020, 76, 1347–1373 Search PubMed.
- A. Grillo, L. Salvi, P. Coruzzi, P. Salvi and G. Parati, Sodium Intake and Hypertension, Nutrients, 2019, 11, 1970 CrossRef CAS PubMed.
- G. Faraco, D. Brea, L. Garcia-Bonilla, G. Wang, G. Racchumi, H. Chang, I. Buendia, M. M. Santisteban, S. G. Segarra, K. Koizumi, Y. Sugiyama, M. Murphy, H. Voss, J. Anrather and C. Iadecola, Dietary salt promotes neurovascular and cognitive dysfunction through a gut-initiated TH17 response, Nat. Neurosci., 2018, 21, 240–249 CrossRef CAS PubMed.
- E. Pinto, Blood pressure and ageing, Postgrad. Med. J., 2007, 83, 109–114 CrossRef PubMed.
- F. C. Luft, J. Z. Miller, C. E. Grim, N. S. Fineberg, J. C. Christian, S. A. Daugherty and M. H. Weinberger, Salt sensitivity and resistance of blood pressure. Age and race as factors in physiological responses, Hypertension, 199, 1 Suppl, I102-8 Search PubMed.
- Q. Ge, Z. Wang, Y. Wu, Q. Huo, Z. Qian, Z. Tian, W. Ren, X. Zhang and J. Han, High salt diet impairs memory-related synaptic plasticity via increased oxidative stress and suppressed synaptic protein expression, Mol. Nutr. Food Res., 2017, 61, 1700134 CrossRef PubMed.
- C.-P. Guo, Z. Wei, F. Huang, M. Qin, X. Li, Y.-M. Wang, Q. Wang, J.-Z. Wang, R. Liu, B. Zhang, H.-L. Li and X.-C. Wang, High salt induced hypertension leads to cognitive defect, Oncotarget, 2017, 8, 95780–95790 CrossRef PubMed.
- G. Chugh, M. Asghar, G. Patki, R. Bohat, F. Jafri, F. Allam, A. T. Dao, C. Mowrey, K. Alkadhi and S. Salim, A High-Salt Diet Further Impairs Age-Associated Declines in Cognitive, Behavioral, and Cardiovascular Functions in Male Fischer Brown Norway Rats, J. Nutr., 2013, 143, 1406–1413 CrossRef CAS PubMed.
- A. Mente, M. O'Donnell, S. Rangarajan, M. McQueen, G. Dagenais, A. Wielgosz, S. Lear, S. T. L. Ah, L. Wei, R. Diaz, A. Avezum, P. Lopez-Jaramillo, F. Lanas, P. Mony, A. Szuba, R. Iqbal, R. Yusuf, N. Mohammadifard, R. Khatib, K. Yusoff, N. Ismail, S. Gulec, A. Rosengren, A. Yusufali, L. Kruger, L. P. Tsolekile, J. Chifamba, A. Dans, K. F. Alhabib, K. Yeates, K. Teo and S. Yusuf, Urinary sodium excretion, blood pressure, cardiovascular disease, and mortality: a community-level prospective epidemiological cohort study, Lancet, 2018, 392, 496–506 CrossRef PubMed.
- M. D. Kendig and M. J. Morris, Reviewing the effects of dietary salt on cognition: mechanisms and future directions, Asia Pac. J. Clin. Nutr., 2019, 28, 6–14 Search PubMed.
- J. E. Toblli, G. DeRosa, G. Cao, P. Piorno and P. Pagano, ACE inhibitor and angiotensin type I receptor antagonist in combination reduce renal damage in obese Zucker rats, Kidney Int., 2004, 65, 2343–2359 CrossRef CAS PubMed.
- R. M. J. Deacon and J. N. P. Rawlins, T-maze alternation in the rodent, Nat. Protoc., 2006, 1, 7–12 CrossRef PubMed.
- R. A. Bevins and J. Besheer, Object recognition in rats and mice: a one-trial non-matching-to-sample learning task to study ‘recognition memory’, Nat. Protoc., 2006, 1, 1306–1311 CrossRef PubMed.
