DOI:
10.1039/D3FO03259K
(Paper)
Food Funct., 2024,
15, 310-325
Ligilactobacillus acidipiscis YJ5 modulates the gut microbiota and produces beneficial metabolites to relieve constipation by enhancing the mucosal barrier†
Received
10th August 2023
, Accepted 21st November 2023
First published on 29th November 2023
Abstract
Constipation is a prevalent gastrointestinal (GI) problem affecting a large number of individuals. This study aimed to investigate peristalsis-promoting potential characteristics of Ligilactobacillus acidipiscis YJ5 and the underlying molecular mechanism. The study demonstrated the relieving effect of L. acidipiscis YJ5 on constipation in both zebrafish and mouse models. L. acidipiscis YJ5 intervention significantly increased intestinal peristalsis by reducing the peak time and increasing the fluorescence disappearance rate in the zebrafish model. In the mouse model, the symptoms of constipation relief induced by L. acidipiscis YJ5 included a shortened first black stool time, an increased number of defecation particles, an accelerated propulsion rate of the small intestine, and an increase in fecal water content. L. acidipiscis YJ5 was found to reduce the expression of colonic aquaporins to normalize the colonic water transport system of constipated mice. Additionally, L. acidipiscis YJ5 reversed loperamide-induced morphological damage in the ileum and colon and increased the colonic mucosal barrier. The results of the 16S rRNA gene analysis indicated that L. acidipiscis YJ5 could reverse the structure of gut microbiota to a near-normal group, including levels of β-diversity, phylum, family, and genus. Furthermore, the fermentation supernatant of L. acidipiscis YJ5 was shown to relieve constipation, and metabolomics analysis revealed that these positive effects were related to its metabolites like malic acid and heliangin.
1. Introduction
Constipation is a widespread gastrointestinal (GI) issue that affects up to 27% of individuals in Western countries.1 It not only has a significant impact on the daily lives of those who suffer from it, but also contributes to elevated psychological stress for these patients.2 However, finding a safe and effective treatment for constipation remains challenging, as existing remedies have been associated with significant side effects such as uncontrollable diarrhea and reliance on medication.3,4 Therefore, it is crucial to develop safe and effective strategies to prevent constipation.
Microecological treatment is emerging as an innovative approach to combat constipation. Extensive research has demonstrated the remarkable efficacy of probiotics in alleviating constipation.5Bifidobacterium and Lactobacillus are the most studied probiotics in the relief of constipation. The primary Lactobacillus strains studied were L. casei, L. plantarum, L. salivarius, L. acidophilus, and L. bulgaricus.6–8 Different strains relieve constipation through different mechanisms. For example, studies have revealed that the Bifidobacterium animalis ssp. lactis BB12 effectively alleviates constipation in mice by modulating the gut microbiota and the stem cell factor (SCF)/c-Kit pathway.9 Similarly, Bifidobacterium longum has been found to relieve constipation in mice by regulating the intestinal barrier.10 Moreover, even strains within the same species display different efficacies against constipation. Research conducted by Linlin et al.11 revealed that Bifidobacteria exhibits distinctive constipation relief effects in BALB/c mice, demonstrating species-specific characteristics. Therefore, it is essential to accurately identify a strain of probiotics that displays a relatively better anti-constipation capacity.
In our previous study, we isolated a novel strain of Ligilactobacillus acidipiscis, named YJ5, from traditional Chinese fermented fruits and vegetables in our laboratory. Ligilactobacillus acidipiscis can be obtained from fermented foods such as traditional Greek Kopanisti cheese, fermented cummingcordia, fermented fish, and traditionally fermented vegetables, as well as from animal feces.12 Although there are many studies on the isolation and identification of Ligilactobacillus acidipiscis, few have investigated its bioactive properties, and most of them have mainly focused on its regulation of immunity.13,14
This study investigates the functional role of L. acidipiscis YJ5 in relieving constipation in zebrafish and mouse models. It explores the mechanisms of its protective effect against constipation development and reveals that L. acidipiscis YJ5 achieves this by producing anti-constipation metabolites. To the best of the author's knowledge, this study is the first to report the relief of constipation by Ligilactobacillus acidipiscis, providing valuable guidance for the treatment of constipation.
2. Materials and methods
2.1. Materials
Loperamide (Lop) is an antidiarrheal medication that induces difficulties in defecation by binding to μ-opioid receptors in the intestines, leading to an increased GI transit time, inhibition of intestinal smooth muscle contractions, and slowed peristalsis.15,16 Here, Lop was chosen as the constipation-inducing agent to create the constipation model. It was obtained from Xi'an Janssen Pharmaceutical Co., Ltd (Xi'an, China). Domperidone (Dom), by blocking dopamine receptors in the GI tract and enhancing acetylcholine release, could improve intestinal motility and alleviate constipation symptoms.17 It was used as a positive control and procured from Shanghai Macklin Biochemical Co., Ltd, respectively. Nile red, skim milk, lactose, and Arabic gum were obtained from Fonterra Co., Ltd (Auckland, New Zealand), Zhejiang YiNuo Biological Co., Ltd (Jinghua, China), and Sinopharm Chemical Reagent Co., Ltd. To prepare the activated carbon solution, a mixture of 10 g of Arabic gum and 80 mL of water was heated until it turned transparent. Following this, 5 g of carbon was incorporated, and the procedure was repeated thrice. The resulting solution was then diluted to 100 mL and stored at 4 °C for future utilization.
2.2. Reagents
The following materials were obtained from the specified suppliers: ELISA kits targeting tumor necrosis factor-α (TNF-α) & interleukin-10 (IL-10) from Wuhan Jiyinmei Biological Technology Co., Ltd (Wuhan, China); Trizol reagent, DEPC-treated water, and a HiScript II One Step RT-PCR Kit from Tiangen Biotech Co., Ltd; Rabbit anti-MUC2 and anti-GAPDH antibodies, QuickBlock™ blocking buffer, Alexa Fluor 350-labeled goat anti-rabbit IgG antibodies, immunostaining fix solution, QuickBlock™ immunostaining blocking buffer, immunostaining fix solution, and ECL Western blotting substrate from Beyotime Life Science Co., Ltd (Shanghai, China); and RIPA buffer from Solarbio Life Science Co., Ltd (Beijing, China).
2.3. Bacterial strains
L. acidipiscis YJ5 was sourced from the Food Probiotics Strain Collections Center, Zhejiang University (Hangzhou, China) and cultured in De Man–Rogosa–Sharpe (MRS) medium. Escherichia coli ATCC 25922, Staphylococcus aureus CMCC 26003, Salmonella typhimurium CMCC 50115, and Listeria monocytogenes ATCC 15313 were acquired from Zhejiang University's microbiology laboratory broth or agar. Lactocaseibacillus rhamnosus GG ATCC 53103 (LGG) from Valio Ltd (Helsinki, Finland) served as the reference strain.
2.4. Probiotic potential assessment
It includes artificial GI tolerance-, autoaggregation-, and adhesion assays along with coaggregation assays of pathogens with probiotic strains. The disk diffusion assay against Gram-positive and Gram-negative pathogens was performed. These tests were conducted following the protocols of our previous study.18
2.5. Preparation of bacterial samples
2.5.1. Preparation of live bacteria.
Live bacterial samples were prepared following the protocols of our previous study.18 Bacterial strains were cultured in MRS broth for 18 hours at 37 °C, and then washed three times with sterilized phosphate-buffered saline (PBS, pH = 7.4) to collect their cells. Free cells were harvested through centrifugation, and the resulting strain cells were adjusted to a concentration of ∼109 CFU mL−1 using PBS.
2.5.2. Preparation of the cell free supernatant (CFS).
The CFS was prepared following the protocols of our previous study with minor modifications.18 Live bacteria in the growing stage were diluted with MRS broth to ∼107 CFU mL−1 and incubated for 18 h at 37 °C. The CFS was obtained by filtering the supernatant using a 0.22 μm filter membrane after centrifugation at 8000g for 15 minutes at 4 °C. In this study, 1%YJ5CFS, 0.5%YJ5CFS and 0.1%YJ5CFS described the addition of 1%, 0.5% and 0.1% CFS of YJ5 into zebrafish aquaculture water, respectively.
2.5.3. Preparation of cell free extract (CFE).
L. acidipiscis YJ5 was inoculated in MRS liquid culture (2% inoculation rate) and cultured at 37 °C for 18 hours. The cultured cells were then refrigerated at 8000g and 4 °C for 15 minutes, washed three times with PBS (pH = 7.4), and suspended in PBS (pH = 7.4). The adjusted bacterial concentration was approximately 5.0 × 1010 CFU mL−1. Ultrasound was used to break up the cells (50% power, 8 s pulse for 20 minutes on an ice bath). After centrifugation at 8000g and 4 °C for 15 minutes, the supernatant was properly filtered with a 0.22 μm membrane and kept at −80 °C, referred to as cell-free extract (CFE).
2.6. Zebrafish experiment
2.6.1. Culture of zebrafish.
Four-month-old AB zebrafish were obtained from the Zebrafish Platform at Zhejiang University and raised using standard protocols (Approval number ZJU 20220189). Culture methods were performed following our previous study.18 The zebrafish were grown in a centralized breeding and recirculating system with 14-hour light and 10-hour dark cycles at 28 ± 0.5 °C and pH 7.0–7.5. Embryos were obtained by mating with adult AB zebrafish, and on the 3rd day after fertilization, the juvenile fish were cultured at a rate of 20 tails per well.
2.6.2. Mortality determination.
Zebrafish at five days post-fertilization (dpf) were selected and placed in six-well plates, with 20 tails per well. They were exposed to varying concentrations of the test samples (0.5%, 1%, 2%, 10% CFS/MRS, and 5%, 10%, 20%, 50% CFE/PBS) for a duration of 24 hours. The experiment was performed in triplicate to ensure reliability and reproducibility.
2.6.3. Observation of the intestinal peristalsis peak in the constipated zebrafish model.
Five dpf zebrafish were randomly divided into different groups with twenty larvae per group. The control group (CON) was cultured in normal E3 medium. The Lop-induced model group (LOP) was cultured in normal E3 medium (1% NaCl, 0.17 mM KCl, 0.33 mM CaCl2, 0.33 mM MgSO4 and 0.00003% methylene blue, pH 7.00) with Lop (10 μg mL−1). The DOM group received 50 μg mL−1 Dom along with Lop treatment. LGG, YJ5(8), YJ5(7), and YJ5(6) groups were treated with LGG at 108 CFU mL−1, and L. acidipiscis YJ5 at 108 CFU mL−1, 107 CFU mL−1, and 106 CFU mL−1, respectively, along with Lop treatment. For the CFS/CFE intervention groups, different concentrations of CFS/CFE were directly added to E3 water with 10 g mL−1 Lop. Following a 24-hour exposure to the test samples, zebrafish intestines were subjected to examination using a Nikon SMZ745T microscope. To determine the first peristaltic peak, a stereomicroscope (Nikon SMZ745T) was utilized, and it was observed between the fourth and fifth sarcomeres of zebrafish, following the method described by de Alvarenga et al.19 The entire experiment was conducted in triplicate to ensure the reliability and consistency of the results.
2.6.4. Assessing intestinal motility by the Nile red staining method.
The Nile red staining method was conducted following Zhou et al.20 with some modifications. The experimental process is shown in Fig. 1L. In brief, 20 tails of 5 dpf zebrafish were placed in each well and treated with 10 μg L−1 Nile red dye for 16 hours. The gut region of interest (ROI) of the dyed zebrafish was imaged using stereoscopic fluorescence microscopy (Nikon SMZ18) at 80× magnification, fluorescence gain, and exposure time. The zebrafish were then washed three times and treated with Dom (50 μg mL−1), LGG, L. acidipiscis YJ5, CFS, and CFE, respectively. The control group (CON) did not receive any samples. After 24 hours of treatment, the ROI of the zebrafish was imaged again using stereoscopic fluorescence microscopy (Nikon SMZ18) with the same parameters. The total fluorescence intensity (FI) of the GI tract was quantitatively analyzed using NIS Elements D advanced software (Nikon). The promotion effect of the samples on intestinal peristalsis of zebrafish was determined by the reduction rate of the fluorescence intensity, which is calculated as follows: |  | (1) |
here, FI(before) and FI(after) represent the fluorescence intensity before and after treatment, respectively.
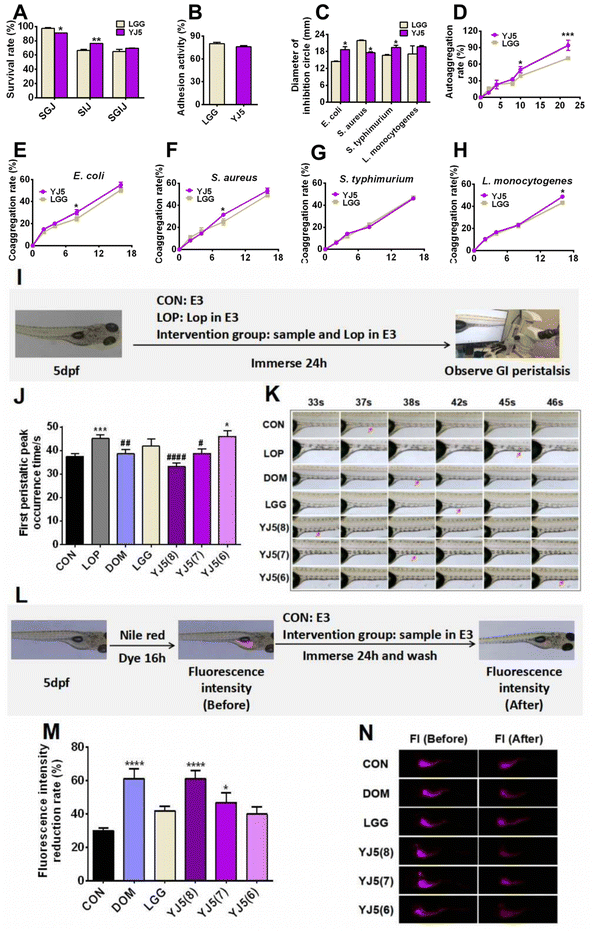 |
| Fig. 1
In vitro probiotic properties and intestinal peristalsis-promoting effects of L. acidipiscis YJ5 on zebrafish. (A) Tolerance of strains to SGJ, SIJ and SGIJ, (B) adhesion activity to HT-29, (C) antibacterial activity, (D) autoaggregation, and (E–H) coaggregation rate strains against four pathogens (E) E. coli, (F) S. aureus, (G) S. typhimurium, and (H) L. monocytogenes. (I) Experimental scheme of the constipation zebrafish model induced by Lop, (J) first peristaltic peak occurrence time, and (K) inverted microscopy observation of the first peristalsis peak of the zebrafish intestine. Note: The red arrow in the figure indicates the site of the intestinal peristalsis peak at the same observation site in different groups of zebrafish intestines. (L) Experimental scheme of the zebrafish model based on the Nile red staining method, (M) fluorescence intensity reduction rate, and (N) inverted fluorescence microscopy observation of the zebrafish intestinal fluorescence intensity. Data in (A) to (H) are expressed as mean ± SD, n = 3 (* P < 0.05, ** P < 0.01, and *** P < 0.001 vs. LGG). Data in (J) and (M) are expressed as mean ± SD, n = 60 (* P < 0.05, ** P < 0.01, *** P < 0.001, and **** P < 0.0001 vs. CON; #P < 0.05, ##P < 0.01, and ####P < 0.0001 vs. LOP). | |
2.7. Experimental design
The research protocol involving experimental animals at Zhejiang Chinese Medical University was reviewed and approved by the Animal Ethical and Welfare Committee for Experimental Animals (IACUC-20220425-28). The mice experiments strictly adhered to the institutional guidelines set forth by the Animal Ethical and Welfare Committee of Zhejiang Chinese Medical University, ensuring the ethical and responsible conduct of the research. All mice were obtained from Shanghai SLAC Laboratory Animal Co., Ltd. Thirty-six male BALB/c mice (20 g ± 2 g) were randomly divided into three groups: CON, LOP, and YJ5 (12 mice per group). The mice were housed four per cage under standard conditions (60% humidity, 12-hour day/night cycle, and 25 °C temperature). After a 7-day adaptation period, the LOP and YJ5 groups received Lop (10 mg per kg bw) with the YJ5 treated group additionally receiving the YJ5 solution (5 × 108 CFU mL−1 in saline). The CON group received saline treatments. Throughout the study, we closely monitored body weight gain and food consumption. On the 13th day, fecal samples were collected from the subjects and preserved at −80 °C for subsequent analysis.
2.8. Determination of fecal excretion function
2.8.1. Defecation test.
On the 13th day, all groups of mice underwent a 16-hour fasting period with access to water. The next morning, the LOP and YJ5 groups were administered Lop (10 mg per kg bw), while the CON group received saline. After 30 minutes, the CON and LOP groups received an activated carbon suspension (10 mL kg−1), and the YJ5 group received L. acidipiscis YJ5-containing activated carbon suspension. After the specified treatment period, each mouse was placed in an individual cage with unrestricted access to food and water. Following a 5-hour interval, fecal samples were collected, and the number of fecal particles was documented. The wet feces weight was measured, and subsequently, the samples were dried to ascertain the dry feces weight. The fecal water content was then calculated using the following formula: |  | (2) |
2.8.2. GI transit test.
The GI transit test was conducted following the guidelines of our previous study with minor modifications.18 After a period of 16-days treatment, all groups of mice were fasted for 16 hours, however, they had access to water. On the 17th day, the CON group received sterile saline, while the LOP and YJ5 groups were given Lop (7.5 mg kg−1). After 30 minutes, the CON and LOP groups received an activated carbon suspension (10 mL kg−1), while the YJ5 group received the activated carbon suspension with L. acidipiscis YJ5. After half an hour, the mice were euthanized, and the complete GI tract was removed. The small intestine transit rate was measured using the following formula: | 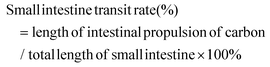 | (3) |
2.9. Determination of the biochemical index
Pre-cooled saline was used to gently flush the colon tissue to remove the remaining impurities. The fat around the tissue was removed with surgical scissors and the appropriate quality of mouse colon tissue was extracted. The pre-cooled normal saline was added according to the ratio of tissue mass to normal saline volume = 1
:
9 (w/v). The tissue grinder was used to grind for 30 s at a frequency of 70 Hz s−1, and the tissue was shaken 4 times until there were no visible large particles in the tissue. After homogenization was complete, the supernatant was centrifuged at 12
000g and 4 °C for 15 min. The supernatant was divided and determined according to the manufacturer's instructions for the IL-10 and TNF-α ELISA kit. Finally, the standard curve is obtained by the external standard method, and the concentration of the substance contained in the sample is calculated according to the absorbance.