- R. M. McLean, T. N. Widyastuti and H. Eyles, Effectiveness of WHO Sodium Benchmarks to Reduce Morbidity and Mortality: Evidence From Australia, Hypertension, 2023, 80, 550–552 CrossRef CAS PubMed.
- W. B. Santos, J. M. D. Matoso, M. Maltez, T. Gonçalves, M. Casanova, I. F. H. Moreira, R. A. Lourenço, W. D. Monteiro, P. T. V. Farinatti, P. P. Soares, W. Oigman, M. F. T. Neves and M. L. G. Correia, Spectral analyses of systolic blood pressure and heart rate variability and their association with cognitive performance in elderly hypertensive subjects, J. Hum. Hypertens., 2015, 29, 488–494 CAS.
- M. O'Donnell, A. Mente, M. H. Alderman, A. J. B. Brady, R. Diaz, R. Gupta, P. López-Jaramillo, F. C. Luft, T. F. Lüscher, G. Mancia, J. F. E. Mann, D. McCarron, M. McKee, F. H. Messerli, L. L. Moore, J. Narula, S. Oparil, M. Packer, D. Prabhakaran, A. Schutte, K. Sliwa, J. A. Staessen, C. Yancy and S. Yusuf, Salt and cardiovascular disease: insufficient evidence to recommend low sodium intake, Eur. Heart J., 2020, 41, 3363–3373 CrossRef PubMed.
- L. J. Appel and K. Foti, Extreme Variability in Urinary Sodium Excretion: Time to Stop Use of Spot Urines to Predict Clinical Outcomes, Hypertension, 2021, 78, 1637–1639 CrossRef CAS PubMed.
- Y.-Z. Liu, J.-K. Chen, Z.-P. Li, T. Zhao, M. Ni, D.-J. Li, C.-L. Jiang and F.-M. Shen, High-salt diet enhances hippocampal oxidative stress and cognitive impairment in mice, Neurobiol. Learn. Mem., 2014, 114, 10–15 CrossRef CAS PubMed.
- N. Nohata, Y. Sone, T. Hanazawa, M. Fuse, N. Kikkawa, H. Yoshino, T. Chiyomaru, K. Kawakami, H. Enokida, M. Nakagawa, M. Shozu, Y. Okamoto and N. Seki, miR-1 as a tumor suppressive microRNA targeting TAGLN2 in head and neck squamous cell carcinoma, Oncotarget, 2011, 2, 29–42 CrossRef PubMed.
- M. A. Lanaspa, M. Kuwabara, A. Andres-Hernando, N. Li, C. Cicerchi, T. Jensen, D. J. Orlicky, C. A. Roncal-Jimenez, T. Ishimoto, T. Nakagawa, B. Rodriguez-Iturbe, P. S. MacLean and R. J. Johnson, High salt intake causes leptin resistance and obesity in mice by stimulating endogenous fructose production and metabolism, Proc. Natl. Acad. Sci. U. S. A., 2018, 115, 3138–3143 CrossRef CAS PubMed.
- H. Takase, K. Hayashi, F. Kin, S. Nakano, M. Machii, S. Takayama, T. Sugiura and Y. Dohi, Dietary salt intake predicts future development of metabolic syndrome in the general population, Hypertens. Res., 2023, 46, 236–243 CrossRef CAS PubMed.
- A. S. Bhagavathula, S. A. Refaat, B. L. Bentley and J. Rahmani, Association between intake of sodium, potassium, sodium-to-potassium ratio, and blood pressure among US adults, Int. J. Vitam. Nutr. Res., 2023, 93, 392–400 CrossRef PubMed.
- H. L. Cheng, F. L. Garden, M. R. Skilton, C. Johnson, J. Webster, C. A. Grimes, R. Q. Ivers and K. S. Steinbeck, Impact of growth, gonadal hormones, adiposity and the sodium-to-potassium ratio on longitudinal adolescent measures of blood pressure at puberty, J. Hum. Hypertens., 2023, 37, 835–843 CAS.
- K. L. Nowak, L. Fried, A. Jovanovich, J. Ix, K. Yaffe, Z. You and M. Chonchol, Dietary Sodium/Potassium Intake Does Not Affect Cognitive Function or Brain Imaging Indices, Am. J. Nephrol., 2018, 47, 57–65 CrossRef CAS PubMed.