2.10. Histopathological examination
Colon and ileum tissues were fixed overnight in a 4% polyformaldehyde solution, embedded in paraffin, and sectioned into slices of 5 μm thickness. Hematoxylin and eosin staining, as well as immunofluorescence staining, was accomplished on the tissue sections. Representative images of the colon and ileum were captured using an optical microscope. Furthermore the length of the villus and the depth of the crypt of the ileum were measured at three different sites of each sample using Nano measure software 1.2.0. Average values of villus length and crypt depth were calculated for statistical analysis.
2.11. Periodic acid Schiff (PAS) staining
Mouse distal colon tissues were fixed in 4% polyformaldehyde. Paraffin-embedded sections (5 μm thick) were prepared for pathological examination. To visualize mucus-containing goblet cells, PAS staining was performed. The goblet cell number with mucus per intestinal villus was detected and analyzed.
2.12. RT-qPCR analysis
Colonic tissues’ total RNA was isolated using the Trizol reagent. The RNA was further reverse transcribed into cDNA using the HiScript III RT SuperMix Kit, as per the provided instructions. Real-time fluorescence quantitative polymerase chain reaction (RT-PCR) was performed to quantify the expression levels of target genes, including GAPDH, Muc2, Muc3, Claudin-1, Occludin, Zo-1, c-Kit, SCF, AQP8, and AQP4, with GAPDH as the internal reference gene for normalization.
2.13. Western blot
Total protein was extracted from 40 mg of colon tissues from mice using RIPA buffer, following the established protocol previously described.20 For western blot analysis, the laboratory employed the detailed procedure as per standard practice. The antibodies used in this study included mucin 2 (MUC2) and GAPDH. To separate proteins, we prepared 10% sodium dodecyl sulfate-polyacrylamide gel (SDS-PAGE) stacking and separation gels, facilitating electrophoretic separation for MUC2 assessment. Protein bands were visualized using the ECL Western blotting substrate, and the ChemiDoc Touch System (Biorad, California, USA) was utilized to detect the protein bands.
2.14. 16S rDNA sequencing and bioinformatics analysis
Total DNA was extracted from fecal samples collected on the 13th day using a QIAamp DNA Stool Mini Kit (Qiagen, Hilden, Germany). The extracted DNA was subjected to 16S rDNA sequencing using a previously established method.21 The obtained sequencing data were analyzed on the Majorbio cloud platform. Alpha-diversity measures, such as the Shannon index, Simpson index, Chao1 index, and Ace index, were calculated to assess the gut microbiota diversity. Beta-diversity was evaluated using partial least squares discriminant analysis (PLS-DA) based on the OTU level. Bacterial community composition at phylum, family, and genus levels was visualized through barplot and heatmap analyses. Additionally, linear discriminant analysis effect size (LEfSe) was performed to identify differentially abundant bacteria among groups. Finally, a heatmap was generated to visualize the correlation between microbial species and metabolic indicators.
2.15. Metabolite profiling analysis
Three replicates from MRS and CFS of L. acidipiscis YJ5 were subjected to metabolomics analysis using a previously reported method.21 Metabolite extraction and UPLC-MS were performed, involving the use of a methanol
:
acetonitrile solution for sample extraction and subsequent sonication and protein precipitation steps. The supernatant was collected, dried under nitrogen, and analyzed using a Waters UPLC system coupled with a Q Exactive HF-X mass spectrometer. The resulting raw data were processed using Progenesis QI software and analyzed on the Majorbio Cloud platform.
2.16. Statistical analysis
Statistical analysis was performed using GraphPad Prism 9.0 (GraphPad Software Inc., San Diego, CA, USA). The data in the manuscript described the values as mean ± SEM. Statistical significance was evaluated using either one-way ANOVA or two-way ANOVA, followed by Tukey's post hoc test.
3. Results
3.1.
In vitro probiotic properties of L. acidipiscis YJ5
Lactobacillus is known for its claimed probiotic properties, including GI tolerance (GIT), adhesion rate, antibacterial activity, and aggregation activity. In this study, we tested these probiotic properties of the newly discovered strain L. acidipiscis YJ5, and the results are shown in Fig. 1A–H. In terms of GIT, after simulated gastric digestion, the survival rate of the positive control bacteria LGG was higher than that of L. acidipiscis YJ5 (P < 0.05, Fig. 1A). However, in simulated intestinal fluid, L. acidipiscis YJ5 showed higher tolerance than LGG (P < 0.01, Fig. 1A). In terms of integrated GI transport, L. acidipiscis YJ5 was more tolerant than LGG, but the difference was not significant (P > 0.05, Fig. 1A). The rate of adhesion of L. acidipiscis YJ5 to HT-29 cells was 76%, which was not significantly different from LGG with strong adhesion ability (P > 0.05, Fig. 1B). Fig. 1C shows the ability of L. acidipiscis YJ5 to inhibit pathogens. Compared with LGG, L. acidipiscis YJ5 showed higher bacteriostasis against Gram-negative bacteria E. coli and S. typhimurium (P < 0.05, Fig. 1C), while lower bacteriostatic ability against Gram-positive bacteria S. aureus (P < 0.05, Fig. 1C). In terms of agglutination, we measured autoaggregation and coaggregation with four pathogens. After 10 h of aggregation, the autoaggregation rate of L. acidipiscis YJ5 was significantly higher than that of LGG (P < 0.05, Fig. 1D), and at 22 h, the advantage of L. acidipiscis YJ5 over LGG was more significant (P < 0.001, Fig. 1D). The coaggregation between L. acidipiscis YJ5, and E. coli and S. aureus was higher than that of LGG at 8 h (P < 0.05, Fig. 1E and F). At 18 h, the coaggregation between L. acidipiscis YJ5 and L. monocytogenes was higher than that of LGG (P < 0.05, Fig. 1H). These findings suggest that L. acidipiscis YJ5 has excellent anti-pathogenic bacteria ability, which is similar to or even better than LGG in vitro. Our previous study has demonstrated that strains with good in vitro probiotic properties could promote intestinal peristalsis.18 Here, we have shown that L. acidipiscis YJ5 possesses favorable in vitro probiotic properties, suggesting that it may help relieve constipation.
3.2.
L. acidipiscis YJ5 could promote intestinal peristalsis in two zebrafish models
Zebrafish, with the advantages of high genetic similarity to humans, a small size and convenience for a large number of experiments, was chosen as an in vivo experimental model of L. acidipiscis YJ5 for relieving constipation. In this study, we used two zebrafish models. One model was used to observe the time of peristaltic peaks under Lop modeling, while the other model was used to observe the rate of Nile red excretion in the intestine without Lop modeling. Lop modeling was not performed in the second model because the polarity of Nile red and Lop is similar, which could result in false positive results.
The experimental design for observing the intestinal peristalsis peak in zebrafish is shown in Fig. 1I. The results of the first zebrafish model are shown in Fig. 1J and K. Compared to the normal group, the time of first peristaltic peak occurrence in the LOP group was significantly longer, indicating the successful construction of constipation models. The first peristaltic peak appeared at 37 s in the normal control group, while the peristaltic wave peak in the LOP group appeared at 45 s. After intervention with the positive drug Dom, the first peristaltic wave peak appeared at 38 s, which was significantly shorter than that in the LOP group. However, LGG had no significant effect on the peristaltic peak time, consistent with the findings reported by Lu et al.22 The peristaltic function of the model group was restored to varying degrees by immersing different concentrations of L. acidipiscis YJ5 (108 CFU mL−1 and 107 CFU mL−1). The effect of L. acidipiscis YJ5 on the peristaltic peak showed a dose effect. At a concentration of 107 CFU mL−1, L. acidipiscis YJ5 significantly shortened the peak time of intestinal peristalsis, and the first peristaltic wave peak appeared at 39 s, which was not significantly different from the CON group. This suggests that bowel movements were restored to normal levels. At a concentration of 108 CFU mL−1, the peristaltic peak of the YJ5 group advanced to 33 s, faster than the normal group. These results suggest that L. acidipiscis YJ5 can promote intestinal peristalsis in the constipation zebrafish model.
The experimental design for observing the fluorescence intensity of the zebrafish gut is shown in Fig. 1L. In this zebrafish model, Nile red filled the zebrafish intestines after 16 hours, starting from 0 h intervention. After 24 h, Nile red fluorescence decreased with fecal excretion, indicating the excretion rate of feces (Fig. 1M and N). The positive drug Dom significantly improved the fluorescence intensity reduction rate compared to the CON group. The LGG group did not show a significant difference compared to the CON group. Both 108 CFU mL−1 and 107 CFU mL−1L. acidipiscis YJ5 treatment increased the fluorescence intensity reduction rate compared to the CON group, with 108 CFU mL−1 having the most significant effect, equivalent to DOM (P < 0.0001, Fig. 1M). These findings confirm the ability of L. acidipiscis YJ5 to promote intestinal peristalsis.
3.3.
L. acidipiscis YJ5 could relieve constipation in mice
In the zebrafish model, L. acidipiscis YJ5 was found to promote intestinal peristalsis. Then, the constipation-relieving ability of L. acidipiscis YJ5 was evaluated in mice. The experimental design is shown in Fig. 2A. There was no significant difference in energy intake among the three experimental groups (Fig. 2B). However, weight gain was significantly lower in the LOP group than that of the CON group, and this phenomenon was reversed by L. acidipiscis YJ5 intervention (Fig. 2C). The YJ5 group also demonstrated a significant reduction of 39.29% in the time to the first black stool defecation compared to the LOP group (P < 0.0001, Fig. 2D), along with a significant increase of 33.27% in the number of fecal pellets within a 5-hour period (P < 0.001, Fig. 2E). Although there was no significant difference in wet and dry fecal weight (Fig. 2F and G) between the LOP group and YJ5 group, the fecal water content of the YJ5 group was significantly increased (P < 0.0001, Fig. 2H). Additionally, the YJ5 group exhibited a significant increase in the GI transit rate of 46.48% in constipated mice (P < 0.01, Fig. 2I). The corresponding picture of small bowel propulsion is shown in Fig. 2J. These results indicate that L. acidipiscis YJ5 has a significant impact on improving the fecal characteristics and intestinal motility in constipated mice.
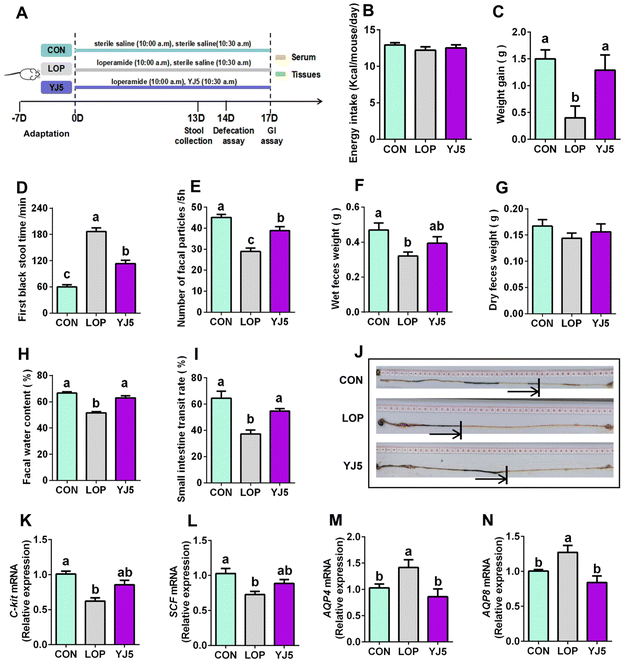 |
| Fig. 2
L. acidipiscis YJ5 intervention improved defecation function in constipated mice. (A) Experimental design, (B) energy intake, (C) weight gain, (D) time to the first black stool defecation, (E) number of fecal particles, (F) fecal wet weight, (G) fecal dry weight, (H) fecal water content, (I) small intestine propulsion rate and (J) experimental picture. The relative mRNA expression of (K) c-Kit, (L) SCF, (M) AQP4 and (N) AQP8. Data represent mean ± SEM ((B) to (I): n = 6; (K) to (N): n = 6). The significant difference between the groups was denoted by different letters (p < 0.05). | |
To explore the mechanism by which L. acidipiscis YJ5 relieves constipation, we investigated its effects on GI motility, specifically myoelectric motility. The slow wave, which represents the starting potential of myoelectric activity and is critical for smooth muscle contraction,23 was examined. We found that SCF and c-Kit gene expression levels were significantly downregulated in the Lop-induced constipation model (Fig. 2K and 2L, P < 0.0001 for c-Kit and P < 0.01 for SCF). However, as shown in Fig. 2K and L, constipated mice treated with L. acidipiscis YJ5 did not show significant upregulation of c-Kit and SCF gene expression compared to the LOP group (P > 0.05). This suggests that YJ5 may alleviate constipation through mechanisms other than increasing the number of ICCs via the c-Kit/SCF signaling pathway.
One of the main symptoms of constipation is the hardening of stool due to the reduced water content. In our study, constipated mice showed a significant reduction in fecal water content, which was improved by treatment with L. acidipiscis YJ5. This improvement may be attributed to the restoration of deteriorated water–electrolyte metabolism, including levels of AQP4/8. Our qPCR results showed that the mRNA expressions of AQP4 and AQP8 were significantly upregulated (P < 0.05) in constipated mice, as shown in Fig. 2M and N. However, after treatment with L. acidipiscis YJ5, the gene expression levels of AQP4 and AQP8 were significantly downregulated compared to the LOP group (P < 0.01), as shown in Fig. 2M and N. This indicates that L. acidipiscis YJ5 may alleviate constipation by regulating water–electrolyte metabolism.
3.4.
L. acidipiscis YJ5 enhanced the intestinal mucosal barrier in constipated mice
The relief of constipation and restoration of the fecal water content are often associated with a healthy intestinal barrier.24 In terms of intestinal morphology, Lop affected the villous morphology of the ileum with the intestinal wall of the colon (Fig. 3A and B). The ileal villi were significantly decreased (P < 0.0001, Fig. 3C) and the crypt depth became significantly shallower (P < 0.01, Fig. 3D) in the LOP group than that of the CON group. However, the YJ5 group showed a longer villus length and deeper crypt depth in the microscopy images of mice, as shown in Fig. 3A. Compared to the LOP group, the YJ5 group exhibited a significant increase in villus length (P < 0.0001) as shown in Fig. 3C, and a significant increase in crypt depth (P < 0.0001) as shown in Fig. 3D. Additionally, as shown in Fig. 3B and E, the thickness of the colon muscle layer was significantly thinner in the Lop-induced constipation model (P < 0.0001), but this phenomenon was reversed by the treatment with L. acidipiscis YJ5 (P < 0.0001).
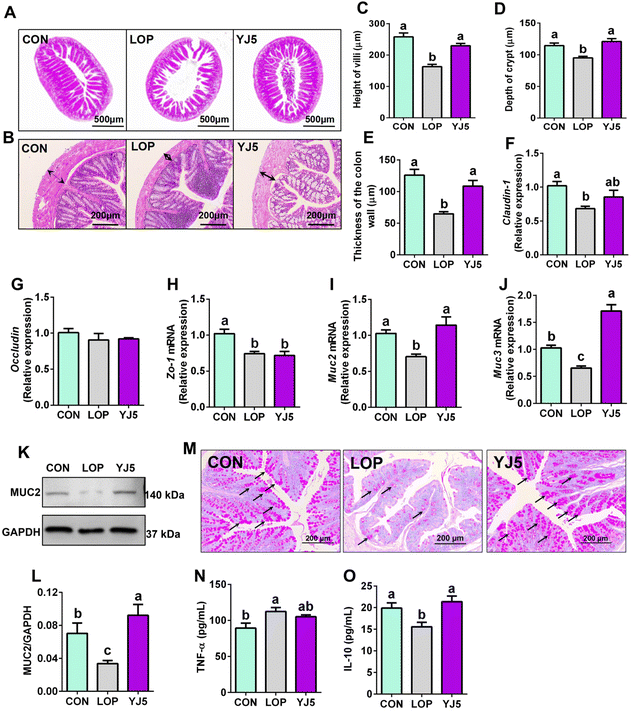 |
| Fig. 3
L. acidipiscis YJ5 enhanced the intestinal mucosal barrier in constipated mice. Histopathological analysis on (A) ileum and (B) colon, (C) height of villi, (D) depth of crypt, and (E) thickness of the colon wall. The relative mRNA expression of (F) Claudin-1, (G) Occludin, (H) Zo-1, (I) Muc2 and (J) Muc3. (K) Western blot analysis of MUC2 and (L) densitometric quantification. (M) PAS staining of goblet cells (the areas stained pink are the goblet cells, which are partially indicated by the black arrow). The levels of (N) TNF-α and (O) IL-10. Data represent mean ± SEM (n = 6). The significant difference between the groups was denoted by different letters (p < 0.05). | |
Regarding the intestinal barrier, the YJ5 group did not exhibit significant effects on the relative mRNA expression of Occludin and Zo-1 (P > 0.05, Fig. 3F and G). However, L. acidipiscis YJ5 supplementation significantly increased the relative mRNA expression of both Muc2 and Muc3 compared to the LOP group (P < 0.0001, P < 0.0001), as shown in Fig. 3I and J. The analysis of WB of protein expressions of MUC2 is shown in Fig. 3K. The protein level of MUC2 was significantly decreased in the LOP group compared to the CON group (P < 0.05, Fig. 3L), and treatment with L. acidipiscis YJ5 reversed this change (P < 0.01, Fig. 3L). This WB result was consistent with the qPCR findings. Since mucin is primarily secreted by goblet cells, we stained goblet cells. After LOP modeling, the number of goblet cells decreased. However, the number of goblet cells recovered after YJ5 treatment, as shown in Fig. 3M.
Upon analyzing the histological findings, the LOP group showed significantly higher levels of TNF-α compared to the CON group (P < 0.05), as shown in Fig. 3N. This indicates the presence of inflammation in the intestines due to constipation. Remarkably, the administration of L. acidipiscis YJ5 substantially reduced the TNF-α level in the colon. Furthermore, the levels of IL-10 were significantly reduced in the LOP group compared to the CON group (P < 0.05), but they were no longer significantly different from the CON group (P > 0.05), as shown in Fig. 3O. These results suggest that L. acidipiscis YJ5 also exhibits anti-inflammatory effects in constipated mice.
3.5. Effect of L. acidipiscis YJ5 on fecal microbiota in constipated mice
A total of 738 OTUs were found in the three groups, with the least (629) and the most (668) being detected in the CON and LOP groups, respectively. The YJ5 group had 664 OTUs (Fig. 4A). To show that the microbiota structure of each group varied, divergence and community composition were estimated by principal component analysis (PLS-DA). The microflora from the CON group was separated from that of the LOP group. However, the gut microbiota from the YJ5 group was close and partially overlapped with the CON group (Fig. 4B). In terms of α-diversity, the Simpson's diversity parameter was increased by the intervention of YJ5 compared to the LOP group (P < 0.05, Fig. 4C). However, the Shannon, Chao1, and Ace diversity results showed non-significant differences among the groups (P > 0.05), as shown in Fig. 4D–F.