- T. Filippini, M. Malavolti, P. K. Whelton, A. Naska, N. Orsini and M. Vinceti, Blood Pressure Effects of Sodium Reduction, Circulation, 2021, 143, 1542–1567 CrossRef CAS PubMed.
- F. Re, I. Hammami, T. J. Littlejohns, M. Arnold, S. Lewington, R. J. Clarke and J. L. Carter, Effects of Within-Person Variability in Spot Urinary Sodium Measurements on Associations With Blood Pressure and Cardiovascular Disease, Hypertension, 2021, 78, 1628–1636 CrossRef CAS PubMed.
- N. J. Aburto, A. Ziolkovska, L. Hooper, P. Elliott, F. P. Cappuccio and J. J. Meerpohl, Effect of lower sodium intake on health: systematic review and meta-analyses, Br. Med. J., 2013, 346, f1326–f1326 CrossRef PubMed.
- H. Schneider, A. Sarkis, L. Sturm, V. Britz, A. Lechner, A. L. Potzel, L. M. Müller, D. A. Heinrich, H. Künzel, H. F. Nowotny, T. M. Seiter, S. Kunz, M. Bidlingmaier, M. Reincke and C. Adolf, Moderate dietary salt restriction improves blood pressure and mental well–being in patients with primary aldosteronism: The salt CONNtrol trial, J. Intern. Med., 2023, 294, 47–57 CrossRef CAS PubMed.
- F.-F. Wei, Y. Wu, R. Xue, X. Liu, X. He, B. Dong, Z. Zhen, X. Chen, W. Liang, J. Zhao, J. He, Y. Dong, J. A. Staessen and C. Liu, Clinical Significance of Mean and Pulse Pressure in Patients With Heart Failure With Preserved Ejection Fraction, Hypertension, 2022, 79, 241–250 CrossRef CAS PubMed.
- A. Smyth, C. Judge, C. Kerins, S. McDermott, A. Niland, C. Corcoran, R. Dineen, A. Alvarez-Iglesias, A. Nolan, A. Mente, M. D. Griffin, P. O'Shea, M. Canavan, S. Yusuf and M. O'Donnell, Dietary counselling to reduce moderate sodium intake: effects on cardiovascular and renal biomarkers: primary findings of the COSIP and STICK phase II feasibility randomised controlled trials, EClinicalMedicine, 2023, 57, 101856 CrossRef PubMed.
- S. Omboni, Does Dietary Salt Loading Impair Ambulatory Blood Pressure Variability? As Yet an Unresolved Issue, Am. J. Hypertens., 2020, 33, 405–406 CrossRef CAS PubMed.
- S. L. Stevens, S. Wood, C. Koshiaris, K. Law, P. Glasziou, R. J. Stevens and R. J. McManus, Blood pressure variability and cardiovascular disease: systematic review and meta-analysis, Br. Med. J., 2016, 354, i4098 CrossRef PubMed.
- S. S. Simmonds, J. Lay and S. D. Stocker, Dietary salt intake exaggerates sympathetic reflexes and increases blood pressure variability in normotensive rats, Hypertension, 2014, 64, 583–589 CrossRef CAS PubMed.
- C. Wang, T.-B. Liu, L. Mu, M. Wang, Y. Li, S.-Y. Yao, M.-X. Zhao and H. Xue, Association between dietary sodium intake and blood pressure variability in Chinese patients with hypertension, China Med. J., 2020, 133, 1066–1072 CrossRef CAS PubMed.
- N. Ozkayar, F. Dede, I. Ates, F. Akyel, T. Yildirim and B. Altun, The relationship between dietary salt intake and ambulatory blood pressure variability in non-diabetic hypertensive patients, Nefrología, 2016, 36, 694–700 CrossRef PubMed.
- K. U. Migdal, M. C. Babcock, A. T. Robinson, J. C. Watso, M. M. Wenner, S. D. Stocker and W. B. Farquhar, The Impact of High Dietary Sodium Consumption on Blood Pressure Variability in Healthy, Young Adults, Am. J. Hypertens., 2020, 33, 422–429 CrossRef CAS PubMed.