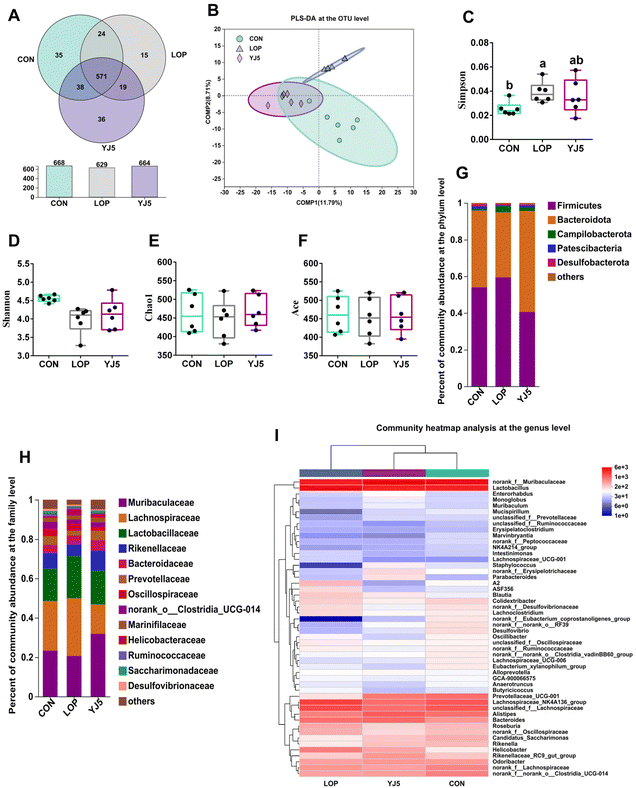 |
| Fig. 4
L. acidipiscis YJ5 altered the structure of the intestinal microbiota. (A) OTU Venn diagram between treatments. β-Diversity: (B) PLS-DA on the OTU level. α-Diversity: (C) Simpson index, (D) Shannon index, (E) Chao1's index, and (F) Ace index. Relative abundance of the gut microbiota at the (G) phylum level and (H) family level. (I) Community heatmap analysis at the genus level. Data are expressed as mean ± SEM, n = 6. The significant difference between the groups was denoted by different letters (p < 0.05). | |
The taxon-based analysis provides insights into the structural composition of the studied groups. At the phylum level, the predominant phyla observed across all groups were Firmicutes, Bacteroidota, Campylobacterota, Patescibacteria, and Desulfobacteria (Fig. 4G). At the family level, Muribaculaceae was enriched in the YJ5 group, as shown in Fig. 4H. The abundances of 30 genera were significantly different among the experimental groups. As shown in Fig. 4I, most of the changes observed in the mice of the LOP group were statistically suppressed after treatment with L. acidipiscis YJ5. Clustering results showed that the genus level in the YJ5 group was more similar to that of the CON group.
The effect of L. acidipiscis YJ5 on the flora of constipated mice was investigated using LEfSe analysis, as shown in Fig. 5A. The YJ5 group exhibited an increased abundance of g_Parabacteroide, g_norank_f_Muribaculaceae, and g_Prevotellaceae_UCG-001 (P < 0.01, P < 0.05, P < 0.05), while g_Helicobacter decreased compared to the LOP group, as shown in Fig. 5C–F. Spearman correlation analysis revealed potential links between environmental factors and YJ5-induced microflora changes, as shown in Fig. 5B. The results showed that the enrichment of g_norank_f_Muribaculaceae was not significantly correlated with the first black stool time, TNF-α, and AQP4/8, but was positively correlated with IL-10 and muc2/3. g_Prevotellaceae_UCG-001 was negatively correlated with the first black stool time, AQP4/8, and TNF-α, but was positively correlated with IL-10, GI, the fecal water content, and muc2. On the other hand, the down-regulated bacteria g_Helicobacter were negatively correlated with IL-10, GI, and the fecal water content, and positively correlated with TNF-α and AQP4, as shown in Fig. 5B.
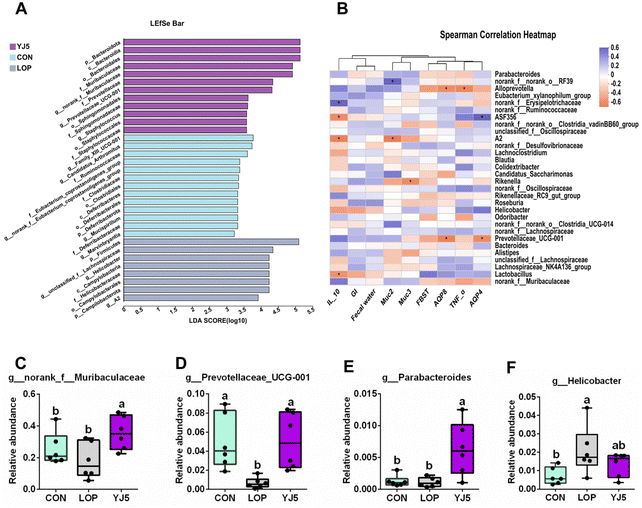 |
| Fig. 5
L. acidipiscis YJ5 changed the composition of the intestinal microbiota. (A) LEfSe analysis of the four experimental groups (log LDA > 2.0), (B) a heat map of the correlation between the gut microbiota and significant altered phenotypes of constipation. The relative abundances of (C) g_norank_f_Muribaculaceae, (D) g_Prevotellaceae_UCG-001, (E) g_Parabacteroide, and (F) g_Helicobacter. Data are expressed as mean ± SEM, n = 6. The significant difference between the groups was denoted by different letters (P < 0.05). | |
3.6. Metabolites of L. acidipiscis YJ5 promoting peristalsis
To determine whether L. acidipiscis YJ5 functions through its own substances or secreted substances, we used the zebrafish model to investigate whether the cell-free supernatant (CFS) and cell-free extract (CFE) of L. acidipiscis YJ5 promoted peristalsis, as shown in Fig. 6. We used the same concentration of MRS medium as a negative control for CFS, and the same concentration of PBS as a negative control for CFE. Firstly, we explored the maximum tolerated dose of CFS, MRS, CFE, and PBS in zebrafish. The results showed that zebrafish could survive when the concentration of CFS and MRS was less than 1% (Fig. 6A). The different dose effects of YJ5's CFS on the intestinal peristaltic peak of zebrafish are shown in Fig. 6B. There was no significant difference between the MRS groups and LOP groups at different concentrations (P > 0.05), suggesting that MRS at 1%, 0.5%, and 0.1% doses did not affect zebrafish peristalsis. While a significant difference between the YJ5 group and CON group in the peak emergence time of the first peristaltic peak of zebrafish was not found (P > 0.05), which means that when the concentration of YJ5's CFS reached 1%, the peak emergence time of the first peristaltic peak of zebrafish was restored to normal levels. However, no effect was observed when the dose of YJ5's CFS was 0.5% and 0.1%. The results of the fluorescence intensity reduction rate also indicated that 1% CFS of YJ5 significantly promoted peristalsis (Fig. 6C). In terms of the results of CFE, we observed that zebrafish survived at concentrations of up to 20% CFE of L. acidipiscis YJ5 and 50% PBS (Fig. 6D). Therefore, the concentration of CFE in the following determination did not exceed 20%. The effect of CFE on zebrafish peristalsis was evaluated also through two methods, as shown in Fig. 6E and F. However, no significant effect of YJ5's CFE on intestinal peristalsis was observed through both methods (P > 0.05). Therefore, we concluded that L. acidipiscis YJ5 promotes peristalsis through its own secreted substances and metabolites rather than its own intracellular substances.
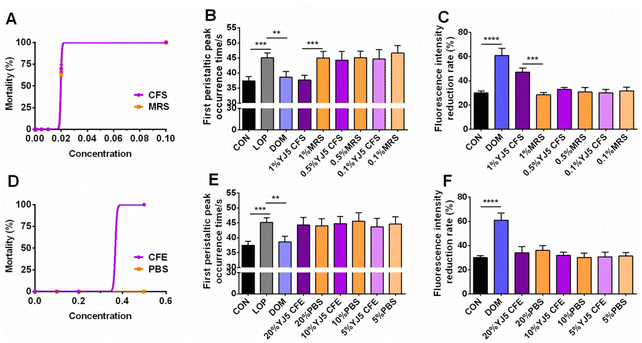 |
| Fig. 6 Mortality of zebrafish in different concentration samples and effects of YJ5CFS/CFE on zebrafish peristalsis. (A) Mortality of zebrafish in the CFS and MRS, (B) first peristaltic peak occurrence time in the YJ5 CFS, (C) fluorescence intensity reduction rate in the YJ5 CFS, (D) mortality of zebrafish in the CFE and PBS, (E) first peristaltic peak occurrence time in the YJ5 CFE, and (F) fluorescence intensity reduction rate in the YJ5 CFE. Data are expressed as mean ± SD, n = 60 (* P < 0.05, ** P < 0.01, *** P < 0.001, and **** P < 0.0001). | |
To investigate the mechanisms underlying the effects of L. acidipiscis YJ5, we conducted non-targeted LC-MS/MS analysis to identify potential anti-constipation metabolites. The principal component analysis (PCA) score plots in positive and negative ion modes showed clear separations between the YJ5's CFS and MRS groups (Fig. 7A and B). Our analysis revealed 959 and 1255 annotated metabolites in the YJ5 and MRS groups, respectively, in both positive and negative ion modes. Additionally, 8 and 32 metabolites were exclusively identified in the YJ5 group in positive and negative ion modes, respectively (Fig. 7C and D). We identified significantly differentially expressed metabolites (DEMs) between the YJ5 and MRS groups, primarily involving peptides, organic acids, nucleic acids, and carbohydrates based on KEGG database analysis. The key metabolic pathways affected by YJ5 included “amino acid metabolism” and “carbohydrate metabolism” (Fig. 7E). Notably, organic acids such as malic acid and vanillactic acid, as well as anti-inflammatory ingredients like heliangin and cordycepin, were enriched in the YJ5 group (Fig. 7F and Fig. S1A†). Our findings suggest that L. acidipiscis YJ5 contributes to anti-constipation effects through the production of diverse active ingredients (Fig. 8).
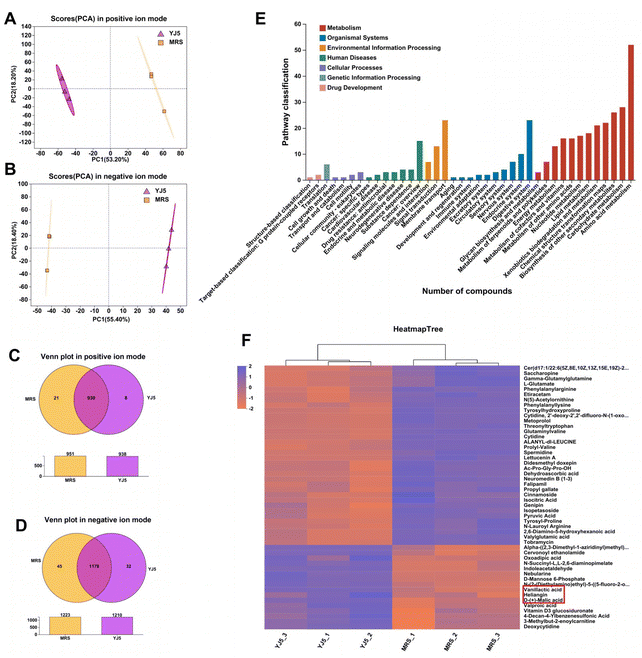 |
| Fig. 7 Differential metabolites identified in comparison between YJ5CFS and MRS groups. (A) Scores (PCA) in the positive ion mode, (B) scores (PCA) in the negative ion mode, (C) Venn plot in the positive ion mode, (D) Venn plot in the negative ion mode, (E) KEGG pathway of differentially expressed metabolites (DEMs), and (F) clustering analysis of DEMs. | |
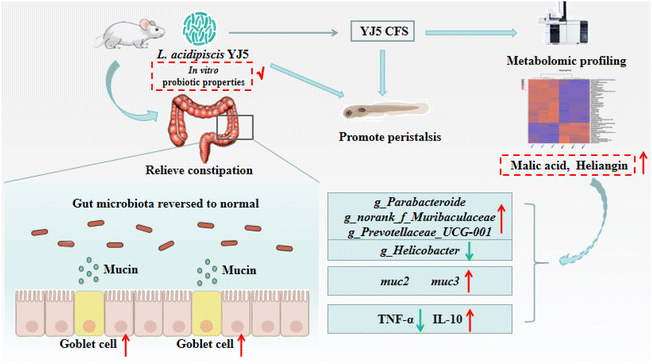 |
| Fig. 8 Schematic of the molecular mechanism of the constipation-relieving effect of L. acidipiscis YJ5. | |
4. Discussion
Constipation is a prevalent GI issue, and microbial therapies with Bifidobacterium and Lactobacillus have been extensively studied. In this study, we demonstrated for the first time that L. acidophilus YJ5, isolated from fermented traditional Chinese fruits and vegetables, has excellent constipation-relieving abilities in zebrafish and mouse models.
In the zebrafish experiment, when the concentration of YJ5 reached ∼107 CFU mL−1, its peristalsis-promoting effect was comparable to that of Dom (positive control). At a concentration of ∼108 CFU mL−1, YJ5 outperformed Dom in promoting peristalsis. Previous research by Lu et al.22 demonstrated that even the most effective probiotics for peristalsis promotion, such as B. lactis BB12 and L. paracasei X11, did not match the peristalsis-promoting ability of Dom (at a concentration of 50 μg mL−1) when used at a bacterial solution concentration of ∼108 CFU mL−1. In comparison to LGG (positive strain), L. acidophilus YJ5 exhibited a better survival rate in the GI tract, as well as higher self-agglutination and cross-agglutination rates with pathogenic bacteria (Fig. 1A and D–H). It also demonstrated stronger bacteriostatic effects against Gram-negative bacteria, including E. coli and S. typhimurium. These in vitro probiotic properties were associated with constipation. For example, higher survival rates are often the basis for many probiotics, because survival in the GI tract imparts the capability to strain to function in the gut.25 It was found that E. coli was significantly more abundant in the chronic constipation group than in the control group.26 Porter et al.27 discovered the risk of chronic constipation attributed to non-typhoid Salmonella. In this study, the in vitro probiotic experiments revealed that L. acidophilus YJ5 could interact with pathogens, which may facilitate the elimination of pathogens from the body. Moreover, the CFS of L. acidophilus YJ5 exhibited inhibitory effects on both E. coli and S. typhimurium. Therefore, the beneficial intestinal peristalsis-promoting function may be attributed to the favorable probiotic properties observed during the in vitro study, and the CFS of L. acidophilus YJ5 may be the key substance.
The relief of constipation is often associated with a healthy intestinal barrier.24 Previous studies have shown that interventions targeting barrier functions can regulate intestinal fluid secretion and hydro-electrolytic balance, leading to increased excretion rates.28,29 Probiotic chocolate containing Streptococcus thermophilus MG510 and Lactiplantibacillus plantarum LRCC5193 (LYC) has been reported to enhance intestinal motility and regulate the mRNA expression of colonic Zo-1 in constipated rats.30 Chitosan oligosaccharides have also been found to relieve constipation and up-regulate the expression of occludin and Claudin-1 genes.31 However, it was surprising that L. acidipiscis YJ5 did not up-regulate the genes involved in the mechanical barrier (Claudin-1, Occludin, and Zo-1) in this study. This may be because different probiotic strains may have varying effects on gene expression in the gut, and the specific characteristics and mechanisms of L. acidipiscis YJ5 might not directly influence the expression of these genes. MUC2, an important factor in the mucosal barrier, plays a role in collecting water molecules and forming a gel layer that covers the intestinal epithelial cell surface, acting as a strong barrier between intestinal contents and mucosal epithelial cells. A water-filled mucus layer in the intestines is crucial for smooth stool passage and easy evacuation.32,33 Interestingly, in contrast to the mechanical barrier genes, L. acidipiscis YJ5 significantly up-regulated the gene and protein expression involved in the mucosal barrier (muc2), suggesting that YJ5 supplementation strengthened the gut mucus barrier functions. The study's finding was consistent with the increased fecal water content and frequency of defecation observed with YJ5 supplementation in constipation. Previous studies have also reported an increase in colonic mucin with probiotics.34,35 Additionally, our findings revealed a significant reduction in goblet cell numbers after constipation induction, which was significantly increased following L. acidipiscis YJ5 intervention. Taken together, these results further demonstrate that L. acidipiscis YJ5 relieves constipation by increasing the number of goblet cells that secrete mucin.
Changes in the intestinal morphology after constipation can lead to mild inflammation and damage to the intestinal barrier. B. adolescentis has been shown to alleviate intestinal inflammation and improve the intestinal immune barrier by increasing the number of Tregs and the concentration of IL-10 in the lamina propria lymphocytes.36 Similarly, L. fermentum KBL375 has been shown to significantly decrease the proinflammatory cytokines IL-1β, IL-6, and TNF-α and significantly increase the anti-inflammatory cytokine IL-10 to reduce intestinal inflammation.37 Our study demonstrated that L. acidipiscis YJ5 increased the anti-inflammatory factor IL-10 and reduced the pro-inflammatory factor TNF-α. Therefore, our study suggests that preventing intestinal damage and reducing inflammation may also contribute to relieving constipation.
The gut microbiota has been shown to be involved in the pathogenesis of constipation.38 In this study, L. acidipiscis YJ5 improved the balance of gut flora and enriched the strains of g_norank_f_Muribaculaceae, Prevotellaceae-UCG-001, and g_Parabacteroide. Muribaculaceae and Prevotellaceae-UCG-001, which are considered beneficial bacteria, have been reported to increase in the reversal of Lop-induced constipation.39,40Parabacteroides is a Gram-negative bacterium that functions as a commensal symbiont, playing a role in promoting the health of the mucosal barrier and preventing the elevation of various proinflammatory cytokines.41,42 The study found that Helicobacter was significantly increased in the LOP group, while L. acidipiscis YJ5 could significantly down-regulate this bacterium. This result is consistent with the study by Hu et al.,43 which found that mulberry could alleviate diphenoxylate-induced constipation by reducing the richness of Helicobacter, which was negatively associated with fecal defecation. Our correlation analysis also found that Helicobacter was positively and negatively correlated with the first black stool time and intestine transit rate (Fig. 5B). As mentioned above, L. acidipiscis YJ5 intervention could extensively modulate the gut microbiota composition in Lop-induced constipation mice.