- K. M. Diaz, P. Muntner, E. B. Levitan, M. D. Brown, D. M. Babbitt and D. Shimbo, The effects of weight loss and salt reduction on visit-to-visit blood pressure variability, J. Hypertens., 2014, 32, 840–848 CrossRef CAS PubMed.
- T. L. Zhou, M. T. J. Schütten, A. A. Kroon, R. M. A. Henry, A. J. H. M. Houben, C. J. H. van der Kallen, M. M. J. van Greevenbroek, P. W. de Leeuw and C. D. A. Stehouwer, Urinary Sodium Excretion and Salt Intake Are Not Associated With Blood Pressure Variability in a White General Population, J. Am. Heart Assoc., 2023, 12, e026578 CrossRef CAS PubMed.
- J. D. McNeely, B. G. Windham and D. E. Anderson, Dietary sodium effects on heart rate variability in salt sensitivity of blood pressure, Psychophysiology, 2008, 45, 405–411 CrossRef PubMed.
- B. A. Linder, M. C. Babcock, K. U. Pollin, J. C. Watso and A. T. Robinson, Short-term high-salt consumption does not influence resting or exercising heart rate variability but increases MCP-1 concentration in healthy young adults, Am. J. Physiol.: Regul., Integr. Comp. Physiol., 2023, 324, R666–R676 CrossRef CAS PubMed.
- Z. E. K. Pogson, T. M. McKeever, S. A. Lewis, S. J. Pacey, M. D. Antoniak, J. R. Britton and A. W. Fogarty, Does a low sodium diet modify heart rate variability? A randomised placebo-controlled double-blind trial, Int. J. Cardiol., 2009, 135, 390–393 CrossRef PubMed.
- A. J. Fiocco, B. Shatenstein, G. Ferland, H. Payette, S. Belleville, M.-J. Kergoat, J. A. Morais and C. E. Greenwood, Sodium intake and physical activity impact cognitive maintenance in older adults: the NuAge Study, Neurobiol. Aging, 2012, 33, 829.e21-28 CrossRef PubMed.
- B. Haring, C. Wu, L. H. Coker, A. Seth, L. Snetselaar, J. E. Manson, J. E. Rossouw and S. Wassertheil-Smoller, Hypertension, Dietary Sodium, and Cognitive Decline: Results From the Women's Health Initiative Memory Study, Am. J. Hypertens., 2016, 29, 202–216 CrossRef CAS PubMed.
- T. M. Rush, D. Kritz-Silverstein, G. A. Laughlin, T. T. Fung, E. Barrett-Connor and L. K. McEvoy, Association between Dietary Sodium Intake and Cognitive Function in Older Adults, J. Nutr., Health Aging, 2017, 21, 276–283 CrossRef CAS PubMed.
- B. Afsar, The Relationship Between Cognitive Function, Depressive Behaviour and Sleep Quality with 24 h Urinary Sodium Excretion in Patients with Essential Hypertension, High Blood Pressure Cardiovasc. Prev., 2013, 20, 19–24 CrossRef CAS PubMed.
- M. Rondanelli, S. B. Solerte and E. Ferrari, Electrolytes and cognitive function in the elderly: relationship between serum sodium and chloride concentrations and psychometric test scores, Panminerva Med., 1998, 40, 191–195 CAS.
- A. M. McGrattan, B. C. M. Stephan, O. M. Shannon, M. Mazidi, M. Gilchrist, M. Smallwood, P. Winyard, N. McMahon, L. C. Blekkenhorst, D. Mohan, S. Bandinelli, L. Robinson, L. Ferrucci and M. Siervo, Independent and interactive associations of dietary nitrate and salt intake with blood pressure and cognitive function: a cross-sectional analysis in the InCHIANTI study, Int. J. Food Sci. Nutr., 2022, 73, 491–502 CrossRef CAS PubMed.
- N. Ruiz-Opazo, L. V. Lopez and J. Tonkiss, Modulation of Learning and Memory in Dahl Rats by Dietary Salt Restriction, Hypertension, 2004, 43, 797–802 CrossRef CAS PubMed.
Footnote |
† These authors contributed equally to this work. |
|
This journal is © The Royal Society of Chemistry 2024 |