Probiotics typically exhibit their bioactivities through their fermentation products or their own components.44 In our study, we used a zebrafish model to determine the anti-constipation ability of the fermented liquid CFS of L. acidipiscis YJ5 and its cell lysate CFE. The results verified that L. acidipiscis YJ5 played a role in relieving constipation which is mainly attributed to its fermentation products. Metabolomic analysis revealed that the main metabolic pathways of L. acidipiscis YJ5 are “amino acid metabolism” and “carbohydrate metabolism”. The most differentially expressed metabolites (DEMs) were enriched in peptides, organic acids, nucleic acids, and carbohydrates. The CFS of L. acidipiscis YJ5 contained high amounts of organic acids, including malic acid and vanillactic acid, which may help relieve constipation. Choi et al. reported that vanillic acid could significantly enhance the amplitude and frequency of contractile responses of the isolated rat colon in vitro.45 In Na et al.'s study,46 malic acid significantly increased the relief of Lop-induced constipation in rats and the secretion of mucin in the colon. Therefore, the effect of increasing goblet cells to secrete mucin of L. acidipiscis YJ5 in our study may be mainly related to its organic acid metabolites. In addition, bio-fermented malic acid could modulate the cecum microbiota of broilers.47 Interestingly, the genera of Helicobacter were decreased in the group fed the diet supplemented with bio-fermented malic acid.47 Our results also showed a decrease in Helicobacter in the YJ5 group, indicating that the fermentation product malic acid of L. acidipiscis YJ5 may modulate gut microbiota to play a role. Heliangin, another fermentation product of L. acidipiscis YJ5 could inhibit the expression levels of TNF-α, NO, IL-6, and PGE2 in macrophage cell lysate.48 Cordycepin, a metabolite that exhibited a substantial increase in YJ5's CFS compared to MRS, has been found to possess the ability to alleviate metabolic inflammation in mice fed a Western diet. The mechanism of action involves targeting the composition of microbiota and the intestinal barrier integrity.49 Therefore, the role of L. acidipiscis YJ5 in improving the mucosal barrier, alleviating metabolic inflammation, and modulating gut microbiota to relieve constipation is mainly due to its anti-constipation metabolites (especially malic acid, vanillactic acid, heliangin, and cordycepin).
In conclusion, our study introduces a novel probiotic strain, L. acidipiscis YJ5, which exhibits potential properties that could be possibly used in alleviating constipation. This study provides guidelines as a foundation to further explore the potential of probiotics in preventing and treating constipation.
Author contributions
Fei Shen: conceptualization, methodology, investigation, formal analysis, and writing – original draft. Qianqian Wang: conceptualization, investigation, and writing. Sami Ullah: investigation. Ya Pan: investigation. Minjie Zhao: investigation. Jing Wang: investigation. Ming Chen: investigation. Fengqin Feng: investigation, conceptualization, supervision, and funding acquisition. Hao Zhong: conceptualization, project administration, and funding acquisition.
Conflicts of interest
The authors of this paper confirm that they have no conflicts of interest to disclose.
Acknowledgements
We extend our heartfelt appreciation to Hangzhou Kangyuan Food Science & Technology Co. for their generous financial support. We thank Yingniang Li from the Core Facilities, Zhejiang University School of Medicine for their technical support. This study received funding from the National Natural Science Foundation of China (grant number 32072224) and the Natural Science Foundation of Zhejiang Province (grant numbers 32001693 and LQ21C200007).
References
- A. Aboubakr and M. S. Cohen, Functional bowel disease, Clin. Geriatr. Med., 2021, 37, 119–129 CrossRef PubMed.
- R. J. Meinds, M. M. van Meegdenburg, M. Trzpis and P. M. A. Broens, On the prevalence of constipation and fecal incontinence, and their co-occurrence, in the Netherlands, Int. J. Colorectal Dis., 2017, 32, 475–483 CrossRef PubMed.
- T. Bokic, M. Storr and R. Schicho, Potential Causes and Present Pharmacotherapy of Irritable bowel syndrome: an overview, Pharmacology, 2015, 96, 76–85 CrossRef CAS PubMed.
- R. Rexwinkel, C. M. A. de Bruijn, M. Gordon, M. A. Benninga and M. M. Tabbers, Pharmacologic treatment in functional abdominal pain disorders in children: a systematic review, Pediatrics, 2021, 147, e2020042101 CrossRef PubMed.
- E. Dimidi, S. M. Scott and K. Whelan, Probiotics and constipation: mechanisms of action, evidence for effectiveness and utilisation by patients and healthcare professionals, Proc. Nutr. Soc., 2020, 79, 147–157 CrossRef PubMed.
- H. J. Kim, M. I. V. Roque, M. Camilleri, D. Stephens, D. D. Burton, K. Baxter, G. Thomforde and A. R. Zinsmeister, A randomized controlled trial of a probiotic combination VSL# 3 and placebo in irritable bowel syndrome with bloating, Neurogastroenterol. Motil., 2005, 17, 687–696 CrossRef CAS PubMed.
- P. J. Whorwell, L. Altringer, J. Morel, Y. Bond, D. Charbonneau, L. O'Mahony, B. Kiely, F. Shanahan and E. M. M. Quigley, Efficacy of an encapsulated probiotic Bifidobacterium infantis, 35624 in women with irritable bowel syndrome, Am. J. Gastroenterol., 2006, 101, 1581–1590 CrossRef PubMed.
- L. O'Mahony, J. McCarthy, P. Kelly, G. Hurley, F. Y. Luo, K. S. Chen, G. C. O'Sullivan, B. Kiely, J. K. Collins, F. Shanahan and E. M. M. Quigley,
Lactobacillus and Bifidobacterium in irritable bowel syndrome: Symptom responses and relationship to cytokine profiles, Gastroenterology, 2005, 128, 541–551 CrossRef PubMed.
- T. Li, Q. Yan, Y. Wen, J. Liu, J. Sun and Z. Jiang, Synbiotic yogurt containing konjac mannan oligosaccharides and Bifidobacterium animalis ssp. lactis BB12 alleviates constipation in mice by modulating the stem cell factor (SCF)/c-Kit pathway and gut microbiota, J. Dairy Sci., 2021, 104, 5239–5255 CrossRef CAS PubMed.
- W. Linlin, C. Mao, W. Jialiang, Y. Qiangqing, W. Gang, Z. Hao, Z. Jianxin and C. Wei,
Bifidobacterium longum relieves constipation by regulating the intestinal barrier of mice, Food Funct., 2022, 13, 5037–5049 RSC.
- W. Linlin, H. Lujun, X. Qi, J. Tian, F. Shuguang, W. Gang, Z. Jianxin, Z. Hao and C. Wei,
Bifidobacteria exert species-specific effects on constipation in BALB/c mice, Food Funct., 2017, 8, 3587–3600 RSC.
- Y. Luo, B.-C. Ma, L.-K. Zou, J.-G. Cheng, Y.-H. Cai, J.-P. Kang, B. Li, X.-H. Gao, P. Wang and J.-J. Xiao, Identification and characterization of lactic acid bacteria from forest musk deer feces, Afr. J. Microbiol. Res., 2012, 6, 5871–5881 Search PubMed.
- T. Thamacharoensuk, M. Taweechotipatr, A. Kajikawa, S. Okada and S. Tanasupawat, Induction of cellular immunity interleukin-12, antiproliferative effect, and related probiotic properties of lactic acid bacteria isolated in Thailand, Ann. Microbiol., 2017, 67, 511–518 CrossRef CAS.
- S. Ren, X. Zhang, H. Guan, L. Wu, M. Yu, D. Hou, Y. Yan and X. Fang,
Lactobacillus acidipiscis induced regulatory gamma delta T cells and attenuated experimental autoimmune encephalomyelitis, Front. Immunol., 2021, 12, 623451 CrossRef CAS PubMed.
- A. Idris, D. C. Mihora and K. Kaye, Loperamide abuse cardiotoxicity. Should loperamide still be an over the counter medication?, Am. J. Emerg. Med., 2018, 36, 1716.e1–1716.e3 CrossRef PubMed.
- K. D. Katz, R. D. Cannon, M. D. Cook, A. Amaducci, R. Day, J. Enyart, G. Burket, L. Porter, T. Roach, J. Janssen and K. E. Williams, Loperamide-induced torsades de pointes: a case series, J. Emerg. Med., 2017, 53, 339–344 CrossRef PubMed.
- J. A. J. Schuurkes and J. M. Vannueten, Domperidone improves myogenically transmitted antroduodenal coordination by blocking dopaminergic receptor-sites, Scand. J. Gastroenterol., 1984, 19, 101–110 CAS.
- F. Shen, J. Zhuang, Q. Wang, J. Zhang, T. Liu, S. Ruan, J. Du, H. Zhong, M. Zhao and F. Feng, Screening of novel probiotics with intestinal peristalsis-promoting potential based on in vitro and in vivo investigations, Food Biosci., 2023, 53, 102681 CrossRef CAS.
- K. A. F. de Alvarenga, E. K. Sacramento, D. V. Rosa, B. R. Souza, V. B. de Rezende and M. A. Romano-Silva, Effects of antipsychotics on intestinal motility in zebrafish larvae, Neurogastroenterol. Motil., 2017, 29, e13006 CrossRef PubMed.
- J. Zhou, S. Y. Guo, Y. Zhang and C. Q. Li, Human prokinetic drugs promote gastrointestinal motility in zebrafish, Neurogastroenterol. Motil., 2014, 26, 589–595 CrossRef CAS PubMed.
- F. Shen, J. Zhuang, Q. Wang, J. Zhang, Y. Huang, Q. Mo, M. Zhao, J. Wang, H. Zhong and F. Feng, Enhancement in the metabolic profile of sea buckthorn juice via fermentation for its better efficacy on attenuating diet-induced metabolic syndrome by targeting gut microbiota, Food Res. Int., 2022, 162, 111948 CrossRef CAS PubMed.
- Y. Lu, J. Zhang, H. Yi, Z. Zhang and L. Zhang, Screening of intestinal peristalsis-promoting probiotics based on a zebrafish model, Food Funct., 2019, 10, 2075–2082 RSC.
-
K. M. Sanders, in Smooth Muscle Spontaneous Activity: Physiological and Pathological Modulation, ed. H. Hashitani and R. J. Lang, 2019, vol. 1124, pp. 3–46 Search PubMed.
- B. Qi, Y. Zhang, D. Ren, X. Qin, N. Wang and X. Yang, Fu brick tea alleviates constipation via regulating the aquaporins-mediated water transport system in association with gut microbiota, J. Agric. Food Chem., 2023, 71, 3862–3875 CrossRef CAS PubMed.
- S. Mojtaba, M.-K. Tayebe, A.-S. Mahboubeh, J. Mandana, A.-R. Sahar, A. Alieh and F. Hamid, Probiotic and antioxidant properties of selenium-enriched Lactobacillus brevis LSe isolated from an Iranian traditional dairy product, J. Trace Elem. Med. Biol., 2017, 40, 1–9 CrossRef PubMed.
- I. L. Khalif, E. M. M. Quigley, E. A. Konovitch and I. D. Maximova, Alterations in the colonic flora and intestinal permeability and evidence of immune activation in chronic constipation, Dig. Liver Dis., 2005, 37, 838–849 CrossRef CAS PubMed.
- C. K. Porter, D. Choi, B. Cash, M. Pimentel, J. Murray, L. May and M. S. Riddle, Pathogen-specific risk of chronic gastrointestinal disorders following bacterial causes of foodborne illness, BMC Gastroenterol., 2013, 13, 46 CrossRef PubMed.
- L. Wang, M. Chai, J. Wang, Q. Yu, G. Wang, H. Zhang, J. Zhao and W. Chen,
Bifidobacterium longum relieves constipation by regulating the intestinal barrier of mice, Food Funct., 2022, 13, 5037–5049 RSC.
- N. H. Crooks, C. Snaith, D. Webster, F. Gao and P. Hawkey, Clinical review: Probiotics in critical care, Crit. Care, 2012, 16, 237 CrossRef.
- J. Y. Eor, P. L. Tan, S. M. Lim, D. H. Choi, S. M. Yoon, S. Y. Yang and S. H. Kim, Laxative effect of probiotic chocolate on loperamide-induced constipation in rats, Food Res. Int., 2019, 116, 1173–1182 CrossRef CAS PubMed.
- X. Zhang, H. Yang, J. Zheng, N. Jiang, G. Sun, X. Bao, A. Lin and H. Liu, Chitosan oligosaccharides attenuate loperamide-induced constipation through regulation of gut microbiota in mice, Carbohydr. Polym., 2021, 253, 117218 CrossRef CAS PubMed.
- T. Pelaseyed, J. H. Bergstrom, J. K. Gustafsson, A. Ermund, G. M. H. Birchenough, A. Schutte, S. van der Post, F. Svensson, A. M. Rodriguez-Pineiro, E. E. L. Nystrom, C. Wising, M. E. V. Johansson and G. C. Hansson, The mucus and mucins of the goblet cells and enterocytes provide the first defense line of the gastrointestinal tract and interact with the immune system, Immunol. Rev., 2014, 260, 8–20 CrossRef CAS PubMed.
- G. Javitt, L. Khmelnitsky, L. Albert, L. S. Bigman, N. Elad, D. Morgenstern, T. Ilani, Y. Levy, R. Diskin and D. Fass, Assembly mechanism of mucin and von willebrand factor polymers, Cell, 2020, 183, 717–729 CrossRef CAS PubMed.
- C. Caballero-Franco, K. Keller, C. De Simone and K. Chadee, The VSL#3 probiotic formula induces mucin gene expression and secretion in colonic epithelial cells, Am. J. Physiol.: Gastrointest. Liver Physiol., 2007, 292, G315–G322 CrossRef CAS PubMed.
- R. Li, S. Xu, B. Li, B. Zhang, W. Chen, D. Dai and Z. Liu, Gut indigenous Ruminococcus gnavus alleviates constipation and stress-related behaviors in mice with loperamide-induced constipation, Food Funct., 2023, 14, 5702–5715 RSC.
- L. Fan, Y. Qi, S. Qu, X. Chen, A. Li, M. Hendi, C. Xu, L. Wang, T. Hou, J. Si and S. Chen,
B. adolescentis ameliorates chronic colitis by regulating Treg/Th2 response and gut microbiota remodeling, Gut Microbes, 2021, 13, e1826746 CrossRef PubMed.
- Y. J. Jang, W.-K. Kim, D. H. Han, K. Lee and G. Ko,
Lactobacillus fermentum, species ameliorate dextran sulfate sodium-induced colitis by regulating the immune response and altering gut microbiota, Gut Microbes, 2019, 10, 696–711 CrossRef CAS PubMed.
- N. Prochazkova, G. Falony, L. O. Dragsted, T. R. Licht, J. Raes and H. M. Roager, Advancing human gut microbiota research by considering gut transit time, Gut, 2023, 72, 180–191 CrossRef CAS PubMed.
- L. Yang, Y. Wan, W. Li, C. Liu, H.-F. Li, Z. Dong, K. Zhu, S. Jiang, E. Shang, D. Qian and J. Duan, Targeting intestinal flora and its metabolism to explore the laxative effects of rhubarb, Appl. Microbiol. Biotechnol., 2022, 106, 1615–1631 CrossRef CAS PubMed.
- J.-E. Kim, Y.-J. Choi, S.-J. Lee, J.-E. Gong, Y.-J. Jin, S.-H. Park, H.-S. Lee, Y.-W. Choi, J.-T. Hong and D.-Y. Hwang, Laxative effects of phlorotannins derived from Ecklonia cava on loperamide-induced constipation in SD rats, Molecules, 2021, 26, 7209 CrossRef CAS PubMed.
- Y.-M. Chen, I. L. Wang, X.-Y. Zhu, W.-C. Chiu and Y.-S. Chiu, Red clover isoflavones influence estradiol concentration, exercise performance, and gut microbiota in female mice, Front. Nutr., 2021, 8, 623698 CrossRef PubMed.
- K. L. Arnolds and C. A. Lozupone, Striking a balance with help from our little friends - how the gut microbiota contributes to immune homeostasis, Yale J. Biol. Med., 2016, 89, 389–395 CAS.
- T.-G. Hu, P. Wen, H.-Z. Fu, G.-Y. Lin, S.-T. Liao and Y.-X. Zou, Protective effect of mulberry (Morus atropurpurea) fruit against diphenoxylate-induced constipation in mice through the modulation of gut microbiota, Food Funct., 2019, 10, 1513–1528 RSC.
- A. Orlando, M. G. Refolo, C. Messa, L. Amati, P. Lavermicocca, V. Guerra and F. Russo, Antiproliferative and proapoptotic effects of viable or heat-killed Lactobacillus paracasei IMPC2.1 and Lactobacillus rhamnosus GG in HGC-27 gastric and DLD-1 colon cell lines, Nutr. Cancer, 2012, 64, 1103–1111 CrossRef CAS PubMed.
- C.-Y. Choi, S.-S. Cho and I.-S. Yoon, Hot-water extract of the branches of Hovenia dulcis Thunb. (Rhamnaceae) ameliorates low-fiber diet-induced constipation in rats, Drug Des., Dev. Ther., 2018, 12, 695–703 CrossRef CAS PubMed.
- J.-R. Na, E. Kim, C.-S. Na and S. Kim, Citric Acid-Enriched Extract of Ripe Prunus mume (Siebold) Siebold & Zucc. induces laxative effects by regulating the expression of aquaporin 3 and prostaglandin E-2 in rats with loperamide-induced constipation, J. Med. Food, 2022, 25, 12–23 CrossRef CAS PubMed.
- K. Qiu, W. He, H. Zhang, J. Wang, G. Qi, N. Guo, X. Zhang and S. Wu, Bio-fermented malic acid facilitates the production of high-quality chicken via enhancing muscle antioxidant capacity of broilers, Antioxidants, 2022, 11, 2309 CrossRef CAS PubMed.
- X. Lu, L. Min, J. Wei, H. Gou, Z. Bao, J. Wang, Z. Wang, Y. Huang and B. An, Heliangin inhibited lipopolysaccharide-induced inflammation through signaling NF-kappa B pathway on LPS-induced RAW 264.7 cells, Biomed. Pharmacother., 2017, 88, 102–108 CrossRef CAS PubMed.
- J. Chen, M. Wang, P. Zhang, H. Li, K. Qu, R. Xu, N. Guo and H. Zhu, Cordycepin alleviated metabolic inflammation in Western diet-fed mice by targeting intestinal barrier integrity and intestinal flora, Pharmacol. Res., 2022, 178, 106191 CrossRef CAS PubMed.
|
This journal is © The Royal Society of Chemistry 2024 |
Click here to see how this site uses Cookies. View our privacy policy here.